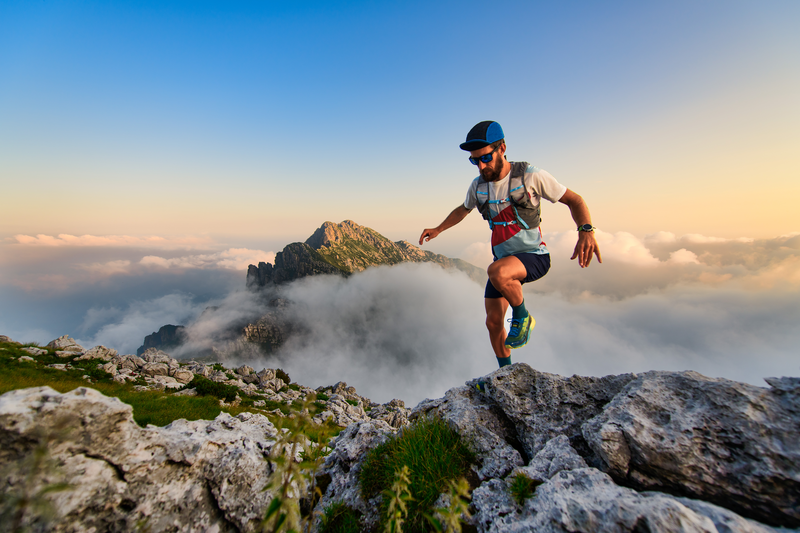
94% of researchers rate our articles as excellent or good
Learn more about the work of our research integrity team to safeguard the quality of each article we publish.
Find out more
BRIEF RESEARCH REPORT article
Front. Neurol. , 10 December 2024
Sec. Neurological Biomarkers
Volume 15 - 2024 | https://doi.org/10.3389/fneur.2024.1515252
Objectives: The aims of this study were to investigate whether CSF sTREM2 may be a potential marker of disease monitoring for amyotrophic lateral sclerosis (ALS).
Methods: We investigated whether CSF sTREM2 levels are altered in ALS patients and are correlated with upper motor neuron (UMN) burden and disease progression.
Results: CSF sTREM2 was greater in the ALS patients than in the controls (p = 0.002). Elevated CSF sTREM2 was associated with the UMN score (r = 0.38, p = 0.009), ΔFS (r = 0.30, p = 0.04) and serum NFL (lg) (r = 0.35, p = 0.015). As the motor band sign (MBS) score increased, the CSF sTREM2 level increased (p-trend = 0.014). Furthermore, the correlations became stronger (UMN score (r = 0.50, p = 0.01) ΔFRS (r = 0.52, p = 0.008) and serum NFL (lg) (r = 0.55, p = 0.004) when estimated only among patients with a disease duration >12 months.
Conclusion: We found that CSF sTREM2 is elevated in ALS patients and may be a novel marker, probably reflecting upper motor unit severity and prognosis.
ALS is a fatal central nervous system neurodegenerative disease and presents as a combination of upper (UMN) and lower motor neuron (LMN) dysfunction that leads to progressive weakness of voluntary skeletal muscles involved in limb movement (1). The challenge for current research is to discover novel ALS biomarkers to identify or monitor disease progression (2). In particular, LMN burden can be quantified by electromyography, but few reliable biomarkers are available to quantify UMN impairment.
Research on postmortem tissue and animal models has revealed an association between microglia and ALS (3, 4). The intricate cellular interplay between immune cells and upper motor neurons has been observed in the motor cortex of both ALS mice and ALS patients (5). Axonal loss in the corticospinal tract is associated with microglia (6). Therefore, the activation of microglia could be an attractive biomarker for tracking this disease and a potential outcome parameter for future clinical trials.
However, it is difficult to directly observe central microglia in clinical practice. Recently, CSF soluble TREM2 (sTREM2) has been evaluated as a biomarker of microglial activation in the context of neuroinflammatory and neurodegenerative diseases (7). Since sTREM2 is a key protein involved in the activation of microglia, the question arises whether sTREM2 levels are pathologically altered in the ALS.
In this study, we first compared CSF sTREM2 protein levels in patients with ALS and controls. We then evaluated the association of sTREM2 with clinical presentation. We found that CSF sTREM2, which may be a candidate marker for ALS disease monitoring, is significantly elevated in ALS patients and is associated with UMN burden and disease progression.
