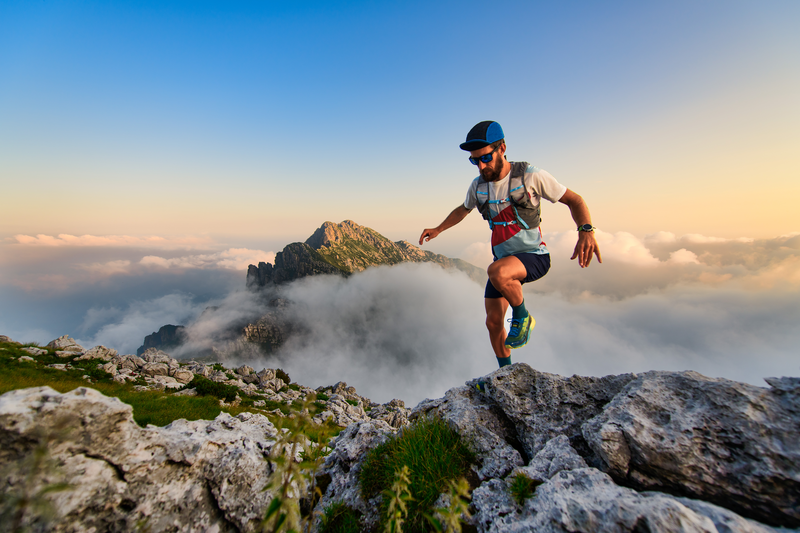
95% of researchers rate our articles as excellent or good
Learn more about the work of our research integrity team to safeguard the quality of each article we publish.
Find out more
SYSTEMATIC REVIEW article
Front. Neurol. , 04 April 2025
Sec. Neurorehabilitation
Volume 16 - 2025 | https://doi.org/10.3389/fneur.2025.1531127
This article is part of the Research Topic Socio-Legal, Ethical, Technical and Medical Considerations on Neuroprivacy and Brain-Machine Interaction Technologies in the era of A.I. View all 10 articles
Background: Stroke is a major global health concern, imposing significant medical and social burdens. Vagus nerve stimulation (VNS), an emerging neuromodulation technology, has shown potential in the treatment of stroke. This bibliometric analysis aims to explore the knowledge structure and research trends in the field of VNS for stroke from 2004 to 2024.
Methods: Publications were retrieved from the Web of Science Core Collection. CiteSpace and VOSviewer were used to conduct bibliometric analyses, including author productivity, institutional contributions, and emerging research themes etc.
Results: A total of 191 eligible publications were analysed. Kilgard, M. P., and Hays, S. A. were the most prolific authors, each contributing 26 publications. The USA (96 publications), China (69 publications), and Scotland (17 publications) were the most prolific countries. The University of Texas at Dallas (33 publications) was the most prolific institution, followed by Chongqing Medical University (19 publications) and the University of Glasgow (15 publications). Future research is expected to focus on: (1) neurophysiological mechanisms of VNS in stroke recovery; (2) synergistic effects of VNS with other rehabilitation therapies; (3) comparative efficacy of non-invasive transauricular VNS versus invasive VNS; (4) safety and effectiveness of VNS for post-stroke functional impairments beyond motor rehabilitation; and (5) optimisation of VNS parameters for stroke treatment.
Conclusion: The field of VNS for stroke has experienced steady growth over the past two decades. This bibliometric analysis provides valuable insights to guide future research, clinical applications, and policy developments.
Globally, stroke has been recognized as the second leading cause of death and the third leading cause of disability (1). Evidence from the Global Burden of Disease study shows that the number of patients with strokes increased by 70% from 1990 to 2019 (2), with a rising incidence among young and middle-aged people under 55-years-old. Moreover, The Lancet Neurology Commission estimate that global stroke-related deaths will increase by 50% from 2020 to 2050, with the number of people living with disabilities expected to rise by about 30% (3). As the aging population increasing, the burden of stroke will escalate significantly, presenting serious challenges to governments and healthcare globally. However, despite continuous advancements in rehabilitation treatments, effectively restoring patients’ functional abilities remains a major issue in stroke management.
Recently, vagus nerve stimulation (VNS), as an innovative neuromodulation technology, has shown potential in stroke rehabilitation by stimulating the vagus nerve to regulate central nervous system function. In 2021, the US Food and Drug Administration (FDA) approved the application of VNS combined with rehabilitation exercises for the treatment of chronic stroke (4). VNS can be administered through either invasive or non-invasive approaches. Invasive VNS (iVNS) involves costly surgery to implant electrodes in the left cervical vagus nerve, which are then stimulated by a pulse generator. Non-invasive VNS, also known as transauricular VNS (tVNS), typically includes transcutaneous VNS via the ear (taVNS) or cervical VNS (tcVNS) (5). Ventureyra (6) first identified tVNS as a novel non-invasive brain modulation technology. tVNS involves the using low-frequency pulse currents to stimulate the auricular branch of the vagus nerve. Moreover, safety, cost-effectiveness, easy to administer, and high acceptability are the advantages of tVNS (7). Recently, a recent randomized controlled trial found that the relative growth of ischemic lesions on 24-h diffusion-weighted magnetic resonance imaging (MRI) was 63% in the tVNS group, while it was 184% in the sham stimulation group (p = 0.109). This suggests that tVNS has a neuroprotective effect in acute stroke (8). Moreover, other studies have shown that taVNS combined with motor rehabilitation can enhance motor function (9), proprioception, and light touch sensation (10) in chronic stroke patients.
Bibliometric analysis holds a significant place in medical research, primarily due to its ability in generating broad evidence synthesis in a specific research field. In the research evolution framework, studies can be categorized into two types: original research and evidence synthesis. Evidence synthesis can be further divided into two forms: deep synthesis (i.e., systematic reviews and meta-analyses) (11) and broad synthesis (i.e., bibliometric analysis) (12). Deep synthesis is typically used to address specific research questions, for example, focusing on the effectiveness of a particular intervention on certain health-related outcomes, thus providing a precise conclusion. In contrast, broad synthesis is suited for analysing the overall knowledge structure of a research field and predicting future research trends.
In the field of stroke, while there have been numerous deep syntheses on VNS technology, there is a lack of broad synthesis as bibliometric analyses. Therefore, it is necessary to conduct a comprehensive bibliometric analysis in the field of VNS for stroke. The current study aims to provide a deep understanding of current research hotspots, trends, and the overall knowledge structure. This work will not only identify key research directions and potential gaps but also serve as a valuable reference for future research collaborations (13).
