- 1Division of General Internal Medicine, Mayo Clinic, Rochester, MN, United States
- 2Department of General Internal Medicine, Mayo Clinic, Jacksonville, FL, United States
- 3Department of Biological Engineering, Massachusetts Institute of Technology, Cambridge, MA, United States
- 4Department of Cardiovascular Medicine, Mayo Clinic, Jacksonville, FL, United States
- 5Center for Clinical and Translational Science, Mayo Clinic, Rochester, MN, United States
- 6Department of Immunology, Mayo Clinic, Jacksonville, FL, United States
- 7Division of Quantitative Health Sciences, Rochester, MN, United States
- 8Department of Cardiovascular Medicine, University of Florida, Gainesville, FL, United States
- 9Department of Biostatistics, Mayo Clinic, Jacksonville, FL, United States
- 10Institute for Stem Cell Biology & Regenerative Medicine, Stanford University School of Medicine, Stanford, CA, United States
Background: Many patients experience persistent symptoms after COVID-19, a syndrome referred to as Long COVID (LC). The goal of this study was to identify novel new or worsening comorbidities self-reported in patients with LC.
Methods: Patients diagnosed with LC (n = 732) at the Mayo Long COVID Care Clinic in Rochester, Minnesota and Jacksonville, Florida were sent questionnaires to assess the development of new or worsening comorbidities following COVID-19 compared to patients with SARS-CoV-2 that did not develop LC (controls). Both groups were also asked questions screening for myalgic encephalomyelitis/chronic fatigue syndrome (ME/CFS), generalized joint hypermobility (GJH) and orthostatic intolerance. 247 people with LC (33.7%) and 40 controls (50%) responded to the surveys.
Results: In this study LC patients averaged 53 years of age and were predominantly White (95%) women (75%). The greatest prevalence of new or worsening comorbidities following SARS-CoV-2 infection in patients with LC vs. controls reported in this study were pain (94.4% vs. 0%, p < 0.001), neurological (92.4% vs. 15.4%, p < 0.001), sleep (82.8% vs. 5.3%, p < 0.001), skin (69.8% vs. 0%, p < 0.001), and genitourinary (60.6% vs. 25.0%, p = 0.029) issues. 58% of LC patients screened positive for ME/CFS vs. 0% of controls (p < 0.001), 27% positive for GJH compared to 10% of controls (p = 0.026), and a positive average score of 4.0 on orthostatic intolerance vs. 0 (p < 0.001). The majority of LC patients with ME/CFS were women (77%).
Conclusion: We found that comorbidities across 12 surveyed categories were increased in patients following SARS-CoV-2 infection. Our data also support the overlap of LC with ME/CFS, GJH, and orthostatic intolerance. We discuss the pathophysiologic, research, and clinical implications of identifying these conditions with LC.
1 Introduction
Soon after the start of the COVID-19 pandemic, patients presented to clinicians with new or worsening symptoms that persisted for many months after the initial SARS-CoV-2 infection (1, 2). This syndrome has been named Long COVID (LC), Post-COVID syndrome, and Post-Acute Sequelae of SARS-CoV-2 Infection (PASC). The US Centers for Disease Control and Prevention (CDC) and the US Department of Health and Human Services define LC as signs, symptoms, and conditions persisting for at least 4 weeks following COVID-19 (3). The National Academies of Science, Engineering, and Medicine (NASEM) defines LC as an infection-associated chronic condition following SARS-CoV-2 infection that lasts at least 3 months, affects at least one organ system, and can be continuous, relapsing, or remitting (4). In the first quarter of 2024, approximately 5.3% of US adults currently were estimated to have LC by the CDC (5). Consequently, the projected economic impact ranges from $140 to $600 billion annually (6).
LC impacts multiple organ systems, leading to exertional intolerance, fatigue, dyspnea, muscle and joint pain, orthostatic intolerance, and cognitive dysfunction (4, 7–9). An electronic health record (EHR) study of over 20,000 people with LC in Florida used an artificial intelligence program to identify subgroups of patients with different organ systems affected by LC that included cardiac and renal (34%); respiratory, sleep, and anxiety (33%); musculoskeletal and nervous (23%); and digestive and respiratory (10%) systems (10). LC is also more likely to occur in patients who are middle-age and of female sex (1, 11–13). CDC assessments estimate that LC also has a disproportionate impact on the transgender, Hispanic, and multiracial populations (5).
LC stands as a prominent recent example of how an infectious agent can be associated with chronic disease (14–17). Infectious disease is an established cause of neurodegeneration, including neurotropic infections such as Herpesviridae, Bornaviridae, Orthomyxoviridae (including influenza), Paramyxoviridae, Picornaviridae, Retroviridae and Flaviviridae (18–20). Post-infectious onset and the role of latent viruses is also increasingly appreciated in the development of myalgic encephalomyelitis/chronic fatigue syndrome (ME/CFS) among other related conditions (21–25). Accordingly, LC research is quickly revealing high rates of multisystem complex chronic diseases such as ME/CFS and postural orthostatic tachcyardiac syndrome (POTS) (26–30). Notably, these conditions are highly comorbid with each other, along with generalized joint hypermobility (GJH), hypermobile Ehlers-Danlos Syndrome (hEDS), and hypermobility spectrum disorders (HSD) (31–36). The constellation of symptoms and comorbidities reported in LC seem to be very similar to those found in ME/CFS, orthostatic intolerance/POTS, and hEDS/HSD (31–38).
Little data exists on a wide range of comorbidities that may arise with LC. The goal of this study was to determine whether new or worsening conditions occurred more frequently in patients diagnosed with LC at our clinic compared to COVID patients that did not develop LC. We additionally examined whether patients with LC were more likely to screen positive for ME/CFS, GJH, or orthostatic intolerance. We propose that the identification of overlapping conditions and their potential underlying mechanisms may offer important insight into research and clinical management of LC.
2 Methods
2.1 Diagnoses
Individuals attending the Long COVID Care Clinic at Mayo Clinic Florida and Rochester were diagnosed by general internal medicine physicians specialized in LC. A majority of LC physicians had additional specialization in fibromyalgia, ME/CFS, POTS, and/or hypermobility syndromes (13). All patients diagnosed with LC had persistent symptoms for at least 3 months following the start of acute SARS-CoV-2 infection. Controls had a confirmed history of SARS-CoV-2 infection but had not received a diagnosis of LC.
2.2 Long COVID clinic data collection
Patient data were collected over 1 month using two REDCap questionnaires sent to patients via the patient portal. The questionnaires were based on the Florida EDS Clinic questionnaire for patients with hEDS/HSD, with questions added or modified for LC, ME/CFS, POTS, and other conditions increasingly suspected in infection-associated chronic conditions (39, 40). Dedicated screening criteria for hypermobility, ME/CFS, and orthostatic intolerance were added. The Long COVID questionnaire obtained self-reported data on 115 demographic and health issues in total. This was sent to a sample of patients diagnosed with LC at the Mayo Clinic Long COVID Clinic in Jacksonville, Florida and Rochester, Minnesota. Health information focused on symptoms and comorbidities throughout the body and whether specific comorbidities were new or worse following COVID. A matching control questionnaire was created where wording was modified to ask participants about symptoms since prior COVID infections without mention of Post-COVID Syndrome or Long COVID, and was sent to a control group in Rochester, Minnesota consisting of individuals who had had prior COVID infection without diagnosis of LC. Specifically, the control participants were from a developing age-sex matched cohort specifically for Long COVID studies, currently in process of recruiting with 80 subjects enrolled at the time of the survey. The Long COVID questionnaire was sent to 494 patients in Florida and 238 patients in Minnesota. A total of 247 individuals completed the Long COVID questionnaire (33.7% response rate): 124 (25.1% response rate, 50.2% of total responses) from Florida and 123 (51.7% response rate, 49.8% of total responses) from Minnesota. The control questionnaire was completed by 40 individuals from Minnesota (50% response rate).
2.3 Statistical analysis
Categorical variables were summarized as frequency (percentage) and continuous variables were reported as median (range). Wilcoxon rank sum test was used to evaluate the difference in continuous variables between LC patients and controls while Fisher’s exact test was used to compare categorical variables. Multivariable logistic regression models were used to evaluate the difference between LC and control patients after adjusting for age and race (White vs. non-White). Firth’s penalized logistic regression was used for outcomes with zero events in the control group. All tests were two-sided and p values <0.05 were considered statistically significant. The analysis was performed using R (version 4.2.2).
2.4 Categories
We assessed whether patients diagnosed with LC observed new or worsening conditions for 14 major systems categories after SARS-CoV-2 infection compared to controls: allergies/sensitivities/intolerances, skin, neurologic, pain, sleep, autoimmune, genitourinary, mood, gastrointestinal, pulmonary, cardiac, endocrinologic, hematologic/oncologic, and dental disorders, as included in the original questionnaire.
A portion of the major categories also included an expanded set of specific conditions that respondents could select based on expert opinion of potentially common and/or underrecognized coexisting conditions (39). Those categories and conditions included:
• Skin: easy bruising, poor/slow wound healing, easy/abnormal scarring, abnormal striae, lipedema, psoriasis, eczema, rosacea, hives, and flushing.
• Neurologic: headache, migraine, Arnold Chiari malformation, intracranial hypertension/elevated CSF pressure, CSF leak, autonomic dysfunction not meeting criteria for postural orthostatic tachycardia syndrome, postural orthostatic tachycardia syndrome, vertigo, neuropathy (small and/or large), tinnitus, craniocervical instability, myalgic encephalomyelitis/chronic fatigue syndrome, autism/autism spectrum disorder, inappropriate sinus tachycardia, and recurrent syncope.
• Pain: fibromyalgia, chronic pain—non-muscular, chronic muscle pain, cervicalgia, central pain syndrome, central sensitization, muscle spasms, and complex regional pain syndrome.
• Sleep: obstructive sleep apnea, snoring, narcolepsy, idiopathic hypersomnia, insomnia, sleep disturbance, restless leg syndrome, parasomnia, and circadian rhythm disorders (such as delayed sleep phase syndrome).
• Genitourinary: bladder prolapse, uterine prolapse, recurrent urinary tract infections, recurrent yeast infections, recurrent vaginal bacterial infections, incontinence, pelvic floor spasm, pelvic floor dysfunction, dyspareunia, interstitial cystitis, endometriosis, and polycystic ovary syndrome (PCOS). Sexual and menstrual concerns were surveyed separately.
Finally, a miscellaneous category was included for emerging phenomena within infection-associated chronic illness to assess the level of recognition of those comorbidities, including mast cell disease, endothelial dysfunction, viral persistence/reactivation, vascular compression syndromes, mitochondrial impairment, and sensitivity to medications, based on expert discussion surrounding emerging areas in the field of infection-associated chronic conditions.
2.5 Screening for hypermobility, ME/CFS, orthostatic intolerance
Several screening criteria were embedded in the REDCap questionnaires to determine whether patients had ME/CFS, GJH, or orthostatic intolerance. The Institute of Medicine/National Academy of Science, Engineering and Medicine (IOM/NASEM) 2015 criteria were used for ME/CFS (41). The self-assessment 5-part hypermobility questionnaire was utilized to assess GJH. This is used in clinical practice to screen for hEDS/HSD as well, acknowledging that GJH may not always be symptomatic and is not alone sufficient to obtain a diagnosis of hEDS or HSD, where other criteria are required (42–44). A modified version of the orthostatic intolerance domain of the Composite Autonomic Symptom Scale 31 (COMPASS-31) questionnaire was used for orthostatic intolerance screening (45) (Supplementary material 1).
