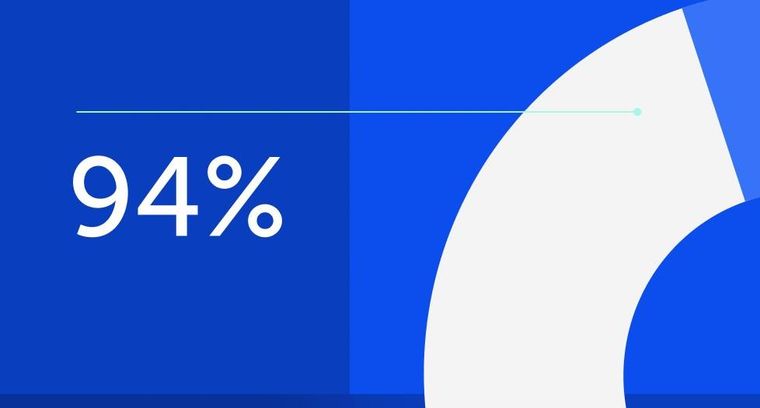
94% of researchers rate our articles as excellent or good
Learn more about the work of our research integrity team to safeguard the quality of each article we publish.
Find out more
STUDY PROTOCOL article
Front. Neurol., 11 March 2025
Sec. Stroke
Volume 16 - 2025 | https://doi.org/10.3389/fneur.2025.1501419
Introduction: Metacognition (MC) impairment is prevalent among stroke survivors but is frequently undiagnosed and untreated. MC impairment hinders stroke survivors’ ability to recognize their deficits, causing them to engage in activities that exceed their capabilities, set unrealistic performance goals and fail to use adaptive compensatory strategies. The present study will evaluate the clinical, neuropsychological and MRI correlates of MC impairment in a cohort of stroke survivors. The secondary objective is to describe the 12-month course of MC impairment.
Methods and analysis: The current study is a prospective cohort study. We will recruit 246 subjects. The project duration is 36 months. Subjects and carers will receive a detailed assessment at a research clinic at three, nine and 15 months after stroke onset (T1/T2/T3). The Chinese version of the Self-Awareness of Deficits Interview (SADI) will be completed by each subject. MC impairment is defined as any SADI subscale score of 2 or more. Potential covariate will be measured as well. Tests of executive functioning will be administered as well. Patients will be examined by MRI within 1 week after the onset of stroke. A stepwise logistic regression will be performed to assess the importance of lesions in the regions of interest. To examine neuropsychological functions in MC impairment, regression analysis of the SADI total and subscale scores will be performed using the significantly correlated neuropsychological functions as predictors. To examine the predictors of MC impairment remission, the demographic, clinical and MRI variables of remitters and non-remitters at T2/T3 will be examined by logistic regression.
Discussion: This project will be the first longitudinal study on MC impairment in stroke survivors. The results will shed light on the association between prefrontal cortex and subcortical lesions and MC impairment risk, symptom severity and outcome.
Metacognition (MC), originally described as the ability to “think about thinking,” includes a broad range of mental processes (1). MC involves the continuous integration of information leads to constructing, defining and refining a complex and evolving representation of the self and the others (1) MC is not a singular concept (2) MC is not intentional but include automatic proceedings (3). MC is not confineable to one person’s mind but its intersubjective in nature (4). MC is not just linked to thoughts about oneself, but are expandable to others (4). MC can be more comprehensively defined as an “umbrella concept” aggregating elements ranging from discrete cognitive processes to more elaborated functions which also include neurocognitive and social cognitive abilities (5).
In the context of stroke, metacognition refers to individuals’ conscious awareness of their cognitive, physical, or emotional functioning and the regulation of these activities through self-monitoring (6). The first major component of MC is self-awareness and the second component is the utilization of self-regulatory and compensatory strategies, including cognitive monitoring and cognitive control (6). Several comparable terms have emerged, such as self-awareness, self-monitoring, self-regulation, subjective cognitive complaints, metamemory or metacognitive awareness (6, 7). Crosson et al. (8) proposed a hierarchical model of self-awareness. The first level, intellectual awareness, is the ability to acknowledge impairment of a body function. Emergent awareness, the next level, is the ability to use this self-knowledge to identify problems with performance of everyday activities. Anticipatory awareness, the highest level of the hierarchy, is the ability to anticipate problems that are likely to occur during activity because of a specific deficit. Toglia and Kirk expanded on this model to include an element of metacognition. Their Dynamic Comprehensive Model of Awareness emphasizes online awareness, an interactive process of performance monitoring and self-regulation during an activity, including the ability to manage environmental and task demands. They also suggested that the components of awareness are less hierarchical and more interdependent (9). Patients with impaired self-awareness engage less in rehabilitation and less often use compensatory strategies (10). Therefore, it is not surprising that impaired self-awareness is associated with low rates of living independently, high rates of family stress, and poor vocational and community outcomes (10). MC impairment can be observed in relation to a wide variety of impairments and phenomena, ranging from physical/motor problems to cognitive deficits (e.g., poor memory) and behavioral disturbances (e.g., apathy) (11). MC impairment is common in patients with cerebral diseases such as dementia (12, 13), traumatic brain injury (11, 14) and stroke (15–17). For instance, in patients with traumatic head injury, 30–67% had MC impairment (11, 14).
MC impairments hinder stroke survivors’ ability to recognize their deficits. MC impairment causes stroke survivors to engage in activities that exceed their capabilities, set unrealistic performance goals and fail to use adaptive compensatory strategies (6). MC impairment contributes to poor therapeutic relationship and rehabilitation outcomes (18, 19), functional capacity (20–22) and recovery (23) in stroke survivors. Similarly, MC impairment is associated with unemployment (24), a poorer quality of life (25), reduced compliance with treatment (26), emotional symptoms and adjustments (27, 28) and behavioral disturbances (11) in patients with head injury. Maladaptive metacognition is associated with anxiety and depression symptoms post-stroke (29). Therefore, MC impairment could be considered as a focus of stroke assessment and rehabilitation (6, 22, 30, 31).
Metacognition impairment appears to be common among stroke survivors. Although small-scale studies reported that 35–46% survivors were affected (15, 18, 32, 33), the prevalence of MC impairment in local stroke survivors remains unknown. The clinical correlates of MC impairment associated with stroke include age (34), a lower educational level, stroke severity, cognitive reserve and impairment (32), verbal memory deficits (31), and anxiety and depressive symptoms (28, 35). Correlates of MC impairment in head injury include younger age (36), severity of injury (34, 36), anxiety and depressive symptoms (25, 37, 38) and executive function (6, 34, 39). Neuroimaging correlates of the severity of MC impairment are unknown; however, the severity of post-stroke depression was shown to correlate with the presence of cerebromicrobleeds (40). Similarly, the remission rate of MC impairment associated with stroke is unknown, although small-scale studies suggested improvements during the first year after stroke (20, 41). Our previous research data yielded a 12-month non-remission rate of 66% for post-stroke depression (PSD), another neuropsychiatric condition, and the clinical correlates of persistent PSD include the baseline severity of depression, severity of stroke and cognitive functioning (42).
