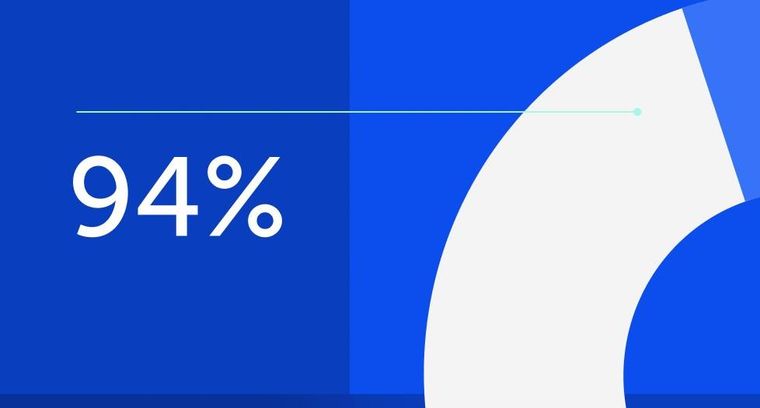
94% of researchers rate our articles as excellent or good
Learn more about the work of our research integrity team to safeguard the quality of each article we publish.
Find out more
ORIGINAL RESEARCH article
Front. Neurol., 18 March 2025
Sec. Pediatric Neurology
Volume 16 - 2025 | https://doi.org/10.3389/fneur.2025.1451346
Background: Early diagnosis and initiation of hypothermia therapy for neonatal hypoxic–ischemic encephalopathy (HIE) are critical within the first 6 h after birth. Collecting urine, especially from neonates with HIE, can be challenging and time-consuming post-birth due to the likelihood of renal injury.
Purpose: We assessed whether urine protein levels, measured via a simple urinalysis on the first day, could correlate with the outcomes of neonatal HIE.
Methods: We conducted urine analyses of patients with neonatal HIE on the first day to establish a correlation between the severity of HIE and neurodevelopmental outcomes at ≥1 year of age. Eighty-three patients were enrolled, encompassing cases of mild (n = 37), moderate (n = 30), and severe (n = 16) HIE. Three cases were excluded due to mortality caused by severe HIE with associated auria. Based on urine protein levels, patients were grouped as 0 to 30 mg/dL (group 1), 30 to 100 mg/dL (group 2), 100 to 300 mg/dL (group 3), and ≥ 300 mg (group 4).
Results: Urine protein levels were correlated with serum lactic acid levels [p = 0.006; r (81) = 0.304; n = 83], clinical staging [p = 0.001; r (81) = 0.36], and neurodevelopmental outcomes at ≥1 year of age [X2 (3, n = 83) = 11.35; p = 0.009]. The odds ratio for moderate-to-severe HIE in group 4 patients was 7.66 [p = 0.010; 95% confidence interval (CI), 1.61–36.33] compared with those in groups 1–3. Those in group 4 had a high positive predictive value (87.50%) and high specificity (94.59%).
Conclusion: Elevated urine protein levels observed in the first urinalysis conducted on the day after birth were found to be associated with serum lactic acid levels, clinical staging, and neurodevelopmental outcomes at ≥1 year of age.
Neonatal hypoxic–ischemic encephalopathy (HIE) is a physiological disorder that is caused by a prolonged or profound mismatch between oxygen demand and oxygen delivery in newborn infants (1). Neonatal HIE is a major cause of neonatal mortality, accounting for about 24% of neonatal deaths (2–4). Neonatal HIE can range from mild to severe. When a neonate has moderate to severe HIE, irreversible cerebral cell damage and death can occur, leading to a syndrome of HIE. HIE can affect multiple organs, including the brain, heart, liver, hemopoietic system, and kidney, and may lead to an altered conscious state, autonomic instability, seizures, decreased cardiac output, liver and renal dysfunction, and even death. HIE is thus an important and major cause of neonatal death and neurodevelopmental consequences (5, 6).
Rescue hypothermia is a treatment for neonatal HIE that has been shown to be effective and associated with few adverse effects in newborns (7–9). While therapeutic hypothermia is used clinically to reduce neurological injury secondary to HIE, it is associated with a 45–55% risk of moderate–severe disability or death (7, 8, 10). Therapeutic hypothermia can be performed as a standard of care for moderate-to-severe HIE; however, in newborns with mild HIE, the benefits have not been confirmed and potential consequences include coagulation disorders and cardiopulmonary instability (11, 12). Therapeutic hypothermia has also been shown to improve the function of other organs, such as the kidneys. The presence of shock, low blood pressure and cardiac output, or hypoxic injury to the kidney may indicate renal injury secondary to neonatal HIE, which may further aggravate the morbidity and mortality associated with HIE.
