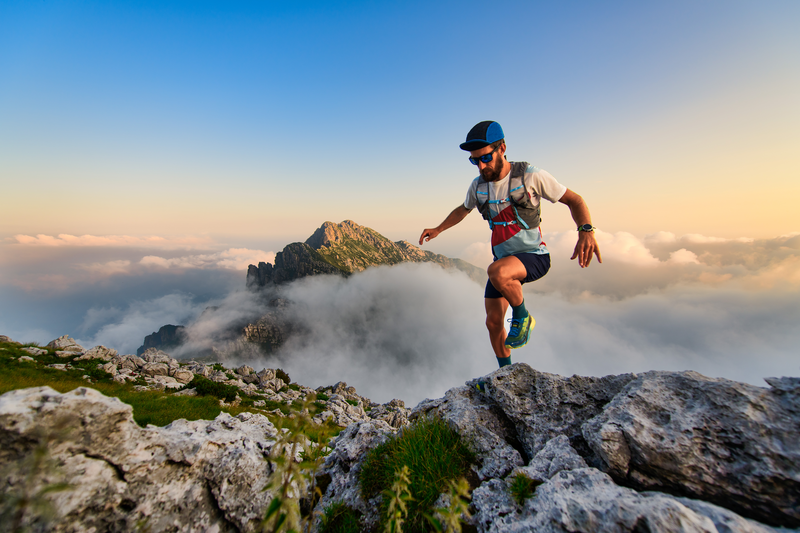
94% of researchers rate our articles as excellent or good
Learn more about the work of our research integrity team to safeguard the quality of each article we publish.
Find out more
ORIGINAL RESEARCH article
Front. Neurol.
Sec. Dementia and Neurodegenerative Diseases
Volume 16 - 2025 | doi: 10.3389/fneur.2025.1555411
This article is part of the Research Topic Post-Stroke Cognitive Decline and Dementia: Unraveling Mechanisms, Models, and Biomarkers View all 9 articles
The final, formatted version of the article will be published soon.
You have multiple emails registered with Frontiers:
Please enter your email address:
If you already have an account, please login
You don't have a Frontiers account ? You can register here
Objective: This study aimed to apply network pharmacology to identify the active components and key targets of Tongqiao Huashuan Granules in vascular dementia (VaD) and to evaluate its effects on autophagy in hippocampal neurons of VaD rats through animal testing.This study first employed network pharmacology (NP) to identify potential components and pathway targets for THg intervention in VaD. A modified two-vessel occlusion (2-VO) method was subsequently analyzed to establish a VaD rat model. Following the interventions, the spatial learning and memory abilities of the rats were assess using a water maze experiment.Morphological and structural changes in neuronal cells within the CA1 region of the rat hippocampus were examined using hematoxylin and eosin (HE) staining. Immunohistochemistry was utilized to assess the proportions of Beclin1-positive and LC3-positive cells in the CA1 region of each rat group, while performed Western blot analysis was conducted to measure protein expression levels of PI3K, p-PI3K, AKT, p-AKT, mTOR, p-mTOR, Beclin1, and LC3 in the hippocampal tissue of the rats.Results: A total of 76 active components were identified through network pharmacology analysis, with notable components including β-sitosterol, kaempferol, and cinnamophilin. In total, 825 key targets were identified, including IL1B, AKT1, JAK1, and MAPK3. THg and VaD shared common genes. The Gene Ontology (GO) functional enrichment analysis yielded a total of 569 items (BP: 366, CC: 97, MF: 106). KEGG pathway enrichment analysis identified 143 signaling pathways, including TNF, MAPK, AGE-RAGE, and PI3K/Akt pathways. Subsequent validation experiments demonstrated that THg enhanced the learning and memory abilities of VaD rats, improve the morphology of neuronal cells in the CA1 region of the hippocampus, and decreasing the proportion of Beclin1-and LC3-positive cells in this region. Additionally, THg was shown to enhance the expression levels of p-PI3K, p-AKT, and p-mTOR proteins while reducing the expression levels of Beclin1 and LC3 proteins.This study represents the first investigation into the effects of THg intervention in VaD, indicating that its mechanism may involve inhibiting autophagy in hippocampal neurons through activation of the PI3K/Akt-mTOR signaling pathway.
Keywords: Tongqiao Huashuan Granules, Vascular Dementia, PI3K/AKT/mTOR, Autophagy, mechanism of action
Received: 04 Jan 2025; Accepted: 05 Mar 2025.
Copyright: © 2025 Jiang, Yu, Cai, Tang, Zhu, Yao and Wang. This is an open-access article distributed under the terms of the Creative Commons Attribution License (CC BY). The use, distribution or reproduction in other forums is permitted, provided the original author(s) or licensor are credited and that the original publication in this journal is cited, in accordance with accepted academic practice. No use, distribution or reproduction is permitted which does not comply with these terms.
* Correspondence:
Jing Cai, The First Affiliated Hospital, Guizhou University of Traditional Chinese Medicine, Guiyang, Guizhou Province, China
Disclaimer: All claims expressed in this article are solely those of the authors and do not necessarily represent those of their affiliated organizations, or those of the publisher, the editors and the reviewers. Any product that may be evaluated in this article or claim that may be made by its manufacturer is not guaranteed or endorsed by the publisher.
Research integrity at Frontiers
Learn more about the work of our research integrity team to safeguard the quality of each article we publish.