The current study included 47 sporadic ALS patients [according with the El Escorial revised criteria (8)] and 23 controls from the Peking University Third Hospital (PUTH). We included as CSF-controls subjects who underwent an intraspinal anesthesia in minor surgery and match for age and sex with ALS group. All participants met the following features: no other neurodegenerative disease such as Alzheimer’s disease, no tumoral or systemic inflammatory disease and no active anti-inflammatory drugs intake. The study was approved by the ethics committee of PUTH (M2024097).
For all patients, baseline demographic information and clinical data were collected directly during the patient’s first visit to PUTH. The demographic and clinical characteristics included age, sex, site of onset, age at onset, disease duration, and disease extent as assessed by the ALS Functional Rating Score-Revised (ALSFRS-R).
UMN score was assessed by Penn’s upper motor neuron scale (9).
Research with Quantitative Susceptibility Mapping (QSM) proposed MBS can serve as a possible radiological sign of severe UMN burden in ALS patients (10). Nineteen ALS participants agreed to undergo a full brain MRI protocol, including T2*/GRE sequence. MRI was performed using 3-T clinical magnetic resonance system (MAGNETOM TRIO TIM, Siemens, Germany). T2*/GRE sequence scans were performed using the T2*-weighted gradient echo protocol. 20-channel head-and-neck collar was used. Imaging sequence parameters were as follows: repetition time 800 ms, echo time 30 ms, flip angle 20°, slice thickness 5 mm, phase FOV 128 × 128 mm. All SWI sequences were assessed on radiological PACS workstations (GE) independently by 2 doctors. Raters were blinded to the participant’s age, sex and other clinical information. The raters evaluated the degree of hypointensity in the motor cortex on SWI using a three-point ordinal scale from 0 to 2, as previously reported (11, 12): a total MBS score of 0–12: The human primary motor cortex is somatotopically organized into 3 regions, the most medial part, the most lateral parts and the intermediate region. Compared with the peripheral gyrus, each region obtains a score, which is isointense (0), mildly hypointense (1), and markedly hypointense (2).
The progression rate (ΔFS) was calculated as follows: ΔFS (13) = (48 − ALSFRS-R Score at Time of Diagnosis)/Duration From Onset to Diagnosis (Months).
As a marker of axonal injury, serum NFL has been shown to be associated with progression and prognosis in patients with ALS (14). Serum NfL levels were measured with an ultrasensitive single-molecule array (Simoa) platform provided by Quanterix (Lexington, MA, United States). Measurements were performed on the fully automated HD-1 Analyzer (Quanterix) instrument using the NF-L Beta kit from Quanterix, which employs an anti-NFL monoclonal antibody produced by UmanDiagnostics (Umeå, Sweden). The interassay coefficients of variation (CVs) were <10%.
The CSF samples were collected in polypropylene tubes immediately by standard lumbar puncture and sent to the laboratory in 2 h. Samples were centrifuged at 2,000 × g for 10 min, and then stored in enzyme-free EP tubes at −80°C. The thaw/freezing cycle was limited not to surpass 2 times. CSF sTREM2 was determined with one ELISA kit (Human TREM2 SimpleStep ELISA kit; Abcam, No. Ab224881). Measurements were performed on the microplate reader (Tecan Spark, Switzerland). The within-batch CV was <5% and the inter-batch CV was <20%.
Cerebrospinal fluid and serum samples were collected on the same day. The measurements were performed in duplicate by experienced technicians who were blind to the clinical information, and the mean concentrations of duplicates were selected for the statistical analyses.
The Shapiro–Wilk test (S–W test) was used to evaluate whether continuous variables conformed to a normal distribution. Quantitative variables are expressed as the means ± standard deviations if they follow a normal distribution; otherwise, they are expressed as medians (interquartile ranges). Qualitative variables are expressed as a percentage of each group. The expression levels of sTREM2 in cerebrospinal fluid and NFL in serum were converted to log 10(X) values to conform to normal conditions.
Differences in baseline features were determined via the χ2 test (for categorical variables) and the Mann–Whitney U test (for continuous variables).
With sex and age as covariables, the independent correlation between the CSF sTREM2 level and ALS status was evaluated via a logistic regression model, and the odds ratio (OR) was calculated.