Neonatal HIE can cause multiple organ injury to the brain, heart, liver, and kidneys, among others. While about 34% of cases of HIE are only result in mild organ injury not evident by clinical observation, 23% of cases result in injury to one organ, 34% to two organs, and 9% to three organs (13, 14). Organ involvement may include the central nervous system (28%), cardiovascular system (25%), lungs (23%), and kidneys (50%) (15, 16). Cerebral palsy resulting from neonatal HIE may also lead to multiple organ dysfunction (17). Renal biomarkers of neonatal HIE, including low molecular weight proteins, such as b2-microglobulin, myoglobin, retinol binding protein, and N-acetyl-b-D-glucosaminidase (NAG), have been widely studied and used to detect renal tubular dysfunction (15, 18). Of these, b2-microglobulin and NAG have shown promise. However, the clinical application of these biomarkers is not widely available. A biomarker that can be measured by a simple urine analysis would therefore be a valuable tool for assessing neonatal HIE severity.
Common serum biomarkers include lactate (19, 20), bilirubin, liver function tests, lactate dehydrogenase (LDH), troponin-T, creatine phosphokinase (CK), urine ratio of lactate/creatinine (L/C), interleukin (IL)-10 (11, 21), and plasma osteopontin (OPN) and glial fibrillary astrocytic protein (GFAP) (22). Serum IL-6 levels and ratio of L/C ratio may predict the disability and mortality in neonatal HIE (23). The acylcarnitine profile, which demonstrates the value of butyrylcarnitine as a prognostic marker, has been used in neonatal HIE (24). Studies have exhibited that biomarkers such as brain-specific proteins (neuron-specific enolase [NSE], S100B, ubiquitin carboxy-terminal hydrolase-L1, and total Tau) and cytokines (tumor necrosis factor alpha, interferon-gamma, IL-6, IL-1β, IL-10, IL-13, and brain-derived neurotrophic factor) are helpful for diagnosing HIE and predicting neurodevelopmental outcomes (25–28).
The kidney is fundamentally associated with the injuries caused by neonatal HIE. Damage to the kidney causes renal injury, which can lead to oliguria or anuria, renal proteinuria, and hematuria, which could be useful for identifying perinatal asphyxia. Early elevation of microproteins in the urine may associated with the severity of neonatal HIE and help predict the outcomes of encephalopathy in newborns being discharged (15, 18). In asphyxiated neonates, early protein elevation in the urine may be a marker for predicting neonatal HIE; however, its relation to short-term and long-term outcomes has not been adequately studied. Additionally, while the urine L/C ratio might be a useful biomarker for differentiating newborns with moderate-to-severe HIE from those with mild HIE, however, urine creatinine analysis requires a large amount of urine. Using a simple urine analysis to predict the severity of neonatal HIE would therefore be a valuable tool.
Early diagnosis and initiation of hypothermia therapy for neonatal hypoxic–ischemic encephalopathy (HIE) are critical within the first 6 h after birth. Collecting urine, especially from neonates with HIE, can be challenging and time-consuming post-birth due to the likelihood of renal injury. We assessed whether urine protein levels, measured via a simple urinalysis on the first day, could correlate with the outcomes of neonatal HIE.
Data on patients born between 2015 and 2021 at the Chung Shan Medical University Hospital (CSMU) with neonatal HIE were collected and reviewed, including the following: history of placental abruption, cord prolapse or fetal distress (a heart rate < 100 bpm before birth), metabolic acidosis (arterial pH of <7.20 or base deficit of ≥10 mmol per liter), or dependent positive-pressure ventilation immediately beyond the tenth minute of life after birth. Neonatal HIE was classified according to the clinical Sarnat staging as follows: stage I (mild), stage II (moderate), and stage III (severe) (7, 8, 29). The CSMU is a medical center in the middle of Taiwan. The initial urine sample was collected on the first day after admission for analysis. Serum lactate, LDH, and troponin levels were analyzed as biomarkers in the first 6 h of life. For patients with stage I–III HIE, the series examination included a head ultrasound (HUS), magnetic resonance imaging (MRI), and electroencephalogram (EEG) monitoring, if available. To classify Sarnat staging before 6 h after birth, an experienced pediatric neurologist and neonatologist were consulted after admission to classify them into Sarnat stage I (mild), II (moderate), and III (severe) HIE (1).
