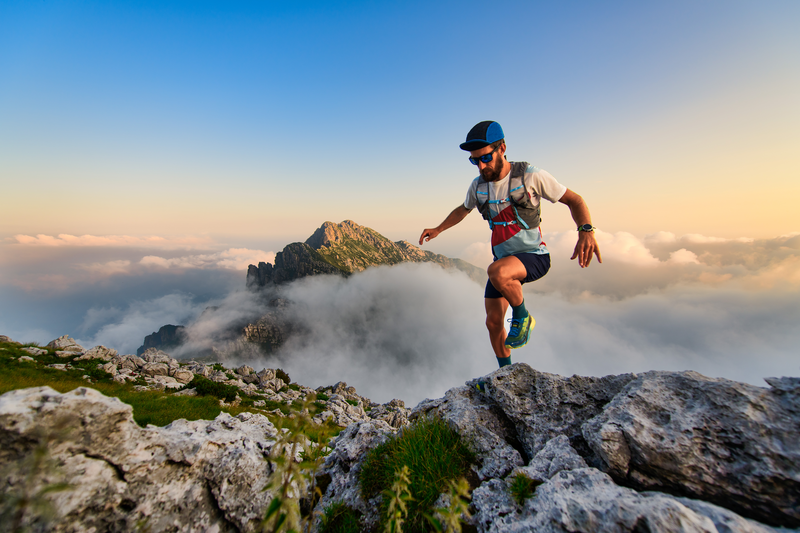
95% of researchers rate our articles as excellent or good
Learn more about the work of our research integrity team to safeguard the quality of each article we publish.
Find out more
ORIGINAL RESEARCH article
Front. Neurol. , 08 April 2025
Sec. Movement Disorders
Volume 16 - 2025 | https://doi.org/10.3389/fneur.2025.1541970
This article is part of the Research Topic Integrating Technology into Advanced Parkinson's Disease Management: From Screening and Evaluation to Treatment and Prevention View all 4 articles
Background: Lateral trunk flexion (LTF) is a common symptom of Parkinson’s disease (PD). The sensory re-weighting system and sensory-motor function are poor in patients with PD and LTF, and this may cause gait impairment. However, the specific characteristics of gait impairment in patients with PD and LTF remain unclear. The aim of this study was to compare the characteristics of the gait functional domains between participants with PD with and without LTF.
Methods: Fifty-eight patients with PD and Hoehn–Yahr grade 2–3 LTF were divided into two groups: the LTF group (n = 22) and the No LTF group (n = 36). The Movement Disorder Society Unified Parkinson’s Disease Rating Scale (MDS-UPDRS)-Part III score and subjective visual vertical (SVV) angle were measured. The participants walked with a motion sensor on a straight 20 m path at a comfortable speed. Fifteen gait variables (10 gait cycles) were evaluated and categorized into pace, rhythm, asymmetry, variability, and postural control functional domains and were compared between groups.
Results: The LTF angle, SVV angle; MDS-UPDRS-Part III total, rigidity, and axial scores; and the coefficients of variance for step length, step time, and stance time were significantly higher in the LTF group than in the No LTF group. No other significant differences were observed between the groups.
Conclusion: Participants with PD and Hoehn–Yahr severity 2–3 LTF had greater gait variability than those without LTF, but maintained similar pace, rhythm, asymmetry, and postural control domains. Patients with PD and LTF may develop abnormal neural networks causing greater gait variability.
Postural abnormalities are typical symptoms of Parkinson’s disease (PD). Lateral trunk flexion (LTF) causes lower-back pain and poor postural balance (1–3). Furthermore, the mortality rate is higher in patients with PD and LTF than in those without LTF. Therefore, LTF is the main therapeutic target of physical therapy and requires early intervention (4). It has been suggested that LTF is caused by a poor postural control system that maintains postural equilibrium and orientation and requires complex interactions between the motor, sensory, and cognitive systems (1). Previous studies have revealed that LTF is associated with muscle rigidity (5) and vertical perception based on vestibular information, defined as the subjective visual vertical (SVV) angle (6, 7). The SVV angle is also associated with the Movement Disorder Society Unified Parkinson’s Disease Rating Scale (MDS-UPDRS)-Part III and the Hoehn–Yahr Scale (8). Therefore, poor vertical visual perception may contribute to postural instability. Furthermore, the processing of vestibular information, especially on the ipsilateral lateral trunk flexion side, has been shown to be impaired in individuals with LTF relative to individuals without LTF (9). Vestibular and proprioceptive dysfunction worsen with the progression of parkinsonism (10, 11). Finally, the impaired integration of sensory and motor functions, specifically a poor sensory re-weighting system, may contribute to postural instability in patients with PD and LTF (1, 2, 12).