The MC impairment is often undiagnosed and thus untreated. Interventions like omega-3 supplementation, viloxazine, and atomoxetine may improve MC in youth with mood disorders or in adults with attention deficit/hyperactivity disorder (43–45). No pharmacological treatment has been found to effectively treat MC impairment in patients with neurological disorders. However, awareness interventions (10, 46, 47), virtual reality games (48, 49) and feedback interventions (50) may be useful treatments for MC impairment. Specific psychotherapies for MC impairment include metacognitive reflection and insight therapy (51), metacognitive grief therapy (52) and metacognitive executive function training (53).
Studies on healthy individuals and lesion studies have suggested that the following areas are important for MC: the orbitofrontal cortex (OFC), dorsolateral prefrontal cortex (DLPC), anterior and posterior cingulate cortex (ACC and PCC), anterior insula and thalamus, particularly the right side (12, 54, 55). MC impairment in dementia is related to OFC and PCC atrophy, hypometabolism and reduced connectivity (12, 54, 56). Lesion studies have suggested that ACC is involved in MC impairment (57). Functional imaging studies indicate that OFC, DLFC, ACC, and PCC is involved with introspection in healthy participants and patients with traumatic brain injury and dementia (12, 54, 56, 58). MC impairment in dementia is related to atrophy of the insula (54, 56). Finally, MC impairment is related to right/bilateral thalamic lesion in stroke (59, 60) and to thalamic atrophy in dementia (54). Our team previously reported an association of thalamic lesions with emotional lability after stroke (61).
Very few structural brain imaging studies have been published on MC impairment in stroke (18, 32, 57, 59, 62). Case reports have linked MC impairment in stroke to infarcts of the right anterior cingulate cortex and right thalamus (57, 59). Cross-sectional studies have associations between MC impairment in stroke and hemispheric lesions (32), lesions in the frontal and temporal lobes, and with lesion size (18, 62). The limitations of these studies include small sample sizes (18, 57, 59), selective sample (62); the lack of a standardized assessment of MC impairment (18, 32) or a detailed examination of infarct locations (18, 32) and no follow-up assessment (18, 32, 57, 59).
The objectives of the proposed study will contribute to the evaluation of the clinical, neuropsychological and MRI correlates and 12-month course of MC impairment in a cohort of stroke survivors. The regions of interest (ROIs) will include the right OFC, DFPC, ACC, PCC, anterior insula and thalamus, and the occipital lobe will be the control region.
The first hypothesis is that subjects with MC impairment will harbor more infarcts relative to unimpaired subjects in the ROIs, but not in the control region. The second hypothesis is that there will be a significant positive correlation between the number and volume of infarcts in the ROIs and the severity of MC impairment. The third hypothesis is that subjects with MC impairment will exhibit poorer executive functioning. A positive correlation is predicted between the Self-Awareness of Deficits Interview (SADI) total and subscale scores and executive function in the MC impairment group. The fourth hypothesis is that 66% (42) of subjects with MC impairment at baseline will continue to exhibit MC impairment at 12 months after the first assessment. The fifth hypothesis is that the baseline severity of MC impairment, severity of stroke and level of executive functioning will predict the persistence of MC impairment (42).
The planned study is a prospective nested case–control study of stroke survivors. Details of recruitment are shown in Figure 1. Patients will be recruited from the Acute Stroke Unit (ASU) of the Prince of Wales Hospital in Hong Kong. The ASU treats approximately 93% of all acute stroke patients admitted to the hospital, with the majority of the remaining 7% sent to the neurosurgery unit. A research assistant will visit the ASU daily to identify all eligible patients and obtain their written consent. All acute stroke patients (n = 600) consecutively admitted to the ASU over a 13-month period will be invited to participate. A research assistant will visit the ASU daily to identify eligible patients and to obtain written consent. Approximately 80% of the 600 patients (480) will have ischaemic stroke. MRI examination will be contraindicated in 10% of patients, leaving 432 potential subjects (480 × 90%). According to our previous findings (63), the mortality rate at 3 months post-stroke is around 12%, leaving 380 [432 × (100% − 12%)] potential subjects. Of these, 25% will not meet the inclusion criteria (63). Hence, the number of possible subjects will be around 285 [380 × (100% − 25%)] (63). Assuming a dropout rate of 20%, 228 [285 × (100–20%)] patients will complete the 12-month follow-up assessment. A healthy control group (n = 285) matched by age, sex, years of education, socioeconomic factors and ethnicity will be recruited from the community. There is no overlap between the patient samples that will be included in the current manuscript and the other manuscript (MS ID 1451431).
The inclusion criteria are:
1. Age >/= 18 years with no upper age limit;
2. Either male or female;
3. Chinese ethnicity;
4. Right handed;
5. Well-documented acute first ischaemic stroke affecting either the right or left hemisphere occurring within a maximum of 7 days prior to admission; and.
6. The ability and willingness to give informed consent.
The exclusion criteria are:
1. Previous history of epilepsy, head injury, hydrocephalus, intracranial tumor, Parkinson’s disease, dementia or other neurological disease(s) other than stroke;
2. History or current diagnosis of depression, bipolar disorder, schizophrenia or alcohol/substance abuse/dependence;
3. Dementia, defined as a Mini-Mental State Examination score below 17 for illiterate individuals, 20 for those with 1–6 years of education and 24 for those with 7 or more years of education (64);
4. Severely impaired language comprehension or expression that precludes a detailed neuropsychological assessment (54);
5. Contraindications for MRI, such as a pacemaker in situ, physical frailty or severe claustrophobia;
6. Lack of a caregiver; and
7. Recurrence of stroke prior to the 3-month assessment. For the control group, the exclusion criteria will include a history of stroke.
Details of the data collection schedule are shown in Table 1. Written consent from patients or relatives (by proxy) will be obtained. The number of exclusions and reasons for them will be recorded. The following demographic, psychosocial and medical data of all participants will be collected: age, sex, education, prior history of hypertension, diabetes mellitus and hyperlipidemia and psychiatric disease and date of onset of stroke. The subjects’ clinical data and information on neurological impairment, including dysarthria, measured by the National Institute of Health Stroke Scale (NIHSS) (65), will be extracted from the Stroke Registry. The Stroke Registry is maintained by a full-time, well-trained research nurse. The following strategies will be implemented to reduce attrition: (a) reminder emails and letters will be sent to subjects; (b) five hundred Hong Kong dollars will be offered upon the completion of all follow up assessments.
Three months after the onset of the index stroke (T1), the patients and their carers will undergo the following assessments at a research clinic. The planned timing of the assessment is consistent with other studies of MC impairment in stroke (20, 33).
A trained research assistant (RA) blinded to the subjects’ radiological data will conduct interviews at a research clinic. MC impairment will be assessed based on interviews with the subjects, using the Chinese version of the SADI. The RA will measure each patient’s level of functioning using the Functional Independence Measure (FIM) (66), Instrumental Activities of Daily Living Scale (IADL) (67) and Barthel Index (BI) (68). To assist with scoring, a carer will also complete a checklist regarding the subject’s post-stroke changes, as recommended by Fleming et al. (69).