3 Results
3.1 Patient demographics
Controls were older (58.5 years) than patients with LC (53.0 years) (p = 0.021) (Table 1). However, most controls (70.0%) and patients with LC (75.3%) self-reported being female, which was not significantly different between groups (p = 0.52) (Table 1). Controls (100%) and patients with LC (93.9%) self-reported as White vs. non-White for race (p = 0.24) (Table 1).
3.2 New or worsening comorbidities after COVID
New or worsening conditions were reported in 11 major systems categories, as well as in the miscellaneous category (Table 2). The major systems included, in descending order of frequency, pain conditions (94.4% vs. 0%, p < 0.001), neurological conditions (92.4% vs. 15.4%, p < 0.001), sleep issues (82.8% vs. 5.3%, p < 0.001), skin issues (69.8% vs. 0%, p < 0.001), genitourinary issues (60.6% vs. 25.0%, p = 0.029), allergies/sensitivities/intolerances (31.8% vs. 10.0%, p < 0.001), mood disorders (31.6% vs. 7.5%, p = 0.001), gastrointestinal disorders (21.9% vs. 2.5%, p = 0.002), autoimmune issues (16.2% vs. 0%, p = 0.006), pulmonary disorders (15.8% vs. 0%, p = 0.002), and cardiac disorders (12.1% vs. 0%, p = 0.012) (Table 2). Presence of a new or worsening comorbidity following COVID-19 in LC patients that were found to be significant are shown in Table 3, adjusted for age and race. Female sex was associated with increased risk of neurologic manifestations and hypermobility in LC (Table 4; Supplementary material 2).
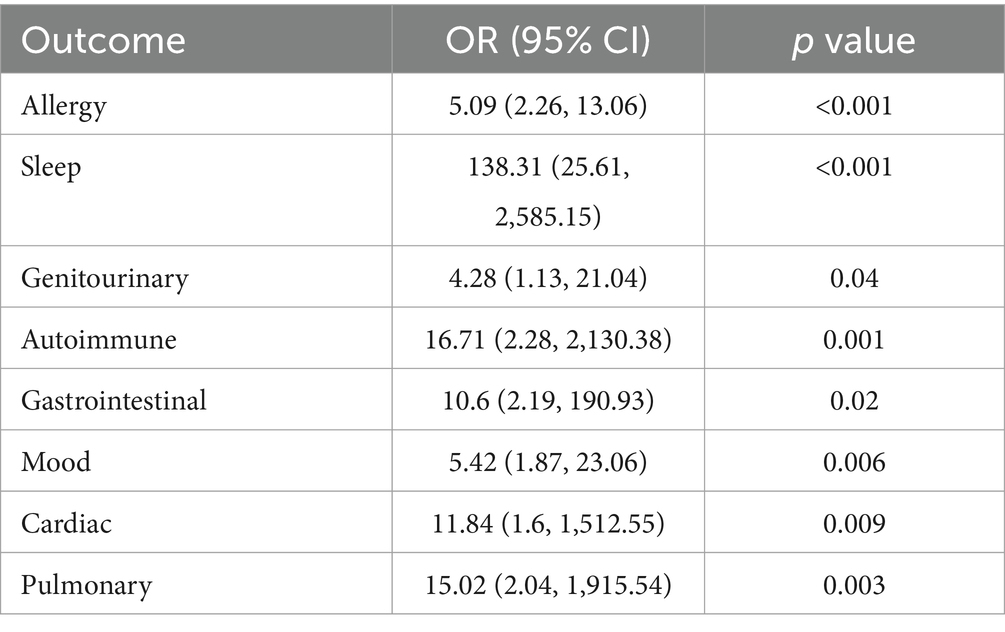
Table 3. Multivariable logistic regression model evaluating new or worsening comorbidities after COVID between Long COVID and controls, adjusted for age and race (White).
Within the miscellaneous category, participants were asked if conditions had been diagnosed or suspected by a clinician after COVID, with answer choices of yes, unknown/unsure, or no. Results of significance in LC vs. controls included mast cell issues (16.2% yes, 36.3% unsure vs. 2.4% yes, 5 unsure, p < 0.001), endothelial dysfunction (3.9% yes, 32.9% unsure vs. 0% yes or unsure, p < 0.001), viral reactivation (e.g., Epstein–Barr virus, herpesvirus, or other viruses) (18.7% yes, 17.8% unsure vs. 2.6% yes, 2.6% unsure, p < 0.001), vascular compression syndromes (3.6% yes, 8.5% unsure vs. 0% yes, 2.4% unsure, p = 0.039), and mitochondrial disease/dysfunction (5.2% yes, 23.9% unsure vs. 0% yes, 2.4% unsure, p < 0.001). The questionnaires also asked about sensitivity or resistance to medications. LC respondents were more likely to report sensitivity to medications than controls (22.5% vs. 4.9%, p = 0.007).
New or worsening diagnoses within the categories of dental, hematologic/oncologic, or endocrinologic disorders did not meet statistical significance in our survey.
3.3 Screened comorbidities
We screened patients for ME/CFS, GJH, and orthostatic intolerance. A majority of patients with LC reported ME/CFS symptoms (58.3%) compared to controls (0%) (p < 0.001) (Table 4). A similar proportion of patients with co-occurring LC and ME/CFS were female (59.7%) vs. male (53.3%) (p = 0.45) We found that around 27% of patients with LC reported GJH compared to only 10% of controls (p = 0.026) (Table 4). Most of the patients with co-occurring LC and GJH were female (90.5%) vs. male (9.5%) (p < 0.0001), and all controls with a positive screen were female (Table 4). LC patients reported orthostatic intolerance symptoms with a score of 4.0 after COVID compared to a score of 0 for controls (p < 0.001) (Table 2). Both males and females with LC had a score of 4.0 for orthostatic intolerance symptoms (p = 0.19).
4 Discussion
Our research demonstrates statistically significant reporting of new diagnoses or worsening of existing conditions following COVID-19 infection present across almost every organ system surveyed in LC compared to controls. Of the major systems and comorbidities that we examined in this study, ones that were new or worse in patients with LC vs. COVID controls were pain (94.4), neurological (92.4%), sleep (82.8%), skin (69.8%), genitourinary (60.6%), allergies/sensitivities/intolerances (31.8%), mood disorders (31.6%), sensitivity to medications (22.5%), gastrointestinal disorders (21.9%), viral reactivation (e.g., Epstein–Barr virus, herpesvirus, or other viruses) (18.7%), autoimmune diagnoses (16.2%), mast cell issues (16.2%), pulmonary disorders (15.8%), cardiac disorders (12.1%), and vascular compression syndromes (3.6%).
Notably, LC patients were more likely to screen positive for ME/CFS, orthostatic intolerance, or GJH than to report being formally diagnosed with one of these conditions. Almost 60% of our patients diagnosed with LC also met IOM/NAM ME/CFS 2015 criteria (41). This finding is consistent with other studies that reported 43–58% of patients with LC have ME/CFS (26–28). We also found that around 30% of LC patients had a positive screen for GJH according to the self-assessment 5-questions for hypermobility (42).
4.1 Comorbidities in LC and related conditions
Our study supports much of the current literature surrounding different comorbidities in LC, ME/CFS, orthostatic intolerance, hypermobile conditions, and related complex chronic disease. Having found that 30% of our LC respondents screened positive for GJH, our findings correspond to a recent UK study of 2,854 patients that also assessed GJH using the self-assessment 5-part hypermobility questionnaire that found that GJH was associated with a 30% increased risk of developing LC (46).
The most frequently reported symptoms in this study were pain and neurologic symptoms/conditions. The development of fatigue, chronic widespread pain, and muscle/joint pain are all common to LC, ME/CFS, fibromyalgia and hEDS/HSD (30, 47–49). New onset or worsening chronic pain has a prevalence of 45–70% across multiple studies of LC patients (50). A cross-sectional survey study of adults with LC in Bangladesh (n = 563) identified five types of commonly occurring pain in LC: muscle pain, chest pain, joint pain, headache, and abdominal pain, all of which were associated with a reduction in quality of life (51). Pre-existing chronic pain conditions are also risk factors for developing LC. In a retrospective EHR study of people with acute COVID (n = 1,038,402), having a chronic pain condition such as fibromyalgia, endometriosis, or irritable bowel syndrome increased the risk of developing LC by 1.47 (95% CI = 1.46, 1.47) (52). Our team also recently found that around 70% of patients seen at the Mayo Florida EDS Clinic are diagnosed with fibromyalgia, indicating high overlap between hEDS, HSD and fibromyalgia (39).
Multiple studies have found that a high percentage of LC patients also have ME/CFS (26–30). Craniocervical disease, Arnold Chiari malformations, idiopathic intracranial hypertension, and other spinal structural and flow issues have been associated with ME/CFS, fibromyalgia, and connective tissue disorders previously (35, 53–57). Additionally, an estimated 50–81% of people with ME/CFS have hypermobility (35, 58). Joint hypermobility disorders themselves are strongly associated with autonomic dysfunction, including chronic orthostatic intolerance and POTS in up to 48% of patients with hEDS/HSD, as well as headaches of various origin (59–64). Neurodivergent conditions including ADHD and autism also have a higher prevalence in people with joint hypermobility (43, 65, 66).
Orthostatic intolerance was frequent both with self-reporting and screening questions in LC. Previous studies have found that around 30–80% of LC patients fulfill the criteria for POTS (29, 67). Patients with LC can also have evidence of autonomic dysfunction including reduced cerebral blood flow and symptoms of orthostatic intolerance on a tilt table test without meeting the heart rate criteria for POTS (68). GJH, hEDS and HSD are strongly associated with autonomic dysfunction, with chronic orthostatic intolerance and POTS found in up to 48% of patients with hEDS/HSD (59–64).
Multiple studies have also found that sleep dysfunction is common in LC patients. A survey study of adults with LC at the Cleveland Clinic found that 58.7% of patients reported normal to mild sleep disturbances, and 41.3% reported moderate to severe sleep disturbances (69). In a separate retrospective survey of LC patients, 82.3% reported poor sleep quality (70). Almost half of subjects involved in a study assessing the effects of altered brain perfusion and oxygen levels in LC reported daytime sleepiness, differentiated from LC fatigue (71). Sleep disorders and dysfunction, including non-restorative sleep, are also prevalent in ME/CFS, fibromyalgia, and hEDS/HSD (41, 72–74).
Among patients with recent COVID-19, the frequently reported skin conditions include maculopapular rashes, urticarial lesions, and chilblains (75). LC patients report a range of skin conditions in clinic, however these are not well documented in the literature, thereby making our study one of the first to assess overlapping dermatologic involvement. Skin conditions are also present in hEDS and mast cell activation syndrome (MCAS). In hEDS, skin manifestations include soft skin, hematomas, atrophic scars, piezogenic pedal papules, and cutaneous stretchability (76). Skin manifestations of MCAS include flushing, pruritus, hives, and angioedema, and non-diagnostic skin manifestations include dermographism, urticaria, rashes, edema, alopecia, poor healing, and onychodystrophy (77). More research is needed on the causes, duration, and type of skin lesions in LC.
Our findings also align with research on genitourinary and reproductive health in LC. LC patients have an increased risk for several reproductive health conditions, many of which are also elevated in people with EDS, ME/CFS, or POTS (78). Women with endometriosis had an elevated risk for developing LC in a large EHR study of non-hospitalized LC patients (48). Similarly, multiple studies have found that endometriosis is elevated in women with EDS, ME/CFS, and POTS compared to general population prevalence (78, 79). Women with PCOS in a large population based cohort study had a 28% increased risk for COVID-19 infection after accounting for age and BMI, and PCOS is also elevated in ME/CFS and POTS (78, 80).