In this study, CiteSpace (14) and VOSviewer (15) were employed for performance analysis and scientific mapping. The Web of Science Core Collection (WoSCC), as the most widely used multidisciplinary database in bibliometric analysis, was chosen as the data source. Following bibliometric analysis guidelines, the initial search terms were determined by reviewing the key terms used in relevant systematic reviews, after which the authors brainstormed additional terms (16). The final search strategy included two group of terms such as “Vagus Nerve Stimulation” and “Stroke,” combined using Boolean logic operators (see Appendix 1). The search and data collection were conducted in WoSCC on August 1st 2024, and limited to publications from January 1st 2004, to July 1st 2024. Only Original Articles and Review Articles published in English were included in the analysis.
A bibliometric analysis of 191 eligible publications from 2000 to 2024 was conducted using CiteSpace and VOSviewer. VOSviewer was used to construct networks for analysing co-authorship by country, institution, and author. CiteSpace were applied to conduct reference co-cited analysis of reference and citation burst analysis from the keywords co-occurrence analysis. Microsoft Excel 2019 (Microsoft Corp., Redmond, WA, United States) was used to manage the data, create the figures of the annual number of publications and citations. To evaluate the impact factor of the publications included in this study, we gathered journal impact factors from the 2023 Journal Citation Report (JCR, Clarivate Analytics, Philadelphia, PA, United States).
A total of 305 documents were retrieved in this search. After excluding 27 non-English documents and 87 non-research types (e.g., Meeting Abstracts = 15, Editorials = 5, Letters = 4, Early Access = 3, Book Chapters = 2, Corrections = 1, News Items = 1, and Proceedings Papers = 1), 191 documents remained, consisting of 128 Original Articles and 63 Review Articles. Figure 1 illustrates the annual publication trends in the field of VNS for stroke from 2008 to 2024. The annual number of publications in this field has risen sharply over the past decade, increasing to over 10 in 2016 (12 publications) and peaking at 36 in 2022. The total citations for these publications amounted to 5,140, with an average of 26.9 citations per publication. The identified documents were indexed by 38 WoSCC categories, with the top five being: Neurosciences (95 publications), Clinical Neurology (70 publications), Rehabilitation (19 publications), Peripheral Vascular Disease (18 publications), and Medicine, Research & Experimental (12 publications). The top five agencies which providing the highest number of funding were the National Institutes of Health (NIH, 45 documents, United States), the U.S. Department of Health and Human Services (45 documents, United States), the National Natural Science Foundation of China (NSFC, 28 documents, China), the NIH National Institute of Neurological Disorders and Stroke (NINDS, 10 documents, United States), and MicroTransponder Inc. (9 documents, United States). Moreover, 142 of the 191 documents (74.3%) were open accessed.
A total of 885 authors contributed at least one publication in the field of VNS for stroke. Table 1 shows the top 11 authors with the highest number of publications, with Kilgard M. P., and Hays S. A. as the most prolific authors, each published 26 documents. They are followed by Rennaker R. L., with 20 publications. All three researchers are affiliated with The University of Texas at Dallas, USA. Among those most prolific authors, Rennaker R. L. achieved the highest average citation impact (ACI) of 70.1. Figure 2 presents the map of collaborative network of authors, with connections based on total link strength (TLS), representing how frequently two authors co-publish in one document. Four major collaborative networks were identified, with Ma J. (red cluster, 27 items) and Dawson J. (green cluster, 20 items) leading the two largest collaboration groups.
Table 1. Top 11 authors with the highest number of publications in the field of VNS for stroke (2008–2024).
Figure 2. Collaborative network of authors in the field of VNS for stroke (2004–2024). (Produced by VOSviewer; each node represents an author, with 71 authors reaching the threshold of 3 publications; size of a node is determined by its total link strength; link between nodes is determined by the number of times the two authors appear in the same publication at the same time; larger nodes and thicker links represent more frequent collaboration between the two authors; nodes of the same colour represent a collaboration cluster).
A total of 33 countries/regions within 356 institutions contributed to at least one publication in the field of VNS for stroke. Performance analyses by country/region and institution are shown in Tables 2, 3. The USA, China, and Scotland emerged as the most productive contributors, with 96, 69, and 17 publications, respectively. Among the 13 countries/regions with the highest number of publications, only China and Malaysia are developing countries. This is likely due to the high cost of VNS equipment, which is more widely used in developed nations. Scotland has the highest ACI, followed by England, indicating that their publications are cited most frequently in this field. The University of Texas at Dallas leads as the most productive institution, followed by Chongqing Medical University in China and the University of Glasgow in Scotland. The University of Minnesota received the highest ACI for papers on this topic. Figures 3, 4 presents the map of collaboration networks of countries/regions and institutions.
Table 2. Top 13 countries/regions with the highest number of publications in the field of VNS for stroke (2004–2024).
Table 3. Top 11 institutions with the highest number of publications in the field of VNS for stroke (2004–2024).
Figure 3. Collaborative network of countries/regions in the field of VNS for stroke (2008–2024). (Produced by VOSviewer; each node represents a country/region, with 33 countries/regions reaching the threshold of 1 publications; size of a node is determined by its total link strength; link between nodes is determined by the number of times the two countries/regions appear in the same publication at the same time; larger nodes and thicker links represent more frequent collaboration between the two countries/regions; nodes of the same colour represent a collaboration cluster).
Figure 4. Collaborative network of institutions in the field of VNS for stroke (2004–2024). (Produced by VOSviewer; each node represents a institution, with 34 institutions reaching the threshold of 3 publications; size of a node is determined by its total link strength; link between nodes is determined by the number of times the two institutions appear in the same publication at the same time; larger nodes and thicker links represent more frequent collaboration between the two institutions; nodes of the same colour represent a collaboration cluster).
In the map of collaboration networks of countries/regions, six collaboration clusters were identified, with USA and China led the two main clusters. Moreover, seven collaboration clusters of institutions were identified, with the University of Texas at Dallas and the University of Glasgow led the two primary clusters.
Documents in this field has been published in 109 different journals. Table 4 highlights the 11 journals with the highest number of publications, with Frontiers in Aging Neuroscience and Neurorehabilitation and Neural Repair ranked first and second, respectively. Brain Stimulation, Stroke, and Neural Regeneration Research were journals with an impact factor over five in 2023. As can be seen from the type of journal, publications in the field of VNS for stroke are multidisciplinary and mainly focuses on the fields of neurology and rehabilitation medicine.