The SADI is a standardized instrument used to assess the nature of self-identified goals and the ability to set realistic goals. This interviewer-scored, semi-structured interview assesses MC using three subscales, including (i) self-awareness of deficits; (ii) self-awareness of functional implications of deficits and (iii) the ability to set realistic goals (69). Each component is scored by a therapist on a four-point Likert scale ranging from zero (no awareness) to three (complete awareness). An example question is “Are you any different now, compared to what you were like before the stroke?” For each subscale, lower scores (0–1) reflect moderate to high MC. Conversely, higher scores (2–3) reflect a low MC (i.e., tendency to deny or minimize the extent of difficulties experienced) (46). The three sub-scale scores can be summed to yield a SADI total score that ranges from 0 to 9, with higher scores indicating lower metacognitive awareness. The duration of administration is 20–30 min (70). MC impairment is defined as any SADI subscale score of 2 or more (46, 71). The interrater reliability coefficients were found to be 0.78, 0.57 and 0.78 for the three subscales and 0.82 for the total SADI score (69). The construct validity of the SADI is supported by significant correlations of this instrument with other standardized measures of self-awareness (27, 72). The SADI also has satisfactory inter-rater (0.82) and test–retest reliability (0.85–0.86) (73) and responsiveness (70). The SADI has previously been used to assess MC impairment in stroke patients (10, 59), and is currently the only standardized self-awareness measure that investigates a client’s ability to set realistic goals (69). The Dysexecutive Questionnaire score (74) was not found to correlate with the SADI score (72). Other scales used to measure MC impairment, such as the Patient Competency Rating Scale (75) and Self-Regulation and Skills Interview (46), have not been translated into and validated in Chinese; whereas the Awareness Questionnaire (76, 77) has not been applied in stroke patients.
A trained RA, blind to the subjects’ radiological data, will administer the following neuropsychological tests (Table 1).
1. The Chinese version of the Montreal Cognitive Assessment (MoCA) (78) measures global cognitive functions. MoCA is a 30-point scale that covers multiple cognitive domains including spatiotemporal orientation, sustained attention, visuospatial function, executive function, verbal memory, language, naming, and abstract thinking. MoCA showed adequate sensitivity and specificity for the detection of post-stroke cognitive impairment (78).
2. The Digit Span Test (79) assesses attention and working memory. This test requires subjects to repeat series of digits of increasing length. Digit span forward is a good measure of simple attention, and most healthy individuals perform within the seven plus/minus two span of apprehension range. The digit backward sub-score, which ranges from 0 to 14, will be used as the index of working memory. A higher score reflects superior performance. Digit Span Test has been commonly applied in stroke studies (80).
3. The Hong Kong List Learning Test is used to assess memory (81). This test, which is based on the California Verbal Learning Test, presents 16 words in 3 learning trials, followed by a 10-min delayed recall and 30-min delayed recall and recognition test. It has been validated in both normal and clinical samples (81). The Hong Kong List Learning Test has been applied in local patients with stroke and neurocognitive disorders (82, 83).
1. The Go–NoGo test (84) assesses response selection/inhibition. A motor response (pressing a button as quickly as possible) is either initiated (Go) or inhibited (NoGo), depending whether a stimulus comprising a green square (Go) or red square (NoGo) appears on a computer screen. Errors of omission (misses) and commission (false alarms) and the reaction time needed to correct trials during the experimental condition are recorded. The Go–NoGo test had been applied in patients with stroke (85, 86).
2. The Wisconsin Card Sorting Test (87) is composed of 4 stimulus cards and 64 response cards. The response cards differ in three dimensions: color (red, green, yellow, or blue), pattern (triangle, star, cross, or circle) and number (one, two, three, or four). The participant is asked to work out a sorting principle for matching each response card to the four stimulus cards (one red triangle, two green stars, three yellow crosses or four blue circles) according to the feedback given by the examiner (correct or incorrect). Once the participant has made 10 consecutive correct matches to the sorting principle, the sorting principle is changed without warning and the participant is required to work out a new sorting principle. The test is terminated when the participant has: (1) successfully maintained 6 correct sorting principles (color, pattern, number, color, pattern, number) or (2) made 128 attempts. To evaluate the participant’s abilities to use abstract reasoning and shift cognitive strategies, each response is recorded as either correct, a perseverative response, a perseverative error or a non-perseverative error for subsequent scoring. The number of completed categories, total number of attempts and number of perseverative errors are selected as the index scores. The Wisconsin Card Sorting Test has been validated and applied in patients with stroke (88, 89).
A trained RA will measure the level of depressive and anxiety symptoms using the Beck Depression Inventory (BDI) (90) and the anxiety subscale of the Hospital Anxiety Depression Scale (HASDA) (91), respectively. BDI is a 21-item, self-report rating inventory that measures characteristic attitudes and symptoms of depression. The BDI takes approximately 10 min to complete. HADSA is 7-item scale. Each item scores on a 4-point Likert scale (e.g., as much as I always do [0]; not quite so much; definitely not so much; and not at all), giving maximum subscale scores of 21. The questionnaire assesses symptoms over the preceding week.
Follow-up assessments of MC impairment will be conducted on all stroke patients at 9 months (T2) and 15 months (T3) post-stroke, or 6 and 12 months after the first assessment. SADI, FIM, BI, MoCA, BDI and HADSA will be repeated during the follow-up assessment.
Patients will be examined by MRI within 1 week after the onset of stroke. All scans will be performed using a 3 T scanner (Philips Achieva 3.0T, X Series, Quasar Dual MRI System). Standardized sequences includes diffusion weighted imaging (DWI), 3D T1-weighted, T2-weighted, fluid attenuated inversion recovery (FLAIR) and susceptibility-weighted imaging (SWI). An experienced neuroradiologist blind to the subjects’ MC impairment status will assess the MRI images. Acute infarct will be defined as a hyperintensive lesion on DWI with corresponding hypointensity on the ADC map. White matter hyperintensities (WMH) will be defined as hyperintensities 5 mm that are ill defined on FLAIR images, but are isointense with normal brain parenchyma on T1 weighted images. Lesions equivalent to the signal characteristics of cerebrospinal fluid on T1-weighted images and measuring more than 3 mm in diameter, and also wedge-shaped cortico-subcortical lesions, will be regarded as old/lacunar infarcts. Microbleeds will be defined as dot-like hypointensities on SWI. The total number of microbleeds will be determined. All raw data will be transferred to the PALS system (Carestream Solutions).
This will include non-uniformity correction (92), spatial standardization and brain extraction (excluding the skull). To ensure the brain structure volumes are comparable among subjects, the MRI data of each subject will be transformed from the original space to a common stereotactic space using multi-scale affine registration (93). Brain regions will be automatically segmented from the head MRI data using the brain extraction tool (94).
Brain tissue will be classified into grey matter, white matter and cerebrospinal fluid (95). Whole-brain segmentation will be achieved using an atlas-based approach (96), which automatically adjusts the existing atlas intensity model to newly inputted data. The ROI and other brain regions will be segmented and their volumes quantified using the Talairach brain atlas (97) and Daemon registration.
Infarcts will be delineated semi-automatically as high-intensity regions on DWI images and WMH as high-density regions on FLAIR images (and isointense on T1 weighted images) using ITK-SNAP software. The segmented infarct and WMH regions will be combined with the ROI and other brain-region masks generated in the previous step. The infarct and WMH pixels that fall within the ROI and other brain regions can then be calculated.
Two hundred and eighty-five patients will be recruited. A previous report of MC impairment in stroke found that 47% of patients with MC impairment presented with frontal infarcts, compared to only 10% of patients without MC impairment (18). The corresponding effect size of frontal infarct would then be 0.415. A sample size of 285 will have a power of 99% for the identification of frontal infarcts as a predictor of MC impairment in stroke, based on a chi square test with one degree of freedom (98).