The development of autoimmune conditions was also more common after COVID for people diagnosed with LC compared to controls. As of June 2024, following this study’s period of data collection, the presence of autoimmune disorders are included in the definition of LC (4). Autoimmunity has been proposed to play a role in LC even without coexisting diagnosis of conditions that are already established as autoimmune, as suggested by basic science research (81–83). Additionally, two EHR studies of adult Korean and Japanese patients found that COVID-19 is associated with a significant risk for developing autoimmune and autoinflammatory connective tissue disorders (84, 85).
Finally, our study confirms that allergies, sensitivities, and intolerances are common in LC. Allergies and sensitivities to environmental and food antigens are common in ME/CFS, and are included in both the Canadian Consensus Criteria and International Consensus Criteria for diagnosis of ME/CFS (86, 87). In an online survey of Dutch (n = 337) and US (n = 252) ME/CFS patients, 19.1% of the Dutch cohort and 46.8% of the US cohort reported “sensitivity to smells, food, medications, or chemicals” (88). These new and worsening sensitivities and intolerances have been theorized to be due to mast cell hyperactivation including MCAS (89–91).
4.2 Emerging mechanisms
Given the widespread effects of LC on the body, it stands to reason that key underlying mechanisms behind this disease would have the ability to affect multiple organ systems simultaneously. While there is no consensus on the etiology of LC, many theories have emerged (13, 38, 92). Proposed underlying pathologies in addition to immune and neurologic dysregulation have included mast cell disease, endothelial dysfunction and other sequelae of vascular damage, viral reactivation, and mitochondrial impairment, all of which were self-reported as occurring more frequently in people with LC than controls in our study (7, 93, 94). Research groups are increasingly evaluating the role of these pathologies in the context of LC and as related to each other, as well as in other infection-associated chronic conditions (93, 95).
Female sex, and particularly pre-menopausal status, have been associated with LC and conditions of hypermobility, orthostatic intolerance, and ME/CFS (12, 51, 96–99). Low testosterone has also been found to be a risk factor for developing LC (1, 100). Variances in hormonal composition has therefore become an area of interest in understanding the development of infection-associated chronic illness. There are key hormonal as well as chromosomal and metabolic factors that significantly impact both innate and adaptive immune responses to infection, and while such differences can be protective in the context of an acute infection, they may provide a double-edged sword that increases risk for infection-associated chronic illness (101). This may also relate to why a majority of autoimmune diseases, such as Sjogren’s syndrome and systemic erythematous lupus, occur more frequently in females than males, given how autoimmune diseases can themselves be triggered by infections (102–107).
Viral and other microbial infections are strongly linked to the development of ME/CFS, with 70–80% of patients reporting having had a viral infection just prior to the development of symptoms (21–25). Viral infections and viral particles could lead to persistent activation of the innate immune response. Additionally, many viruses are known to localize to mitochondria and utilize mitochondrial machinery for viral replication including SARS-CoV-2, which could lead to mitochondrial dysfunction in numerous cell types and organs (94, 108–116). This corresponds to mitochondrial dysfunction being a hallmark pathology of ME/CFS, and likely LC (38, 93). Oxidative stress, reduced perfusion to tissue, and metabolic dysfunction are additional emerging pathologies that could conceivably contribute to extracellular membrane and connective tissue breakdown (91).
Nervous system involvement is prominent in LC, both as an affected system as well as a mechanism of symptom development. Autonomic dysfunction, observed at higher rates in LC, ME/CFS, and hEDS/HSD, and often manifesting as dysregulated heart rate and blood pressure control, directly contributes to the fatigue, exercise intolerance, and orthostatic symptoms seen in many patients (7, 29, 61, 68, 93, 117). Autoantibodies have also been found in the context of dysautonomia, adding to inflammatory aggravation (82). Small fiber neuropathy commonly coexists with hypermobility and autonomic disorders, contributing to dysthesias and thermodysregulation as well (61). Insular cortex hyperactivation has also been noted across hypermobility, neurodevelopmental conditions such as autism, and dysautonomia, and multiple brain signaling abnormalities have been described in ME/CFS including of the brainstem (93, 118, 119). Advanced neuroimaging has demonstrated altered neurochemical milieu and neuroinflammation in ME/CFS and LC (120–122). Central sensitization syndrome has been proposed as a potential theory behind neurologic symptomatology, but has fallen out of favor given its inability to account for major manifestations such as post-exertional malaise as well as the demonstrated presence of the aformentioned neurologic and immunologic contributors (7, 8, 50, 123, 124).
Hyperactivation of mast cells is a likely mechanism that may link LC, hEDS/HSD, ME/CFS, POTS, and multiple other co-occurring conditions (125–128). Mast cells are antigen presenting cells found throughout the body that activate in response to infections and toxins (129). Mast cells may be activated by SARS-CoV-2 because they express receptors for viral entry, including H1, ACE2, TMPRSS2, NRP1, integrins and cathepsins (126, 127, 130). They then promote proinflammatory T helper (Th)2 responses and Toll-like receptor (TLR)4 induced interleukin (IL)-1β, which has been found to be elevated in LC patients (131–134). A Th2-type immune environment allows viral infections to have an advantage and/or persist, because Th1-type immune responses are needed to clear viral infections (131, 135). Aberrant mast cell activation has also been implicated in exacerbation of autonomic symptoms (136). In particular, mast cells have been found to activate the vagus nerve by release of IL-1β and tumor necrosis factor (TNF) (137, 138). Mast cells are also involved in thromboembolic responses that mediate many poor outcomes in COVID-19 (126, 127). Mast cell hyperactivation is also observed in patients with hEDS/HSD where they respond to defective connective tissue and promote the breakdown of extracellular matrix/connective tissue. Importantly, mast cell numbers and function differ by sex and genetic background, which may partly explain the frequency of LC, ME/CFS, and hEDS/HSD in White females (127, 139, 140).
Finally, tissue laxity worsened by the presence of inflammation may contribute to structural and vascular conditions (53, 56). The inherent connective tissue abnormalities in hEDS/HSD and related conditions can lead to vascular and structural instability. This laxity may impair structural integrity of blood vessels, leading to venous pooling and subsequent orthostatic intolerance. Patients with hEDS and HSD frequently exhibit signs of altered baroreflex sensitivity and increased sympathetic activity, issues that can impair normal autonomic responses to postural and environmental changes (61, 63). Orthostatic flow dysfunction via stasis of venous flow and pressurization, with concomitant venous hypovolemia above the pelvis, has been hypothesized to play a role in patients with pelvic pain secondary to pelvic congestion syndromes (141–143). A newer phenotype along the idiopathic intracranial hypertension spectrum, cerebral venous outflow disorders, was recently described with a high co-occurrence in connective tissue disorders and especially EDS (57). Therefore, it is reasonable to consider the potential contribution of vascular and spinal compression syndromes in LC.
4.3 Future research
The multiple intertwined pathophysiologic processes in LC underscores the need for comprehensive research to unravel the precise mechanisms and develop targeted therapeutic strategies. Future research should also explore the frequency of coexisting conditions across the different topics surveyed here, including the order and timeline in which these typically develop, to see if certain diagnoses are more likely to create a risk of or co-develop within LC. Future research should further study mechanisms in LC and similar infection-associated chronic illnesses. Areas could include, but are not limited to, examining the role of breakdown of connective tissue in these illnesses; immune dysfunction (such as mast cell activation), autoimmunity, and chronic inflammation; metabolic and mitochondrial dysfunction; sex and hormonal differences; tissue hypoperfusion; nutrient and mineral depletion; and persistent infections. The true prevalence of the lesser studied pathologies should be better elucidated as well. While our study suggests that potentially critical pathologies such as vascular and endothelial dysfunction, mast cell activation, viral reactivation, and structural/outflow contributors are starting to be discussed with patients, it is likely that involvement of these pathologies are underestimated.
4.4 Clinical implications
A strength of our survey is that we simultaneously assessed for numerous potential coexisting conditions. Our findings bring to question the impact of potential underdiagnosis of associated conditions by clinicians. While there are no formal guidelines or approved treatments currently for management of LC, people living with LC may find symptom improvement through management correlating with correctly identified coexisting pathologies and conditions. Long COVID clinics also specializing in connective tissue disorders, ME/CFS, POTS, fibromyalgia, mast cell activation, and associated conditions have effectively applied similar principles to the appropriate LC patients (13, 144, 145).
For example, people with LC identified to have features of post-exertional malaise, often associated with ME/CFS, can benefit from applying a pacing activity management strategy (28, 146, 147). Other non-pharmacologic interventions beneficial for post-exertional malaise, sensory sensitivity, orthostatic intolerance, and other symptoms may also be easily trialed by patients if present (98, 145, 148). People with LC and commonly overlapping illnesses, such as ME/CFS and POTS, should be screened for connective tissue disorders and vice versa. Screening for hypermobile tendencies may open opportunities for management and reduction in joint instability and chronic pain, dysautonomia, and other symptoms.
Similarly, therapeutics employed in overlapping conditions may be harnessed for LC (149). Pharmacologic options utilized in POTS may be helpful in cases of orthostatic intolerance as well as those meeting POTS criteria, as can fibromyalgia medications for pain associated with LC (7, 13, 98). Mast cell therapies and agents that improve endothelial dysfunction have also been associated with symptom improvement in LC (7, 13, 150, 151). Low-dose naltrexone has been helpful in patients with LC, ME/CFS, fibromyalgia, POTS, autism, MCAS, hypermobility, and autoimmune disorders. The beneficial effects of LDN may be secondary to neuroimmune attenuating effects which may further suggest potential overlapping pathologies of these conditions (13, 152–160). Identifying conditions or syndromes with autoimmune associations that could follow COVID-19 infection can allow for informed trials of immunomodulatory therapy (144, 161–166). The role of antimicrobials in managing infection-associated chronic illness is under investigation (144). Identification of structural contributors that are amenable to intervention may alleviate symptoms in select cases (56, 141, 142, 167).
Clinicians suspecting LC should apply the current ICD-10 code, U09.9—Post COVID-19 condition along with the code M35.89 for “other specified systemic involvement of connective tissue” related to COVID-19 if applicable (168, 169). Accordingly, diagnostic codes for overlapping conditions as mentioned here, such as ME/CFS (G93.32) and OI/POTS (G90.A) should also be considered. Additional diagnostic codes are being developed for coexisting pathologies. Use of these codes can improve electronic capture, thereby allowing for more accurate research and management related to these groups.
4.5 Limitations
Our study has several limitations. Both study sites were part of a single tertiary/quaternary care center and as such the findings from this cohort of patients may not represent all patient populations. The disparity in representation is also evident in that 93.9% of LC respondents were White. Controls were based only in Minnesota while LC patients were seen at both Minnesota and Florida sites and could be from any location, and there were no responses from non-White individuals for the control group. Furthermore, the control group utilized for this study was limited in number and, consequently, the study had uneven sample sizes. It is possible that severe symptoms affected the ability of some LC patients to participate in the call for survey responses. All participants in this study were at least 3 months from a COVID infection; however, the specific length of LC and whether the patient had multiple infections were not recorded. Although our survey population was aged similar to other studies at an average of 52 years, this could lead to a lower percentage of GJH being reported as GJH reduces dramatically post-menopause. The primarily female sample reflects the female predominance in infection-associated chronic illness groups, however a larger, more balanced sample would be better powered to assess sex differences within systems.