Cerebrospinal fluid sTREM2 levels were correlated with clinical data via Pearson and Spearman correlations.
The χ2 trend test was used to examine the trend of sTREM2 (dividing sTREM2 into 2 groups on the basis of the median) to change with MBS score.
Two-sided p-values <0.05 were considered statistically significant.
SPSS 26.0 was used for statistical analysis. Graphs were generated via GraphPad Prism 9.5.
In this study, we aimed to verify whether sTREM2 levels are associated with ALS. We performed intergroup comparisons of participant characteristics and CSF sTREM2 levels. The demographic and biomarker values of the control and ALS patients are shown in Table 1. Age and gender did not differ between the groups. As expected, the groups differed with respect to their biomarker profiles (Figure 1). Logistic regression models were designed to identify adjusted estimates of the association of sTREM2 levels in CSF with ALS status (control = 0; ALS = 1). sTREM2 levels in CSF were an independent predictor of ALS status after adjusting for age and sex, with an odds ratio (OR) = 1.22 (CI 95% = 1.06–1.40, p = 0.005). Compared with those in controls, the levels of CSF sTREM2 in ALS patients are significantly greater and may be an independent risk factor for ALS.
Figure 1. (A) The levels of soluble sTREM2 are significantly greater in ALS patients than in controls (p < 0.05). (B) Positive correlation between sTREM2 levels (lg) and the ΔFRS. (C) The positive correlation between sTREM2 levels (lg) and NFL. (D) Positive correlation between sTREM2 levels (lg) and the UMN score.
Next, considering the close association between microglia in the central nervous system and motor units in the cortex, we explored whether there was an association between sTREM2 and UMN burden. We collected and analyzed the UMN score and MBS, as previously described. The UMN score was significantly positively correlated with the sTREM2 level (r = 0.38, p = 0.009) (Figure 1). In addition, we compared sTREM2 levels across different MBS score groups. Adjusting for changes in the MBS, the levels of sTREM2 increased (p-trend = 0.014) (Figure 2). These findings suggest that sTREM2 may reflect the potential effects of activated microglia on nearby motor neurons.
Figure 2. (A) An ALS patient with T2* intermediate regions of the motor cortex with marked hypointensity. (B) CSF sTREM2 at different MBS levels.
We studied the relationships between CSF sTREM2 and biomarkers of axonal injury and disease progression using Pearson and Spearman correlations. A significant correlation was found between the sTREM2 level and the serum NFL level (r = 0.35, p = 0.015) and between the sTREM2 level and the ΔFS value (r = 0.30, p = 0.04) in ALS patients (Figure 1). Interestingly, we found no correlation between CSF sTREM2 levels and the ALSFRS-R score (p = 0.15) or disease course (p = 0.08).
Considering that the role of microglia may differ across different stages of the disease, we performed subgroup analysis. The ALS group was divided into subgroups according to the duration of disease (bounded by 12 months). When this correlation was estimated only among patients with a disease course >12 months, the sTREM2 (lg) was still associated with the UMN score (r = 0.50, p = 0.01), ΔFS (r = 0.52, p = 0.008) and serum NFL (lg) (r = 0.55, p = 0.004). In addition, there was a significant negative correlation (r = −0.40, p = 0.047) with ALSFRS-R (Figure 3). Nevertheless, no association was found in the subgroup with a disease duration <12 months. It seems to indicate that the role of sTREM2 in different pathological stages of the disease is not entirely consistent.
Figure 3. Subgroup analysis: ALS patients with a disease course >12 months. (A) Positive correlation between sTREM2 levels (lg) and the ΔFRS. (B) The negative correlation between sTREM2 levels (lg) and FRS.
In this study, we found that CSF sTREM2 levels in patients with ALS were elevated and correlated with the UMN burden, which may provide some diagnostic support, especially for patients with insignificant upper motor neuron involvement. In addition, we found that patients with high sTREM2 levels had faster disease progression, which suggests a potential biomarker for disease monitoring in ALS patients.
sTREM2 may be a candidate indicator of upper motor unit involvement in ALS. The sTREM2 protein, which is expressed mainly in microglia of the central nervous system and is abundant in cerebrospinal fluid, has been evaluated as a biomarker of microglial activation (15), playing a key role in the signaling pathway that triggers microglial activation. A recent study revealed that activation of the pyroptosis pathway in ALS white matter microglia contributes to neuronal degeneration in the motor cortex and pyramidal tract, possibly by affecting axonal health (16).
