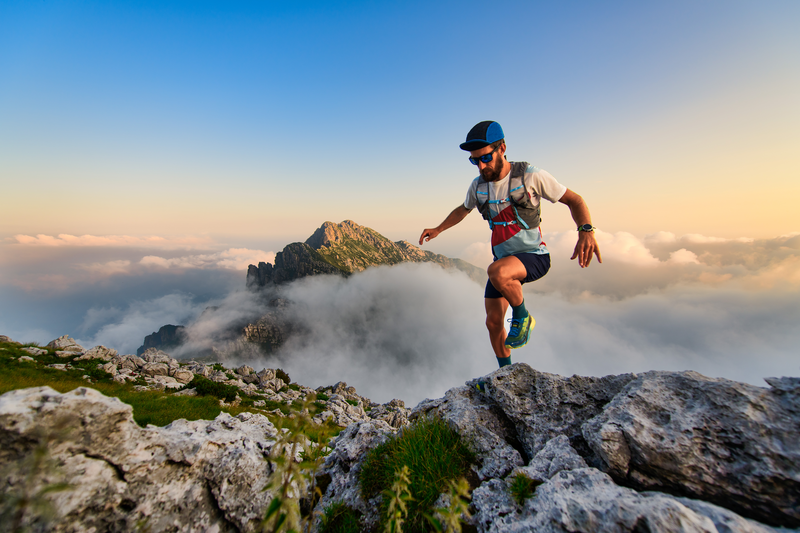
94% of researchers rate our articles as excellent or good
Learn more about the work of our research integrity team to safeguard the quality of each article we publish.
Find out more
BRIEF RESEARCH REPORT article
Front. Neurol. , 23 September 2024
Sec. Neurotrauma
Volume 15 - 2024 | https://doi.org/10.3389/fneur.2024.1467419
Traumatic brain injury (TBI) remains a significant global public health epidemic with adverse health and cost implications. Due to its complex, heterogeneous nature and wide-ranging impacts, definitive TBI treatments remain elusive. As such, continued laboratory research using animal models is warranted. In accordance with guidelines set forth for the humane treatment of research animals, TBI animal models are often administered analgesics for pain management. The choice of drug, timing, dose, and formulation of analgesic can vary depending on the study’s unique needs and can potentially and unintentionally influence experimental results. In TBI studies utilizing rats as animal models, buprenorphine is a common analgesic administered. In addition to pain management in such studies, investigators must also monitor the research animals post-operatively and make the decision for humane euthanasia before intended experimental survival timepoint if the animals are assessed to be excessively suffering. This study investigated the differences in adult, male Sprague Dawley rats used for various TBI studies that reached weight-loss-induced humane endpoints following a single administration of buprenorphine slow-release LAB (bup-SR-LAB) or buprenorphine slow-release HCl (bup-SR-HCl). Our findings indicate that TBI-induced rats receiving bup-SR-LAB in conjunction with a secondary surgical insult such as artificial intracranial pressure elevation and/or osmotic pump implantation reach a weight-loss-induced humane euthanasia endpoint more often compared to sham-injured rats. When stratifying into the same groups, we did not find this pattern to hold true for rats administered bup-SR-HCl. Overall, this study contributes to the limited body of literature addressing different analgesic formulations’ effects on laboratory animals.
Traumatic brain injury (TBI) is a significant global public health burden with an estimated yearly incidence between 27 to 69 million people and with no definitive cure (1–3). It is a complex and highly heterogenous condition that can result in a wide range of cognitive (4–6), emotional (7–9), sensory (10), and physical (11–13) impairments that can be both acute and chronic (14–16). The complexity of TBI pathophysiology has thus far prevented effective treatments from being developed, underscoring a need for continued research. To better understand the underlying mechanisms of TBI and develop effective therapeutics, various pre-clinical animal models have been established for research use. Such models are beneficial for characterizing and manipulating molecular mechanisms and chemical cascades that occur post-injury.