However, the gait impairment features associated with LTF and the factors related to gait impairment have not yet been fully revealed. Lord et al. reported that gait function consists of five functional domains: pace, rhythm, asymmetry, variability, and postural control (13). They also reported that patients with PD and postural and gait impairments have deficits in the pace, variability, and asymmetry domains relative to those of healthy older adults (14, 15). However, they did not divide patients with PD into LTF and No LTF groups. Thus, the characteristics of the five gait functional domains in the LTF group were not reported. Other previous studies have analyzed some variables corresponding to each functional domain: pace (gait speed), rhythm (double-support time), and asymmetry (step-time asymmetry, swing-time asymmetry), but have found no significant differences in any variables between the LTF and No LTF groups (2, 3, 16). Central pattern generators generate limb movement patterns and regulate rhythms (17). Thus, the pace, rhythm, and asymmetry domains are controlled by central pattern generators. Geroin and Tramonti suggested that individuals with PD and LTF sustain a gait control system based on central pattern generators (2, 16). In contrast, the variability domain is thought to require the integration of sensory information and is thus controlled by the central nervous system involving the spinal cord and cerebral cortex (18, 19). Individuals with LTF show significantly worse executive, attentional, and language functions than those without LTF (20). Additionally, individuals with LTF have reduced functional connectivity in the left insula, bilateral supplementary motor area, and right middle frontal gyrus compared with those without LTF (21). There may be cognitive-motor dysfunction and different processes controlling gait through abnormal neural networks in patients with PD and LTF. Thus, individuals with LTF may exhibit greater gait variability and instability.
This study compared the characteristics of the functional gait domains between individuals with PD and LTF and those without LTF. We hypothesized that gait variability and instability would be greater in the LTF group. Clarifying the characteristics of gait disorders in individuals with LTF will deepen our understanding of the pathophysiology of LTF and serve as the basis for prioritizing treatment in functional areas that are prone to decline.
This was a cross-sectional study. Sufficient rest periods were provided between assessments to minimize the effects of fatigue.
Fifty-eight patients with PD (mean age: 76.1 ± 8.7 years; disease duration: 9.2 years) participated in this study. The PD diagnosis process followed the Parkinson’s disease Clinical Practice Guidelines 2018 and cases were classified as clinically probable PD, which has a sensitivity and specificity of over 80%. Only patients with a confirmed diagnosis were included in the study. The inclusion criteria were as follows: (1) the ability to walk independently for >20 m and (2) a classification of 2 to 3 on the Hoehn–Yahr severity scale. The exclusion criteria were as follows: (1) medication-induced dyskinesia or (2) visual impairment hindering accurate measurement of the SVV angle.
The participants were divided into two groups: the LTF group (n = 22; mean age: 74.5 ± 6.7 years, disease duration: 9.3 years), and the No LTF group (n = 36; mean age: 76.8 ± 9.4 years, disease duration: 9.1 years) (Table 1). We captured images showing the lateral view of patients with PD with a variable combination of trunk flexion sides and degrees, calculated according to validated software-based methods. ImageJ software1 was used to measure the relevant angles. The LTF angle was defined as the angle between a vertical line and a line along with the fifth lumbar spinous process to the seventh cervical spinous process. Patients with PD who exhibited LTF of more than 5 degrees were defined as the LTF group in the upright position (22). After receiving approval from the Ethics Review Committee of the Hokkaido Neurological Hospital (FY2022, No. 4), an opt-out document was published on the hospital’s official website.
The MDS-UPDRS-part III total score and sub-scores for each element were measured. The sub-scores were calculated for tremor (items 15–18), rigidity (item 3), bradykinesia (items 2, 4–8, and 14), and axial scores (items 1 and 9–13) (23, 24).