Using 46% (36) as an estimate of the frequency of MC impairment, 131 (285 × 46%) cases will be identified. A sample size of 131 subjects with MC impairment would provide a power of 80% (99) for detecting any correlate of the SADI total score with a correlation value of 0.14. This magnitude of correlation is considerably lower than the reported associations between the severity of MC impairment and severity of injury (0.40) (100), emotions (0.21) (25), verbal memory deficits (0.81) (31) number of lesions (0.30) (58) and executive functions measured with Wisconsin Card Sorting Test perseverative responses and errors (0.28–0.31) (39) and Go–NoGo test omission and commission errors (0.19–0.28) (101).
Assuming a drop-out rate of 20%, 105 (131 × 80%) patients with MC impairment will attend the follow-ups. Although the remission rate of MC impairment is unknown, previous studies have reported remission rates of 35–50% in PSD (42). Therefore, this sample will provide a power of 80% for identifying any predictor with an odds ratio of 2.0. This odds ratio is lower than the figure of 5.3 reported in our previous work on cerebral microbleeds and PSD outcomes (42), assuming that the R2 of the other variables (stroke severity, depressive symptoms, cognitive function) is 0.21 (42) in the multivariate logistic regression (98).
Missing data will be handled by the last observation carried forward method. All of the variables will be tested for normality using Kolmogorov–Smirnov tests with a significance threshold of p < 0.05. We will first compare the SADI and other neuropsychological test scores between stroke subjects and normal controls. To examine the correlates of MC impairment, the demographic, clinical and MRI variables (age, education, prior history of hypertension, diabetes mellitus, hyperlipidemia and psychiatric disease, NIHSS, HADSA BDI, MoCA, ROI infarcts, microbleeds and WMH volumes) will be compared between patients with and without MC impairment at T1. The χ2 test, Student’s t-test or Mann–Whitney U test will be applied as appropriate. A stepwise logistic regression will be performed to assess the importance of lesions in the ROIs, together with other significant variables in the above univariate analyses, effect sizes and confidence intervals will be computed. For the patients with MC impairment at T1, the SADI total and subscale scores for groups with and without ROI infarcts will be compared using a covariance analysis.
To examine neuropsychological functioning in MC impairment, the performance of the groups with MC impairment, without MC impairment and the normal controls on executive and basic cognitive function tasks will be compared using analysis of variance. The correlation between the SADI total and subscale scores and the performance of the group with MC impairment on the above neuropsychological tests will be computed using Pearson’s or Spearman’s correlation coefficients, as appropriate. Finally, a regression analysis of the SADI total and subscale scores will be performed using significantly correlated neuropsychological functions as predictors. To examine the interplay between lesion location and severity, executive function and depressive symptoms, interaction terms will be created and analyzed.
To examine the predictors of remission of MC impairment, the demographic, clinical and MRI variables of remitters and non-remitters at T2/T3 will be examined using logistic regression. We will also test a series of generalized estimating equation models to evaluate the associations of clinical and brain MRI characteristics with the risk of MC impairment across all follow-up assessments (T1/T2/T3). First, we will run a univariate model to fit a logistic regression. Next, we will examine the associations between the demographic variables, concurrent medical conditions and risk of MC impairment. The second model will include the baseline SADI scores, NIHSS scores and cognitive and executive function scores. Brain MRI characteristics will be entered in the final model, effect sizes and confidence intervals will be computed. The level of significance will be set at 0.05.
Structural equation modeling will be applied to help elucidate causal pathways to remission of MC impairment. A priori model is constructed with explicit root causes (demographic, clinical and MRI variables) and mediators (MC impairment severity, depressive and anxiety symptoms, functional impairments) leading to remission. Following structural equation modeling, a posteriori model is selected based on data fit and clinical plausibility.
We try to achieve a homologous patient population by narrowing the age, ethnicity, handedness and duration of MC impairment. Patients with other causes of MC impairment, such as psychiatric or neurological disorders are excluded. This project will be the first longitudinal study to examine the role of OFC, DFPC, ACC, PCC, anterior insula, amygdala and thalamus in a large sample of consecutively admitted stroke survivors with MC impairment. Compared to previous neuroimaging, this study has large sample size, standardized assessment of MC impairment, detailed examination of infarct locations and no follow-up assessment. The results will shed light on the association between the above brain regions and MC impairment risk. They are thus likely to be applicable to the large population of neurological patients at risk of MC impairment.
The findings should also stimulate and guide further research in this field. For example, the information on neuroimaging correlates will guide the design of functional imaging studies of brain network involved in MC impairment. Whereas knowledge on executive function impairment may facilitate the design of potential non-pharmacological treatment. Finally, information on the rate and predictors of remission will provide useful information on clinical trials.
The findings regarding the relationship between MC impairment and location of infarcts and executive function may have important clinical implications. In fact, studies have shown that the lack of awareness is related to more difficulties with treatment adherence, poorer rehabilitation outcomes and greater emotional symptoms, behavioral disturbances and unemployment, caregiver burden, all of which increase the financial impact on society (11, 19, 24, 26, 27). Interventions that decrease MC impairment may eventually bring important clinical benefits. For instance, the information on the location of infarcts may prompt development of stimulation paradigms of specific brain regions using repetitive transcranial magnetic stimulation or transcranial direct current stimulation. These types interventions have been tested in patients with depression after stroke (102, 103). Alternatively, data on executive function deficits may suggest the possibility of interventions on improving executive functions. This type of cognitive rehabilitation has already been tested in patients with depression after stroke (104).
This study has some limitations. Patients of non-Chinese ethnicity. Who cannot give consent because of dementia or aphasia-associated left-side infarcts, were excluded. This selection bias may limit the generalizability of the findings. Furthermore, unmeasured confounders may affect the results. Finally, none of the patients will receive treatment for their MC impairment.
The current manuscript focuses solely on MC impairment in stroke, while a separate study published in Front. Psychol. – Neuropsychology (105) will examine loss of empathy in stroke only. MC impairment and loss of empathy are different domains of neuropsychiatric deficits. The patient cohorts that will be used in these two manuscripts will be entirely different.
The study protocol will provide a comprehensive overview of the predictors, latent factors, and outcomes of MC impairment in stroke patients. It will also inform clinical practice and advance the rehabilitation of stroke.
The final protocol was approved by the Joint Chinese University of Hong Kong-New Territories East Cluster Clinical Research Ethics Committee, Hong Kong SAR, China (reference number: 2023.427). Written informed consent will be obtained from each participant.
WKT: Conceptualization, Methodology, Writing – original draft, Writing – review & editing. EH: Conceptualization, Methodology, Writing – review & editing. TWHL: Conceptualization, Methodology, Writing – review & editing.
The author(s) declare that no financial support was received for the research, authorship, and/or publication of this article.
The authors declare that the research was conducted in the absence of any commercial or financial relationships that could be construed as a potential conflict of interest.
The author(s) declared that they were an editorial board member of Frontiers, at the time of submission. This had no impact on the peer review process and the final decision.
All claims expressed in this article are solely those of the authors and do not necessarily represent those of their affiliated organizations, or those of the publisher, the editors and the reviewers. Any product that may be evaluated in this article, or claim that may be made by its manufacturer, is not guaranteed or endorsed by the publisher.
