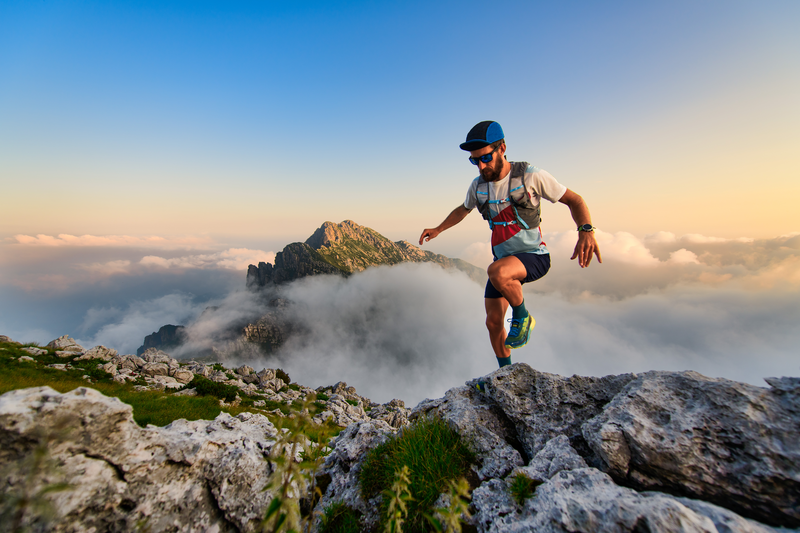
95% of researchers rate our articles as excellent or good
Learn more about the work of our research integrity team to safeguard the quality of each article we publish.
Find out more
ORIGINAL RESEARCH article
Front. Neurol.
Sec. Sleep Disorders
Volume 16 - 2025 | doi: 10.3389/fneur.2025.1537780
This article is part of the Research Topic Mechanisms and Interventions for Post-Operative Neurocognitive Disorder and Sleep Disruptions View all 10 articles
The final, formatted version of the article will be published soon.
You have multiple emails registered with Frontiers:
Please enter your email address:
If you already have an account, please login
You don't have a Frontiers account ? You can register here
Background: Elderly surgical patients are at high risk of perioperative sleep disturbances (PSD), and the underlying pathogenic mechanisms remain unclear. The relationship between peripheral inflammatory status and PSD pathogenesis currently lacks substantial clinical evidence.Objective: This study aims to evaluate the association between peripheral inflammation indicators and PSD in elderly patients undergoing laparoscopic hepatobiliary surgery, and to analyze the dynamic changes in peripheral inflammation in PSD patients throughout the perioperative period.Method and Materials: Using retrospective data, this study compares peripheral inflammatory markers (NLR, MLR, PLR, SII, IL-6, and IL-10) in patients with PSD versus those with normal sleep patterns before and after surgery. Receiver operating characteristic (ROC) curves were employed to evaluate the discriminative power of these indicators for PSD. Logistic regression models were employed to assess risk associations between inflammatory markers and PSD. Dynamic changes in peripheral inflammation were compared before surgery, on the day the surgery ended, and one day post-surgery between patients with PSD and those with normal sleep, exploring potential correlations with PSD pathogenesis.Result: The study ultimately included clinical data from 156 patients. Findings indicated that elevated NLR and SII levels before and after surgery, alongside decreased plasma IL-10 levels post-surgery, are associated with a higher incidence of PSD. Peripheral inflammatory markers on the day of surgery were not significantly predictive of post-PSD. Multivariable logistic regression analyses identified NLR, SII, IL-6, and IL-10 as independent predictors of pre-PSD, while NLR, SII, and IL-10 remained independently associated with post-PSD.Conclusion: Dynamic changes in peripheral inflammation during the perioperative period are associated with PSD in elderly patients undergoing laparoscopic hepatobiliary surgery. These findings may support the early identification and screening of high-risk PSD patients, providing new insights into the underlying mechanisms of PSD pathogenesis.
Keywords: perioperative sleep disturbances, peripheral inflammation, Surgery, immune cells, Elderly
Received: 27 Feb 2025; Accepted: 03 Apr 2025.
Copyright: © 2025 Wei, Zhu, Zhao, Zou, Hu, Huang, Li, Pan, Kong, Tan and Chen. This is an open-access article distributed under the terms of the Creative Commons Attribution License (CC BY). The use, distribution or reproduction in other forums is permitted, provided the original author(s) or licensor are credited and that the original publication in this journal is cited, in accordance with accepted academic practice. No use, distribution or reproduction is permitted which does not comply with these terms.
* Correspondence:
Siyou Tan, Hunan Provincial People's Hospital, Changsha, 410005, Hunan Province, China
Wenyan Chen, Hunan Provincial People's Hospital, Changsha, 410005, Hunan Province, China
Disclaimer: All claims expressed in this article are solely those of the authors and do not necessarily represent those of their affiliated organizations, or those of the publisher, the editors and the reviewers. Any product that may be evaluated in this article or claim that may be made by its manufacturer is not guaranteed or endorsed by the publisher.
Research integrity at Frontiers
Learn more about the work of our research integrity team to safeguard the quality of each article we publish.