The bucket method was used to assess the SVV angles (25). Each participant was instructed to sit in a chair with a backrest. They were then instructed to maintain their head and body in a vertical position. The examiner placed a bucket in front of each participant’s face. The participants reported when they perceived that the stick presented at the bottom of the bucket was vertical. The SVV angle was defined as the angle between the vertical axis and the stick. The initial stick position deviated 25° from the vertical axis, and the rotational direction was randomly either clockwise or counterclockwise. If lateral bending of the head or any other disturbances occurred, the SVV measurement was repeated. A positive SVV angle was determined to be in the same direction as the lateral flexion of the trunk. The average angles of four trials were calculated.
A six-channel sensory system, Physilog®5 (Gait up, Renens, Switzerland; sampling frequency, 128 Hz), was attached to the outside of the participants’ shoes. The participants walked along a straight 14 m path at a comfortable speed. To eliminate the effects of acceleration and deceleration during gait initiation and termination, the analysis range for defining straight gait was the middle 10 m (Figure 1). Data from 10 gait cycles were analyzed. Fifteen gait variables were calculated: the step length (SL), step velocity (SV), step time (ST), stance time (STT), swing time (SWGT), symmetry index of step length (SL_SI), symmetry index of the step velocity (SV_SI), symmetry index of the step time (ST_SI), symmetry index of the stance time (STT_SI), symmetry index of the swing time (SWGT_SI), coefficient of variation in step length (SL_CV), coefficient of variation in step velocity (SV_CV), coefficient of variation in step time (ST_CV), coefficient of variation in stance time (STT_CV), and coefficient of variation in swing time (SWGT_CV). The symmetry index was calculated as previously described (26, 27). These gait variables were categorized into five functional domains according to Lord’s methodology: pace (SL, SV, SWGT_CV), rhythm (ST, STT, SWGT), asymmetry (ST_SI, STT_SI, SWGT_SI), variability (SL_CV, SV_CV, STT_SI, SWGT_SI), and postural control (SL_SI) (13).
Figure 1. Experimental setup: The participants walked at a self-selected walking speed along a 14 m walkway. A Physilog®5 motion sensor was attached to the outside of their shoes.
First, the Shapiro–Wilk test was performed to confirm normality, followed by either an unpaired t-test or Mann–Whitney U test, using the Bonferroni correction for between-group comparisons. The categorical variable (gender) was tested using the chi-squared (χ2) test. The effect size (Cohen’s d) was calculated. Spearman’s rank correlation coefficient was calculated to assess the association between LTF and SVV angles. JASP was used to perform all the statistical analyses at a significance level of 5%.
No significant between-group differences were found in age, disease duration, or Hoehn–Yahr scores (p = 0.85, d = 0.07; p = 0.84, d = 0.07; p = 0.12, d = 0.61, respectively; Table 1). Additionally, there was no significant difference in the sex distribution between the groups (p = 0.15). The LTF angle; the SVV angle; and MDS-UPDRS-part III total, rigidity, and axial scores were significantly higher in the LTF group than in the No LTF group (p < 0.001, d = 2.87; p < 0.001, d = 1.72; p < 0.001, d = 2.27; p < 0.001, d = 2.64; p < 0.001, d = 3.35, respectively; Table 1).
Figure 2 shows a radar chart illustrating the gait functional domain pattern of the LTF group, with values normalized to those of the No LTF group. Table 2 presents the mean values and standard deviation of the 15 gait variables for each group. ST_CV, STT_CV, and SL_CV were significantly different between groups (p < 0.001, d = 1.74; p < 0.001, d = 1.23; p < 0.001, d = 1.17, respectively; Table 2). No significant differences were observed in the other variables between the groups.
Figure 2. Ladar chart of gait function. This figure illustrates the developmental processes of walking variables in each group, normalized to the average values of the adult group as 100%. The variables included SL (step length), SV (step velocity), SW (step width), ST (step time), STT (stance time), SWGT (swing time), CV (coefficient of variation), and SI (symmetry index).
Although, no significant correlation between the SVV angle and LTF angle was observed in the No LTF group (ρ = 0.12 and p = 0.67, respectively), a significantly positive correlation between the SVV angle and LTF angle (ρ = 0.74 and p < 0.001, respectively) was observed in the LTF group (Figure 3).
Figure 3. Correlation between the lateral trunk flexion (LTF) angle and the subjective visual vertical (SVV) angle.
