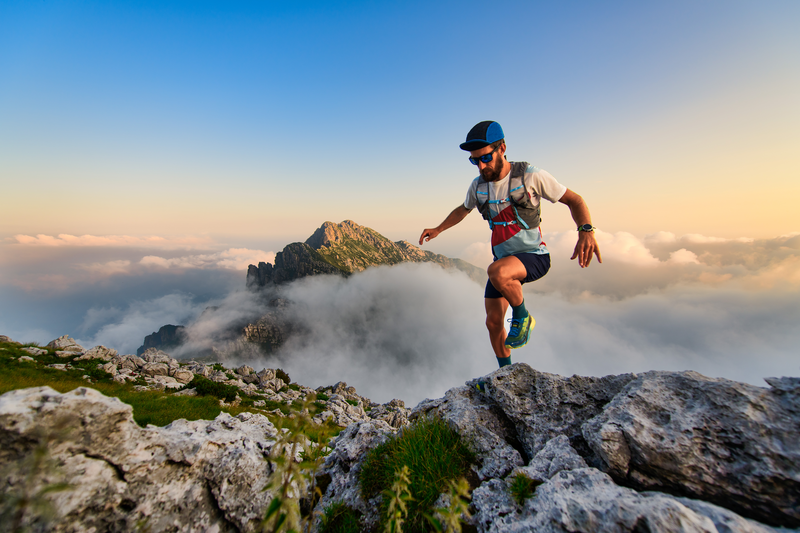
94% of researchers rate our articles as excellent or good
Learn more about the work of our research integrity team to safeguard the quality of each article we publish.
Find out more
REVIEW article
Front. Neurol.
Sec. Dementia and Neurodegenerative Diseases
Volume 16 - 2025 | doi: 10.3389/fneur.2025.1496382
The final, formatted version of the article will be published soon.
You have multiple emails registered with Frontiers:
Please enter your email address:
If you already have an account, please login
You don't have a Frontiers account ? You can register here
Objectives: This meta-analysis aims to systematically evaluate the effects of virtual reality (VR)-based interventions on cognitive function, emotional state, and quality of life in patients with mild cognitive impairment (MCI).Methods: A comprehensive literature search was conducted using five databases from their inception to June 2024. The inclusion criteria focused on randomized controlled trials (RCTs) that examined VR-based interventions in adults aged 60 or older diagnosed with MCI. The primary outcome was cognitive function, while secondary outcomes included emotional state, quality of life, and dynamic balance. To investigate potential sources of heterogeneity, subgroup analyses and meta-regression were conducted. Publication bias was assessed using funnel plots and Egger’s regression test. A “trim and fill” method was employed to adjust for any detected publication bias. The certainty of the evidence was evaluated using the GRADE framework with the GRADEpro GDT software.Results: A total of 30 RCTs involving 1,365 participants from 9 countries across 4 continents were included. The meta-analysis revealed that VR-based interventions significantly improved global cognition, as assessed by the Montreal Cognitive Assessment (MoCA; SMD = 0.82, 95% CI: 0.27 to 1.38, P = 0.003, GRADE: moderate) and the Mini-Mental State Examination (MMSE; SMD = 0.83, 95% CI: 0.40 to 1.26, P = 0.0001, GRADE: low). Additionally, VR interventions enhanced attention, as measured by the Digit Span Backward (DSB; SMD = 0.61, 95% CI: 0.21 to 1.02, P = 0.003, GRADE: low) and Digit Span Forward (DSF; SMD = 0.89, 95% CI: 0.34 to 1.45, P = 0.002, GRADE: low). Improvements were also observed in quality of life, as indicated by scores on the Instrumental Activities of Daily Living (IADL; SMD = 0.22, 95% CI: 0.00 to 0.45, P = 0.049, GRADE: moderate). Conclusions: The findings indicate that VR interventions can significantly improve global cognition, attention, and quality of life in individuals with MCI. Subgroup analyses further revealed that optimal cognitive outcomes were associated with semi-immersive VR, session durations of ≤ 60 minutes, intervention frequencies exceeding twice per week, studies conducted in Asia and Europe, and participant groups with a male proportion of ≤ 40%.
Keywords: virtual reality, Mild Cognitive Impairment, Cognitive Function, emotional state, Quality of Life
Received: 14 Sep 2024; Accepted: 10 Mar 2025.
Copyright: © 2025 Li, Zhang, Tang, Ye and Tang. This is an open-access article distributed under the terms of the Creative Commons Attribution License (CC BY). The use, distribution or reproduction in other forums is permitted, provided the original author(s) or licensor are credited and that the original publication in this journal is cited, in accordance with accepted academic practice. No use, distribution or reproduction is permitted which does not comply with these terms.
* Correspondence:
Min Tang, Ningbo Rehabilitation Hospital, Ningbo, China
Disclaimer: All claims expressed in this article are solely those of the authors and do not necessarily represent those of their affiliated organizations, or those of the publisher, the editors and the reviewers. Any product that may be evaluated in this article or claim that may be made by its manufacturer is not guaranteed or endorsed by the publisher.
Research integrity at Frontiers
Learn more about the work of our research integrity team to safeguard the quality of each article we publish.