In this study, the UMN score and MBS were used as indicators of the severity of upper motor neuron involvement. We observed that the sTREM2 level tended to increase in ALS patients with lower MBS signals and wider distributions. Iron deposition in the brain mainly occurs in malnourished microglia, and inflammatory changes and blood-brain barrier disorders play roles in iron homeostasis (17). Activated microglia can play a role in phagocytosis, swallowing excess iron in neurodegenerative diseases (18). In turn, iron overload impairs the motility of microglia and promotes aging and proinflammatory phenotypes (19). However, the direct relationship between sTREM2 and iron deposition has not been fully elucidated. Shi et al. (20) reported that CSF ferritin can act as an alternative biomarker of sTREM2-mediated microglial function in AD patients, playing an upstream role in inducing neuroinflammation. Previous studies have suggested that the “motor band sign” is related to the iron deposits shown at autopsy (21, 22). These degenerative iron accumulations are observed as hypointensities in the primary motor cortex on T2-weighted, T2*-weighted or susceptibility-weighted imaging (SWI), which are more predominant with UMN-ALS than with LMN-ALS (23, 24). Therefore, we included the MBS and speculated that there may also be a certain connection between the MBS and sTREM2.
sTREM2 may be a candidate indicator for disease progression in ALS patients. The sTREM2 may bind to pathological proteins and may affect signal communication with nearby cells (25), which raises the possibility that sTREM2 may be causally linked to disease progression. In this study, we found a close positive correlation between sTREM2 and ΔFS and NFL, indicating a link with the pathological phenomenon of axonal damage (26). Consistent with previous studies (27), no association was found with the FRS, but we did find an association in patients with an advanced disease course (>12 months). We speculate that the early increase in sTREM2 expression may reflect an initial immune response to the deposition of pathological aggregates, but may initiate destructive effects after a certain stage. It is likely that microglia adopt an M2-like neuroprotective state early in disease but transition to an M1-like toxic state as ALS progresses, and such transitions in the microglial activation state occur asynchronously (28). However, Rosén et al. (29) reported no difference in CSF sTREM2 levels among ALS patients, mimics, and controls. The reason for this difference may be due to differences in the research subjects and the detection methods used. In the study by Rosén et al. (29), 9 patients presented with manifestations of FTD, and 3 patients had a family history of ALS; our study subjects were patients with sporadic ALS. Because ALS is a highly heterogeneous disease, differences in inclusion criteria and disease stage may also affect outcomes. In addition, we used ELISA to analyze samples, whereas Rosén et al. (29) used the Meso Scale Discovery method.
Previously, microglial sTREM2 was proposed as a novel biomarker for other neurological inflammatory disorders (30–32), such as AD and MS. Recent studies have demonstrated an interaction between TDP-43 and TREM2 in vitro and in vivo as well as in ALS patient tissues by mass spectrometry and surface plasmon resonance analysis (33).
In this study, we found that CSF sTREM2 may also be a biomarker for disease monitoring in ALS patients.
This research, however, is subject to several limitations. First, the sample size is small, which can lead to sample bias or the ability to extend the results to the ALS population. Second, the lack of analysis of longitudinal patient data, including clinical data and sTREM2 levels, may limit any conclusions about progression. Third, the semiquantitative analysis of the MBS using the 3 Tesla MR system in this study may not be as accurate as the quantitative analysis (7 T QSM sequence). Therefore, the results should be replicated in large-sample, longitudinal data to analyze the effects of CSF sTREM2-associated inflammatory activity at different stages of the disease and to determine whether it is associated with disease progression.
In conclusion, our results support that CSF sTREM2 may serve as a novel biomarker of disease monitoring for ALS, indicating upper motor neuron and axonal damage. Characterization of biomarkers of CSF sTREM2 and microglial activation will increase the understanding of potential neuroimmune mechanisms relevant to ALS.
The original contributions presented in the study are included in the article/supplementary material, further inquiries can be directed to the corresponding authors.