Table 4. Top 11 journals publishing the highest number of publications in the field of VNS for stroke (2004–2024).
The top 10 RCTs which received the highest number of citations in the field of VNS for stroke are shown in Table 5. Among them, Dawson J. et al. (2021) entitled: “Vagus nerve stimulation paired with rehabilitation for upper limb motor function after ischaemic stroke (VNS-REHAB): a randomized, blinded, pivotal, device trial” received the highest number of citations. All these RCTs investigate motor function rehabilitation after stroke, with most focusing on upper limb function, and only one study exploring post-stroke sensation and emotion.
Table 5. Top 10 RCTs with the highest number of citations in the field of VNS for stroke (2008–2024).
CiteSpace was used to visualize both the reference co-cited analysis, and the citation bursts analysis from the keywords co-occurrence analysis in the field of VNS for stroke. A total of 11 clusters were identified in Figure 5. The research trends over the past 20 years are illustrated in the Figure 5, spanning from 2008 on the left to 2024 on the right. Two decades ago, VNS was predominantly an invasive procedure, involving the surgical placement of electrodes directly on the vagus nerve. Early studies focused on the mechanisms of VNS, particularly in cluster #11 endovascular therapy (26), followed by basic experimental research in cluster #5 rat models. Around the same time, transauricular VNS (cluster #7 taVNS) emerged and gradually found clinical application. Later, non-invasive brain stimulation methods, such as #6 transcranial direct current stimulation (tDCS), which is similar to VNS, were introduced for stroke treatment. Research has shown that VNS exerts cluster #4 neuroprotective effects by activating anti-inflammatory pathways (27). Further studies discovered that cluster #3 spreading depolarization (SD) occurs during acute cerebral infarction, and VNS can reduce cluster #3 SD frequency following focal ischemia (28), leading to stroke recovery. In recent years, researchers have conducted cluster #1 meta-analyses of previous evidence, consolidating the body of knowledge on VNS for stroke.
Figure 5. Knowledge structure of the field of VNS for stroke, based on the reference co-citation analysis (2004–2024). (Produced by CiteSpace; time slicing = 01/01/2004 to 01/07/2024, year per slice = 1; from left to right represents the evolution of the research clusters from 2004 to 2024; each cluster is made up of its contained publications represented by nodes, and the size of the cluster is determined by the number of publications it contains).
To determine the robustness of the knowledge structure and the turning point paper derived from the reference co-citation analysis, a sensitivity analysis was performed. Appendix 2 shows the results of the reference co-citation analysis with an adjusted time slice from 1 year to 5 years, which demonstrates the robustness of the previous findings.
Analysing the most frequently mentioned keywords helps to identify emerging research trends. Figure 6 shows time zone view of keyword co-occurrence analysis in the field of VNS for stroke. Since 2011, VNS has evolved from focusing on cerebral ischemia to gradually shifting toward stroke rehabilitation. Researchers have explored the effects of VNS on the motor cortex, and its application in the treatment of heart failure has also been gradually investigated. In recent years, VNS research has increasingly concentrated on rehabilitation for ischemic stroke, with continuous optimization of stimulation parameters. At the same time, the development of non-invasive neuromodulation methods, such as transcranial direct current stimulation (tDCS), has opened up new avenues for VNS research.
Figure 6. Time zone view of keyword co-occurrence analysis in the field of VNS for stroke (2008–2024). (Produced by CiteSpace; time slicing = 01/01/2004 to 01/07/2024, year per slice = 1; from left to right represents the evolution of the authors’ keywords from 2008 to 2024).
Figure 7 displays the top 10 author’s keywords with the strongest citation bursts, as revealed by the keyword co-occurrence analysis. Over the past 20 years, terms like cerebral ischemia, cerebral blood flow, heart failure, vagus nerve, and motor cortex have exhibited the most significant citation bursts, indicating that these topics have garnered considerable attention from researchers in this field. In the past 5 years, keywords such as cortical reorganization, motor learning, transcutaneous vagus nerve stimulation, stroke rehabilitation, and upper extremity have emerged, suggesting that these areas may be at the forefront of current research. This suggests that research is gradually shifting from investigating pathophysiological mechanisms to exploring functional recovery and remodelling. There is also a clear trend from basic research toward translational studies, with an increasing focus on neuroplasticity and neuromodulation technologies.
Figure 7. Top10 keywords with the strongest citation bursts in the field of VNS for stroke (2008–2024).
In this study, we visualize and analyse 191 documents authored by 885 researchers from 365 institutions across 33 countries. These documents were published in 109 journals and indexed in 38 categories within the WoSCC. This bibliometric analysis examines the evolution of the knowledge structure and emerging research trends in VNS for stroke from 2004 to 2024.
The results indicate that annual number of publications in the field of VNS for stroke has steadily increased since the 2008, peaking in 2022–2023. This trend suggests that the field has significant development potential in the future. By analysing the number of authors’ publications and their collaboration networks, it was found that the three most published authors, Kilgard M. P., Hays S. A., and Rennaker R. L., and their teams developed a technology for targeted plasticity therapy, now known as pair VNS (29). Pair VNS with rehabilitation training to improve the plasticity of the corticospinal motor network, thereby improving synaptic connections to muscles involved in rehabilitation. In the rat model, it was found that pairing VNS with movement or auditory stimuli could enhance plasticity in the motor and auditory cortices, respectively (30). In a mouse model, auditory training combined with VNS-induced plastic reorganization of the auditory cortex, leading to an improvement in tinnitus symptoms (31). Furthermore, VNS combined with exercise training significantly improves motor function in patients compared to exercise training alone. Research indicates that moderate intensity VNS can boost motor cortex plasticity, while high intensity VNS may have adverse effects (32) Beyond facilitating motor function recovery, VNS has been shown to promote the reduction of olfactory and auditory fear responses (33) and improve anxiety levels (34). When combined with tactile rehabilitation, VNS significantly enhances recovery of sensory and motor functions after peripheral nerve injuries (35). Furthermore, the team explored optimizing VNS parameters and the duration of stimulation based on individual neural activity to achieve better outcomes (36).