The urine analysis findings included the color, appearance, specific gravity, pH, protein, urine glucose, ketones, urine red blood cell (RBC), white blood cell (WBC), leukocyte esterase, nitrite, bilirubin (30), and urobilinogen. The urine samples were analyzed with either the Sysmex UC-3500 or UF-5000 (Sysmex cooperation, Kobe, Japan), was dropped onto each pad of a dedicated test strip within the UC-3500 analyzer. The entire sequence, from sample aspiration, to color comparison, and the final output of the results, is fully automatic. The test paper MEDITAPE UC-9A (Sysmex cooperation, Kobe, Japan) was used. UF-5000 fluorescence flow cytometry technology and hydrodynamic focusing was also applied for urine sediment analysis. Each patient’s first urine sample on the first day was sent for urine analysis and the data, including urine RBC count per high power field (HPF), WBC count per HPF, and urine protein level were analyzed. The patients were classified according to urine protein level into group 1 [zero or trace levels (0 to 30 mg/dL)], group 2 [“+” (30 to 100 mg/dL)], group 3 [“++” (100 to 300 mg/dL)], and group 4 [“+++” or more (> 300 mg/dL)].
To assess the neurological outcomes of neonatal HIE, MRIs, hearing assessments, a history of seizures before the age of 1 year, and neurodevelopmental examinations of patients aged ≥1 year were evaluated. An unremarkable MRI indicated that there were no visible lesions in the brain parenchymal region; a mild abnormal MRI indicated brain lesions in the parenchyma but not the basal ganglion, thalamus, midbrain, or brainstem; and a severe abnormal MRI indicated brain lesions as diffuse white matter involvements or brain lesions in one of basal ganglia, thalamus, and brain stem with or without involvement of other brain regions. All the newborns’ hearing was assessed using the newborn hearing screening for automatic auditory brainstem evoked response (aABR). For those patients who failed the aABR twice, ABR, otoacoustic emission (OAE), and steady state evoked potential (SSEP) tests were performed (31). The degree of hearing loss was classified as normal (≤ 35 dB nHL) or abnormal (> 35 dB nHL) (32, 33). Patient outcomes of neurodevelopment were evaluated at the corrected age of 1 year either through clinical evaluation or the Bayley Scales of Infant and Toddler Development, Third Edition (Bayley-III). Patient charts were retrospectively reviewed in all cases.
Significant differences were using the independent t-test to compare the means of two independent groups or the chi-squared test to compare the categorical variables. The Pearson correlation coefficient was provided to measure the linear association between two variables and the value r = 1 indicates a perfect positive correlation; the value r = −1 means a perfect negative correlation. In the case of a nonparametric sample distribution, a Mann–Whitney U test was performed. The Spearman’s Rho (rs) calculator was used to measure the strength of an association between two variables in the non-parametric test. The one-way analysis of variance (ANOVA) test for independent variables was to compare the means of 3 or more independent samples. Significance was set at p < 0.05.
A total of 103 patients with HIE were enrolled. After excluding those with congenital anomalies (n = 7), premature birth (< 36 weeks; n = 9), and confirmed genetic defects (n = 1) (Figure 1), 86 cases were included. Three cases experienced post-birth mortality due to severe HIE and auria. Of the 83 cases, 37 had stage I (mild), 30 had stage II (moderate), and 16 had stage III (severe) HIE. The birth weight, gender, age, and method of delivery were not significantly different between the four groups according to urine protein level (Table 1). The time of first urine collection was 7.3 ± 3.5 h (range; 2–16 h) after birth in stage 1; 8.1 ± 3.7 h (range; 3–19 h) after birth in stage 2; and 8.7 ± 3.4 h (range; 3–13 h) after birth in stage 3. The timing of the initial urine collections were extended in advanced staging, but no significant differences were observed.
Figure 1. Flow chart of patient selection. MRI, magnetic resonance imaging. Bayley-III, Bayley Scales of Infant and Toddler development, third edition.
According to urine protein level, patients were divided into group 1 [zero or trace levels (0–30 mg/dL)], group 2 [“+” (30 to 100 mg/dL)], group 3 [“++” (100 to 300 mg/ dL)], and group 4 [“+++” or more (> 300 mg/ dL)]. Using one-way ANOVA, the urine protein level was significantly correlated with the staging (f-ratio 7.27, p = 0.007) (Table 2). Using linear regression analysis, ŷ (urine protein) = 0.26 (staging) + 1.40 (r = 0.36 according to the Pearson Correlation Coefficient Calculator) (p = 0.001, n = 83).
