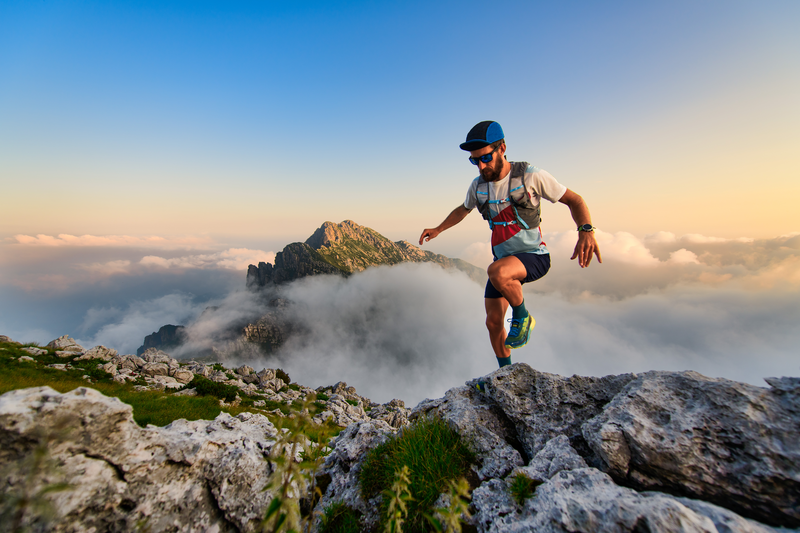
94% of researchers rate our articles as excellent or good
Learn more about the work of our research integrity team to safeguard the quality of each article we publish.
Find out more
REVIEW article
Front. Neurol.
Sec. Endovascular and Interventional Neurology
Volume 16 - 2025 | doi: 10.3389/fneur.2025.1479229
The final, formatted version of the article will be published soon.
You have multiple emails registered with Frontiers:
Please enter your email address:
If you already have an account, please login
You don't have a Frontiers account ? You can register here
Carotid-ophthalmic aneurysms (COA) are complex and severe intracranial arterial lesions, and their treatment and management have always been a focus of clinical research. In recent years, the introduction of flow diverters (FD) has provided a revolutionary method for the treatment of intracranial aneurysms (IA). Although FD has achieved significant success in reducing the risk of COA rupture, the complex anatomical structure and critical function of the ophthalmic artery (OphA) mean that covering the OphA with FD may lead to adverse ophthalmic outcomes. This review aims to systematically examine the ocular complications and their mechanisms when FD covers the OphA in the treatment of COA, emphasizing the potential risks that clinicians should be aware of when applying FD treatment, to reduce complications and improve the overall prognosis of patients.
Keywords: Flow-diverter, Carotid-ophthalmic aneurysms, Ophthalmic Artery, Neuro-ophthalmic complications, Systematic review
Received: 11 Aug 2024; Accepted: 06 Mar 2025.
Copyright: © 2025 Ma, He, Lin, Pan and Zhang. This is an open-access article distributed under the terms of the Creative Commons Attribution License (CC BY). The use, distribution or reproduction in other forums is permitted, provided the original author(s) or licensor are credited and that the original publication in this journal is cited, in accordance with accepted academic practice. No use, distribution or reproduction is permitted which does not comply with these terms.
* Correspondence:
Changwei Zhang, Department of Neurosurgery, West China Hospital, Sichuan University, Chengdu, China
Disclaimer: All claims expressed in this article are solely those of the authors and do not necessarily represent those of their affiliated organizations, or those of the publisher, the editors and the reviewers. Any product that may be evaluated in this article or claim that may be made by its manufacturer is not guaranteed or endorsed by the publisher.
Research integrity at Frontiers
Learn more about the work of our research integrity team to safeguard the quality of each article we publish.