Dawson et al. (17) conducted several clinical trials, including pivotal, randomized, triple-blind, multi-site, sham-controlled studies (19, 37). These studies demonstrate that VNS combined with rehabilitation significantly improves moderate to severe upper extremity weakness resulting from chronic ischemic stroke and exhibits good long-term compliance (38). The VNS aids in the brain’s relearning and restoration of damaged motor functions by enhancing nervous system plasticity.
Through performance analysis and science mapping, the current research trends were summarised.
First, studies indicate that the biological mechanism of VNS in the treatment of stroke primarily involves the inhibition of inflammatory pathways activated after stroke, thus reducing secondary damage. Specifically, VNS has been shown to effectively decrease the release of inflammatory cytokines (39), stabilise endothelial cell function (40), maintain the integrity of the blood-brain barrier (41), and mitigate excitotoxicity resulting from stroke (42). By increasing the release of neurotransmitters such as norepinephrine and acetylcholine, VNS promotes prominent plasticity and long-term enhancement, contributing to the reorganization and recovery of motor and cognitive functions (43). In the clinical setting, VNS shows potential for promoting neuroplasticity and functional recovery, particularly in the rehabilitation of chronic stroke patients. Numerous studies have shown that when VNS is combined with rehabilitation training, it significantly improves the plasticity of neural networks and facilitates the reconstruction of damaged neural pathways, thus accelerating the recovery of motor functions (30, 31, 38). Despite the valuable information provided by existing studies on the role of VNS in stroke treatment, the underlying neurophysiological mechanisms of VNS remain not fully elucidated. In particular, the specific mechanisms of action in different types of strokes and at various recovery stages require further exploration through more in-depth experimental and clinical studies.
Secondly, traditional VNS requires surgical implantation of a device. Although VNS received approval from the US FDA for clinical treatment as early as the last century, this invasive method is associated with certain adverse effects, including paralysis of the vocal cord and dysphagia (44, 45). Moreover, patients often face high medical costs (46). In recent years, the emergence of non-invasive VNS methods, such as taVNS and tcVNS, has provided patients with a safer and more cost-effective alternative approach (47). According to Peuker’s anatomical study of 22 cadavers, the auricular branch of the vagus nerve (ABVN) was 100% distributed in the cymba of the auricle. Furthermore, there was a notable distribution of ABVN in other regions of the ear, including the crus of the helix, the tragus, and the antihelix (48). This discovery offers an anatomical basis for the development of non-invasive VNS. In traditional Chinese medicine, ear acupuncture theory and ear acupuncture emphasise stimulating specific areas of the ear to positively influence bodily health. ABVN covers the primary bundle of the vagus nerve and stimulates the human ear, allowing the stimulation to travel through the vagal bundle and reach the brain via the brainstem. This results in a notable increase in activation in areas such as the angular gyrus, caudate, cerebellum, cingulate, and frontal cortex (49). Stimulating these regions may induce changes in the activity of vagus nerve pathways, affecting the structures of the brainstem and central nervous system, and producing regulatory effects similar to those of the invasive VNS without the need for surgery (50). The reported side effects are mainly related to the administration of transcutaneous electrical current, causing local skin irritation. In general, tVNS is safe and well tolerated at the doses tested in research studies (51). This non-surgical treatment approach is gaining traction, particularly with taVNS. Future research will focus on optimizing the design of non-invasive VNS devices, exploring their effectiveness in stroke rehabilitation, and comparing them with traditional implantable VNS (51). The advancement of this technology offers patients new rehabilitation options and is expected to reduce treatment risks and costs.
Thirdly, the combination of VNS with other rehabilitation techniques is emerging as a significant research direction, particularly regarding the synergistic effects in multimodal treatment. Studies have demonstrated that VNS combined with rehabilitation training significantly enhances the recovery of upper limb motor function in patients with stroke. For instance, an one-year observational study (22) and a multicenter, three-year observational study (52) found that VNS combined with rehabilitation was more effective than with traditional rehabilitation methods (38). Furthermore, integration of upper limb robotic training with selectively applied auricular VNS significantly reduced wrist and hand spasticity during upper limb extension training in stroke patients with stroke (24). This combined approach has also been shown to improve arm function in individuals with chronic stroke (20). In another study, Szulczewski (53) reported that combining taVNS with controlled slow breathing further enhanced therapeutic effects. Future research will focus on exploring the synergy between VNS and different exercise training modalities, particularly optimizing stimulation parameters to maximise functional recovery and neuroplasticity while minimising potential side effects (36, 54). This exploration of precision treatment will offer new avenues for the combined application of VNS and various rehabilitation technologies, with the potential to deliver more effective rehabilitation programmes for stroke patients. These findings highlight the complementary nature of VNS with other rehabilitation technologies in addressing various stroke symptoms. Specifically, when VNS is combined with neurorehabilitation robots, it may produce even greater therapeutic benefits. The synergistic effects of transcranial direct current stimulation (tDCS) and transcutaneous vagal nerve stimulation (taVNS) on brain response are also being investigated (55). Although it is rarely used in stroke, VNS combined with other neuromodulation techniques (e.g., BCI, TMS, VR based rehabilitation) would provide insight into future innovations.
Furthermore, while there is a substantial body of research on the recovery of motor and sensory functions after stroke using VNS, studies addressing other functional disorders are relatively scarce, such as cognitive impairment, speech disorders, and swallowing difficulties. These areas are poised to become important research directions in the future. Post-stroke cognitive impairment is often the result of hypoperfusion, ischemia, hypoxia, and neuroinflammation associated with chronic stroke. The VNS has the potential to improve cognitive impairment by initiating anti-inflammatory mechanisms (56). However, most existing studies on the cognitive effects of VNS focus on healthy individuals, and its efficacy in stroke patients still requires validation through large-scale clinical trials to confirm both effectiveness and safety. In the study of swallowing disorders following stroke, Long L’s team discovered that transauricular VNS (taVNS) can slightly improve dysphagia in rats after ischemic stroke. This improvement is achieved primarily by promoting myelin regeneration, inducing angiogenesis, and inhibiting inflammatory responses in rat white matter (57). Similarly, Wang et al. (58) demonstrated that transcutaneous ear VNS can help improve dysphagia after acute stroke. Despite these findings, there remains a lack of studies on the application of VNS in post-stroke dysphagia, which requires further exploration and validation of the existing clinical evidence. Regarding aphasia resulting from stroke, VNS may facilitate the recovery of language functions by modulating brain areas associated with language (59). A study by Morrison et al. (60) indicated that when VNS is paired with mandibular movement, it can enhance the plasticity of the oral-facial neural network, potentially serving as an auxiliary method to rehabilitate motor aphasia after stroke. However, the efficacy of VNS on sensory aphasia and mixed aphasia remains unclear, highlighting a gap in relevant research that warrants further investigation. Since each stroke patient experiences varying degrees of neurological damage and functional rehabilitation needs, exploring the efficacy of VNS across different types of functional disorders will be a central focus of future research. By further investigating the role of VNS in cognitive, language, swallowing, and other functional disorders, researchers hope to develop more precise treatment strategies to improve rehabilitation outcomes across various dimensions after stroke.