Table 2. Correlation between urine protein level and clinical staging, hearing impairment, history of seizures before 1 year of age, and neurodevelopmental outcomes at ≥1 year of age (n = 83).
Table 2 shows the correlation between the HIE stage and urine protein levels. The urine protein classification among the four groups were significantly different [X2 (3, n = 83) = 9.96; p = 0.019]. Of the 48 neonates in group 1 and 2 (urine protein <100 mg/dL), 24 had stage I HIE (50%) and 24 (50%) had stage II or III; whereas of the 35 neonates in groups 3 and 4 (urine protein >100 mg/dL), 13 had the stage I (37.1%) and 22 (62.9%) had stage II or III HIE. Of the 16 neonates in group 4, only two (12.5%) had stage 1 HIE. The odds ratio of stage II or III requiring rescue hypothermia therapy for those with urine protein levels >300 mg/dLwas 7.66 (p = 0.010; 95% confidence interval (CI), 1.61–36.33) compared with those patients with urine protein levels <300 mg/dL. Receiver operating characteristic (ROC) curve of urine protein and cut-off value to predict HIE staging. ROC plots of urine protein and the area under the curve (AUC) for HIE (AUC 0.676, p = 0.004, 95% CI = 0.568–0.785). The cut-off value for maximizing AUC of urine protein was “+++” or more [> 300 mg/dL] (Figure 2).
Figure 2. Receiver operating characteristic (ROC) curve of urine protein and cut-off value to predict HIE staging. ROC plots of urine protein and the area under the curve (AUC) for HIE (AUC 0.676, p = 0.004, 95% CI = 0.568–0.785). The cut-off value for maximizing AUC of urine protein was “+++” or more [> 300 mg/dL].
A urine protein level ≥ 300 mg/dL had a high positive predictive value (87.50%; 95% CI, 0.63–0.97) and a high specificity (94.59%; 95% CI, 0.81–0.99) for stage II or stage III HIE requiring emergent hypothermia therapy, but had a low negative predictive value (52.24%; 95% CI, 0.47 to 0.57) and a low sensitivity (30.43%; 95% CI, 0.18–0.46) (Table 2).
Urine protein levels were significantly correlated with the first serum lactic acid level after admission according to linear regression analysis (p = 0.006; r(81) = 0.304; n = 83). However, urine protein levels were not significantly correlated with the first LDH level taken after admission according to the linear regression analysis [p = 0.198; r(81) = 0.304; n = 83].
Available urine protein levels was associated with the first serum creatinine; 0.84 ± 0.21 mg/dL in group 1, 0.94 ± 0.28 mg/dL in group 2, 0.97 ± 0.19 mg/dL in group 3, and 1.05 ± 0.21 mg/dL in group 4. The creatinine level increased with higher urine protein, and was significantly different in group 1 versus group 4 (p = 0.022).
Urine protein levels were significantly correlated with clinical staging [X2 (3, n = 83) = 9.96; p = 0.019] (Table 2 and Figure 3) and patient outcomes of neurodevelopment at ≥1 year of age [X2 (3, n = 83) = 11.35; p = 0.009] for all HIE stages, but not with the hearing outcomes or a history of seizures before 1 year of age (Table 2 and Figure 3). For those who underwent therapeutic hypothermia (n = 46), urine protein levels were significantly correlated with clinical staging [X2 (1, n = 46) =10.98; p < 0.001] (Table 3 and Figure 3) and patient outcomes of neurodevelopment at ≥1 year of age [X2 (1, n = 46) = 6.38; p = 0.012] (Table 3) but not with hearing outcomes, MRI finings, or a history of seizures before 1 year of age (Table 3 and Figure 3).
Figure 3. The urine protein level correlated with the clinical staging and neurodevelopmental outcomes ≥1 year old. (A) Patients were divided into groups according to urine protein level were significantly correlated with clinical staging [X2 (3, n = 83) = 9.96; p = 0.019]; (B) Urine protein levels were significantly correlated with neurodevelopmental outcomes at ≥1 year of age [X2 (3, n = 83) = 11.35; p = 0.009] for all HIE stages; (C) For those who underwent therapeutic hypothermia (clinical staging II and III, n = 46), more urine protein levels were significantly correlated with advanced clinical staging [X2 (1, n = 46) =10.98; p < 0.001] and (D) neurodevelopmental outcomes at ≥1 year of age [X2 (1, n = 46) = 6.38; p = 0.012].