The studies involving humans were approved by Ethics committee of Peking University Third Hospital. The studies were conducted in accordance with the local legislation and institutional requirements. The participants provided their written informed consent to participate in this study. Written informed consent was obtained from the individual(s) for the publication of any potentially identifiable images or data included in this article.
LJ: Data curation, Writing – original draft, Writing – review & editing. JY: Data curation, Investigation, Writing – review & editing. WW: Investigation, Supervision, Writing – review & editing. XL: Investigation, Methodology, Software, Supervision, Writing – review & editing. YF: Data curation, Supervision, Writing – review & editing, Project administration. DF: Data curation, Supervision, Writing – review & editing, Funding acquisition.
The author(s) declare that financial support was received for the research, authorship, and/or publication of this article. This study was supported by the National Natural Science Foundation of China (81873784 and 82071426) and Clinical Cohort Construction Program of Peking University Third Hospital, Grant/Award Number: BYSYDL2019002.
The authors declare that the research was conducted in the absence of any commercial or financial relationships that could be construed as a potential conflict of interest.
The authors declare that no Generative AI was used in the creation of this manuscript.
All claims expressed in this article are solely those of the authors and do not necessarily represent those of their affiliated organizations, or those of the publisher, the editors and the reviewers. Any product that may be evaluated in this article, or claim that may be made by its manufacturer, is not guaranteed or endorsed by the publisher.
1. Feldman, EL, Goutman, SA, Petri, S, Mazzini, L, Savelieff, MG, Shaw, PJ, et al. Amyotrophic lateral sclerosis. Lancet. (2022) 400:1363–80. doi: 10.1016/S0140-6736(22)01272-7
2. Goutman, SA, Hardiman, O, Al-Chalabi, A, Chió, A, Savelieff, MG, Kiernan, MC, et al. Recent advances in the diagnosis and prognosis of amyotrophic lateral sclerosis. Lancet Neurol. (2022) 21:480–93. doi: 10.1016/S1474-4422(21)00465-8
3. Dols-Icardo, O, Montal, V, Sirisi, S, López-Pernas, G, Cervera-Carles, L, Querol-Vilaseca, M, et al. Motor cortex transcriptome reveals microglial key events in amyotrophic lateral sclerosis. Neurol Neuroimmunol Neuroinflamm. (2020) 7:e829. doi: 10.1212/NXI.0000000000000829
4. Maniatis, S, Äijö, T, Vickovic, S, Braine, C, Kang, K, Mollbrink, A, et al. Spatiotemporal dynamics of molecular pathology in amyotrophic lateral sclerosis. Science. (2019) 364:89–93. doi: 10.1126/science.aav9776
5. Jara, JH, Genç, B, Stanford, MJ, Pytel, P, Roos, RP, Weintraub, S, et al. Evidence for an early innate immune response in the motor cortex of ALS. J Neuroinflammation. (2017) 14:129. doi: 10.1186/s12974-017-0896-4
6. Jiang, YQ, Armada, K, and Martin, JH. Neuronal activity and microglial activation support corticospinal tract and proprioceptive afferent sprouting in spinal circuits after a corticospinal system lesion. Exp Neurol. (2019) 321:113015. doi: 10.1016/j.expneurol.2019.113015
7. Filipello, F, Goldsbury, C, You, SF, Locca, A, Karch, CM, and Piccio, L. Soluble TREM2: innocent bystander or active player in neurological diseases? Neurobiol Dis. (2022) 165:105630. doi: 10.1016/j.nbd.2022.105630
8. Brooks, BR, Miller, RG, Swash, M, and Munsat, TL. El Escorial revisited: revised criteria for the diagnosis of amyotrophic lateral sclerosis. Amyotroph Lateral Scler Other Motor Neuron Disord. (2000) 1:293–9. doi: 10.1080/146608200300079536