Finally, despite being an important brain stimulation technology, in addition to treating stroke, has been used as a potential therapy for epilepsy, depression, anxiety, chronic heart failure, and fibromyalgia (44), there is currently no consensus on the “optimal” stimulation parameters for VNS. The interplay of various stimulation parameters, such as current intensity, pulse width, frequency, duty cycle, and duration, complicates the clinical application of VNS (61). However, as research into VNS theory and application deepens, future studies may leverage neuroimaging methods such as functional magnetic resonance imaging (fMRI) and positron emission tomography (PET), to monitor the brain’s response to VNS. These technologies allow for real-time observation of changes in brain activity and neural networks, providing valuable insights into the effects of VNS on different brain regions (62). Furthermore, the application of biomarkers can support personalised treatment approaches. By analysing patients’ gene expression, inflammation levels, and other biological indicators, researchers can better understanding of the mechanisms of VNS in various patients. To be specific, gene expression can help researchers identify genes and pathways that influence neuroregulation, and inflammation levels can help assess how well VNS regulates the immune system. A meta-analysis showed that taVNS was effective in modulating IL-1ß and IL-10, iVNS modulated IL-6 (63). Other biological indicators, such as hormone levels and neurotransmitters, can provide insight into the functional state of the nervous system. By integrating these data, researchers can determine each patient’s unique response to VNS treatment, thereby identifying the most suitable parameter settings and rehabilitation strategies for individualised treatment (64). By integrating neuroimaging and biomarkers, future research is expected to develop more precise stimulation parameters and personalised rehabilitation programmes. This approach not only aims to optimise the efficacy of VNS but may also can improve overall functional recovery in stroke patients, encompassing motor, cognitive, and other areas. A deeper exploration of this direction will pave the way for personalised application of VNS, making it more targeted and effective in clinical practice.
This bibliometric analysis identifies potential collaborators, institutions, research hotspots, and future trends in the field of VNS for stroke. Our findings will serve as a valuable guide for clinicians, researchers, industry partners, policymakers, and other stakeholders. However, this study has some limitations.
First, our data were extracted solely from WoSCC, which may have overlooked relevant studies published in other databases, such as PubMed, Scopus, and Embase. Nonetheless, WoSCC is a comprehensive and multidisciplinary database, making the results of this study highly reliable. Second, data collection was restricted to papers published on August 1, 2024. Given that VNS is currently a hot research topic with a wealth of related literature and considering that the WoSCC database is continuously updated, papers published after August 1, 2024, were not included in this analysis. This limitation may affect our comprehensive understanding of the latest research trends in the field.
To our knowledge, this is the first bibliometric analysis focused on VNS for stroke. The annual number of publications in this field has steadily increased over the past 20 years. Future research hotspots and trends primarily include the following aspects: Investigating the neurophysiological mechanisms underlying vagus nerve therapy for stroke. Exploring the synergistic effects of VNS combined with various rehabilitation training modalities. Conducting comparative studies between non-invasive VNS and invasive VNS. Assessing the efficacy and safety of VNS for other functional disorders beyond post-stroke motor rehabilitation. Determining the “optimal” parameters for VNS in stroke rehabilitation. Current research shows regional imbalances, and it is suggested that closer collaboration between countries, researchers, and institutions will enhance the development of personalised VNS treatment and rehabilitation programmes for stroke patients.
The original contributions presented in the study are included in the article/Supplementary material, further inquiries can be directed to the corresponding author.
JD: Writing – original draft. ZY: Data curation, Writing – original draft. QW: Data curation, Project administration, Visualization, Writing – original draft. ZL: Writing – review & editing.
The author(s) declare that financial support was received for the research and/or publication of this article. This research was funded by the Science and Technology Project of Changzhou Municipal Health Commission (No. QN202372) and Jiangsu Provincial Administration of Traditional Chinese Medicine Project (No. MS2023085).
The authors declare that the research was conducted in the absence of any commercial or financial relationships that could be construed as a potential conflict of interest.
The authors declare that no Gen AI was used in the creation of this manuscript.
All claims expressed in this article are solely those of the authors and do not necessarily represent those of their affiliated organizations, or those of the publisher, the editors and the reviewers. Any product that may be evaluated in this article, or claim that may be made by its manufacturer, is not guaranteed or endorsed by the publisher.