Table 3. Correlation between urine protein level and clinical staging, MRI findings, hearing impairment, history of seizures before 1 year of age, and neurodevelopmental outcomes at ≥1 year of age in patients who underwent hypothermia therapy (n = 46).
According to urine RBC count, patients were classified into group A (urine RBCs/ HPF ≤ 5), group B (urine RBCs/ HPF > 5 and ≤ 10), and group C (urine RBCs/ HPF > 10). According to the linear regression analysis, none of the groups were significantly correlated with clinical staging (p = 0.728), LDH levels (p = 0.121), or lactic acid levels (p = 0.198). Thus, the urine RBC count was not correlated with clinical staging or blood biomarkers associated with neonatal HIE.
According to urine WBC/ HPF count, patients were classified into the following groups: ≤ 5, > 5 and ≤ 10, and > 10 WBCs/ HPF. According to the linear regression analysis, the urine WBC count was not correlated with clinical staging (p = 0.519), LDH level (p = 0.934), or lactic acid level (p = 0.549). Thus, the urine WBC/ HPF was not correlated with clinical staging or blood biomarkers associated with neonatal HIE.
This study makes significant contributions by delineating the first-day urine protein levels after birth and assessing their correlation with both the clinical Sarnat staging of neonatal HIE and patient neurodevelopmental outcomes at ≥1 year of age. Other outcomes, such as hearing impairment and a history of seizures before 1 year of age, were not associated with the urine protein level. Urine protein levels after birth serve as a biomarker to identify neonates with HIE. However, other urine biomarkers like RBC and WBC counts showed no association with clinical staging or neurodevelopmental outcomes.
These findings reflect the fact that renal injury could be associated with the degree of brain injury in patients with neonatal HIE. Neonatal HIE can cause multiple organ injury, including of the brain, liver, heart, and kidney. Neonatal HIE can cause renal injury, but cause less injury to brain could occur, which in some cases is reflected as severe urine proteinuria with less brain injury. Urine collection, particularly in neonates with HIE, may be difficult after birth, as oliguria or anuria are potential symptoms (15). Indeed, anuria could be a sign of severe HIE (13, 15, 34). Patients who underwent therapeutic hypothermia had less renal impairment than those who did not undergo hypothermia therapy. Kidney injury may persist in asphyxiated newborns despite improvement in serum creatinine (34). The positive predictive value of oliguria was moderate for neonatal HIE, though collecting urine to measure urine creatinine and other urine biomarkers may not be possible in this population (15). Urine protein levels were not associated with MRI findings in those who underwent therapeutic hypothermia, which probably was due to the fact that the treatment protected the brain from injury.
Blood troponin and LDH levels were not significantly correlated with urine protein levels in our study. This may be explained by the different degree of individual organ injury in patients with neonatal HIE (14). Lactic acid levels are more sensitively correlated with the degree of neonatal HIE than LDH and troponin levels (19, 20, 35). Early elevation of lactic acid levels may be correlated with the severity of neonatal HIE and help predict residual encephalopathy in newborns at discharge (20, 36). Troponin levels were found to be significantly higher in neonates with hypotension and injury of liver but not acute renal injury in neonatal HIE (5, 36).
This study had some limitations. First, a limited number of cases of HIE were included to analyze, which could have introduced bias into our findings. The data also had some bias related to HIE, which comprise fewer cases. Therefore, future studies that include a greater number of cases are warranted. However, the level of bias should be reduced since our patients were taken from a specific population without differences in gestational age, body weight, or gender, and those with medical conditions other than HIE (congenital anomalies, genetic disorder, or preterm birth) were excluded. Second, aggressive imaging studies were not available for those patients with stage 1 HIE and favorable outcomes. However, to confirm the absence of significant brain parenchymal lesions, patients in this population could undergo a series of HUS and clinical follow-up for 1 year to support the image finding. Third, urine analyses are often difficult to perform after birth as some patients have renal damage which may limit its clinical application, particularly for early hypothermia therapy. Collecting the first urine with a Foley catheter is an alternative choice for urine collection. Because urine creatinine analysis requires a large amount of urine, the creatinine values were not examined in HIE patients in our patients. However, measuring urine protein from a small sample could be utilized as a biomarker for predicting neurodevelopmental outcomes.
High levels of urine protein detected in a simple analysis of the first urine sample taken within the first day after birth may be associated with neurodevelopmental outcomes in patients aged one year or older. Using urine protein levels as a biomarker for neonatal HIE could serve as a tool to correlate with neurodevelopmental outcomes at ≥1 year of age.