This study compared the characteristics of the functional gait domains between participants with PD and LTF and those without LTF. There were no significant differences between the groups in terms of age, disease duration, or Hoehn–Yahr score. Additionally, this study was designed to minimize the influence of fatigue. The LTF group maintained pace, rhythm, asymmetry, and postural control domains, but showed a decline in the variability domain, indicating that gait variability was greater in the LTF group than in the No LTF group.
The LTF group in this study also showed a significantly higher SVV angle, which indicated that they had abnormal vertical perception through the integration of vestibular and visual sensing relative to the vertical perception of those in the No LTF group (Table 1). Gait variability is influenced by the integration of sensory information in the cerebral cortex and spinal cord (18). Gait variability can be regarded as the final motor output based on various types of sensory feedback information (28). Individuals with PD and LTF have been shown to have sensory integration (visual, proprioceptive, and vestibular sensory) disabilities (1, 12) and visuospatial cognitive dysfunction (20, 29) compared to individuals with PD but no LTF. Kohsaka et al. (30) showed that the LTF and SVV angles are associated with hypofunction of the right inferior parietal lobule, superior parietal lobule, and superior temporal gyrus. These regions are the key cortical areas that integrate multisensory signals from the visual, vestibular, and somatosensory systems, which are necessary for postural control and visuospatial cognitive function (17). Additionally, it has been suggested that the control of gait variability is important for the interaction between the cerebral cortex, basal ganglia, and brainstem networks, which integrate motor, sensory, and cognitive systems, respectively (1). However, the interaction between the basal ganglia and cerebellum is disrupted in patients with PD and postural instability (31). The cerebellum is also involved in attentional function (32). There may be a different control process for controlling gait through abnormal neural networks compared with the control process in patients with PD and no LTF. Finally, this study found significantly higher MDS-UPDRS-Part III rigidity and axial scores in the LTF group (Table 1). These physical symptoms may also be related to the observed increase in gait variability (1). Therefore, it is suggested that the LTF group had an increased CV owing to declines in the sensory integration system, visuospatial cognitive function (33), and physical function.
Contrary to our hypothesis, no significant differences were observed between the groups in the postural control domain. This result was similar to those of previous studies, which also found no group differences in SL_SI (2, 16). Postural stability during walking is achieved through feed-forward and feedback postural control (28). Impaired anticipatory postural control in patients with PD occurs at Hoehn–Yahr stage 2 (34–36). Furthermore, postural reflex impairment in PD appears at Hoehn–Yahr stage 3. Therefore, both groups in this study may have had impaired postural control. Different postural control impairments between the two groups may become prominent from Hoehn–Yahr stage 4 and higher.
Postural sway during gait has been significantly correlated with variables in the pace and rhythm domains (37). Additionally, SL_SI, defined as the postural control domain in this study, may include factors in the pace and asymmetry domains. Therefore, no significant between-group differences were found in the postural control domain, as there were no differences in pace, rhythm, or asymmetry.
There were no significant differences in the pace, rhythm, or asymmetry domains between the groups (Table 2). This is consistent with the findings of previous studies (2, 16) and suggests that the pace, rhythm, and asymmetry domains construct factors associated with basic gait patterns and are controlled by central-pattern generators (17). Variables associated with pace and rhythm, such as walking speed, cadence, and stride, show pronounced impairments from Hoehn–Yahr Stage 4 and beyond (38). Additionally, several studies have reported that asymmetric features disappear at Hoehn–Yahr stage 2 (2, 15, 16). Thus, our results suggest that both groups at Hoehn–Yahr severity stages 2 and 3 may maintain the function of the central-pattern generator.
Parkinsonism progresses from one-sided to bilateral impairment and asymmetry features disappear at Hoehn–Yahr stage 2 (2, 15, 16); however, it should be noted that PD remains an asymmetrical disease. The large standard deviation of the symmetry index value may have hindered the detection of significant differences. The degree of asymmetry varied among individuals with PD, regardless of the presence or absence of LTF (Table 2). Patients with PD at Hoehn–Yahr severity stages 2–3 may exhibit various asymmetrical gait patterns. Therefore, both groups in this study may have had similar bilateral impairments and asymmetrical features could not be detected.