9. Quinn, C, Edmundson, C, Dahodwala, N, and Elman, L. Reliable and efficient scale to assess upper motor neuron disease burden in amyotrophic lateral sclerosis. Muscle Nerve. (2020) 61:508–11. doi: 10.1002/mus.26764
10. Costagli, M, Donatelli, G, Biagi, L, Caldarazzo Ienco, E, Siciliano, G, Tosetti, M, et al. Magnetic susceptibility in the deep layers of the primary motor cortex in amyotrophic lateral sclerosis. Neuroimage Clin. (2016) 12:965–9. doi: 10.1016/j.nicl.2016.04.011
11. Zejlon, C, Sennfält, S, Finnsson, J, Connolly, B, Petersson, S, Granberg, T, et al. Motor band sign is specific for amyotrophic lateral sclerosis and corresponds to motor symptoms. Ann Clin Transl Neurol. (2024) 11:1280–9. doi: 10.1002/acn3.52066
12. Yasui, M, Koh, J, Nakayama, Y, Sakata, M, Hiwatani, Y, Ishiguchi, H, et al. Diagnostic utility of susceptibility-weighted imaging in amyotrophic lateral sclerosis. J Neurol Sci. (2023) 444:120524. doi: 10.1016/j.jns.2022.120524
13. Kimura, F, Fujimura, C, Ishida, S, Nakajima, H, Furutama, D, Uehara, H, et al. Progression rate of ALSFRS-R at time of diagnosis predicts survival time in ALS. Neurology. (2006) 66:265–7. doi: 10.1212/01.wnl.0000194316.91908.8a
14. Thouvenot, E, Demattei, C, Lehmann, S, Maceski-Maleska, A, Hirtz, C, Juntas-Morales, R, et al. Serum neurofilament light chain at time of diagnosis is an independent prognostic factor of survival in amyotrophic lateral sclerosis. Eur J Neurol. (2020) 27:251–7. doi: 10.1111/ene.14063
15. Wang, S, Mustafa, M, Yuede, CM, Salazar, SV, Kong, P, Long, H, et al. Anti-human TREM2 induces microglia proliferation and reduces pathology in an Alzheimer’s disease model. J Exp Med. (2020) 217:e20200785. doi: 10.1084/jem.20200785
16. Van Schoor, E, Ospitalieri, S, Moonen, S, Tomé, SO, Ronisz, A, Ok, O, et al. Increased pyroptosis activation in white matter microglia is associated with neuronal loss in ALS motor cortex. Acta Neuropathol. (2022) 144:393–411. doi: 10.1007/s00401-022-02466-9
17. Ward, RJ, Dexter, DT, and Crichton, RR. Iron, neuroinflammation and neurodegeneration. Int J Mol Sci. (2022) 23:7267. doi: 10.3390/ijms23137267
18. Dusek, P, Hofer, T, Alexander, J, Roos, PM, and Aaseth, JO. Cerebral iron deposition in neurodegeneration. Biomol Ther. (2022) 12:714. doi: 10.3390/biom12050714
19. Angelova, DM, and Brown, DR. Microglia and the aging brain: are senescent microglia the key to neurodegeneration? J Neurochem. (2019) 151:676–88. doi: 10.1111/jnc.14860
20. Shi, X, Zhong, X, Zhou, H, Zhou, N, Hu, Y, and Ning, Y. The association between cerebrospinal ferritin and soluble triggering receptor expressed on myeloid cells 2 along Alzheimer’s continuum. Front Neurol. (2022) 13:961842. doi: 10.3389/fneur.2022.961842
21. Swanson, MEV, Mrkela, M, Murray, HC, Cao, MC, Turner, C, Curtis, MA, et al. Microglial CD68 and L-ferritin upregulation in response to phosphorylated-TDP-43 pathology in the amyotrophic lateral sclerosis brain. Acta Neuropathol Commun. (2023) 11:69. doi: 10.1186/s40478-023-01561-6
22. Chakraborty, S, Gupta, A, Nguyen, T, and Bourque, P. The “motor band sign”: susceptibility-weighted imaging in amyotrophic lateral sclerosis. Can J Neurol Sci. (2015) 42:260–3. doi: 10.1017/cjn.2015.40
23. Adachi, Y, Sato, N, Saito, Y, Kimura, Y, Nakata, Y, Ito, K, et al. Usefulness of SWI for the detection of iron in the motor cortex in amyotrophic lateral sclerosis. J Neuroimaging. (2015) 25:443–51. doi: 10.1111/jon.12127
24. Conte, G, Contarino, VE, Casale, S, Morelli, C, Sbaraini, S, Scola, E, et al. Amyotrophic lateral sclerosis phenotypes significantly differ in terms of magnetic susceptibility properties of the precentral cortex. Eur Radiol. (2021) 31:5272–80. doi: 10.1007/s00330-020-07547-5