This is a survey-based study which is inherently limited by the recollection of participants regarding symptoms and diagnoses. It is important to acknowledge however that a number of the conditions evaluated in this survey are also only recently being recognized by many infection-associated chronic illness experts, and as such likely underdiagnosed even in our study population. As such, respondents who were evaluated more recently may have been more likely to receive diagnoses based on growing knowledge and recognition of certain comorbidities, compared to those evaluated much earlier in the rise of LC. The screening instruments incorporated into the survey are also insufficient to independently establish a formal diagnosis. Although inclusion of EHR data may have offered additional information, EHR data are especially fallible in the context of new and emerging diseases that may not have associated ICD coding yet.
5 Conclusion
In this study, we identified that a number of symptoms and comorbidities are new or worse following SARS-CoV-2 infection in patients diagnosed with LC compared to controls. The LC patients in this study also had similar co-occurrence of ME/CFS, GJH and orthostatic intolerance as other studies. Our paper provides a foundation to examine these relationships further. These findings highlight the presence of multiple underrecognized conditions in the context of LC, especially those involving autonomic dysfunction, connective tissue compromise and immune dysregulation. As the prevalence of different pathologies and conditions is better characterized in LC, research can further elucidate mechanisms that offer the potential for more effective treatments.
Software
Study data were collected and managed using REDCap electronic data capture tools hosted at Mayo Clinic. 1, 2 REDCap (Research Electronic Data Capture) is a secure, web-based software platform designed to support data capture for research studies, providing (1) an intuitive interface for validated data capture; (2) audit trails for tracking data manipulation and export procedures; (3) automated export procedures for seamless data downloads to common statistical packages; and (4) procedures for data integration and interoperability with external sources (170, 171).
Data availability statement
The datasets presented in this article are not readily available because requests for raw data must be approved by the Mayo Clinic IRB and require a data transfer agreement by standard protocol. Requests to access the datasets should be directed to Stephanie Grach, Z3JhY2guc3RlcGhhbmllQG1heW8uZWR1.
Ethics statement
The studies involving humans were approved by the Mayo Clinic Institutional Review Board (IRB# 23-010442). The studies were conducted in accordance with the local legislation and institutional requirements. The Ethics Committee/Institutional Review Board waived the requirement of written informed consent for participation from the participants or the participants’ legal guardians/next of kin because the survey did not include identifiable health information.
Author contributions
SG: Visualization, Project administration, Methodology, Investigation, Data curation, Conceptualization, Writing – review & editing, Writing – original draft. DD: Writing – review & editing, Writing – original draft, Methodology, Funding acquisition. BP: Writing – review & editing, Writing – original draft. DF: Writing – review & editing, Visualization, Resources, Formal analysis. CA: Writing – review & editing, Methodology. BM: Writing – review & editing, Resources. IC: Writing – review & editing, Resources. MM: Conceptualization, Writing – review & editing. JO: Writing – review & editing. KB: Writing – review & editing, Resources. NC: Writing – review & editing. ZL: Writing – review & editing, Formal analysis. RH: Writing – review & editing, Supervision, Resources, Methodology. MT: Writing – review & editing, Supervision. RG: Writing – review & editing, Supervision, Resources, Methodology. DK: Writing – review & editing, Writing – original draft, Methodology, Conceptualization.
Funding
The author(s) declare that financial support was received for the research, authorship, and/or publication of this article. The statistical analysis for this study was supported by the UL1TR002377 internal grant to DVD. Components of this study were additionally supported by funding from the Mayo Clinic RACER Award to DRTK; Mayo Clinic STARDOM Award to DF and DRTK; Mayo Clinic’s Division of General Internal Medicine to DRTK, KAB, DF; the Ralph E. Pounds and Kathy Olesker Pounds Fund in Research Related to Chronic Pain to DF; American Heart Association 20TPA35490415 to DF; National Institutes of Health R01 HL164520, R21 AI154927, R21 AI152318, R21 AI145356 to DF, and R21 AI163302, R21 AI180863-91A1, AHA 23SCEFIA1153413, and DoD CDMRP PR210385 to KAB. Publication costs funded by Mayo Clinic Department of Medicine, Division of General Internal Medicine.
Conflict of interest
RTH is a consultant for Nestle Nutrition.
The remaining authors declare that the research was conducted in the absence of any commercial or financial relationships that could be construed as a potential conflict of interest.
The author(s) declared that they were an editorial board member of Frontiers, at the time of submission. This had no impact on the peer review process and the final decision.
Publisher’s note
All claims expressed in this article are solely those of the authors and do not necessarily represent those of their affiliated organizations, or those of the publisher, the editors and the reviewers. Any product that may be evaluated in this article, or claim that may be made by its manufacturer, is not guaranteed or endorsed by the publisher.
Supplementary material
The Supplementary material for this article can be found online at: https://www.frontiersin.org/articles/10.3389/fneur.2024.1482917/full#supplementary-material
References
1. Sigfrid, L, Drake, TM, Pauley, E, Jesudason, EC, Olliaro, P, Lim, WS, et al. Long COVID in adults discharged from UK hospitals after COVID-19: a prospective, multicentre cohort study using the ISARIC WHO clinical characterisation protocol. Lancet Reg Health Eur. (2021) 8:100186. doi: 10.1016/j.lanepe.2021.100186
2. Logue, JK, Franko, NM, McCulloch, DJ, McDonald, D, Magedson, A, Wolf, CR, et al. Sequelae in adults at 6 months after COVID-19 infection. JAMA Netw Open. (2021) 4:e210830. doi: 10.1001/jamanetworkopen.2021.0830
3. (ASPA) ASfPA. Terms & definitions. COVID.gov (2023). Available at: https://www.covid.gov/be-informed/longcovid/about (Accessed August 18, 2024).
4. National Academies of Sciences E, Medicine In: HV Fineberg, L Brown, T Worku, and I Goldowitz, editors. A long COVID definition: a chronic, systemic disease state with profound consequences. Washington, DC: The National Academies Press (2024). 186.
5. Bureau NCfHSUSC. Household pulse survey, 2022–2024. Long COVID. Available at: https://www.cdc.gov/nchs/covid19/pulse/long-covid.htm (Accessed August 18, 2024).
6. Mirin, AA. A preliminary estimate of the economic impact of long COVID in the United States. Fatigue: Biomed, Health Behav. (2022) 10:190–9. doi: 10.1080/21641846.2022.2124064
7. Davis, HE, McCorkell, L, Vogel, JM, and Topol, EJ. Long COVID: major findings, mechanisms and recommendations. Nat Rev Microbiol. (2023) 21:133–46. doi: 10.1038/s41579-022-00846-2
8. Ganesh, R, Grach, SL, Ghosh, AK, Bierle, DM, Salonen, BR, Collins, NM, et al. The female-predominant persistent immune dysregulation of the post-COVID syndrome. Mayo Clin Proc. (2022) 97:454–64. doi: 10.1016/j.mayocp.2021.11.033
9. Munipalli, B, Seim, L, Dawson, NL, Knight, D, and Dabrh, AMA. Post-acute sequelae of COVID-19 (PASC): a meta-narrative review of pathophysiology, prevalence, and management. SN Compr Clin Med. (2022) 4:90. doi: 10.1007/s42399-022-01167-4
10. Zhang, H, Zang, C, Xu, Z, Zhang, Y, Xu, J, Bian, J, et al. Data-driven identification of post-acute SARS-CoV-2 infection subphenotypes. Nat Med. (2023) 29:226–35. doi: 10.1038/s41591-022-02116-3
11. Sudre, CH, Murray, B, Varsavsky, T, Graham, MS, Penfold, RS, Bowyer, RC, et al. Attributes and predictors of long COVID. Nat Med. (2021) 27:626–31. doi: 10.1038/s41591-021-01292-y
12. Sylvester, SV, Rusu, R, Chan, B, Bellows, M, O'Keefe, C, and Nicholson, S. Sex differences in sequelae from COVID-19 infection and in long COVID syndrome: a review. Curr Med Res Opin. (2022) 38:1391–9. doi: 10.1080/03007995.2022.2081454
13. Hurt, RT, Yadav, S, Schroeder, DR, Croghan, IT, Mueller, MR, Grach, SL, et al. Longitudinal progression of patients with long COVID treated in a post-COVID clinic: a cross-sectional survey. J Prim Care Community Health. (2024) 15:21501319241258671. doi: 10.1177/21501319241258671
14. Grut, V, Biström, M, Salzer, J, Stridh, P, Jons, D, Gustafsson, R, et al. Interactions between high seroreactivity to human herpes virus 6A and Epstein-Barr virus in MS development: a presymptomatic case-control study. Ann Neurol. (2024) 96:302–5. doi: 10.1002/ana.27009
15. Smatti, MK, Cyprian, FS, Nasrallah, GK, Al Thani, AA, Almishal, RO, and Yassine, HM. Viruses and autoimmunity: a review on the potential interaction and molecular mechanisms. Viruses. (2019) 11:1–18. doi: 10.3390/v11080762
16. Azadeh, N, Limper, AH, Carmona, EM, and Ryu, JH. The role of infection in interstitial lung diseases: a review. Chest. (2017) 152:842–52. doi: 10.1016/j.chest.2017.03.033
17. Vesco, KK, Whitlock, EP, Eder, M, Lin, J, Burda, BU, Senger, CA, et al. U.S. preventive services task force evidence syntheses, formerly systematic evidence reviews. Screening for cervical cancer: a systematic evidence review for the US preventive services task force. Rockville (MD): Agency for Healthcare Research and Quality (US) (2011).