When conducting TBI survival studies, particularly those involving longer-term experimental timepoints, an additional variable that must be considered is the appropriate pain management of the animals (17–20). Depending on the species and interventions performed, different drugs, formulations, routes of administration, and dosages are utilized (18, 21). In TBI procedures utilizing rats, buprenorphine is an effective and commonly used analgesic (22, 23). Buprenorphine is a high-affinity partial agonist at the mu-opioid receptor as well as an antagonist at the kappa-opioid receptor, providing analgesia with lower risks of respiratory depression and sedation compared to full opioid agonists (24). Previous studies have shown mixed results regarding the impact of buprenorphine on various physiological and behavioral parameters in animal models, with some indications for potential effects on outcomes in TBI research (25–29). Our group has previously reported that a single dose of slow-release buprenorphine administered once following induction of a central fluid percussion injury (cFPI) to the brain in rats precipitates glial cell morphological changes (30, 31). However, as a whole the different analgesic impacts on research animals undergoing a variety of procedures, both TBI and elsewise, remain underreported on.
The current study is a secondary retrospective analysis of rats from various TBI studies in our lab receiving two different formulations and veterinarian-recommended dosages of buprenorphine, buprenorphine slow-release LAB (bup-SR-LAB) and buprenorphine slow-release HCl (bup-SR-HCl). During the execution of these previous studies, it was observed that a higher-than-expected number of animals were reaching humane endpoints due to weight loss after procedures. In the current study, we investigated rats administered buprenorphine following sham injury or cFPI-induced TBI that reached a humane endpoint due to weight loss, thereby requiring euthanasia before intended experimental endpoint.
This study is a retrospective analysis of animals generated for other TBI studies. All animal studies were conducted in accordance with ARRIVE guidelines (32) and approved by the Institutional Animal Care and Use Committee at Virginia Commonwealth University (approval #AM10251), which adhere to regulations including, but not limited to, the Virginia Commonwealth University institutional ethical guidelines concerning the care and use of laboratory animals, and those set forth in the Guide for the Care and Use of Laboratory Animals: 8th Edition (33). Adult (12-16-week-old), male Sprague Dawley rats were included in this study. Animals were housed in individual cages on a 12-h light–dark cycle with free access to food and water as well as full veterinary oversight.
All animals used in this analysis were originally generated for and were part of other studies, some of which have been incorporated in previously published works (31, 34, 35). During the time from January 1, 2019, through December 31, 2021, a total of 274 animals were generated by the laboratory and 140 met initial inclusion criteria for this analysis (Figure 1). Included animals were all administered either bup-SR-LAB (1 mg/mL; ZooPharm, Laramie, WY, United States) from January 1, 2019, through December 31, 2020, or bup-SR-HCl (1 mg/mL; ZooPharm, Laramie, WY, United States) from January 1, 2021, through December 31, 2021. All bup-SR doses were administered subcutaneously (SC) 15 min post-injury. No other notable changes were made to surgical preparations, procedures, or animal care during this time.
Figure 1. Flow chart of study inclusion and exclusion criteria. This flow chart depicts the total number of Sprague Dawley rats generated during the period of analysis from January 2019 through December of 2021. Rats generated for non-survival studies and rats not administered buprenorphine after surgery were excluded from this analysis. The final number of animals eligible to be included in the study were divided into the two main cohorts of interest: those receiving the bup-SR-LAB formulation and those receiving the bup-SR-HCl formulation administered subcutaneously at 15 min post-surgery.