25. Song, WM, Joshita, S, Zhou, Y, Ulland, TK, Gilfillan, S, and Colonna, M. Humanized TREM2 mice reveal microglia-intrinsic and-extrinsic effects of R47H polymorphism. J Exp Med. (2018) 215:745–60. doi: 10.1084/jem.20171529
26. Khalil, M, Teunissen, CE, Otto, M, Piehl, F, Sormani, MP, Gattringer, T, et al. Neurofilaments as biomarkers in neurological disorders. Nat Rev Neurol. (2018) 14:577–89. doi: 10.1038/s41582-018-0058-z
27. Jerico, I, Vicuna-Urriza, J, Blanco-Luquin, I, Macias, M, Martinez-Merino, L, Roldan, M, et al. Profiling TREM2 expression in amyotrophic lateral sclerosis. Brain Behav Immun. (2023) 109:117–26. doi: 10.1016/j.bbi.2023.01.013
28. Clarke, BE, and Patani, R. The microglial component of amyotrophic lateral sclerosis. Brain. (2020) 143:3526–39. doi: 10.1093/brain/awaa309
29. Rosén, C, Mitre, B, Nellgård, B, Axelsson, M, Constantinescu, R, Andersen, PM, et al. High levels of neurofilament light and YKL-40 in cerebrospinal fluid are related to poor outcome in ALS. J Neurol Sci. (2024) 463:123112. doi: 10.1016/j.jns.2024.123112
30. Ewers, M, Franzmeier, N, Suarez-Calvet, M, Morenas-Rodriguez, E, Caballero, MAA, Kleinberger, G, et al. Increased soluble TREM2 in cerebrospinal fluid is associated with reduced cognitive and clinical decline in Alzheimer's disease. Sci Transl Med. (2019) 11:eaav6221. doi: 10.1126/scitranslmed.aav6221
31. Suarez-Calvet, M, Kleinberger, G, Araque Caballero, MA, Brendel, M, Rominger, A, Alcolea, D, et al. sTREM2 cerebrospinal fluid levels are a potential biomarker for microglia activity in early-stage Alzheimer’s disease and associate with neuronal injury markers. EMBO Mol Med. (2016) 8:466–76. doi: 10.15252/emmm.201506123
32. Öhrfelt, A, Axelsson, M, Malmeström, C, Novakova, L, Heslegrave, A, Blennow, K, et al. Soluble TREM-2 in cerebrospinal fluid from patients with multiple sclerosis treated with natalizumab or mitoxantrone. Mult Scler. (2016) 22:1587–95. doi: 10.1177/1352458515624558
Keywords: soluble TREM2, cerebrospinal fluid, amyotrophic lateral sclerosis, MBS, UMN burden
Citation: Jiao L, Yang J, Wang W, Liu X, Fu Y and Fan D (2024) sTREM2 cerebrospinal fluid levels are a potential biomarker in amyotrophic lateral sclerosis and associate with UMN burden. Front. Neurol. 15:1515252. doi: 10.3389/fneur.2024.1515252
Received: 22 October 2024; Accepted: 28 November 2024;
Published: 10 December 2024.
Edited by:
Erdem Tüzün, Istanbul University, TürkiyeReviewed by:
Sabine Liebscher, LMU Munich University Hospital, GermanyCopyright © 2024 Jiao, Yang, Wang, Liu, Fu and Fan. This is an open-access article distributed under the terms of the Creative Commons Attribution License (CC BY). The use, distribution or reproduction in other forums is permitted, provided the original author(s) and the copyright owner(s) are credited and that the original publication in this journal is cited, in accordance with accepted academic practice. No use, distribution or reproduction is permitted which does not comply with these terms.
*Correspondence: Yu Fu, bGlsYWNfZnVAMTI2LmNvbQ==; Dongsheng Fan, ZHNmYW4yMDEwQGFsaXl1bi5jb20=
Disclaimer: All claims expressed in this article are solely those of the authors and do not necessarily represent those of their affiliated organizations, or those of the publisher, the editors and the reviewers. Any product that may be evaluated in this article or claim that may be made by its manufacturer is not guaranteed or endorsed by the publisher.
Research integrity at Frontiers
Learn more about the work of our research integrity team to safeguard the quality of each article we publish.