18. Jang, H, Boltz, DA, Webster, RG, and Smeyne, RJ. Viral parkinsonism. Biochim Biophys Acta. (2009) 1792:714–21. doi: 10.1016/j.bbadis.2008.08.001
19. Cairns, DM, Itzhaki, RF, and Kaplan, DL. Potential involvement of varicella zoster virus in Alzheimer's disease via reactivation of quiescent herpes simplex virus type 1. J Alzheimers Dis. (2022) 88:1189–200. doi: 10.3233/JAD-220287
20. Sipilä, PN, Heikkilä, N, Lindbohm, JV, Hakulinen, C, Vahtera, J, Elovainio, M, et al. Hospital-treated infectious diseases and the risk of dementia: a large, multicohort, observational study with a replication cohort. Lancet Infect Dis. (2021) 21:1557–67. doi: 10.1016/S1473-3099(21)00144-4
21. Hickie, I, Davenport, T, Wakefield, D, Vollmer-Conna, U, Cameron, B, Vernon, SD, et al. Post-infective and chronic fatigue syndromes precipitated by viral and non-viral pathogens: prospective cohort study. BMJ. (2006) 333:575. doi: 10.1136/bmj.38933.585764.AE
22. Cameron, B, Flamand, L, Juwana, H, Middeldorp, J, Naing, Z, Rawlinson, W, et al. Serological and virological investigation of the role of the herpesviruses EBV, CMV and HHV-6 in post-infective fatigue syndrome. J Med Virol. (2010) 82:1684–8. doi: 10.1002/jmv.21873
23. Schreiner, P, Harrer, T, Scheibenbogen, C, Lamer, S, Schlosser, A, Naviaux, RK, et al. Human Herpesvirus-6 reactivation, mitochondrial fragmentation, and the coordination of antiviral and metabolic phenotypes in Myalgic encephalomyelitis/chronic fatigue syndrome. Immunohorizons. (2020) 4:201–15. doi: 10.4049/immunohorizons.2000006
24. Rasa, S, Nora-Krukle, Z, Henning, N, Eliassen, E, Shikova, E, Harrer, T, et al. Chronic viral infections in myalgic encephalomyelitis/chronic fatigue syndrome (ME/CFS). J Transl Med. (2018) 16:268. doi: 10.1186/s12967-018-1644-y
25. Apostolou, E, and Rosén, A. Epigenetic reprograming in myalgic encephalomyelitis/chronic fatigue syndrome: a narrative of latent viruses. J Intern Med. (2024) 296:93–115. doi: 10.1111/joim.13792
26. Bonilla, H, Quach, TC, Tiwari, A, Bonilla, AE, Miglis, M, Yang, PC, et al. Myalgic encephalomyelitis/chronic fatigue syndrome is common in post-acute sequelae of SARS-CoV-2 infection (PASC): results from a post-COVID-19 multidisciplinary clinic. Front Neurol. (2023) 14:1090747. doi: 10.3389/fneur.2023.1090747
27. Legler, F, Meyer-Arndt, L, Mödl, L, Kedor, C, Freitag, H, Stein, E, et al. Long-term symptom severity and clinical biomarkers in post-COVID-19/chronic fatigue syndrome: results from a prospective observational cohort. EClinicalMedicine. (2023) 63:102146. doi: 10.1016/j.eclinm.2023.102146
28. Jason, LA, and Dorri, JA. ME/CFS and post-exertional malaise among patients with long COVID. Neurol Int. (2022) 15:1–11. doi: 10.3390/neurolint15010001
29. Seeley, MC, Gallagher, C, Ong, E, Langdon, A, Chieng, J, Bailey, D, et al. High incidence of autonomic dysfunction and postural orthostatic tachycardia syndrome in patients with long COVID: implications for management and health care planning. Am J Med. (2023). doi: 10.1016/j.amjmed.2023.06.010
30. Ciaffi, J, Vanni, E, Mancarella, L, Brusi, V, Lisi, L, Pignatti, F, et al. Post-acute COVID-19 joint pain and new onset of rheumatic musculoskeletal diseases: a systematic review. Diagnostics (Basel). (2023) 63:259–262. doi: 10.3390/diagnostics13111850
31. van Campen, C, Rowe, PC, and Visser, FC. Low sensitivity of abbreviated tilt table testing for diagnosing postural tachycardia syndrome in adults with ME/CFS. Front Pediatr. (2018) 6:349. doi: 10.3389/fped.2018.00349
32. Ramírez-Morales, R, Bermúdez-Benítez, E, Martínez-Martínez, LA, and Martínez-Lavín, M. Clinical overlap between fibromyalgia and myalgic encephalomyelitis. A systematic review and meta-analysis. Autoimmun Rev. (2022) 21:103129. doi: 10.1016/j.autrev.2022.103129
33. Brock, I, Chopra, P, Maitland, A, and Francomano, C. eP305 - frequency and co-occurrence of comorbidities in the Ehlers-Danlos syndromes. Mol Genet Metab. (2021) 132:S194. doi: 10.1016/S1096-7192(21)00387-5
34. Miller, AJ, Stiles, LE, Sheehan, T, Bascom, R, Levy, HP, Francomano, CA, et al. Prevalence of hypermobile Ehlers-Danlos syndrome in postural orthostatic tachycardia syndrome. Auton Neurosci. (2020) 224:102637. doi: 10.1016/j.autneu.2020.102637
35. Bragée, B, Michos, A, Drum, B, Fahlgren, M, Szulkin, R, and Bertilson, BC. Signs of intracranial hypertension, hypermobility, and craniocervical obstructions in patients with myalgic encephalomyelitis/chronic fatigue syndrome. Front Neurol. (2020) 11:828. doi: 10.3389/fneur.2020.00828
36. Mudie, K, Ramiller, A, Whittaker, S, and Phillips, LE. Do people with ME/CFS and joint hypermobility represent a disease subgroup? An analysis using registry data. Front Neurol. (2024) 15:1324879. doi: 10.3389/fneur.2024.1324879
37. Logarbo, BP, Yang, M, Longo, MT, Kingry, C, and Courseault, J. Long COVID and the diagnosis of underlying hypermobile Ehlers-Danlos syndrome and hypermobility spectrum disorders. PM R. (2023) 16:935–7. doi: 10.1002/pmrj.13120
38. Komaroff, AL, and Lipkin, WI. Insights from myalgic encephalomyelitis/chronic fatigue syndrome may help unravel the pathogenesis of postacute COVID-19 syndrome. Trends Mol Med. (2021) 27:895–906. doi: 10.1016/j.molmed.2021.06.002
39. Fairweather, D, Bruno, KA, Darakjian, AA, Bruce, BK, Gehin, JM, Kotha, A, et al. High overlap in patients diagnosed with hypermobile Ehlers-Danlos syndrome or hypermobile spectrum disorders with fibromyalgia and 40 self-reported symptoms and comorbidities. Front Med (Lausanne). (2023) 10:1096180. doi: 10.3389/fmed.2023.1096180
40. Knight, DRT, Bruno, KA, Singh, A, Munipalli, B, Gajarawala, S, Solomon, M, et al. Cardiac defects of hypermobile Ehlers-Danlos syndrome and hypermobility spectrum disorders: a retrospective cohort study. Front Cardiovasc Med. (2024) 11:1332508. doi: 10.3389/fcvm.2024.1332508
41. Committee on the Diagnostic Criteria for Myalgic Encephalomyelitis/Chronic Fatigue S, Board on the Health of Select P, Institute of M. The National Academies Collection: reports funded by National Institutes of Health. Beyond myalgic encephalomyelitis/chronic fatigue syndrome: Redefining an illness. Washington (DC): National Academies Press (US) (2015).
42. Hakim, AJ, and Grahame, R. A simple questionnaire to detect hypermobility: an adjunct to the assessment of patients with diffuse musculoskeletal pain. Int J Clin Pract. (2003) 57:163–6. doi: 10.1111/j.1742-1241.2003.tb10455.x
43. Glans, MR, Thelin, N, Humble, MB, Elwin, M, and Bejerot, S. The relationship between generalised joint hypermobility and autism Spectrum disorder in adults: a large, cross-sectional, case control comparison. Front Psychiatry. (2021) 12:803334. doi: 10.3389/fpsyt.2021.803334
44. Malfait, F, Francomano, C, Byers, P, Belmont, J, Berglund, B, Black, J, et al. The 2017 international classification of the Ehlers-Danlos syndromes. Am J Med Genet C Semin Med Genet. (2017) 175:8–26. doi: 10.1002/ajmg.c.31552
45. Sletten, DM, Suarez, GA, Low, PA, Mandrekar, J, and Singer, W. COMPASS 31: a refined and abbreviated composite autonomic symptom score. Mayo Clin Proc. (2012) 87:1196–201. doi: 10.1016/j.mayocp.2012.10.013
46. Eccles, JA, Dorina, C, Quadt, L, Hakim, AJ, Gall, N, Bowyer, V, et al. Is joint hypermobility linked to self-reported non-recovery from COVID-19? Case control evidence from the British COVID symptom study biobank. BMJ Public Health. (2024) 2:e000478. doi: 10.1136/bmjph-2023-000478
47. Aiyegbusi, OL, Hughes, SE, Turner, G, Rivera, SC, McMullan, C, Chandan, JS, et al. Symptoms, complications and management of long COVID: a review. J R Soc Med. (2021) 114:428–42. doi: 10.1177/01410768211032850
48. Subramanian, A, Nirantharakumar, K, Hughes, S, Myles, P, Williams, T, Gokhale, KM, et al. Symptoms and risk factors for long COVID in non-hospitalized adults. Nat Med. (2022) 28:1706–14. doi: 10.1038/s41591-022-01909-w
49. Gavrilova, N, Soprun, L, Lukashenko, M, Ryabkova, V, Fedotkina, TV, Churilov, LP, et al. New clinical phenotype of the post-Covid syndrome: fibromyalgia and joint hypermobility condition. Pathophysiology. (2022) 29:24–9. doi: 10.3390/pathophysiology29010003
50. Calvache-Mateo, A, Navas-Otero, A, Heredia-Ciuró, A, Matín-Núñez, J, Torres-Sánchez, I, López-López, L, et al. Post-COVID patients with new-onset chronic pain 2 years after infection: cross-sectional study. Pain Manag Nurs. (2023) 24:528–34. doi: 10.1016/j.pmn.2023.04.010
51. Kabir, MF, Yin, KN, Jeffree, MS, Ahmedy, FB, Zainudin, MF, Htwe, O, et al. Clinical presentation of post-COVID pain and its impact on quality of life in long COVID patients: a cross-sectional household survey of SARS-CoV-2 cases in Bangladesh. BMC Infect Dis. (2024) 24:375. doi: 10.1186/s12879-024-09267-3
52. Bergmans, RS, Clauw, DJ, Flint, C, Harris, H, Lederman, S, and Schrepf, A. Chronic overlapping pain conditions increase the risk of long COVID features, regardless of acute COVID status. Pain. (2024) 165:1112–20. doi: 10.1097/j.pain.0000000000003110
53. Edwards, CC 3rd, Edwards, CC 2nd, Heinlein, S, and Rowe, PC. Case report: recurrent cervical spinal stenosis masquerading as myalgic encephalomyelitis/chronic fatigue syndrome with orthostatic intolerance. Front Neurol. (2023) 14:1284062. doi: 10.3389/fneur.2023.1284062
54. Holman, AJ. Positional cervical spinal cord compression and fibromyalgia: a novel comorbidity with important diagnostic and treatment implications. J Pain. (2008) 9:613–22. doi: 10.1016/j.jpain.2008.01.339
55. Heffez, DS, Ross, RE, Shade-Zeldow, Y, Kostas, K, Shah, S, Gottschalk, R, et al. Clinical evidence for cervical myelopathy due to Chiari malformation and spinal stenosis in a non-randomized group of patients with the diagnosis of fibromyalgia. Eur Spine J. (2004) 13:516–23. doi: 10.1007/s00586-004-0672-x
56. Henderson, FC Sr, Rosenbaum, R, Narayanan, M, Koby, M, Tuchman, K, Rowe, PC, et al. Atlanto-axial rotary instability (fielding type 1): characteristic clinical and radiological findings, and treatment outcomes following alignment, fusion, and stabilization. Neurosurg Rev. (2021) 44:1553–68. doi: 10.1007/s10143-020-01345-9
57. Midtlien, JP, Curry, BP, Chang, E, Kiritsis, NR, Aldridge, JB, and Fargen, KM. Characterizing a new clinical phenotype: the co-existence of cerebral venous outflow and connective tissue disorders. Front Neurol. (2023) 14:1305972. doi: 10.3389/fneur.2023.1305972