The Supplementary material for this article can be found online at: https://www.frontiersin.org/articles/10.3389/fneur.2025.1531127/full#supplementary-material
1. eClinicalMedicine. The rising global burden of stroke. eClinicalMedicine. (2023) 59:102028. doi: 10.1016/j.eclinm.2023.102028
2. GBD 2019 Stroke Collaborators. Global, regional, and national burden of stroke and its risk factors, 1990–2019: a systematic analysis for the Global Burden of Disease Study 2019. Lancet Neurol. (2021) 20:795–820. doi: 10.1016/S1474-4422(21)00252-0
3. Feigin, VL, and Owolabi, MOWorld Stroke Organization–Lancet Neurology Commission Stroke Collaboration Group. Pragmatic solutions to reduce the global burden of stroke: a World Stroke Organization-Lancet Neurology Commission. Lancet Neurol. (2023) 22:1160–206. doi: 10.1016/S1474-4422(23)00277-6
4. Schambra, HM, and Hays, SA. Vagus nerve stimulation for stroke rehabilitation: neural substrates, neuromodulatory effects and therapeutic implications. J Physiol. (2024) 603:723–35. doi: 10.1113/JP285566
5. Baig, SS, Kamarova, M, Ali, A, Su, L, Dawson, J, Redgrave, JN, et al. Transcutaneous vagus nerve stimulation (tVNS) in stroke: the evidence, challenges and future directions. Auton Neurosci. (2022) 237:102909. doi: 10.1016/j.autneu.2021.102909
6. Ventureyra, EC. Transcutaneous vagus nerve stimulation for partial onset seizure therapy. A new concept. Childs Nerv Syst. (2000) 16:101–2. doi: 10.1007/s003810050021
7. Farmer, AD, Strzelczyk, A, Finisguerra, A, Gourine, AV, Gharabaghi, A, Hasan, A, et al. International consensus based review and recommendations for minimum reporting standards in research on transcutaneous vagus nerve stimulation (version 2020). Front Hum Neurosci. (2020) 14:568051. doi: 10.3389/fnhum.2020.568051
8. Arsava, EM, Topcuoglu, MA, Ay, I, Ozdemir, AO, Gungor, IL, Togay Isikay, C, et al. Assessment of safety and feasibility of non-invasive vagus nerve stimulation for treatment of acute stroke. Brain Stimul. (2022) 15:1467–74. doi: 10.1016/j.brs.2022.10.012
9. Badran, BW, Peng, X, Baker-Vogel, B, Hutchison, S, Finetto, P, Rishe, K, et al. Motor activated auricular vagus nerve stimulation as a potential neuromodulation approach for post-stroke motor rehabilitation: a pilot study. Neurorehabil Neural Repair. (2023) 37:374–83. doi: 10.1177/15459683231173357
10. Baig, SS, Falidas, K, Laud, PJ, Snowdon, N, Farooq, MU, Ali, A, et al. Transcutaneous auricular vagus nerve stimulation with upper limb repetitive task practice may improve sensory recovery in chronic stroke. J Stroke Cerebrovasc Dis. (2019) 28:104348. doi: 10.1016/j.jstrokecerebrovasdis.2019.104348
11. Moher, D, Liberati, A, Tetzlaff, J, and Altman, DGPRISMA Group. Preferred reporting items for systematic reviews and meta-analyses: the PRISMA statement. PLoS Med. (2009) 6:e1000097. doi: 10.1371/journal.pmed.1000097
12. Bornmann, L, Haunschild, R, and Mutz, R. Growth rates of modern science: a latent piecewise growth curve approach to model publication numbers from established and new literature databases. Humanit Soc Sci Commun. (2021) 8:224. doi: 10.1057/s41599-021-00903-w
13. Yu, D, Kou, G, Xu, Z, and Shi, S. Analysis of collaboration evolution in AHP research: 1982–2018. Int J Inf Technol Decis Mak. (2021) 20:7–36. doi: 10.1142/S0219622020500406
14. Chen, CM. CiteSpace II: detecting and visualizing emerging trends and transient patterns in scientific literature. J Am Soc Inf Sci Technol. (2006) 57:359–77. doi: 10.1002/asi.20317
15. Eck, NJV, and Waltman, L. Software survey: VOSviewer, a computer program for bibliometric mapping. Scientometrics. (2010) 84:523–38. doi: 10.1007/s11192-009-0146-3
16. Öztürk, O, Kocaman, R, and Kanbach, DK. How to design bibliometric research: an overview and a framework proposal. Rev Manag Sci. (2024) 18:3333–61. doi: 10.1007/s11846-024-00738-0
17. Dawson, J, Liu, CY, Francisco, GE, Cramer, SC, Wolf, SL, Dixit, A, et al. Vagus nerve stimulation paired with rehabilitation for upper limb motor function after ischaemic stroke (VNS-REHAB): a randomised, blinded, pivotal, device trial. Lancet. (2021) 397:1545–53. doi: 10.1016/S0140-6736(21)00475-X
18. Dawson, J, Pierce, D, Dixit, A, Kimberley, TJ, Robertson, M, Tarver, B, et al. Safety, feasibility, and efficacy of vagus nerve stimulation paired with upper-limb rehabilitation after ischemic stroke. Stroke. (2016) 47:143–50. doi: 10.1161/STROKEAHA.115.010477
19. Kimberley, TJ, Pierce, D, Prudente, CN, Francisco, GE, Yozbatiran, N, Smith, P, et al. Vagus nerve stimulation paired with upper limb rehabilitation after chronic stroke. Stroke. (2018) 49:2789–92. doi: 10.1161/STROKEAHA.118.022279
20. Capone, F, Miccinilli, S, Pellegrino, G, Zollo, L, Simonetti, D, Bressi, F, et al. Transcutaneous vagus nerve stimulation combined with robotic rehabilitation improves upper limb function after stroke. Neural Plast. (2017) 2017:7876507. doi: 10.1155/2017/7876507
21. Wu, D, Ma, J, Zhang, L, Wang, S, Tan, B, and Jia, G. Effect and safety of transcutaneous auricular vagus nerve stimulation on recovery of upper limb motor function in subacute ischemic stroke patients: a randomized pilot study. Neural Plast. (2020) 2020:8841752. doi: 10.1155/2020/8841752
22. Dawson, J, Engineer, ND, Prudente, CN, Pierce, D, Francisco, G, Yozbatiran, N, et al. Vagus nerve stimulation paired with upper-limb rehabilitation after stroke: one-year follow-up. Neurorehabil Neural Repair. (2020) 34:609–15. doi: 10.1177/1545968320924361
23. Li, JN, Xie, CC, Li, CQ, Zhang, GF, Tang, H, Jin, CN, et al. Efficacy and safety of transcutaneous auricular vagus nerve stimulation combined with conventional rehabilitation training in acute stroke patients: a randomized controlled trial conducted for 1 year involving 60 patients. Neural Regen Res. (2022) 17:1809–13. doi: 10.4103/1673-5374.332155