The five experimental design groups used for this retrospective investigation encompassed all general conditions of the animals the laboratory generated. The five groups were as follows: group (1) sham-injured controls (Sham; bup-SR-LAB n = 13, bup-SR-HCl n = 3); group (2) sham-injured animals with osmotic pump implantation (Sham + Implant; bup-SR-LAB n = 10, bup-SR-HCl n = 12); group (3) animals sustaining only cFPI (TBI; bup-SR-LAB n = 17, bup-SR-HCl n = 4); group (4) animals sustaining cFPI followed by osmotic pump implantation and secondary ICP elevation (TBI + ICP + Implant; bup-SR-LAB n = 16, bup-SR-HCl n = 14); group (5) animals sustaining cFPI followed by either osmotic pump implantation or secondary ICP elevation (TBI + ICP or Implant; bup-SR-LAB n = 23, bup-SR-HCl n = 28). For all studies included in this retrospective analysis, a random number generator determined animal group prior to surgery.
Methodology for surgical preparation and injury induction of animals used for this retrospective study have been published previously (31, 34, 35). Briefly, anesthesia was induced with 4% isoflurane in 30% O2/70% room air. Animals were ventilated with 1.5–2.5% isoflurane in 30% O2/70% room air throughout surgery duration, injury, and post-injury ICP elevation and/or osmotic pump implantation. Body temperature was maintained at 37°C with a rectal thermometer connected to a feedback-controlled heating pad (Harvard Apparatus, Holliston, MA, United States). Animals were placed in a stereotaxic frame (David Kopf Instruments, Tujunga, CA, United States) and a 4.8 mm diameter circular craniectomy was made along the sagittal suture midway between bregma and lambda, leaving the dura intact. Following the central craniectomy, a 2 mm diameter burr hole was drilled over the left lateral ventricle positioned at 1.3 mm lateral, 0.8 mm posterior, and 2.5–3 mm ventral to bregma. A 25G canula connected was to a pressure transducer and an 11 Elite microinfusion pump (Harvard Apparatus) in a closed, fluid-filled system was advanced into the lateral ventricle to measure intracranial pressure (ICP). Bone wax was used to seal the burr hole used for ICP measurements before preparation for central fluid percussion injury (cFPI). Procedures used to induce cFPI were consistent with those described previously (31, 34, 36, 37). Briefly, a Luer-Lok™ syringe (BD, Franklin Lakes, NJ, United States) hub was affixed to the craniectomy site with methyl methacrylate (Hygenic, Akron, OH, United States) applied around the hub and was allowed to harden. Animals were removed from the stereotaxic frame and placed on a raised platform for connection to the fluid percussion device, maintaining an unbroken fluid-filled system from the intact dura through the cylinder via an adaptor. To induce a mild-to-moderate cFPI, a pendulum was released onto the end of the fluid-filled cylinder of the percussion device, producing a pressure pulse that reached the animal at 2.05 ± 0.10 atmospheres for a duration of approximately 22.5 ms. This pressure pulse was transduced through the intact dura to the CSF and spread diffusely throughout the brain. The magnitude of the pressure pulse was measured via transducer affixed to the injury device and output on an oscilloscope display (Tektronix, Beaverton, OR, United States). For sham-injured animals, the same procedures were performed except the final pendulum release. Immediately following injury, animals were reconnected to the ventilator and the hub, dental acrylic, and bone wax were removed en bloc and gel foam was placed overtop the craniectomy site to stop bleeding. The animals were replaced in the stereotaxic frame and the ICP probe was reinserted into the lateral ventricle for post-injury ICP monitoring or manual ICP elevation. For animals undergoing manual ICP elevation to 15, 20, or 25 mmHg, infusion of sterile normal saline at a rate of 1.3–13 μL/min was performed. Once a target ICP was achieved, it was maintained at a steady state for 1 h. Fifteen min following the sham injury or cFPI, buprenorphine (1 mg/kg of a 1 mg/mL bup-SR-LAB solution or 0.9 mg/kg of a 1 mg/mL solution of bup-SR-HCl from ZooPharm, Laramie, WY, United States) was administered subcutaneously, with dosages following veterinarian recommendations. One hour following the sham injury or cFPI, animals in groups 2, 4, and 5 underwent implantation of a mini osmotic pump into the subcutaneous neck facia. A canula was run from the osmotic pump to a brain infusion adaptor implanted into the ICP burr hole for infusion into the left lateral ventricle.