58. Eccles, JA, Thompson, B, Themelis, K, Amato, ML, Stocks, R, Pound, A, et al. Beyond bones: the relevance of variants of connective tissue (hypermobility) to fibromyalgia, ME/CFS and controversies surrounding diagnostic classification: an observational study. Clin Med (Lond). (2021) 21:53–8. doi: 10.7861/clinmed.2020-0743
59. Roma, M, Marden, CL, De Wandele, I, Francomano, CA, and Rowe, PC. Postural tachycardia syndrome and other forms of orthostatic intolerance in Ehlers-Danlos syndrome. Auton Neurosci. (2018) 215:89–96. doi: 10.1016/j.autneu.2018.02.006
60. Gazit, Y, Nahir, AM, Grahame, R, and Jacob, G. Dysautonomia in the joint hypermobility syndrome. Am J Med. (2003) 115:33–40. doi: 10.1016/S0002-9343(03)00235-3
61. De Wandele, I, Calders, P, Peersman, W, Rimbaut, S, De Backer, T, Malfait, F, et al. Autonomic symptom burden in the hypermobility type of Ehlers-Danlos syndrome: a comparative study with two other EDS types, fibromyalgia, and healthy controls. Semin Arthritis Rheum. (2014) 44:353–61. doi: 10.1016/j.semarthrit.2014.05.013
62. Wallman, D, Weinberg, J, and Hohler, AD. Ehlers-Danlos syndrome and postural tachycardia syndrome: a relationship study. J Neurol Sci. (2014) 340:99–102. doi: 10.1016/j.jns.2014.03.002
63. Celletti, C, Borsellino, B, Castori, M, Censi, F, Calcagnini, G, Camerota, F, et al. A new insight on postural tachycardia syndrome in 102 adults with hypermobile Ehlers-Danlos syndrome/hypermobility spectrum disorder. Monaldi Arch Chest Dis. (2020) 90:259–262. doi: 10.4081/monaldi.2020.1286
64. Fermo, OP. Evaluation and management of orthostatic headache in hypermobility disorders. Front Neurol. (2023) 14:1321350. doi: 10.3389/fneur.2023.1321350
65. Baeza-Velasco, C. Neurodevelopmental atypisms in the context of joint hypermobility, hypermobility spectrum disorders, and Ehlers-Danlos syndromes. Am J Med Genet C Semin Med Genet. (2021) 187:491–9. doi: 10.1002/ajmg.c.31946
66. Kindgren, E, Quiñones Perez, A, and Knez, R. Prevalence of ADHD and autism spectrum disorder in children with hypermobility spectrum disorders or hypermobile Ehlers-Danlos syndrome: a retrospective study. Neuropsychiatr Dis Treat. (2021) 17:379–88. doi: 10.2147/NDT.S290494
67. Vernon, SD, Funk, S, Bateman, L, Stoddard, GJ, Hammer, S, Sullivan, K, et al. Orthostatic challenge causes distinctive symptomatic, hemodynamic and cognitive responses in long COVID and Myalgic encephalomyelitis/chronic fatigue syndrome. Front Med (Lausanne). (2022) 9:917019. doi: 10.3389/fmed.2022.917019
68. Campen, C, and Visser, FC. Long-haul COVID patients: prevalence of POTS are reduced but cerebral blood flow abnormalities remain abnormal with longer disease duration. Healthcare (Basel). (2022) 10:1–9. doi: 10.3390/healthcare10102105
69. Pena-Orbea, C, Lapin, B, Li, Y, Englund, K, Heinzinger, C, Foldvary-Schaefer, N, et al. Sleep disturbance severity and correlates in post-acute sequelae of COVID-19 (PASC). J Gen Intern Med. (2023) 38:2015–7. doi: 10.1007/s11606-023-08187-3
70. Nowakowski, S, Kokonda, M, Sultana, R, Duong, BB, Nagy, SE, Zaidan, MF, et al. Association between sleep quality and mental health among patients at a post-COVID-19 recovery clinic. Brain Sci. (2022) 12:1–11. doi: 10.3390/brainsci12050586
71. Chien, C, Heine, J, Khalil, A, Schlenker, L, Hartung, TJ, Boesl, F, et al. Altered brain perfusion and oxygen levels relate to sleepiness and attention in post-COVID syndrome. Ann Clin Transl Neurol. (2024) 11:2016–29. doi: 10.1002/acn3.52121
72. Ferré, A. Chronic fatigue syndrome and sleep disorders: clinical associations and diagnostic difficulties. Neurologia (Engl Ed). (2016) doi: 10.1016/j.nrl.2015.11.019
73. Wolfe, F, Clauw, DJ, Fitzcharles, MA, Goldenberg, DL, Häuser, W, Katz, RL, et al. 2016 revisions to the 2010/2011 fibromyalgia diagnostic criteria. Semin Arthritis Rheum. (2016) 46:319–29. doi: 10.1016/j.semarthrit.2016.08.012
74. Verbraecken, J, Declerck, A, Van de Heyning, P, De Backer, W, and Wouters, EF. Evaluation for sleep apnea in patients with Ehlers-Danlos syndrome and Marfan: a questionnaire study. Clin Genet. (2001) 60:360–5. doi: 10.1034/j.1399-0004.2001.600507.x
75. Ică, OM, Mitroi, G, Ianoşi, SL, Tutunaru, CV, Leru, PM, Matei, D, et al. Defining the short-term and long-term skin manifestations of COVID-19: insights after more than three years of the pandemic. Romanian J Morphol Embryol. (2023) 64:291–304. doi: 10.47162/RJME.64.3.01
76. Edimo, CO, Wajsberg, JR, Wong, S, Nahmias, ZP, and Riley, BA. The dermatological aspects of hEDS in women. Int J Womens Dermatol. (2021) 7:285–9. doi: 10.1016/j.ijwd.2021.01.020
77. Mihele, DM, Nistor, PA, Bruma, G, Mitran, CI, Mitran, MI, Condrat, CE, et al. Mast cell activation syndrome update-a dermatological perspective. J Pers Med. (2023) 13:1–21. doi: 10.3390/jpm13071116
78. Pollack, B, von Saltza, E, McCorkell, L, Santos, L, Hultman, A, Cohen, AK, et al. Female reproductive health impacts of long COVID and associated illnesses including ME/CFS, POTS, and connective tissue disorders: a literature review. Front Rehabil Sci. (2023) 4:1122673. doi: 10.3389/fresc.2023.1122673
79. McIntosh, LJ, Mallett, VT, Frahm, JD, Richardson, DA, and Evans, MI. Gynecologic disorders in women with Ehlers-Danlos syndrome. J Soc Gynecol Investig. (1995) 2:559–64. doi: 10.1177/107155769500200309
80. Subramanian, A, Anand, A, Adderley, NJ, Okoth, K, Toulis, KA, Gokhale, K, et al. Increased COVID-19 infections in women with polycystic ovary syndrome: a population-based study. Eur J Endocrinol. (2021) 184:637–45. doi: 10.1530/EJE-20-1163
81. Chen, H-J, Appelman, B, Willemen, H, Bos, A, Prado, J, Geyer, CE, et al. Transfer of IgG from long COVID patients induces symptomology in mice. bioRxiv. (2024) doi: 10.1101/2024.05.30.596590
82. El-Rhermoul, FZ, Fedorowski, A, Eardley, P, Taraborrelli, P, Panagopoulos, D, Sutton, R, et al. Autoimmunity in long Covid and POTS. Oxf Open Immunol. (2023) 4:1–9. doi: 10.1093/oxfimm/iqad002
83. Santos Guedes de Sa, K, Silva, J, Bayarri-Olmos, R, Brinda, R, Alec Rath Constable, R, Colom Diaz, PA, et al. A causal link between autoantibodies and neurological symptoms in long COVID. medRxiv. (2024) doi: 10.1101/2024.06.18.24309100
84. Lim, SH, Ju, HJ, Han, JH, Lee, JH, Lee, WS, Bae, JM, et al. Autoimmune and autoinflammatory connective tissue disorders following COVID-19. JAMA Netw Open. (2023) 6:e2336120. doi: 10.1001/jamanetworkopen.2023.36120
85. Kim, MS, Lee, H, Lee, SW, Kwon, R, Rhee, SY, Lee, JA, et al. Long-term autoimmune inflammatory rheumatic outcomes of COVID-19: a binational cohort study. Ann Intern Med. (2024) 177:291–302. doi: 10.7326/M23-1831
86. Carruthers, BM, Jain, AK, De Meirleir, KL, Peterson, DL, Klimas, NG, Lerner, AM, et al. Myalgic encephalomyelitis/chronic fatigue syndrome. J Chronic Fatigue Syndr. (2003) 11:7–115. doi: 10.1300/J092v11n01_02
87. Carruthers, BM, van de Sande, MI, De Meirleir, KL, Klimas, NG, Broderick, G, Mitchell, T, et al. Myalgic encephalomyelitis: international consensus criteria. J Intern Med. (2011) 270:327–38. doi: 10.1111/j.1365-2796.2011.02428.x
88. Vaes, AW, Van Herck, M, Deng, Q, Delbressine, JM, Jason, LA, and Spruit, MA. Symptom-based clusters in people with ME/CFS: an illustration of clinical variety in a cross-sectional cohort. J Transl Med. (2023) 21:112. doi: 10.1186/s12967-023-03946-6
89. Palmer, RF, Dempsey, TT, and Afrin, LB. Chemical intolerance and mast cell activation: a suspicious synchronicity. J Xenobiot. (2023) 13:704–18. doi: 10.3390/jox13040045
90. Weinstock, LB, Brook, JB, Walters, AS, Goris, A, Afrin, LB, and Molderings, GJ. Mast cell activation symptoms are prevalent in long-COVID. Int J Infect Dis. (2021) 112:217–26. doi: 10.1016/j.ijid.2021.09.043
91. Afrin, LB, Weinstock, LB, and Molderings, GJ. COVID-19 hyperinflammation and post-COVID-19 illness may be rooted in mast cell activation syndrome. Int J Infect Dis. (2020) 100:327–32. doi: 10.1016/j.ijid.2020.09.016
92. Couzin-Frankel, J. Clues to long COVID. Science. (2022) 376:1261–5. doi: 10.1126/science.add4297
93. Arron, HE, Marsh, BD, Kell, DB, Khan, MA, Jaeger, BR, and Pretorius, E. Myalgic encephalomyelitis/chronic fatigue syndrome: the biology of a neglected disease. Front Immunol. (2024) 15:1386607. doi: 10.3389/fimmu.2024.1386607
94. Clough, E, Inigo, J, Chandra, D, Chaves, L, Reynolds, JL, Aalinkeel, R, et al. Mitochondrial dynamics in SARS-COV2 spike protein treated human microglia: implications for neuro-COVID. J Neuroimmune Pharmacol. (2021) 16:770–84. doi: 10.1007/s11481-021-10015-6
95. Bateman, L, and Hanson, M In: NINDS, editor. Report of the ME/CFS research roadmap working group of council (2024) Available at: https://www.ninds.nih.gov/about-ninds/who-we-are/advisory-council/nandsc-mecfs-research-roadmap-working-group
96. Castori, M, Camerota, F, Celletti, C, Grammatico, P, and Padua, L. Ehlers-Danlos syndrome hypermobility type and the excess of affected females: possible mechanisms and perspectives. Am J Med Genet A. (2010) 152A:2406–8. doi: 10.1002/ajmg.a.33585
97. Malfait, F, and De Paepe, A. The Ehlers-Danlos syndrome. Adv Exp Med Biol. (2014) 802:129–43. doi: 10.1007/978-94-007-7893-1_9
98. Grach, SL, Seltzer, J, Chon, TY, and Ganesh, R. Diagnosis and management of myalgic encephalomyelitis/chronic fatigue syndrome. Mayo Clin Proc. (2023) 98:1544–51. doi: 10.1016/j.mayocp.2023.07.032
99. Boris, JR, Shadiack, EC 3rd, McCormick, EM, MacMullen, L, George-Sankoh, I, and Falk, MJ. Long-term POTS outcomes survey: diagnosis, therapy, and clinical outcomes. J Am Heart Assoc. (2024) 13:e033485. doi: 10.1161/JAHA.123.033485
100. Silva, J, Takahashi, T, Wood, J, Lu, P, Tabachnikova, A, Gehlhausen, JR, et al. Sex differences in symptomatology and immune profiles of long COVID. medRxiv. (2024) doi: 10.1101/2024.02.29.24303568
101. Dunn, SE, Perry, WA, and Klein, SL. Mechanisms and consequences of sex differences in immune responses. Nat Rev Nephrol. (2024) 20:37–55. doi: 10.1038/s41581-023-00787-w
102. Whitacre, CC. Sex differences in autoimmune disease. Nat Immunol. (2001) 2:777–80. doi: 10.1038/ni0901-777
103. Bruno, KA, Morales-Lara, AC, Bittencourt, EB, Siddiqui, H, Bommarito, G, Patel, J, et al. Sex differences in comorbidities associated with Sjögren's disease. Front Med (Lausanne) (2022) 9:958670. doi: 10.3389/fmed.2022.958670
104. Calissendorff, J, Cramon, PK, Hallengren, B, Khamisi, S, Lantz, M, Planck, T, et al. Long-term outcome of Graves' disease: a gender perspective. Womens Health Rep (New Rochelle). (2023) 4:487–96. doi: 10.1089/whr.2023.0073
105. Hughes, M, Pauling, JD, Armstrong-James, L, Denton, CP, Galdas, P, and Flurey, C. Gender-related differences in systemic sclerosis. Autoimmun Rev. (2020) 19:102494. doi: 10.1016/j.autrev.2020.102494