This study has several limitations. First, only SL_SI could be calculated for evaluation of the postural control domain because of the limitations of the Physilog®5 system. Lord et al. regarded SL_SI, step width, and the coefficient of variation of the step width as postural control domain values. To identify the detailed characteristics of postural control in individuals with PD and LTF, it will be necessary to include these variables in future studies. Second, although the SVV angle can be measured as an evaluation of vestibular dysfunction, this result involves subjective perception. Therefore, other factors, including cognitive function, may have influenced the SVV angle results. It is important to use objective evaluations, such as qualitative head-impulse tests and eye-movement measurements in conjunction with the SVV angle. Third, the results of this study may only be applicable to individuals with Hoehn–Yahr severity stages 2–3. To identify more detailed gait characteristics in the LTF group, further studies should include subjects with Hoehn–Yahr stages 1 and 4. Fourth, the two groups had a wide range of disease durations (1–22 years). Previous studies have focused on the factors that affect gait variability and found that aging, cognitive function, central nervous system disorders, fatigue, medication, sensory re-weighting ability, and visuospatial cognition have positive effects (28, 39, 40). Disease duration may be a key variable affecting the LTF angle, the SVV angle, and gait control. Further analysis based on disease duration is necessary. Fifth, this study did not assess the UPDRS-Part 2 score. Thus, we were unable to clearly distinguish between tremor-dominant and postural impairment and gait difficulty-dominant types in our patients. Finally, the two groups were divided into 5° LTF angle subgroups; however, subjects with a near threshold were included in both groups. To better understand the characteristics of both groups, further analyses with larger sample sizes and differentiation between subjects near and outside the boundary are necessary.
This study compared the characteristics of functional gait domains between individuals with PD and LTF and those without LTF. Gait variability was greater in participants with PD and LTF at Hoehn–Yahr stages 2–3 than in those without LTF. In contrast, patients with LTF and Hoehn–Yahr stages 2–3 maintained the pace, rhythm, asymmetry, and postural control domains. The results of this study suggest that, for patients with PD and LTF, it is crucial for physical therapists to implement therapeutic interventions aimed at enhancing their ability to control gait variability. It may be essential to address abnormal vertical perception and improve physical functions, such as rigidity. Highly challenging balance and gait training (41) and/or rigidity control therapy, such as botulinum toxin treatment, may be important. However, there is insufficient evidence to demonstrate that long-term botulinum toxin treatment improves gait function (42, 43).
The raw data supporting the conclusions of this article will be made available by the authors, without undue reservation.
The studies involving humans were approved by the Ethics Review Committee of Hokkaido Neurological Hospital (FY2022, No. 4). The studies were conducted in accordance with the local legislation and institutional requirements. The participants provided their written informed consent to participate in this study.
KO: Conceptualization, Methodology, Investigation, Data curation, Formal analysis, Writing – original draft, Writing – review & editing. HM: Conceptualization, Data curation, Investigation, Methodology, Writing – review & editing, Funding acquisition, Supervision. KN: Investigation, Methodology, Writing – review & editing. YN: Investigation, Methodology, Writing – review & editing. SH: Methodology, Investigation, Writing – review & editing. FM: Methodology, Investigation, Writing – review & editing.
The author(s) declare that financial support was received for the research and/or publication of this article. This study was supported by a Hokkaido Neurological Hospital operating grant and in part by a Japanese Grant-in-Aid for Scientific Research (HM: 24K02423).
We would like to thank Editage (www.editage.jp) for English language editing.
The authors declare that the research was conducted in the absence of any commercial or financial relationships that could be construed as a potential conflict of interest.
The author(s) declare that no Gen AI was used in the creation of this manuscript.
All claims expressed in this article are solely those of the authors and do not necessarily represent those of their affiliated organizations, or those of the publisher, the editors and the reviewers. Any product that may be evaluated in this article, or claim that may be made by its manufacturer, is not guaranteed or endorsed by the publisher.