24. Chang, JL, Coggins, AN, Saul, M, Paget-Blanc, A, Straka, M, Wright, J, et al. Transcutaneous auricular vagus nerve stimulation (tAVNS) delivered during upper limb interactive robotic training demonstrates novel antagonist control for reaching movements following stroke. Front Neurosci. (2021) 15:767302. doi: 10.3389/fnins.2021.767302
25. Dawson, J, Engineer, ND, Cramer, SC, Wolf, SL, Ali, R, O’Dell, MW, et al. Vagus nerve stimulation paired with rehabilitation for upper limb motor impairment and function after chronic ischemic stroke: subgroup analysis of the randomized, blinded, pivotal, VNS-REHAB device trial. Neurorehabil Neural Repair. (2023) 37:367–73. doi: 10.1177/15459683221129274
26. Thompson, GW, Levett, JM, Miller, SM, Hill, MR, Meffert, WG, Kolata, RJ, et al. Bradycardia induced by intravascular versus direct stimulation of the vagus nerve. Ann Thorac Surg. (1998) 65:637–42. doi: 10.1016/s0003-4975(97)01351-9
27. Yang, LY, Bhaskar, K, Thompson, J, Duval, K, Torbey, M, and Yang, Y. Non-invasive vagus nerve stimulation reduced neuron-derived IL-1β and neuroinflammation in acute ischemic rat brain. Brain Hemorrhages. (2021) 3:45–56. doi: 10.1016/j.hest.2021.06.003
28. Lindemann, J, Rakers, C, Matuskova, H, Simon, BJ, Kinfe, T, and Petzold, GC. Vagus nerve stimulation reduces spreading depolarization burden and cortical infarct volume in a rat model of stroke. PLoS One. (2020) 15:e0236444. doi: 10.1371/journal.pone.0236444
29. Hays, SA, Rennaker, RL, and Kilgard, MP. Targeting plasticity with vagus nerve stimulation to treat neurological disease. Prog Brain Res. (2013) 207:275–99. doi: 10.1016/B978-0-444-63327-9.00010-2
30. Loerwald, KW, Buell, EP, Borland, MS, Rennaker, RL 2nd, Hays, SA, and Kilgard, MP. Varying stimulation parameters to improve cortical plasticity generated by VNS-tone pairing. Neuroscience. (2018) 388:239–47. doi: 10.1016/j.neuroscience.2018.07.038
31. Borland, MS, Buell, EP, Riley, JR, Carroll, AM, Moreno, NA, Sharma, P, et al. Precise sound characteristics drive plasticity in the primary auditory cortex with VNS-sound pairing. Front Neurosci. (2023) 17:1248936. doi: 10.3389/fnins.2023.1248936
32. Morrison, RA, Danaphongse, TT, Abe, ST, Stevens, ME, Ezhil, V, Seyedahmadi, A, et al. eds. High intensity VNS disrupts VNS-mediated plasticity in motor cortex. Brain Res. (2021) 1756:147332. doi: 10.1016/j.brainres.2021.147332
33. Souza, RR, Oleksiak, CR, Tabet, MN, Rennaker, RL, Hays, SA, Kilgard, MP, et al. Vagus nerve stimulation promotes extinction generalization across sensory modalities. Neurobiol Learn Mem. (2021) 181:107425. doi: 10.1016/j.nlm.2021.107425
34. Mathew, E, Tabet, MN, Robertson, NM, Hays, SA, Rennaker, RL, Kilgard, MP, et al. Vagus nerve stimulation produces immediate dose-dependent anxiolytic effect in rats. J Affect Disord. (2020) 265:552–7. doi: 10.1016/j.jad.2019.11.090
35. Darrow, MJ, Mian, TM, Torres, M, Haider, Z, Danaphongse, T, Seyedahmadi, A, et al. The tactile experience paired with vagus nerve stimulation determines the degree of sensory recovery after chronic nerve damage. Behav Brain Res. (2021) 396:112910. doi: 10.1016/j.bbr.2020.112910
36. Hays, SA, Rennaker, RL, and Kilgard, MP. How to fail with paired VNS therapy. Brain Stimul. (2023) 16:1252–8. doi: 10.1016/j.brs.2023.08.009
37. Kimberley, TJ, Prudente, CN, Engineer, ND, Pierce, D, Tarver, B, Cramer, SC, et al. Study protocol for a pivotal randomised study assessing vagus nerve stimulation during rehabilitation for improved upper limb motor function after stroke. Eur Stroke J. (2019) 4:363–77. doi: 10.1177/2396987319855306
38. Dawson, J, and Abdul-Rahim, AH. Paired vagus nerve stimulation for treatment of upper extremity impairment after stroke. Int J Stroke. (2022) 17:1061–6. doi: 10.1177/17474930221094684
39. Zhang, L, Zhang, X, Liu, Y, Wang, S, and Jia, G. Vagus nerve stimulation promotes the M1-to-M2 transition via inhibition of TLR4/NF-κB in microglial to rescue the reperfusion injury. J Stroke Cerebrovasc Dis. (2022) 31:106596. doi: 10.1016/j.jstrokecerebrovasdis.2022.106596
40. Chen, H, Feng, Z, Min, L, Tan, M, Zhang, D, Gong, Q, et al. Vagus nerve stimulation prevents endothelial necroptosis to alleviate blood-spinal cord barrier disruption after spinal cord injury. Mol Neurobiol. (2023) 60:6466–75. doi: 10.1007/s12035-023-03477-7
41. Yang, C, Hawkins, KE, Doré, S, and Candelario-Jalil, E. Neuroinflammatory mechanisms of blood-brain barrier damage in ischemic stroke. Am J Physiol Cell Physiol. (2019) 316:C135–53. doi: 10.1152/ajpcell.00136.2018
42. Morais, A, Liu, TT, Qin, T, Sadhegian, H, Ay, I, Yagmur, D, et al. Vagus nerve stimulation inhibits cortical spreading depression exclusively through central mechanisms. Pain. 161:1661–9. doi: 10.1097/j.pain.0000000000001856
43. Neren, D, Johnson, MD, Legon, W, Bachour, SP, Ling, G, and Divani, AA. Vagus nerve stimulation and other neuromodulation methods for treatment of traumatic brain injury. Neurocrit Care. (2016) 24:308–19. doi: 10.1007/s12028-015-0203-0
44. Kalagara, R, Chennareddy, S, Reford, E, Bhimani, AD, Cummins, DD, Downes, MH, et al. Complications of implanted vagus nerve stimulation: a systematic review and meta-analysis. Cerebrovasc Dis. (2024):112–20. doi: 10.1159/000536362
45. Kim, AY, Marduy, A, de Melo, PS, Gianlorenco, AC, Kim, CK, Choi, H, et al. Safety of transcutaneous auricular vagus nerve stimulation (taVNS): a systematic review and meta-analysis. Sci Rep. (2022) 12:22055. doi: 10.1038/s41598-022-25864-1