1. Lysaker, PH, Minor, KS, Lysaker, JT, Hasson-Ohayon, I, Bonfils, K, Hochheiser, J, et al. Metacognitive function and fragmentation in schizophrenia: relationship to cognition, self-experience and developing treatments. Schizophr Res Cogn. (2020) 19:100142. doi: 10.1016/j.scog.2019.100142
2. Martiadis, V, Pessina, E, Raffone, F, Iniziato, V, Martini, A, and Scognamiglio, P. Metacognition in schizophrenia: a practical overview of psychometric metacognition assessment tools for researchers and clinicians. Front Psych. (2023) 14:1155321. doi: 10.3389/fpsyt.2023.1155321
3. Hasson-Ohayon, I, Gumley, A, McLeod, H, and Lysaker, PH. Metacognition and intersubjectivity: reconsidering their relationship following advances from the study of persons with psychosis. Front Psychol. (2020) 11:567. doi: 10.3389/fpsyg.2020.00567
4. Semerari, A, Carcione, A, Dimaggio, G, Falcone, M, Nicolò, G, Procacci, M, et al. How to evaluate metacognitive functioning in psychotherapy? The metacognition assessment scale and its applications. Clin Psychol Psychother. (2003) 10:238–61. doi: 10.1002/cpp.362
5. Fekete, Z, Vass, E, Balajthy, R, Tana, Ü, Nagy, AC, Oláh, B, et al. Efficacy of metacognitive training on symptom severity, neurocognition and social cognition in patients with schizophrenia: a single-blind randomized controlled trial. Scand J Psychol. (2022) 63:321–33. doi: 10.1111/sjop.12811
6. Al Banna, M, Redha, NA, Abdulla, F, Nair, B, and Donnellan, C. Metacognitive function poststroke: a review of definition and assessment. J Neurol Neurosurg Psychiatry. (2015) 87:161–6. doi: 10.1136/jnnp-2015-310305
7. Richardson, C, McKay, A, and Ponsford, JL. The trajectory of awareness across the first year after traumatic brain injury: the role of biopsychosocial factors. Brain Inj. (2014) 28:1711–20. doi: 10.3109/02699052.2014.954270
8. Crosson, B, Barco, PP, Velozo, CA, Bolesta, MM, Cooper, PV, Werts, D, et al. Awareness and compensation in postacute head injury rehabilitation. J Head Trauma Rehabil. (1989) 4:46–54. doi: 10.1097/00001199-198909000-00008
9. Toglia, J, and Kirk, U. Understanding awareness deficits following brain injury. NeuroRehabilitation. (2000) 15:57–70. doi: 10.3233/NRE-2000-15104
10. Kersey, J, Juengst, SB, and Skidmore, E. Effect of strategy training on self-awareness of deficits after stroke. Am J Occup Ther. (2019) 73:7303345020p1–7. doi: 10.5014/ajot.2019.031450
11. Bach, LJ, and David, AS. Self-awareness after acquired and traumatic brain injury. Neuropsychol Rehabil. (2006) 16:397–414. doi: 10.1080/09602010500412830
12. Wilson, RS, Sytsma, J, Barnes, LL, and Boyle, PA. Anosognosia in Dementia. Curr Neurol Neurosci Rep. (2016) 16:77. doi: 10.1007/s11910-016-0684-z
13. Mograbi, DC, Huntley, J, and Critchley, H. Self-awareness in dementia: a taxonomy of processes, overview of findings, and integrative framework. Curr Neurol Neurosci Rep. (2021) 21:69. doi: 10.1007/s11910-021-01155-6
14. Dromer, E, Kheloufi, L, and Azouvi, P. Impaired self-awareness after traumatic brain injury: a systematic review. Part 1: assessment, clinical aspects and recovery. Ann Phys Rehabil Med. (2021) 64:101468. doi: 10.1016/j.rehab.2020.101468
15. Boosman, H, van Heugten, CM, Winkens, I, Heijnen, VA, and Visser-Meily, JM. Awareness of memory functioning in patients with stroke who have a good functional outcome. Brain Inj. (2014) 28:959–64. doi: 10.3109/02699052.2014.888763
16. Deotto, A, Westmacott, R, Fuentes, A, deVeber, G, and Desrocher, M. Does stroke impair academic achievement in children? The role of metacognition in math and spelling outcomes following pediatric stroke. J Clin Exp Neuropsychol. (2018) 41:257–69. doi: 10.1080/13803395.2018.1533528
17. Wheeler, M, Williams, OA, Johns, L, Chiu, EG, Slavkova, ED, and Demeyere, N. Unravelling the complex interactions between self-awareness, cognitive change, and mood at 6-months post-stroke using the Y-shaped model. Neuropsychol Rehabil. (2022) 33:680–702. doi: 10.1080/09602011.2022.2042329
18. Hartman-Maeir, A, Soroker, N, Oman, SD, and Katz, N. Awareness of disabilities in stroke rehabilitation--a clinical trial. Disabil Rehabil. (2003) 25:35–44. doi: 10.1080/713813429
19. O’Connell, EL, Lawson, DW, New, PW, and Stolwyk, RJ. Agreement between patients and nurses of neurobehavioral disability following stroke in an inpatient rehabilitation setting. Disabil Rehabil. (2020) 42:2868–75. doi: 10.1080/09638288.2019.1572792
20. Ekstam, L, Uppgard, B, Kottorp, A, and Tham, K. Relationship between awareness of disability and occupational performance during the first year after a stroke. Am J Occup Ther. (2007) 61:503–11. doi: 10.5014/ajot.61.5.503
21. McKay, C, Rapport, LJ, Coleman Bryer, R, and Casey, J. Self-evaluation of driving simulator performance after stroke. Top Stroke Rehabil. (2011) 18:549–61. doi: 10.1310/tsr1805-549
22. Skidmore, ER, Swafford, M, Juengst, SB, and Terhorst, L. Self-awareness and recovery of Independence with strategy training. Am J Occup Ther. (2017) 72:7201345010p1–5. doi: 10.5014/ajot.2018.023556
23. Gialanella, B, Monguzzi, V, Santoro, R, and Rocchi, S. Functional recovery after hemiplegia in patients with neglect. Stroke. (2005) 36:2687–90. doi: 10.1161/01.str.0000189627.27562.c0
24. Kelley, E, Sullivan, C, Loughlin, JK, Hutson, L, Dahdah, MN, Long, MK, et al. Self-awareness and neurobehavioral outcomes, 5 years or more after moderate to severe brain injury. J Head Trauma Rehabil. (2014) 29:147–52. doi: 10.1097/htr.0b013e31826db6b9
25. Sasse, N, Gibbons, H, Wilson, L, Martinez-Olivera, R, Schmidt, H, Hasselhorn, M, et al. Self-awareness and health-related quality of life after traumatic brain injury. J Head Trauma Rehabil. (2013) 28:464–72. doi: 10.1097/htr.0b013e318263977d
26. Schönberger, M, Humle, F, and Teasdale, TW. The development of the therapeutic working Alliance, patients’ awareness and their compliance during the process of brain injury rehabilitation. Brain Inj. (2006) 20:445–54. doi: 10.1080/02699050600664772
27. Ownsworth, T, and Fleming, J. The relative importance of metacognitive skills, emotional status, and executive function in psychosocial adjustment following acquired brain injury. J Head Trauma Rehabil. (2005) 20:315–32. doi: 10.1097/00001199-200507000-00004