The original contributions presented in the study are included in the article/supplementary material, further inquiries can be directed to the corresponding author/s.
The studies involving humans were approved by the Internal Review Board of Chung Shan Medical University Hospital provided the ethics approval (IRB #: CS2-14003). The studies were conducted in accordance with the local legislation and institutional requirements. Written informed consent for participation in this study was provided by the participants’ legal guardians/next of kin.
Y-CH: Data curation, Formal analysis, Project administration, Writing – review & editing. J-NS: Data curation, Formal analysis, Project administration, Supervision, Writing – review & editing. I-CL: Conceptualization, Data curation, Formal analysis, Funding acquisition, Investigation, Methodology, Project administration, Resources, Software, Supervision, Validation, Visualization, Writing – original draft, Writing – review & editing.
The author(s) declare that financial support was received for the research and/or publication of this article. This work was supported by the grant Chung Shan Medical University Hospital CSH-2024-C-008 and Feng Chia University FCU/CSMU 110–002.
We thank all those who participated in the present project.
The authors declare that the research was conducted in the absence of any commercial or financial relationships that could be construed as a potential conflict of interest.
All claims expressed in this article are solely those of the authors and do not necessarily represent those of their affiliated organizations, or those of the publisher, the editors and the reviewers. Any product that may be evaluated in this article, or claim that may be made by its manufacturer, is not guaranteed or endorsed by the publisher.
1. Badurdeen, S, Roberts, C, Blank, D, Miller, S, Stojanovska, V, Davis, P, et al. Haemodynamic instability and brain injury in neonates exposed to hypoxia(−)Ischaemia. Brain Sci. (2019) 9:49. doi: 10.3390/brainsci9030049
2. Armstrong, K, Franklin, O, Sweetman, D, and Molloy, EJ. Cardiovascular dysfunction in infants with neonatal encephalopathy. Arch Dis Child. (2012) 97:372–5. doi: 10.1136/adc.2011.214205
3. Saugstad, OD, Rootwelt, T, and Aalen, O. Resuscitation of asphyxiated newborn infants with room air or oxygen: an international controlled trial: the Resair 2 study. Pediatrics. (1998) 102:e1. doi: 10.1542/peds.102.1.e1
4. Deorari, AK, Broor, S, Maitreyi, RS, Agarwal, D, Kumar, H, Paul, VK, et al. Incidence, clinical spectrum, and outcome of intrauterine infections in neonates. J Trop Pediatr. (2000) 46:155–60. doi: 10.1093/tropej/46.3.155
5. Lee, IC, Yu, CS, Wong, SH, and Lue, KH. Troponin I levels in neonatal hypoxic-ischemic encephalopathy are related to cardiopulmonary comorbidity and neurodevelopmental outcomes. J Clin Med. (2021) 10:4010. doi: 10.3390/jcm10174010
6. Allen, KA, and Brandon, DH. Hypoxic ischemic encephalopathy: pathophysiology and experimental treatments. Newborn Infant Nurs Rev. (2011) 11:125–33. doi: 10.1053/j.nainr.2011.07.004
7. Azzopardi, DV, Strohm, B, Edwards, AD, Dyet, L, Halliday, HL, Juszczak, E, et al. Moderate hypothermia to treat perinatal asphyxial encephalopathy. N Engl J Med. (2009) 361:1349–58. doi: 10.1056/NEJMoa0900854
8. Edwards, AD, Brocklehurst, P, Gunn, AJ, Halliday, H, Juszczak, E, Levene, M, et al. Neurological outcomes at 18 months of age after moderate hypothermia for perinatal hypoxic ischaemic encephalopathy: synthesis and meta-analysis of trial data. BMJ. (2010) 340:c363. doi: 10.1136/bmj.c363
9. Nakamura, T, Asanuma, H, Kusuda, S, Imai, K, Hosono, S, Kato, R, et al. Multicenter study for brain/body hypothermia for hypoxic-ischemic encephalopathy: changes in HMGB-1. Pediatrics Int. (2017) 59:1074–9. doi: 10.1111/ped.13377