1. Barone, P, Santangelo, G, Amboni, M, Pellecchia, MT, and Vitale, C. Pisa syndrome in Parkinson's disease and parkinsonism: clinical features, pathophysiology, and treatment. Lancet Neurol. (2016) 15:1063–74. doi: 10.1016/S1474-4422(16)30173-9
2. Geroin, C, Smania, N, Schena, F, Dimitrova, E, Verzini, E, Bombieri, F, et al. Does the Pisa syndrome affect postural control, balance, and gait in patients with Parkinson's disease? An observational cross-sectional study. Parkinsonism Relat Disord. (2015) 21:736–41. doi: 10.1016/j.parkreldis.2015.04.020
3. Geroin, C, Artusi, CA, Gandolfi, M, Zanolin, E, Ceravolo, R, Capecci, M, et al. Does the degree of trunk bending predict patient disability, motor impairment, falls, and Back pain in Parkinson's disease? Front Neurol. (2020) 11:207. doi: 10.3389/fneur.2020.00207
4. Laudisio, A, Lo Monaco, MR, Vetrano, DL, Pisciotta, MS, Brandi, V, Gemma, A, et al. Association of Pisa Syndrome with Mortality in patients with Parkinson's disease. J Am Med Dir Assoc. (2019) 20:1037–1041.e1. doi: 10.1016/j.jamda.2019.01.141
5. Su, PC, Tseng, HM, and Liou, HH. Postural asymmetries following unilateral subthalomotomy for advanced Parkinson's disease. Mov Disord. (2002) 17:191–4. doi: 10.1002/mds.1269
6. Scocco, DH, Wagner, JN, Racosta, J, Chade, A, and Gershanik, OS. Subjective visual vertical in Pisa syndrome. Parkinsonism Relat Disord. (2014) 20:878–83. doi: 10.1016/j.parkreldis.2014.04.030
7. Gandor, F, Basta, D, Gruber, D, Poewe, W, and Ebersbach, G. Subjective visual vertical in PD patients with lateral trunk flexion. Parkinsons Dis. (2016) 2016:1–4. doi: 10.1155/2016/7489105
8. Pereira, CB, Kanashiro, AK, Maia, FM, and Barbosa, ER. Correlation of impaired subjective visual vertical and postural instability in Parkinson's disease. J Neurol Sci. (2014) 346:60–5. doi: 10.1016/j.jns.2014.07.057
9. Vitale, C, Marcelli, V, Furia, T, Santangelo, G, Cozzolino, A, Longo, K, et al. Vestibular impairment and adaptive postural imbalance in parkinsonian patients with lateral trunk flexion. Mov Disord. (2011) 26:1458–63. doi: 10.1002/mds.23657
10. Keijsers, NL, Admiraal, MA, Cools, AR, Bloem, BR, and Gielen, CC. Differential progression of proprioceptive and visual information processing deficits in Parkinson's disease. Eur J Neurosci. (2005) 21:239–48. doi: 10.1111/j.1460-9568.2004.03840.x
11. Rossi, M, Soto, A, Santos, S, Sesar, A, and Labella, T. A prospective study of alterations in balance among patients with Parkinson's disease. Protocol of the postural evaluation. Eur Neurol. (2009) 61:171–6. doi: 10.1159/000189270
12. Vaugoyeau, M, Viel, S, Assaiante, C, Amblard, B, and Azulay, JP. Impaired vertical postural control and proprioceptive integration deficits in Parkinson's disease. Neuroscience. (2007) 146:852–63. doi: 10.1016/j.neuroscience.2007.01.052
13. Lord, S, Galna, B, Verghese, J, Coleman, S, Burn, D, and Rochester, L. Independent domains of gait in older adults and associated motor and nonmotor attributes: validation of a factor analysis approach. J Gerontol A Biol Sci Med Sci. (2013) 68:820–7. doi: 10.1093/gerona/gls255
14. Galna, B, Lord, S, and Rochester, L. Is gait variability reliable in older adults and Parkinson's disease? Towards an optimal testing protocol. Gait Posture. (2013) 37:580–5. doi: 10.1016/j.gaitpost.2012.09.025
15. Galna, B, Lord, S, Burn, DJ, and Rochester, L. Progression of gait dysfunction in incident Parkinson's disease: impact of medication and phenotype. Mov Disord. (2015) 30:359–67. doi: 10.1002/mds.26110
16. Tramonti, C, Di Martino, S, Unti, E, Frosini, D, Bonuccelli, U, Rossi, B, et al. Gait dynamics in Pisa syndrome and Camptocormia: the role of stride length and hip kinematics. Gait Posture. (2017) 57:130–5. doi: 10.1016/j.gaitpost.2017.05.029