28. Donnellan, C, Al Banna, M, Redha, N, Al Sharoqi, I, Al-Jishi, A, Bakhiet, M, et al. Association between metacognition and mood symptoms poststroke. J Geriatr Psychiatry Neurol. (2016) 29:212–20. doi: 10.1177/0891988716640374
29. Pedersen, SG, Anke, A, Friborg, O, Ørbo, MC, Løkholm, MT, Kirkevold, M, et al. Metacognitive beliefs, mood symptoms, and fatigue four years after stroke: an explorative study. PLoS One. (2024) 19:e0305896. doi: 10.1371/journal.pone.0305896
30. Leung, DPK, and Liu, KPY. Review of self-awareness and its clinical application in stroke rehabilitation. Int J Rehabil Res. (2011) 34:187–95. doi: 10.1097/mrr.0b013e3283487f31
31. Barrett, AM, Galletta, EE, Zhang, J, Masmela, JR, and Adler, US. Stroke survivors over-estimate their medication self-administration (MSA) ability, predicting memory loss. Brain Inj. (2014) 28:1328–33. doi: 10.3109/02699052.2014.915984
32. Santos, CO, Caeiro, L, Ferro, JM, Albuquerque, R, and Figueira, ML. Denial in the first days of acute stroke. J Neurol. (2006) 253:1016–23. doi: 10.1007/s00415-006-0148-5
33. Björkdahl, A, Lundgren-Nilsson, Å, and Sunnerhagen, KS. How can we tell who is aware? Where does the veracity lie? J Stroke Cerebrovasc Dis. (2012) 21:812–8. doi: 10.1016/j.jstrokecerebrovasdis.2011.04.013
34. Zimmermann, N, Mograbi, DC, Hermes-Pereira, A, Fonseca, RP, and Prigatano, GP. Memory and executive functions correlates of self-awareness in traumatic brain injury. Cogn Neuropsychiatry. (2017) 22:346–60. doi: 10.1080/13546805.2017.1330191
35. Gil-Pagés, M, Sánchez-Carrión, R, Tormos, JM, Enseñat-Cantallops, A, and García-Molina, A. A positive relationship between cognitive reserve and cognitive function after stroke: dynamic proxies correlate better than static proxies. J Int Neuropsychol Soc. (2019) 25:910–21. doi: 10.1017/s1355617719000638
36. Lloyd, O, Ownsworth, T, Fleming, J, Jackson, M, and Zimmer-Gembeck, M. Impaired self-awareness after pediatric traumatic brain injury: protective factor or liability? J Neurotrauma. (2021) 38:616–27. doi: 10.1089/neu.2020.7191
37. McBrinn, J, Colin Wilson, F, Caldwell, S, Carton, S, Delargy, M, McCann, J, et al. Emotional distress and awareness following acquired brain injury: an exploratory analysis. Brain Inj. (2008) 22:765–72. doi: 10.1080/02699050802372208
38. Richardson, C, McKay, A, and Ponsford, JL. Factors influencing self-awareness following traumatic brain injury. J Head Trauma Rehabil. (2015) 30:E43–54. doi: 10.1097/htr.0000000000000048
39. Ciurli, P, Bivona, U, Barba, C, Onder, G, Silvestro, D, Azicnuda, E, et al. Metacognitive unawareness correlates with executive function impairment after severe traumatic brain injury. J Int Neuropsychol Soc. (2010) 16:360–8. doi: 10.1017/s135561770999141x
40. Tang, WK, Chen, YK, Lu, JY, Chu, WCW, Mok, VCT, Ungvari, GS, et al. Cerebral microbleeds and symptom severity of post-stroke depression: a magnetic resonance imaging study. J Affect Disord. (2011) 129:354–8. doi: 10.1016/j.jad.2010.08.007
41. Turner, BJ, Ownsworth, TL, Turpin, M, Fleming, JM, and Griffin, J. Self-identified goals and the ability to set realistic goals following acquired brain injury: a classification framework. Aust Occup Ther J. (2008) 55:96–107. doi: 10.1111/j.1440-1630.2007.00660.x
42. Tang, WK, Chen, Y, Liang, H, Chu, WC, Mok, VC, Ungvari, GS, et al. Cerebral microbleeds as a predictor of 1-year outcome of Poststroke depression. Stroke. (2014) 45:77–81. doi: 10.1161/strokeaha.113.002686
43. Lee, SI, Song, D-H, Shin, DW, Kim, JH, Lee, YS, Hwang, J-W, et al. Efficacy and safety of atomoxetine hydrochloride in Korean adults with attention-deficit hyperactivity disorder. Asia Pac Psychiatry. (2014) 6:386–96. doi: 10.1111/appy.12160
44. Vesco, AT, Young, AS, Arnold, LE, and Fristad, MA. Omega-3 supplementation associated with improved parent-rated executive function in youth with mood disorders: secondary analyses of the omega 3 and therapy (OATS) trials. J Child Psychol Psychiatry. (2017) 59:628–36. doi: 10.1111/jcpp.12830
45. Nasser, A, Hull, JT, Liranso, T, Fry, N, Cutler, AJ, Rubin, J, et al. Authors’ reply to Singh and Balasundaram: comment on “a phase III, randomized, double-blind, placebo-controlled trial assessing the efficacy and safety of Viloxazine extended-release capsules in adults with attention-deficit/hyperactivity disorder”. CNS Drugs. (2022) 36:1333–5. doi: 10.1007/s40263-022-00967-5
46. Ownsworth, TL, McFarland, K, and RossD, MY. Self-awareness and psychosocial functioning following acquired brain injury: an evaluation of a group support Programme. Neuropsychol Rehabil. (2000) 10:465–84. doi: 10.1080/09602010050143559
47. Jaywant, A, Steinberg, C, Lee, A, and Toglia, J. Feasibility and acceptability of the multicontext approach for individuals with acquired brain injury in acute inpatient rehabilitation: a single case series. Neuropsychol Rehabil. (2020) 32:211–30. doi: 10.1080/09602011.2020.1810710
48. Llorens, R, Navarro, MD, Alcañiz, M, and Noé, E. Self-awareness rehabilitation through a multi-touch virtual game board after acquired brain injury. Stud Health Technol Inform. (2012) 181:297–301. doi: 10.3233/978-1-61499-121-2-297
49. Jahromi, MK, and Mosallanejad, L. The impact of reality therapy on metacognition, stress and hope in addicts. Global J Health Sci. (2014) 6:281–7. doi: 10.5539/gjhs.v6n6p281
50. Schmidt, J, Lannin, N, Fleming, J, and Ownsworth, T. Feedback interventions for impaired self-awareness following brain injury: a systematic review. J Rehabil Med. (2011) 43:673–80. doi: 10.2340/16501977-0846
51. De Jong, S, Van Donkersgoed, RJ, Timmerman, ME, Aan Het Rot, M, Wunderink, L, Arends, J, et al. Metacognitive reflection and insight therapy (MERIT) for patients with schizophrenia. Psychol Med. (2019) 49:303–13. doi: 10.1017/S0033291718000855
52. Wenn, JA, O’Connor, M, Kane, RT, Rees, CS, and Breen, LJ. A pilot randomised controlled trial of metacognitive therapy for prolonged grief. BMJ Open. (2019) 9:e021409. doi: 10.1136/bmjopen-2017-021409
53. Tamm, L, and Nakonezny, PA. Metacognitive executive function training for young children with ADHD: a proof-of-concept study. Atten Defic Hyperact Disord. (2015) 7:183–90. doi: 10.1007/s12402-014-0162-x