106. Danchenko, N, Satia, JA, and Anthony, MS. Epidemiology of systemic lupus erythematosus: a comparison of worldwide disease burden. Lupus. (2006) 15:308–18. doi: 10.1191/0961203306lu2305xx
107. Sundaresan, B, Shirafkan, F, Ripperger, K, and Rattay, K. The role of viral infections in the onset of autoimmune diseases. Viruses. (2023) 15:1–32. doi: 10.3390/v15030782
108. Taylor, MP, and Kirkegaard, K. Modification of cellular autophagy protein LC3 by poliovirus. J Virol. (2007) 81:12543–53. doi: 10.1128/JVI.00755-07
109. Kyei, GB, Dinkins, C, Davis, AS, Roberts, E, Singh, SB, Dong, C, et al. Autophagy pathway intersects with HIV-1 biosynthesis and regulates viral yields in macrophages. J Cell Biol. (2009) 186:255–68. doi: 10.1083/jcb.200903070
110. Gannagé, M, Dormann, D, Albrecht, R, Dengjel, J, Torossi, T, Rämer, PC, et al. Matrix protein 2 of influenza a virus blocks autophagosome fusion with lysosomes. Cell Host Microbe. (2009) 6:367–80. doi: 10.1016/j.chom.2009.09.005
111. Kim, SJ, Syed, GH, Khan, M, Chiu, WW, Sohail, MA, Gish, RG, et al. Hepatitis C virus triggers mitochondrial fission and attenuates apoptosis to promote viral persistence. Proc Natl Acad Sci USA. (2014) 111:6413–8. doi: 10.1073/pnas.1321114111
112. Sin, J, McIntyre, L, Stotland, A, Feuer, R, and Gottlieb, RA. Coxsackievirus B escapes the infected cell in ejected mitophagosomes. J Virol. (2017) 91:e01347–17. doi: 10.1128/JVI.01347-17
113. Oh, SJ, Lim, BK, Yun, J, and Shin, OS. CVB3-mediated mitophagy plays an important role in viral replication via abrogation of interferon pathways. Front Cell Infect Microbiol. (2021) 11:704494. doi: 10.3389/fcimb.2021.704494
114. Yang, Y, Wu, Y, Meng, X, Wang, Z, Younis, M, Liu, Y, et al. SARS-CoV-2 membrane protein causes the mitochondrial apoptosis and pulmonary edema via targeting BOK. Cell Death Differ. (2022) 29:1395–408. doi: 10.1038/s41418-022-00928-x
115. Faizan, MI, Chaudhuri, R, Sagar, S, Albogami, S, Chaudhary, N, Azmi, I, et al. NSP4 and ORF9b of SARS-CoV-2 induce pro-inflammatory mitochondrial DNA release in inner membrane-derived vesicles. Cells. (2022) 11:1–36. doi: 10.3390/cells11192969
116. Di Florio, DN, Beetler, DJ, McCabe, EJ, Sin, J, Ikezu, T, and Fairweather, D. Mitochondrial extracellular vesicles, autoimmunity and myocarditis. Front Immunol. (2024) 15:1374796. doi: 10.3389/fimmu.2024.1374796
117. Fedorowski, A, and Sutton, R. Autonomic dysfunction and postural orthostatic tachycardia syndrome in post-acute COVID-19 syndrome. Nat Rev Cardiol. (2023) 20:281–2. doi: 10.1038/s41569-023-00842-w
118. Csecs, JLL, Iodice, V, Rae, CL, Brooke, A, Simmons, R, Quadt, L, et al. Joint hypermobility links neurodivergence to dysautonomia and pain. Front Psych. (2021) 12:786916. doi: 10.3389/fpsyt.2021.786916
119. Shan, ZY, Barnden, LR, Kwiatek, RA, Bhuta, S, Hermens, DF, and Lagopoulos, J. Neuroimaging characteristics of myalgic encephalomyelitis/chronic fatigue syndrome (ME/CFS): a systematic review. J Transl Med. (2020) 18:335. doi: 10.1186/s12967-020-02506-6
120. Thapaliya, K, Marshall-Gradisnik, S, Eaton-Fitch, N, Eftekhari, Z, Inderyas, M, and Barnden, L. Imbalanced brain neurochemicals in long COVID and ME/CFS: a preliminary study using MRI. Am J Med. (2024). doi: 10.1016/j.amjmed.2024.04.007
121. Nakatomi, Y, Mizuno, K, Ishii, A, Wada, Y, Tanaka, M, Tazawa, S, et al. Neuroinflammation in patients with chronic fatigue syndrome/myalgic encephalomyelitis: an 11C-(R)-PK11195 PET study. J Nucl Med. (2014) 55:945–50. doi: 10.2967/jnumed.113.131045
122. Horowitz, T, Dudouet, P, Campion, JY, Kaphan, E, Radulesco, T, Gonzalez, S, et al. Persistent brain metabolic impairment in long COVID patients with persistent clinical symptoms: a nine-month follow-up [(18)F]FDG-PET study. Eur J Nucl Med Mol Imaging. (2024) 51:3215–22. doi: 10.1007/s00259-024-06775-x
123. Goudman, L, De Smedt, A, Roggeman, S, Fernández-de-Las-Peñas, C, Hatem, SM, Schiltz, M, et al. Association between experimental pain measurements and the central sensitization inventory in patients at least 3 months after COVID-19 infection: a cross-sectional pilot study. J Clin Med. (2023) 12:1–14. doi: 10.3390/jcm12020661
124. Perumal, R, Shunmugam, L, Naidoo, K, Abdool Karim, SS, Wilkins, D, Garzino-Demo, A, et al. Long COVID: a review and proposed visualization of the complexity of long COVID. Front Immunol. (2023) 14:1117464. doi: 10.3389/fimmu.2023.1117464
125. Arun, S, Storan, A, and Myers, B. Mast cell activation syndrome and the link with long COVID. Br J Hosp Med (Lond). (2022) 83:1–10. doi: 10.12968/hmed.2022.0123
126. Theoharides, TC. Potential association of mast cells with coronavirus disease 2019. Ann Allergy Asthma Immunol. (2021) 126:217–8. doi: 10.1016/j.anai.2020.11.003
127. Fairweather, D, Beetler, DJ, Di Florio, DN, Musigk, N, Heidecker, B, and Cooper, LT Jr. COVID-19, myocarditis and pericarditis. Circ Res. (2023) 132:1302–19. doi: 10.1161/CIRCRESAHA.123.321878
128. Theoharides, TC, Tsilioni, I, and Ren, H. Recent advances in our understanding of mast cell activation - or should it be mast cell mediator disorders? Expert Rev Clin Immunol. (2019) 15:639–56. doi: 10.1080/1744666X.2019.1596800
129. Toyoshima, S, and Okayama, Y. Neuro-allergology: mast cell-nerve cross-talk. Allergol Int. (2022) 71:288–93. doi: 10.1016/j.alit.2022.04.002
130. Yu, F, Liu, X, Ou, H, Li, X, Liu, R, Lv, X, et al. The histamine receptor H1 acts as an alternative receptor for SARS-CoV-2. MBio. (2024) 15:e01088–24. doi: 10.1128/mbio.01088-24
131. Fairweather, D, Frisancho-Kiss, S, Yusung, SA, Barrett, MA, Davis, SE, Gatewood, SJ, et al. Interferon-gamma protects against chronic viral myocarditis by reducing mast cell degranulation, fibrosis, and the profibrotic cytokines transforming growth factor-beta 1, interleukin-1 beta, and interleukin-4 in the heart. Am J Pathol. (2004) 165:1883–94. doi: 10.1016/S0002-9440(10)63241-5
132. Conti, P, Caraffa, A, Tetè, G, Gallenga, CE, Ross, R, Kritas, SK, et al. Mast cells activated by SARS-CoV-2 release histamine which increases IL-1 levels causing cytokine storm and inflammatory reaction in COVID-19. J Biol Regul Homeost Agents. (2020) 34:1629–32. doi: 10.23812/20-2EDIT
133. Conti, P, Ronconi, G, Lauritano, D, Mastrangelo, F, Caraffa, A, Gallenga, CE, et al. Impact of TNF and IL-33 cytokines on mast cells in neuroinflammation. Int J Mol Sci. (2024) 25:1–16. doi: 10.3390/ijms25063248
134. Ganesh, R, Yadav, S, Hurt, RT, Mueller, MR, Aakre, CA, Gilman, EA, et al. Pro inflammatory cytokines profiles of patients with long COVID differ between variant epochs. J Prim Care Community Health. (2024) 15:21501319241254751. doi: 10.1177/21501319241254751
135. Abston, ED, Coronado, MJ, Bucek, A, Bedja, D, Shin, J, Kim, JB, et al. Th2 regulation of viral myocarditis in mice: different roles for TLR3 versus TRIF in progression to chronic disease. Clin Dev Immunol. (2012) 2012:129486. doi: 10.1155/2012/129486
136. Mathias, CJ, Owens, A, Iodice, V, and Hakim, A. Dysautonomia in the Ehlers-Danlos syndromes and hypermobility spectrum disorders-with a focus on the postural tachycardia syndrome. Am J Med Genet C Semin Med Genet. (2021) 187:510–9. doi: 10.1002/ajmg.c.31951
137. Yu, S, Gao, G, Peterson, BZ, and Ouyang, A. TRPA1 in mast cell activation-induced long-lasting mechanical hypersensitivity of vagal afferent C-fibers in guinea pig esophagus. Am J Physiol Gastrointest Liver Physiol. (2009) 297:G34–42. doi: 10.1152/ajpgi.00068.2009
138. Yang, H, George, SJ, Thompson, DA, Silverman, HA, Tsaava, T, Tynan, A, et al. Famotidine activates the vagus nerve inflammatory reflex to attenuate cytokine storm. Mol Med. (2022) 28:57. doi: 10.1186/s10020-022-00483-8
139. Mackey, E, Thelen, KM, Bali, V, Fardisi, M, Trowbridge, M, Jordan, CL, et al. Perinatal androgens organize sex differences in mast cells and attenuate anaphylaxis severity into adulthood. Proc Natl Acad Sci USA. (2020) 117:23751–61. doi: 10.1073/pnas.1915075117
140. Mackey, E, and Moeser, AJ. Sex differences in mast cell-associated disorders: a life span perspective. Cold Spring Harb Perspect Biol. (2022) 14:1–14. doi: 10.1101/cshperspect.a039172
141. Smith, SJ, Sichlau, MJ, Smith, BH, Knight, DRT, Chen, B, and Rowe, PC. Improvement in chronic pelvic pain, orthostatic intolerance and interstitial cystitis symptoms after treatment of pelvic vein insufficiency. Phlebology. (2023) 39:202–13. doi: 10.1177/02683555231219737
142. Ormiston, CK, Padilla, E, Van, DT, Boone, C, You, S, Roberts, AC, et al. May-Thurner syndrome in patients with postural orthostatic tachycardia syndrome and Ehlers-Danlos syndrome: a case series. Eur Heart J Case Rep. (2022) 6:1–7. doi: 10.1093/ehjcr/ytac161
143. Smith, SJ, Smith, BH, Sichlau, MJ, Chen, B, Knight, D, and Rowe, PC. Nonpelvic comorbid symptoms of 45 patients with pain of pelvic venous origin, before and after treatment. Phlebology. (2024):2683555241273109. doi: 10.1177/02683555241273109
144. Bonilla, H, Peluso, MJ, Rodgers, K, Aberg, JA, Patterson, TF, Tamburro, R, et al. Therapeutic trials for long COVID-19: a call to action from the interventions taskforce of the RECOVER initiative. Front Immunol. (2023) 14:1129459. doi: 10.3389/fimmu.2023.1129459
145. Bateman, L, Bested, AC, Bonilla, HF, Chheda, BV, Chu, L, Curtin, JM, et al. Myalgic encephalomyelitis/chronic fatigue syndrome: essentials of diagnosis and management. Mayo Clin Proc. (2021) 96:2861–78. doi: 10.1016/j.mayocp.2021.07.004
146. Vernon, SD, Hartle, M, Sullivan, K, Bell, J, Abbaszadeh, S, Unutmaz, D, et al. Post-exertional malaise among people with long COVID compared to myalgic encephalomyelitis/chronic fatigue syndrome (ME/CFS). Work. (2023) 74:1179–86. doi: 10.3233/WOR-220581
147. Ghali, A, Lacombe, V, Ravaiau, C, Delattre, E, Ghali, M, Urbanski, G, et al. The relevance of pacing strategies in managing symptoms of post-COVID-19 syndrome. J Transl Med. (2023) 21:375. doi: 10.1186/s12967-023-04229-w
148. Hoffmann, K, Hainzl, A, Stingl, M, Kurz, K, Biesenbach, B, Bammer, C, et al. Interdisciplinary, collaborative D-A-CH (Germany, Austria and Switzerland) consensus statement concerning the diagnostic and treatment of myalgic encephalomyelitis/chronic fatigue syndrome. Wien Klin Wochenschr. (2024) 136:103–23. doi: 10.1007/s00508-024-02372-y
149. Zadeh, FH, Wilson, DR, and Agrawal, DK. Long COVID: complications, underlying mechanisms, and treatment strategies. Arch Microbiol Immunol. (2023) 7:36–61.
