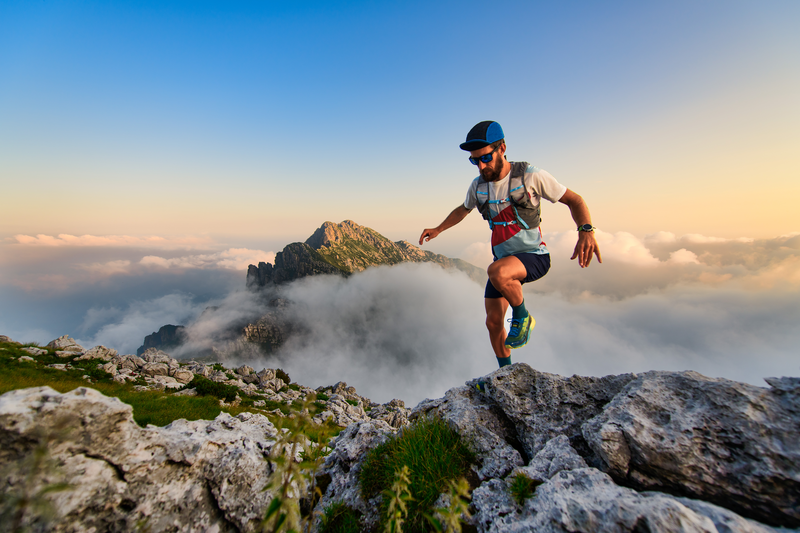
94% of researchers rate our articles as excellent or good
Learn more about the work of our research integrity team to safeguard the quality of each article we publish.
Find out more
ORIGINAL RESEARCH article
Front. Neurol.
Sec. Neurogenetics
Volume 16 - 2025 | doi: 10.3389/fneur.2025.1438207
This article is part of the Research Topic Genetics in Rare Neurological Diseases: From Discovery to Targeted Treatment View all 12 articles
The final, formatted version of the article will be published soon.
You have multiple emails registered with Frontiers:
Please enter your email address:
If you already have an account, please login
You don't have a Frontiers account ? You can register here
Amyotrophic lateral sclerosis (ALS) is a severe neurodegenerative disease characterized by a progressive loss of motor neurons and muscle atrophy. Genetic factors are known to play important roles in ALS and concomitant presence of rare variants in ALS patients have been increasingly reported. In order to explore the genetic variants in ALS patients within the context of oligogenic inheritance and to elucidate the clinical heterogeneity observed in these patients, we conducted whole-genome sequencing on 34 familial ALS (FALS) probands. In one proband, we identified a CHCHD10 p.Gly66Val variant, along with three additional variants: UNC13A p.Leu1034Val, SUSD1 p.Trp704Ser, and SQSTM1 p.His359del. Variants in CHCHD10 have been found in patients with ALS and other neurodegenerative diseases with significant clinical variability, but the combinatorial effect of variants in CHCHD10 and other ALS-related genes have not been fully studied. Our findings suggest that the combined impact of these four variants contributes to the clinical presentation of ALS in this patient, distinguishing it from other, less severe neuromuscular disorders associated with CHCHD10 mutations. This patient exhibited a slow disease progression and a prolonged survival duration, in line with features of ALS patients with CHCHD10 variants. This implies that the CHCHD10 p.Gly66Val variant may play a predominant role in shaping the patient's phenotype, while the other three variants may primarily contribute to the occurrence of ALS.Overall, our study further supports the oligogenic pathogenic basis of ALS and offers new insights into understanding the intricate clinical presentations associated with CHCHD10 variants.
Keywords: Amyotrophic Lateral Sclerosis, CHCHD10, oligogenic pathogenesis, Whole-genome sequencing, Genotype-phenotype analysis
Received: 25 May 2024; Accepted: 28 Feb 2025.
Copyright: © 2025 Wang, Mi, Wang, Jiang, Mao, Heng, Li and Deng. This is an open-access article distributed under the terms of the Creative Commons Attribution License (CC BY). The use, distribution or reproduction in other forums is permitted, provided the original author(s) or licensor are credited and that the original publication in this journal is cited, in accordance with accepted academic practice. No use, distribution or reproduction is permitted which does not comply with these terms.
* Correspondence:
Min Deng, Peking University Third Hospital, Haidian, China
Disclaimer: All claims expressed in this article are solely those of the authors and do not necessarily represent those of their affiliated organizations, or those of the publisher, the editors and the reviewers. Any product that may be evaluated in this article or claim that may be made by its manufacturer is not guaranteed or endorsed by the publisher.
Research integrity at Frontiers
Learn more about the work of our research integrity team to safeguard the quality of each article we publish.