17. Takakusaki, K. Functional neuroanatomy for posture and gait control. J Mov Disord. (2017) 10:1–17. doi: 10.14802/jmd.16062
18. Kraan, CM, Tan, AHJ, and Cornish, KM. The developmental dynamics of gait maturation with a focus on spatiotemporal measures. Gait Posture. (2017) 51:208–17. doi: 10.1016/j.gaitpost.2016.10.021
19. Schoneburg, B, Mancini, M, Horak, F, and Nutt, JG. Framework for understanding balance dysfunction in Parkinson's disease. Mov Disord. (2013) 28:1474–82. doi: 10.1002/mds.25613
20. Artusi, CA, Montanaro, E, Tuttobene, S, Romagnolo, A, Zibetti, M, and Lopiano, L. Pisa syndrome in Parkinson's disease is associated with specific cognitive alterations. Front Neurol. (2019) 10:577. doi: 10.3389/fneur.2019.00577
21. Yao, MS, Zhou, LC, Tan, YY, Jiang, H, Chen, ZC, Zhu, L, et al. Gait characteristics and brain activity in Parkinson's disease with concomitant postural abnormalities. Aging Dis. (2020) 11:791–800. doi: 10.14336/AD.2019.0929
22. Tinazzi, M, Geroin, C, Bhidayasiri, R, Bloem, BR, Capato, T, Djaldetti, R, et al. Task force consensus on nosology and cut-off values for axial postural abnormalities in parkinsonism. Mov Disord Clin Pract. (2022) 9:594–603. doi: 10.1002/mdc3.13460
23. Berganzo, K, Tijero, B, Gonzalez-Eizaguirre, A, Somme, J, Lezcano, E, Gabilondo, I, et al. Motor and non-motor symptoms of Parkinson's disease and their impact on quality of life and on different clinical subgroups. Neurologia. (2016) 31:585–91. doi: 10.1016/j.nrl.2014.10.010
24. Jankovic, J. Parkinson's disease: clinical features and diagnosis. J Neurol Neurosurg Psychiatry. (2008) 79:368–76. doi: 10.1136/jnnp.2007.131045
25. Zwergal, A, Rettinger, N, Frenzel, C, Dieterich, M, Brandt, T, and Strupp, M. A bucket of static vestibular function. Neurology. (2009) 72:1689–92. doi: 10.1212/WNL.0b013e3181a55ecf
26. Cabral, S, Resende, RA, Clansey, AC, Deluzio, KJ, Selbie, WS, and Veloso, AP. A global gait asymmetry index. J Appl Biomech. (2016) 32:171–7. doi: 10.1123/jab.2015-0114
27. Nigg, S, Vienneau, J, Maurer, C, and Nigg, BM. Development of a symmetry index using discrete variables. Gait Posture. (2013) 38:115–9. doi: 10.1016/j.gaitpost.2012.10.024
28. Ayoubi, F, Launay, CP, Annweiler, C, and Beauchet, O. Fear of falling and gait variability in older adults: a systematic review and meta-analysis. J Am Med Dir Assoc. (2015) 16:14–9. doi: 10.1016/j.jamda.2014.06.020
29. Ninomiya, S, Morita, A, Teramoto, H, Akimoto, T, Shiota, H, and Kamei, S. Relationship between postural deformities and frontal function in Parkinson's disease. Parkinsons Dis. (2015) 2015:462143:1–5. doi: 10.1155/2015/462143
30. Kohsaka, M, Oeda, T, Takaya, S, Tomita, S, Park, K, Yamamoto, K, et al. Cortical involvement of lateral trunk flexion and verticality misperception in Parkinson's disease. Brain Commun. (2025) 7:fcaf040. doi: 10.1093/braincomms/fcaf040
31. Gardoni, A, Agosta, F, Sarasso, E, Basaia, S, Canu, E, Leocadi, M, et al. Cerebellar alterations in Parkinson's disease with postural instability and gait disorders. J Neurol. (2023) 270:1735–44. doi: 10.1007/s00415-022-11531-y
32. Fjell, AM, Westlye, LT, Greve, DN, Fischl, B, Benner, T, van der Kouwe, AJ, et al. The relationship between diffusion tensor imaging and volumetry as measures of white matter properties. NeuroImage. (2008) 42:1654–68. doi: 10.1016/j.neuroimage.2008.06.005
33. Hirata, K, Hattori, T, Kina, S, Chen, Q, Ohara, M, and Yokota, T. Striatal dopamine denervation impairs gait automaticity in drug-naive Parkinson's disease patients. Mov Disord. (2020) 35:1037–45. doi: 10.1002/mds.28024
34. Halliday, SE, Winter, DA, Frank, JS, Patla, AE, and Prince, F. The initiation of gait in young, elderly, and Parkinson's disease subjects. Gait Posture. (1998) 8:8–14. doi: 10.1016/S0966-6362(98)00020-4