10. Laptook, A, Tyson, J, Shankaran, S, McDonald, S, Ehrenkranz, R, Fanaroff, A, et al. Elevated temperature after hypoxic-ischemic encephalopathy: risk factor for adverse outcomes. Pediatrics. (2008) 122:491–9. doi: 10.1542/peds.2007-1673
11. Murray, DM. Biomarkers in neonatal hypoxic-ischemic encephalopathy-review of the literature to date and future directions for research. Handb Clin Neurol. (2019) 162:281–93. doi: 10.1016/B978-0-444-64029-1.00013-8
12. Saw, CL, Rakshasbhuvankar, A, Rao, S, Bulsara, M, and Patole, S. Current practice of therapeutic hypothermia for mild hypoxic ischemic encephalopathy. J Child Neurol. (2019) 34:402–9. doi: 10.1177/0883073819828625
13. Perlman, JM, Tack, ED, Martin, T, Shackelford, G, and Amon, E. Acute systemic organ injury in term infants after asphyxia. Am J Diseases Children. (1989) 143:617–20. doi: 10.1001/archpedi.1989.02150170119037
14. Martín-Ancel, A, García-Alix, A, Gayá, F, Cabañas, F, Burgueros, M, and Quero, J. Multiple organ involvement in perinatal asphyxia. J Pediatr. (1995) 127:786–93. doi: 10.1016/S0022-3476(95)70174-5
15. Aggarwal, A, Kumar, P, Chowdhary, G, Majumdar, S, and Narang, A. Evaluation of renal functions in asphyxiated newborns. J Trop Pediatr. (2005) 51:295–9. doi: 10.1093/tropej/fmi017
16. Hankins, GD, Koen, S, Gei, AF, Lopez, SM, Van Hook, JW, and Anderson, GD. Neonatal organ system injury in acute birth asphyxia sufficient to result in neonatal encephalopathy. Obstet Gynecol. (2002) 99:688–91. doi: 10.1097/00006250-200205000-00004
17. Allen, J, Zareen, Z, Doyle, S, Whitla, L, Afzal, Z, Stack, M, et al. Multi-organ dysfunction in cerebral palsy. Front Pediatr. (2021) 9:9. doi: 10.3389/fped.2021.668544
18. Banupriya, C, Ratnakar, DP, Mondal, N, Vishnu, B, and Koner, BC. Can urinary excretion rate of malondialdehyde, uric acid and protein predict the severity and impending death in perinatal asphyxia? Clin Biochem. (2008) 41:968–73. doi: 10.1016/j.clinbiochem.2008.04.011
19. Polackova, R, Salounova, D, and Kantor, L. Lactate as an early predictor of psychomotor development in neonates with asphyxia receiving therapeutic hypothermia. Biomed Papers Med Facul Univ Palacky Olomouc Czechoslovakia. (2018) 162:144–8. doi: 10.5507/bp.2017.052
20. Wu, TW, Tamrazi, B, Hsu, KH, Ho, E, Reitman, AJ, Borzage, M, et al. Cerebral lactate concentration in neonatal hypoxic-ischemic encephalopathy: in relation to time, characteristic of injury, and serum lactate concentration. Front Neurol. (2018) 9:293. doi: 10.3389/fneur.2018.00293
21. Chiang, MC, Lien, R, Chu, SM, Yang, PH, Lin, JJ, Hsu, JF, et al. Serum lactate, brain magnetic resonance imaging and outcome of neonatal hypoxic ischemic encephalopathy after therapeutic hypothermia. Pediatr Neonatol. (2016) 57:35–40. doi: 10.1016/j.pedneo.2015.04.008
22. Wu, PM, Lin, CH, Lee, HT, Shih, HI, Huang, CC, and Tu, YF. Early blood biomarkers distinguish inflammation from neonatal hypoxic-ischemia encephalopathy. Neurochem Res. (2020) 45:2712–22. doi: 10.1007/s11064-020-03119-7
23. Celik, Y, Atici, A, Gulasi, S, Makharoblidze, K, Eskandari, G, Sungur, MA, et al. The effects of selective head cooling versus whole-body cooling on some neural and inflammatory biomarkers: a randomized controlled pilot study. Ital J Pediatr. (2015) 41:79. doi: 10.1186/s13052-015-0188-5
24. Lopez-Suarez, O, Concheiro-Guisan, A, Sanchez-Pintos, P, Cocho, JA, Fernandez Lorenzo, JR, and Couce, ML. Acylcarnitine profile in neonatal hypoxic-ischemic encephalopathy: the value of butyrylcarnitine as a prognostic marker. Medicine. (2019) 98:e15221. doi: 10.1097/MD.0000000000015221
25. Yang, L, Li, D, and Chen, S. Hydrogen water reduces NSE, IL-6, and TNF-α levels in hypoxic-ischemic encephalopathy. Open Med. (2016) 11:399–406. doi: 10.1515/med-2016-0072
