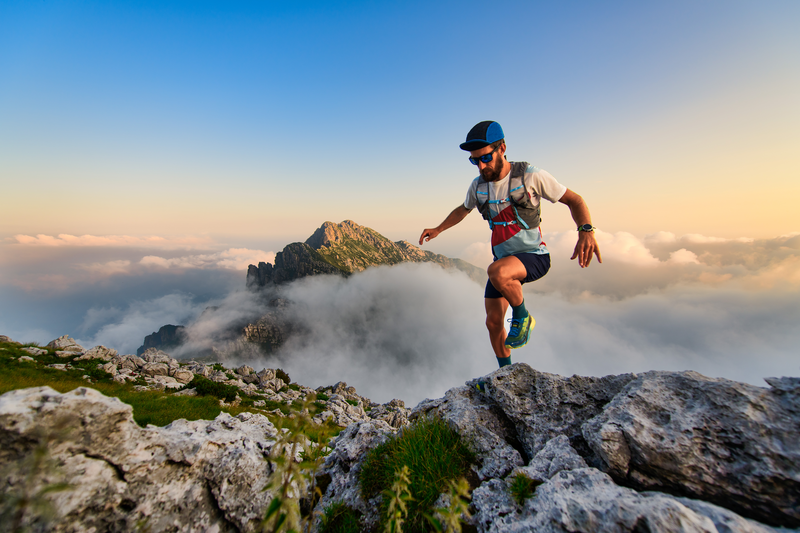
94% of researchers rate our articles as excellent or good
Learn more about the work of our research integrity team to safeguard the quality of each article we publish.
Find out more
SYSTEMATIC REVIEW article
Front. Neurol.
Sec. Neurocritical and Neurohospitalist Care
Volume 16 - 2025 | doi: 10.3389/fneur.2025.1557820
The final, formatted version of the article will be published soon.
You have multiple emails registered with Frontiers:
Please enter your email address:
If you already have an account, please login
You don't have a Frontiers account ? You can register here
As Severe traumatic brain injury (TBI) is a major cause of pediatric morbidity and mortality. The clinical benefits of intracranial pressure (ICP) monitoring in pediatric TBI remain debated. This meta-analysis aims to assess the impact of ICP monitoring on outcomes in children with severe TBI.Following PRISMA guidelines, a comprehensive search was conducted in PubMed, EMBASE, Cochrane Library, and Web of Science. Studies comparing pediatric severe TBI patients with and without ICP monitoring were included. Primary outcomes included in-hospital mortality and complications, while secondary outcomes included craniotomy/craniectomy rate, length of hospital stay and ICU stay, mechanical ventilation duration, and medical costs. Quality assessment was performed using the Methodological Index for Non-Randomized Studies (MINORS) for cohort studies. The weighted mean difference (WMD) for continuous variables and odds ratio (OR) for dichotomous variables were calculated, along with 95% confidence intervals (CIs). Meta-analysis was performed using RevMan 5.4.1 software.Eight studies (12,987 patients) were included. ICP monitoring showed no significant impact on overall in-hospital mortality (OR, 1.14; p = 0.65), though propensity score matching (PSM) studies indicated a lower mortality rate with ICP monitoring (OR, 0.62; p = 0.005). However, ICP monitoring was associated with higher risks of infection-related (OR, 7.21; p<0.001) and respiratory complications (OR, 5.79; p<0.001), thromboembolic events (OR, 5.37; p<0.001), increased craniotomy/craniectomy rates (OR, 2.34; P=0.01), longer hospital (OR, 12.00; p<0.001) and ICU stays (OR, 7.82; p<0.001), extended mechanical ventilation durations (OR, 5.82; p< 0.001), and higher medical costs (WMD, 10.49; p = 0.006). This meta-analysis found no overall reduction in in-hospital mortality with ICP monitoring in pediatric severe TBI, potentially due to baseline severity imbalances in retrospective studies. However, PSM studies suggest a mortality benefit, indicating that ICP monitoring may be effective when confounding is minimized. Increased complication risks, longer hospital/ICU stays, prolonged ventilation, and higher costs were associated with monitoring, though these may reflect injury severity rather than monitoring itself. Given the limitations of this study, these findings should be interpreted cautiously.
Keywords: Intracranial pressure monitoring, Severe traumatic brain injury, pediatric, Mortality, complications, Meta-analysis
Received: 09 Jan 2025; Accepted: 28 Feb 2025.
Copyright: © 2025 Xue, Zhang and Liu. This is an open-access article distributed under the terms of the Creative Commons Attribution License (CC BY). The use, distribution or reproduction in other forums is permitted, provided the original author(s) or licensor are credited and that the original publication in this journal is cited, in accordance with accepted academic practice. No use, distribution or reproduction is permitted which does not comply with these terms.
* Correspondence:
Yan Liu, School of Nursing, West China Hospital, Sichuan University, Chengdu, 610041, Sichuan Province, China
Disclaimer: All claims expressed in this article are solely those of the authors and do not necessarily represent those of their affiliated organizations, or those of the publisher, the editors and the reviewers. Any product that may be evaluated in this article or claim that may be made by its manufacturer is not guaranteed or endorsed by the publisher.
Research integrity at Frontiers
Learn more about the work of our research integrity team to safeguard the quality of each article we publish.