35. Cohen, RG, Nutt, JG, and Horak, FB. Recovery from multiple APAs delays gait initiation in Parkinson's disease. Front Hum Neurosci. (2017) 11:60. doi: 10.3389/fnhum.2017.00060
36. Moreira-Neto, A, Ugrinowitsch, C, Coelho, DB, de Lima-Pardini, AC, Barbosa, ER, Teixeira, LA, et al. Freezing of gait, gait initiation, and gait automaticity share a similar neural substrate in Parkinson's disease. Hum Mov Sci. (2022) 86:103018. doi: 10.1016/j.humov.2022.103018
37. Costa, TM, Simieli, L, Bersotti, FM, Mochizuki, L, Barbieri, FA, and Coelho, DB. Gait and posture are correlated domains in Parkinson's disease. Neurosci Lett. (2022) 775:136537. doi: 10.1016/j.neulet.2022.136537
38. Godi, M, Arcolin, I, Giardini, M, Corna, S, and Schieppati, M. A pathophysiological model of gait captures the details of the impairment of pace/rhythm, variability and asymmetry in parkinsonian patients at distinct stages of the disease. Sci Rep. (2021) 11:21143. doi: 10.1038/s41598-021-00543-9
39. Tian, Q, Chastan, N, Bair, WN, Resnick, SM, Ferrucci, L, and Studenski, SA. The brain map of gait variability in aging, cognitive impairment and dementia-a systematic review. Neurosci Biobehav Rev. (2017) 74:149–62. doi: 10.1016/j.neubiorev.2017.01.020
40. Osoba, MY, Rao, AK, Agrawal, SK, and Lalwani, AK. Balance and gait in the elderly: a contemporary review. Laryngoscope Investig Otolaryngol. (2019) 4:143–53. doi: 10.1002/lio2.252
41. Rennie, L, Opheim, A, Dietrichs, E, Lofgren, N, and Franzen, E. Highly challenging balance and gait training for individuals with Parkinson's disease improves pace, rhythm and variability domains of gait - a secondary analysis from a randomized controlled trial. Clin Rehabil. (2021) 35:200–12. doi: 10.1177/0269215520956503
42. Slouha, E, Ibrahim, F, Esposito, S, Mursuli, O, Rezazadah, A, Clunes, LA, et al. Botulinum toxin for the Management of Parkinson's disease: a systematic review. Cureus. (2024) 16:e53309. doi: 10.7759/cureus.53309
Keywords: Parkinson’s disease, lateral trunk flexion, subjective visual vertical, gait performance, gait variability
Citation: Ota K, Mani H, Nochimura K, Nakashiro Y, Hamada S and Moriwaka F (2025) Five functional domains associated with gait performance in Parkinson’s disease and lateral trunk flexion. Front. Neurol. 16:1541970. doi: 10.3389/fneur.2025.1541970
Received: 11 December 2024; Accepted: 10 March 2025;
Published: 08 April 2025.
Edited by:
Onanong Phokaewvarangkul, Chulalongkorn University, ThailandReviewed by:
Jacky Ganguly, Institute of Neurosciences, Kolkata (I-NK), IndiaCopyright © 2025 Ota, Mani, Nochimura, Nakashiro, Hamada and Moriwaka. This is an open-access article distributed under the terms of the Creative Commons Attribution License (CC BY). The use, distribution or reproduction in other forums is permitted, provided the original author(s) and the copyright owner(s) are credited and that the original publication in this journal is cited, in accordance with accepted academic practice. No use, distribution or reproduction is permitted which does not comply with these terms.
*Correspondence: Hiroki Mani, bWFuaS1oaXJva2lAb2l0YS11LmFjLmpw
†These authors have contributed equally to this work
Disclaimer: All claims expressed in this article are solely those of the authors and do not necessarily represent those of their affiliated organizations, or those of the publisher, the editors and the reviewers. Any product that may be evaluated in this article or claim that may be made by its manufacturer is not guaranteed or endorsed by the publisher.
Research integrity at Frontiers
Learn more about the work of our research integrity team to safeguard the quality of each article we publish.