150. Izzo, R, Trimarco, V, Mone, P, Aloè, T, Capra Marzani, M, Diana, A, et al. Combining L-arginine with vitamin C improves long-COVID symptoms: the LINCOLN survey. Pharmacol Res. (2022) 183:106360. doi: 10.1016/j.phrs.2022.106360
151. Charfeddine, S, ibn Hadjamor, H, Torjmen, S, Kraiem, S, Hammami, R, Bahloul, A, et al. Sulodexide in the treatment of patients with long COVID 19 symptoms and endothelial dysfunction: the results of TUN-EndCOV study. Arch Cardiovasc Dis. (2022) 14:127. doi: 10.1016/j.acvdsp.2021.10.007
152. Eissa, N, Al-Houqani, M, Sadeq, A, Ojha, SK, Sasse, A, and Sadek, B. Current enlightenment about etiology and pharmacological treatment of autism spectrum disorder. Front Neurosci. (2018) 12:304. doi: 10.3389/fnins.2018.00304
153. Bolton, MJ, Chapman, BP, and Van Marwijk, H. Low-dose naltrexone as a treatment for chronic fatigue syndrome. BMJ Case Rep. (2020) 13:e232502. doi: 10.1136/bcr-2019-232502
154. Yang, J, Shin, KM, Do, A, Bierle, DM, Abu Dabrh, AM, Yin, Z, et al. The safety and efficacy of low-dose naltrexone in patients with fibromyalgia: a systematic review. J Pain Res. (2023) 16:1017–23. doi: 10.2147/JPR.S395457
155. Younger, J, Noor, N, McCue, R, and Mackey, S. Low-dose naltrexone for the treatment of fibromyalgia: findings of a small, randomized, double-blind, placebo-controlled, counterbalanced, crossover trial assessing daily pain levels. Arthritis Rheum. (2013) 65:529–38. doi: 10.1002/art.37734
156. O'Kelly, B, Vidal, L, McHugh, T, Woo, J, Avramovic, G, and Lambert, JS. Safety and efficacy of low dose naltrexone in a long covid cohort; an interventional pre-post study. Brain Behav Immun Health. (2022) 24:100485. doi: 10.1016/j.bbih.2022.100485
157. Stallkamp Tidd, SJ, Cantrell, C, Greene, BD, and Wilson, R. Low-dose naltrexone use in postural orthostatic tachycardia syndrome: a case series. Cureus. (2023) 15:e43426. doi: 10.7759/cureus.43426
158. Weinstock, LB, Brook, JB, Myers, TL, and Goodman, B. Successful treatment of postural orthostatic tachycardia and mast cell activation syndromes using naltrexone, immunoglobulin and antibiotic treatment. BMJ Case Rep. (2018) 2018:bcr-2017-221405. doi: 10.1136/bcr-2017-221405
159. Marcus, NJ, Robbins, L, Araki, A, Gracely, EJ, and Theoharides, TC. Effective doses of low-dose naltrexone for chronic pain - an observational study. J Pain Res. (2024) 17:1273–84. doi: 10.2147/JPR.S451183
160. Lie, M, van der Giessen, J, Fuhler, GM, de Lima, A, Peppelenbosch, MP, van der Ent, C, et al. Low dose naltrexone for induction of remission in inflammatory bowel disease patients. J Transl Med. (2018) 16:55. doi: 10.1186/s12967-018-1427-5
161. Pasricha, PJ, McKnight, M, Villatoro, L, Barahona, G, Brinker, J, Hui, K, et al. A syndrome of joint hypermobility, autonomic dysfunction, gastrointestinal dysfunction and autoimmune markers (JAG-A): clinical associations and response to intravenous immunoglobulin therapy. medRxiv. 2023
162. Achleitner, M, Steenblock, C, Dänhardt, J, Jarzebska, N, Kardashi, R, Kanczkowski, W, et al. Clinical improvement of long-COVID is associated with reduction in autoantibodies, lipids, and inflammation following therapeutic apheresis. Mol Psychiatry. (2023) 28:2872–7. doi: 10.1038/s41380-023-02084-1
163. Stein, E, Heindrich, C, Wittke, K, Kedor, CA-O, Kim, LA-O, Freitag, HA-O, et al. Observational study of repeat immunoadsorption (RIA) in post-COVID ME/CFS patients with elevated ß2-adrenergic receptor autoantibodies-an interim report. J Clin Med. (2023) 12:6428. doi: 10.3390/jcm12196428
164. McCarthy, MW. Intravenous immunoglobulin as a potential treatment for long COVID. Expert Opin Biol Ther. (2023) 23:1211–7. doi: 10.1080/14712598.2023.2296569
165. Preßler, H, Machule, ML, Ufer, F, Bünger, I, Li, LY, Buchholz, E, et al. IA-PACS-CFS: a double-blinded, randomized, sham-controlled, exploratory trial of immunoadsorption in patients with chronic fatigue syndrome (CFS) including patients with post-acute COVID-19 CFS (PACS-CFS). Trials. (2024) 25:172. doi: 10.1186/s13063-024-07982-5
166. Vogel, JM, Pollack, B, Spier, E, McCorkell, L, Jaudon, TW, Fitzgerald, M, et al. Designing and optimizing clinical trials for long COVID. Life Sci. (2024) 355:122970. doi: 10.1016/j.lfs.2024.122970
167. McGeoch, CLB, Steinberg, RS, Bortfeld, KS, Almuwaqqat, Z, Rheudasil, JM, Bhatia, NK, et al. Radiofrequency venous ablation for symptomatic relief in postural orthostatic tachycardia syndrome: a case series. Eur Heart J Case Rep. (2024) 8:ytae029. doi: 10.1093/ehjcr/ytae029
168. CDC. New ICD-19-CM code for post-COVID conditions, following the 2019 novel coronavirus (COVID-19) (2021). Available at: https://www.cdc.gov/nchs/data/icd/announcement-new-icd-code-for-post-covid-condition-april-2022-final.pdf (Accessed August 18, 2024).
169. CDC. Technical updates. CDC archive. (2021). Available at: https://archive.cdc.gov/www_cdc_gov/nssp/news/2021/03-march/technical-updates.html (Accessed August 18, 2024).
170. Harris, PA, Taylor, R, Thielke, R, Payne, J, Gonzalez, N, and Conde, JG. Research electronic data capture (REDCap)—a metadata-driven methodology and workflow process for providing translational research informatics support. J Biomed Inform. (2009) 42:377–81. doi: 10.1016/j.jbi.2008.08.010
Keywords: SARS-CoV-2, generalized joint hypermobility, myalgic encephalomyelitis/chronic fatigue syndrome, hypermobile Ehlers-Danlos syndrome, orthostatic intolerance, pain, virus, mast cells
Citation: Grach SL, Dudenkov DV, Pollack B, Fairweather D, Aakre CA, Munipalli B, Croghan IT, Mueller MR, Overgaard JD, Bruno KA, Collins NM, Li Z, Hurt RT, Tal MC, Ganesh R and Knight DTR (2024) Overlapping conditions in Long COVID at a multisite academic center. Front. Neurol. 15:1482917. doi: 10.3389/fneur.2024.1482917
Edited by:
Ilene Sue Ruhoy, Mount Sinai South Nassau, United StatesReviewed by:
Alexis Cutchins, Emory University, United StatesMary Dimmock, Independent Researcher, Waterford, NY, United States
Copyright © 2024 Grach, Dudenkov, Pollack, Fairweather, Aakre, Munipalli, Croghan, Mueller, Overgaard, Bruno, Collins, Li, Hurt, Tal, Ganesh and Knight. This is an open-access article distributed under the terms of the Creative Commons Attribution License (CC BY). The use, distribution or reproduction in other forums is permitted, provided the original author(s) and the copyright owner(s) are credited and that the original publication in this journal is cited, in accordance with accepted academic practice. No use, distribution or reproduction is permitted which does not comply with these terms.
*Correspondence: Stephanie L. Grach, R3JhY2guU3RlcGhhbmllQG1heW8uZWR1
†These authors have contributed equally to this work and share first authorship
‡These authors have contributed equally to this work and share senior authorship