46. Kotagal, P. Neurostimulation: vagus nerve stimulation and beyond. Semin Pediatr Neurol. (2011) 18:186–94. doi: 10.1016/j.spen.2011.06.005
47. Hilz, MJ. Transcutaneous vagus nerve stimulation—a brief introduction and overview. Auton Neurosci. (2022) 243:103038. doi: 10.1016/j.autneu.2022.103038
48. Peuker, ET, and Filler, TJ. The nerve supply of the human auricle. Clin Anat. (2002) 15:35–7. doi: 10.1002/ca.1089
49. Badran, BW, Dowdle, LT, Mithoefer, OJ, NT, LB, Coatsworth, J, Brown, JC, et al. Neurophysiologic effects of transcutaneous auricular vagus nerve stimulation (taVNS) via electrical stimulation of the tragus: a concurrent taVNS/fMRI study and review. Brain Stimul. (2018) 11:492–500. doi: 10.1016/j.brs.2017.12.009
50. Badran, BW, and Austelle, CW. The future is noninvasive: a brief review of the evolution and clinical utility of vagus nerve stimulation. Focus. (2022) 20:3–7. doi: 10.1176/appi.focus.20210023
51. Redgrave, J, Day, D, Leung, H, Laud, PJ, Ali, A, Lindert, R, et al. Safety and tolerability of transcutaneous vagus nerve stimulation in humans; a systematic review. Brain Stimul. (2018) 11:1225–38. doi: 10.1016/j.brs.2018.08.010
52. Francisco, GE, Engineer, ND, Dawson, J, Kimberley, TJ, Cramer, SC, Prudente, CN, et al. Vagus nerve stimulation paired with upper-limb rehabilitation after stroke: 2- and 3-year follow-up from the pilot study. Arch Phys Med Rehabil. (2023) 104:1180–7. doi: 10.1016/j.apmr.2023.02.012
53. Szulczewski, MT. Transcutaneous auricular vagus nerve stimulation combined with slow breathing: speculations on potential applications and technical considerations. Neuromodulation. (2022) 25:380–94. doi: 10.1111/ner.13458
54. Engineer, ND, Kimberley, TJ, Prudente, CN, Dawson, J, Tarver, WB, and Hays, SA. Targeted vagus nerve stimulation for rehabilitation after stroke. Front Neurosci. (2019) 13:280. doi: 10.3389/fnins.2019.00280
55. Sun, JB, Tian, QQ, Yang, XJ, Deng, H, Li, N, Meng, LX, et al. Synergistic effects of simultaneous transcranial direct current stimulation (tDCS) and transcutaneous auricular vagus nerve stimulation (taVNS) on the brain responses. Brain Stimul. (2021) 14:417–9. doi: 10.1016/j.brs.2021.02.010
56. Ridengnaxi, E, and Wang, Y. Vagus nerve stimulation for improvement of vascular cognitive impairment. Neuropsychiatr Dis Treat. (2024) 20:1445–51. doi: 10.2147/NDT.S465249
57. Long, L, Zang, Q, Jia, G, Fan, M, Zhang, L, Qi, Y, et al. Transcutaneous auricular vagus nerve stimulation promotes white matter repair and improves dysphagia symptoms in cerebral ischemia model rats. Front Behav Neurosci. (2022) 16:811419. doi: 10.3389/fnbeh.2022.811419
58. Wang, Y, He, Y, Jiang, L, Chen, X, Zou, F, Yin, Y, et al. Effect of transcutaneous auricular vagus nerve stimulation on post-stroke dysphagia. J Neurol. (2023) 270:995–1003. doi: 10.1007/s00415-022-11465-5
59. Saway, BF, Palmer, C, Hughes, C, Triano, M, Suresh, RE, Gilmore, J, et al. The evolution of neuromodulation for chronic stroke: from neuroplasticity mechanisms to brain-computer interfaces. Neurotherapeutics. (2024) 21:e00337. doi: 10.1016/j.neurot.2024.e00337
60. Morrison, RA, Hays, SA, and Kilgard, MP. Vagus nerve stimulation as a potential adjuvant to rehabilitation for post-stroke motor speech disorders. Front Neurosci. (2021) 15:715928. doi: 10.3389/fnins.2021.715928
61. Thompson, SL, O’Leary, GH, Austelle, CW, Gruber, E, Kahn, AT, Manett, AJ, et al. A review of parameter settings for invasive and non-invasive vagus nerve stimulation (VNS) applied in neurological and psychiatric disorders. Front Neurosci. (2021) 15:709436. doi: 10.3389/fnins.2021.709436
62. Peng, X, Baker-Vogel, B, Sarhan, M, Short, EB, Zhu, W, Liu, H, et al. Left or right ear? A neuroimaging study using combined taVNS/fMRI to understand the interaction between ear stimulation target and lesion location in chronic stroke. Brain Stimul. (2023) 16:1144–53. doi: 10.1016/j.brs.2023.07.050
63. Melo, PSD, et al. A mechanistic analysis of the neural modulation of the inflammatory system through vagus nerve stimulation: a systematic review and meta-analysis. Neuromodulation. (2025) 28:43–53. doi: 10.1016/j.neurom.2024.03.002
Keywords: VNS, stroke, rehabilitation, knowledge map, bibliometric analysis
Citation: Deng J, Yang Z, Wang QM and Lv ZG (2025) Two decades of vagus nerve stimulation for stroke: a bibliometric analysis. Front. Neurol. 16:1531127. doi: 10.3389/fneur.2025.1531127
Received: 19 November 2024; Accepted: 21 March 2025;
Published: 04 April 2025.
Edited by:
Gianluigi M. Riva, Bocconi University, ItalyReviewed by:
Federico Villagra, Aberystwyth University, United KingdomCopyright © 2025 Deng, Yang, Wang and Lv. This is an open-access article distributed under the terms of the Creative Commons Attribution License (CC BY). The use, distribution or reproduction in other forums is permitted, provided the original author(s) and the copyright owner(s) are credited and that the original publication in this journal is cited, in accordance with accepted academic practice. No use, distribution or reproduction is permitted which does not comply with these terms.
*Correspondence: Zhi Gang Lv, eGludG9uZzExOEAxNjMuY29t
Disclaimer: All claims expressed in this article are solely those of the authors and do not necessarily represent those of their affiliated organizations, or those of the publisher, the editors and the reviewers. Any product that may be evaluated in this article or claim that may be made by its manufacturer is not guaranteed or endorsed by the publisher.
Research integrity at Frontiers
Learn more about the work of our research integrity team to safeguard the quality of each article we publish.