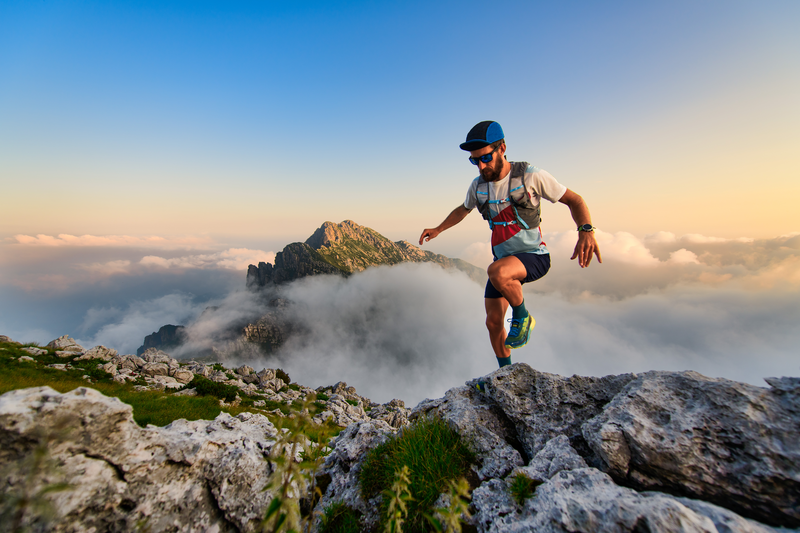
94% of researchers rate our articles as excellent or good
Learn more about the work of our research integrity team to safeguard the quality of each article we publish.
Find out more
SYSTEMATIC REVIEW article
Front. Neurol.
Sec. Neurotrauma
Volume 16 - 2025 | doi: 10.3389/fneur.2025.1546080
The final, formatted version of the article will be published soon.
You have multiple emails registered with Frontiers:
Please enter your email address:
If you already have an account, please login
You don't have a Frontiers account ? You can register here
Traumatic brain injury (TBI) is a leading cause of death and disability globally and is associated with long-term cognitive and neurobehavioural deficits. Methylphenidate has been proposed to address these lasting symptoms, however comprehensive evidence is lacking. This systematic review aimed to assess the effects of methylphenidate on multiple cognitive and neurobehavioural domains in adults with TBI. The search conducted across five databases yielded 1,019 results, of which 25 were relevant to this review. Meta-analyses were conducted where homogenous data was available. Significant results favouring methylphenidate were recorded by meta-analyses for one of five cognition outcome measures (Trail Making Test A) (p=0.005, CI [-5.19, -0.91]), as well as the depression domain (p<0.00001, CI [-0.78, -0.39]) and the fatigue domain (p<0.00001, CI [-0.98, -0.67]). Insufficient data was available in the aggression, apathy, agitation, memory, motor function, post-concussion syndrome and sleep domains for inclusion in meta-analysis. Qualitative review of evidence in these domains found limited and mixed evidence on the efficacy of methylphenidate, though significant benefits have been demonstrated in these various domains in small, randomised studies. Eleven of the 25 studies were judged as containing some to high risk of bias. However, this review identified supportive evidence for the beneficial effects of methylphenidate to improve depression and fatigue in adults with TBI, with some possible benefits for cognition and other symptoms. Heterogeneity was high and risk of bias was variable across studies, somewhat limiting credibility of results. Methylphenidate may enhance the ongoing care of TBI patients, by addressing neurobehavioral and cognitive symptoms simultaneously. Further large-scale and high-quality clinical trials evaluating a comprehensive range of possible benefits to symptoms should be conducted to more conclusively elucidate the potential of methylphenidate for clinical efficacy in TBI.
Keywords: Methylphenidate, Cognition, Depression, Fatigue, traumatic brain injury Italian (Italy) Field Code Changed Formatted: Italian (Italy) Italian (Italy) Field Code Changed Formatted: Italian (Italy)
Received: 16 Dec 2024; Accepted: 24 Feb 2025.
Copyright: © 2025 Rees, Saunders, Krynicki, Belli, Ahmed, Di Pietro and Stevens. This is an open-access article distributed under the terms of the Creative Commons Attribution License (CC BY). The use, distribution or reproduction in other forums is permitted, provided the original author(s) or licensor are credited and that the original publication in this journal is cited, in accordance with accepted academic practice. No use, distribution or reproduction is permitted which does not comply with these terms.
* Correspondence:
Jemima Rees, Neuroscience and Ophthalmology, Department of Inflammation and Ageing, School of Infection, Inflammation and Immunology, College of Medicine and Health, University of Birmingham, Birmingham, United Kingdom
Disclaimer: All claims expressed in this article are solely those of the authors and do not necessarily represent those of their affiliated organizations, or those of the publisher, the editors and the reviewers. Any product that may be evaluated in this article or claim that may be made by its manufacturer is not guaranteed or endorsed by the publisher.
Research integrity at Frontiers
Learn more about the work of our research integrity team to safeguard the quality of each article we publish.