26. Massaro, AN, Wu, YW, Bammler, TK, Comstock, B, Mathur, A, McKinstry, RC, et al. Plasma biomarkers of brain injury in neonatal hypoxic-ischemic encephalopathy. J Pediatr. (2018) 194:e61:67–75.e1. doi: 10.1016/j.jpeds.2017.10.060
27. Graham, EM, Everett, AD, Delpech, JC, and Northington, FJ. Blood biomarkers for evaluation of perinatal encephalopathy: state of the art. Curr Opin Pediatr. (2018) 30:199–203. doi: 10.1097/MOP.0000000000000591
28. Zhu, Y, Yun, Y, Jin, M, Li, G, Li, H, Miao, P, et al. Identification of novel biomarkers for neonatal hypoxic-ischemic encephalopathy using iTRAQ. Ital J Pediatr. (2020) 46:67. doi: 10.1186/s13052-020-00822-7
29. Sarnat, HB, and Sarnat, MS. Neonatal encephalopathy following fetal distress. A clinical and electroencephalographic study. Arch Neurol. (1976) 33:696–705. doi: 10.1001/archneur.1976.00500100030012
30. Haga, M, Kawabata, K, Sumiya, W, Kurita, S, Imanishi, T, Kanno, C, et al. The relationship between serum Total bilirubin and severity of hypoxic injury in neonatal hypoxic-ischemic encephalopathy. Am J Perinatol. (2020) 39:0944–50. doi: 10.1055/s-0040-1718879
31. Wroblewska-Seniuk, KE, Dabrowski, P, Szyfter, W, and Mazela, J. Universal newborn hearing screening: methods and results, obstacles, and benefits. Pediatr Res. (2017) 81:415–22. doi: 10.1038/pr.2016.250
32. Hsueh, CY, Huang, CY, Yang, CF, Chang, CC, Lin, WS, Cheng, HL, et al. Hearing characteristics of infantile-onset Pompe disease after early enzyme-replacement therapy. Orphanet J Rare Dis. (2021) 16:348. doi: 10.1186/s13023-021-01817-1
33. Viswanathan, N, Vidler, M, and Richard, B. Hearing thresholds in newborns with a cleft palate assessed by auditory brain stem response. Cleft Palate Craniofacial J. (2008) 45:187–92. doi: 10.1597/06-078.1
34. Nour, I, Elmaghraby, R, Shehata, R, El-Refaey, A, Aldomiaty, H, Mosbah, A, et al. Selective head cooling and acute kidney injury in neonates with hypoxic ischemic encephalopathy. J Neonatal-Perinatal Med. (2020) 13:21–30. doi: 10.3233/NPM-180200
35. Lee, IC, Wong, SH, Wang, XA, and Yu, CS. Identifying early diagnostic biomarkers associated with neonatal hypoxic-ischemic encephalopathy. Diagnostics. (2021) 11:2056. doi: 10.3390/diagnostics11050897
Keywords: newborn, hypoxic–ischemic encephalopathy, biomarker, proteinuria, neurodevelopmental outcomes
Citation: Hu Y-C, Sheu J-N and Lee I-C (2025) The role of proteinuria on a simple urinalysis in neonatal hypoxic–ischemic encephalopathy: association with clinical and neurodevelopmental outcomes. Front. Neurol. 16:1451346. doi: 10.3389/fneur.2025.1451346
Received: 19 June 2024; Accepted: 05 March 2025;
Published: 18 March 2025.
Edited by:
Jo Madeleine Wilmshurst, University of Cape Town, South AfricaReviewed by:
Riffat Mehboob, National Institutes of Health (NIH), United StatesCopyright © 2025 Hu, Sheu and Lee. This is an open-access article distributed under the terms of the Creative Commons Attribution License (CC BY). The use, distribution or reproduction in other forums is permitted, provided the original author(s) and the copyright owner(s) are credited and that the original publication in this journal is cited, in accordance with accepted academic practice. No use, distribution or reproduction is permitted which does not comply with these terms.
*Correspondence: Inn-Chi Lee, eTYxMEBjc211LmVkdS50dw==
Disclaimer: All claims expressed in this article are solely those of the authors and do not necessarily represent those of their affiliated organizations, or those of the publisher, the editors and the reviewers. Any product that may be evaluated in this article or claim that may be made by its manufacturer is not guaranteed or endorsed by the publisher.
Research integrity at Frontiers
Learn more about the work of our research integrity team to safeguard the quality of each article we publish.