54. Shany-Ur, T, Lin, N, Rosen, HJ, Sollberger, M, Miller, BL, and Rankin, KP. Self-awareness in neurodegenerative disease relies on neural structures mediating reward-driven attention. Brain. (2014) 137:2368–81. doi: 10.1093/brain/awu161
55. Filevich, E, Forlim, CG, Fehrman, C, Forster, C, Paulus, M, Shing, YL, et al. I know that I know nothing: cortical thickness and functional connectivity underlying meta-ignorance ability in pre-schoolers. Dev Cogn Neurosci. (2020) 41:100738. doi: 10.1016/j.dcn.2019.100738
56. Hallam, B, Chan, J, Gonzalez Costafreda, S, Bhome, R, and Huntley, J. What are the neural correlates of meta-cognition and Anosognosia in alzheimer’s disease? A systematic review. Neurobiol Aging. (2020) 94:250–64. doi: 10.1016/j.neurobiolaging.2020.06.011
57. Palermo, S, Leotta, D, Bongioanni, MR, and Amanzio, M. Unawareness of deficits in ischemic injury: role of the cingulate cortex. Neurocase. (2013) 20:540–55. doi: 10.1080/13554794.2013.826686
58. Sherer, M, Hart, T, Whyte, J, Nick, TG, and Yablon, SA. Neuroanatomic basis of impaired self-awareness after traumatic brain injury. J Head Trauma Rehabil. (2005) 20:287–300. doi: 10.1097/00001199-200507000-00002
59. Ownsworth, TL, Turpin, M, Andrew, B, and Fleming, J. Participant perspectives on an individualised self-awareness intervention following stroke: a qualitative case study. Neuropsychol Rehabil. (2008) 18:692–712. doi: 10.1080/09602010701595136
60. Bartoli, M, Palermo, S, Stanziano, M, Cipriani, GE, Leotta, D, Valentini, MC, et al. Reduced self-awareness following a combined polar and paramedian bilateral thalamic infarction. A possible relationship with SARS-COV-2 risk of contagion? Front Psychol. (2020) 11:570160. doi: 10.3389/fpsyg.2020.570160
61. Tang, WK, Chen, YK, Lu, JY, Mok, VC, Xiang, YT, Ungvari, GS, et al. Microbleeds and post-stroke emotional lability. J Neurol Neurosurg Psychiatry. (2009) 80:1082–6. doi: 10.1136/jnnp.2009.175372
62. van der Stelt, CM, Fama, ME, Mccall, JD, Snider, SF, and Turkeltaub, PE. Intellectual awareness of naming abilities in people with chronic post-stroke aphasia. Neuropsychologia. (2021) 160:107961. doi: 10.1016/j.neuropsychologia.2021.107961
63. Tang, WK, Chan, SS, Chiu, HF, Ungvari, GS, Wong, KS, Kwok, TC, et al. Poststroke depression in Chinese patients: frequency, psychosocial, clinical, and radiological determinants. J Geriatr Psychiatry Neurol. (2005) 18:45–51. doi: 10.1177/0891988704271764
64. Li, H, Jia, J, and Yang, Z. Mini-mental state examination in elderly Chinese: a population-based normative study. J Alzheimers Dis. (2016) 53:487–96. doi: 10.3233/jad-160119
65. Brott, T, Adams, HP, Olinger, CP, Marler, JR, Barsan, WG, Biller, J, et al. Measurements of acute cerebral infarction: a clinical examination scale. Stroke. (1989) 20:864–70. doi: 10.1161/01.str.20.7.864
66. Tse, YE. Validity of functional independence measure (Hong Kong version) [dissertation]. [Hong Kong]: Hong Kong Polytechnic University (1999).
67. Tong, AYC, and Man, DWK. The validation of the Hong Kong Chinese version of the Lawton instrumental activities of daily living scale for institutionalized elderly persons. OTJR Occupat Participat Health. (2002) 22:132–42. doi: 10.1177/153944920202200402
68. Mahoney, FI, and Barthel, DW. Functional evaluation: the Barthel index. Md State Med J. (1965) 14:61–5.
69. Fleming, J, Strong, J, and Ashton, R. Self-awareness of deficits in adults with traumatic brain injury: how best to measure? Brain Inj. (1996) 10:1–16. doi: 10.1080/026990596124674
70. Smeets, SM, Ponds, RW, Verhey, FR, and van Heugten, CM. Psychometric properties and feasibility of instruments used to assess awareness of deficits after acquired brain injury. J Head Trauma Rehabil. (2012) 27:433–42. doi: 10.1097/htr.0b013e3182242f98
71. Llorens, R, Noé, E, Ferri, J, and Alcañiz, M. Videogame-based group therapy to improve self-awareness and social skills after traumatic brain injury. J Neuroeng Rehabil. (2015) 12:37. doi: 10.1186/s12984-015-0029-1
72. Bogod, NM, Mateer, CA, and Macdonald, SWS. Self-awareness after traumatic brain injury: a comparison of measures and their relationship to executive functions. J Int Neuropsychol Soc. (2003) 9:450–8. doi: 10.1017/s1355617703930104
73. Simmond, M, and Fleming, J. Reliability of the self-awareness of deficits interview for adults with traumatic brain injury. Brain Inj. (2003) 17:325–37. doi: 10.1080/0269905021000013219
74. Wilson, BA, Alderman, N, Burguess, P, and Emslie, J. Manual of the behavioural assessment of the dysexecutive syndrome. Bury St Edmunds, UK: Thames Valley Test Company (1996).
75. Leathem, JM, Murphy, LJ, and Flett, RA. Self- and informant-ratings on the patient competency rating scale in patients with traumatic brain injury. J Clin Exp Neuropsychol. (1998) 20:694–705. doi: 10.1076/jcen.20.5.694.1122
76. Sherer, M, Bergloff, P, Boake, C, High, W Jr, and Levin, E. The awareness questionnaire: factor structure and internal consistency. Brain Inj. (1998) 12:63–8. doi: 10.1080/026990598122863
77. Hu, F, Liu, C, Cao, S, Wang, X, Liu, W, Li, T, et al. Cross-cultural adaptation and validation of the simplified Chinese version of the Fremantle Back awareness questionnaire in patients with low back pain. Eur Spine J. (2022) 31:935–42. doi: 10.1007/s00586-021-07085-8
78. Wei, X, Ma, Y, Wu, T, Yang, Y, Yuan, Y, Qin, J, et al. Which cutoff value of the Montreal cognitive assessment should be used for post-stroke cognitive impairment? A systematic review and meta-analysis on diagnostic test accuracy. Int J Stroke. (2023) 18:908–16. doi: 10.1177/17474930231178660
79. Yeung, P, Wong, L, Chan, C, Leung, JL, and Yung, C. A validation study of the Hong Kong version of Montreal cognitive assessment (HK-MOCA) in Chinese older adults in Hong Kong. Hong Kong Med J. (2014) 20:504–10. doi: 10.12809/hkmj144219
80. Geva, S, Truneh, T, Seghier, ML, Hope, TM, Leff, AP, Crinion, JT, et al. Lesions that do or do not impair digit span: a study of 816 stroke survivors. Brain Commun. (2021) 3:31. doi: 10.1093/braincomms/fcab031
81. Wechsler, D. Manual for the Wechsler adult intelligence scale-III Psychological Corporation San Antonio, TX, USA (1997).
82. Gong, X, Wong, PC, Fung, HH, Mok, VC, Kwok, TC, Woo, J, et al. The Hong Kong grocery shopping dialog task (HK-GSDT): a quick screening test for neurocognitive disorders. Int J Environ Res Public Health. (2022) 19:13302. doi: 10.3390/ijerph192013302
83. Wong, GK, Nung, RC, Sitt, JC, Mok, VC, Wong, A, Poon, WS, et al. Development of a delayed cerebral infarction load scoring system (DCI score). Acta Neurochir Suppl. (2020) 127:145–8. doi: 10.1007/978-3-030-04615-6_21
84. Chan, A, and Kwok, I. The Hong Kong list learning test: manual and preliminary norm. Hong Kong, People’s republic of China: Department of Psychology, Chinese University of Hong Kong (1999).
85. Ko, MH, Yoon, JY, Jo, YJ, Son, MN, Kim, DS, Kim, GW, et al. Home-based transcranial direct current stimulation to enhance cognition in stroke: randomized controlled trial. Stroke. (2022) 53:2992–3001. doi: 10.1161/STROKEAHA.121.037629
86. Colombo, M, Aggujaro, S, Lombardi, N, Pedrocchi, A, Molteni, F, and Guanziroli, E. Motor and cognitive modulation of a single session of transcutaneous auricular vagus nerve stimulation in post stroke patients: a pilot study. IEEE Open J Eng Med Biol. (2023) 4:292–9. doi: 10.1109/OJEMB.2023.3268011
87. Rubia, K, Russell, T, Overmeyer, S, Brammer, MJ, Bullmore, ET, Sharma, T, et al. Mapping motor inhibition: conjunctive brain activations across different versions of go/no-go and stop tasks. NeuroImage. (2001) 13:250–61. doi: 10.1006/nimg.2000.0685
88. Chiu, EC, Wu, WC, Hung, JW, and Tseng, YH. Validity of the Wisconsin card sorting test in patients with stroke. Disabil Rehabil. (2018) 40:1967–71. doi: 10.1080/09638288.2017.1323020
89. Jankowska, A, Hadław-Klimaszewska, O, Rakoczy, J, Woldańska-Okońska, M, and Irzmański, R. The impact of rehabilitation on the recovery of executive functions in stroke patients with different location of the ischemic focus. Int J Occup Med Environ Health. (2024) 37:452–67. doi: 10.13075/ijomeh.1896.02129
90. Heaton, RK, Chelune, GJ, Talley, JL, Kay, GG, and Curtiss, G. Wisconsin card sorting test manual. Revised and expanded. Lutz, U.S.A: Psychological Assessment Resources, Inc. (1993).
91. Shek, DT. Reliability and factorial structure of the Chinese version of the Beck depression inventory. J Clin Psychol. (1990) 46:35–43. doi: 10.1002/1097-4679(199001)46:1<35::AID-JCLP2270460106>3.0.CO;2-W
92. Leung, C, Ho, S, Kan, CS, Hung, C, and Chen, C. Hospital anxiety and depression scale--Chinese version. Int J Psychosom. (1993) 40:29–34. doi: 10.1037/t05677-000
93. Sled, JG, and Pike, GB. Understanding intensity non-uniformity in MRI In: Medical image computing and computer-assisted intervention — MICCAI’98 MICCAI 1998 lecture notes in computer science. Heidelberg, Berlin: Springer (1998). 1–15.
94. Kannala, J, Rahtu, E, and Heikkila, J. Affine registration with multi-scale autoconvolution, IEEE. Genova, Italy: International Conference on Image Processing (2005).
95. Smith, SM. Fast robust automated brain extraction. Hum Brain Mapp. (2002) 17:143–55. doi: 10.1002/hbm.10062
96. Cocosco, CA, Zijdenbos, AP, and Evans, AC. A fully automatic and robust brain MRI tissue classification method. Med Image Anal. (2003) 7:513–27. doi: 10.1016/s1361-8415(03)00037-9
97. Han, X, and Fischl, B. Atlas renormalization for improved brain Mr image segmentation across scanner platforms. IEEE Trans Med Imaging. (2007) 26:479–86. doi: 10.1109/tmi.2007.893282
98. Lancaster, JL, Tordesillas-Gutiérrez, D, Martinez, M, Salinas, F, Evans, A, Zilles, K, et al. Bias between MNI and Talairach coordinates analyzed using the ICBM-152 brain template. Hum Brain Mapp. (2007) 28:1194–205. doi: 10.1002/hbm.20345
99. Cohen, J. Statistical power analysis for the behavioral sciences. 2nd ed. Hillsdale, NJ: L. Erlbaum Associates (1988).
101. Tang, WK, Chen, Y, Lam, WW, Mok, V, Wong, A, Ungvari, GS, et al. Emotional incontinence and executive function in ischemic stroke: a case-controlled study. J Int Neuropsychol Soc. (2009) 15:62–8. doi: 10.1017/S1355617708090061
102. Valiengo, LC, Goulart, AC, de Oliveira, JF, Benseñor, IM, Lotufo, PA, and Brunoni, AR. Transcranial direct current stimulation for the treatment of post-stroke depression: results from a randomised, sham-controlled, double-blinded trial. J Neurol Neurosurg Psychiatry. (2017) 88:170–5. doi: 10.1136/jnnp-2016-314075
103. Frey, J, Najib, U, Lilly, C, and Adcock, A. Novel TMS for stroke and depression (NoTSAD): accelerated repetitive transcranial magnetic stimulation as a safe and effective treatment for post-stroke depression. Front Neurol. (2020) 11:788. doi: 10.3389/fneur.2020.00788
104. Maier, M, Ballester, BR, Leiva Bañuelos, N, Duarte Oller, E, and Verschure, PF. Adaptive conjunctive cognitive training (ACCT) in virtual reality for chronic stroke patients: a randomized controlled pilot trial. J Neuroeng Rehabil. (2020) 17:42. doi: 10.1186/s12984-020-0652-3
Keywords: stroke, metacognition, executive function, MRI, prefrontal cortex, anterior insula, amygdala, thalamus
Citation: Tang WK, Hui E and Leung TWH (2025) Metacognition impairment in stroke. Front. Neurol. 16:1501419. doi: 10.3389/fneur.2025.1501419
Received: 25 September 2024; Accepted: 27 February 2025;
Published: 11 March 2025.
Edited by:
Ana Catarina Fonseca, University of Lisbon, PortugalReviewed by:
Pasquale Scognamiglio, ASL Napoli 3 Sud, ItalyCopyright © 2025 Tang, Hui and Leung. This is an open-access article distributed under the terms of the Creative Commons Attribution License (CC BY). The use, distribution or reproduction in other forums is permitted, provided the original author(s) and the copyright owner(s) are credited and that the original publication in this journal is cited, in accordance with accepted academic practice. No use, distribution or reproduction is permitted which does not comply with these terms.
*Correspondence: Wai Kwong Tang, dGFuZ3drQGN1aGsuZWR1Lmhr
Disclaimer: All claims expressed in this article are solely those of the authors and do not necessarily represent those of their affiliated organizations, or those of the publisher, the editors and the reviewers. Any product that may be evaluated in this article or claim that may be made by its manufacturer is not guaranteed or endorsed by the publisher.
Research integrity at Frontiers
Learn more about the work of our research integrity team to safeguard the quality of each article we publish.