Following recovery, rats were returned to individual housing in clean home cages. Comprehensive postoperative monitoring was done to obtain a more complete picture of animal recovery in which a three-category moribundity score was used. Specifically, animals were assessed for (1) general appearance (dehydration, decreased body weight < 20%, abnormal posture, swelling, prolapses), (2) skin/fur appearance (discoloration, urine stain, pallor, redness, cyanosis, wounds, sores, abscesses, ulcers, ruffled fur), and (3) locomotion (hyperactivity, lethargy, coma, ataxia, tremors). Each category was scored from 0 (best score) to 2 (worst score). Animals were assessed using this scale and weighed daily for at least 3 days post-surgery and weekly thereafter. Animals raising any humane concerns were monitored more frequently, being weighed and assessed daily until humane concerns resolved or until a humane endpoint was reached.
All statistical analyses were conducted using R (R4.3.3) (38). Pairwise Fisher exact tests were used for comparisons between groups. Statistical significance threshold was set at p = 0.05.
Of the 274 animals generated by the laboratory during the defined analysis period from January 1, 2019, through December 31, 2021, a total of 140 animals met initial inclusion criteria for this retrospective analysis (Figure 1). One hundred and thirteen animals were excluded from this assessment due to being part of non-survival studies or acute studies in which the animals’ experimental endpoint was intended for less than 2 weeks post-cFPI. A total of 21 animals incorporated into Ryu et al. Two thousand and twenty two were not administered any form of bup-SR and were therefore excluded from this study (31). Ultimately, 79 animals administered a vet-recommended dose of SC bup-SR-LAB (1 mg/kg), and 61 animals administered a vet-recommended dose of SC bup-SR-HCl (0.9 mg/kg) 15 min post-cFPI had experimental endpoints of at least 2 weeks post-injury and met all appropriate inclusion criteria to be stratified for further analysis (Figure 1).
As stated previously, all animals were weighed on day of surgery prior to undergoing planned procedures. Mean pre-surgery weight (i.e., day 0) was 428 g with a standard deviation of 54.4 g (Supplementary Figure S1). Assessments for humane euthanasia were made during daily welfare checks using aforementioned criteria. Specifically, 77% (17/22) of the humane endpoint bup-SR-LAB animals had weight loss noted as a primary driver for humane euthanasia. The reasons for humane endpoint for the remaining bup-SR-LAB animals were suture integrity issues, 14% (3/22), and procedural complications, 9% (2/22). All animals receiving bup-SR-HCl that were euthanized prematurely had weight loss concerns primarily contributing to humane endpoints.
Of the 74 total rats administered bup-SR-LAB, 77% (57/74) survived the entirety of the intended study period without weight-associated humane concerns (Figure 2A). Eight bup-SR-LAB rats (11%) dropped below 80% of their pre-injury body weight, which was the humane weight-loss threshold. An additional nine bup-SR-LAB rats (12%) did not reach humane weight-loss threshold of 80%, but did have weight loss trajectories that, when coupled with other observed humane indications, resulted in humane endpoints. Of the 61 total rats administered bup-SR-HCl, 89% (54/61) survived the entirety of the intended study period without humane concerns (Figure 2B). Four bup-SR-HCl rats (7%) dropped below 80% of their pre-injury weight. An additional three bup-SR-HCl rats (5%) did not reach the weight-loss humane threshold but had weight loss trajectories that, coupled with other humane concerns, resulted in humane endpoints (Figure 2B).
Figure 2. Reductions in the percentage of pre-injury body weight following TBI reached humane endpoints for a subset of animals administered bup-SR-LAB or bup-SR-HCl. Line grants depicting the percentage of pre-surgery body weight for all rats included in this analysis administered either (A) bup-SR-LAB or (B) bup-SR-HCl. Each line represents an individual rat. Points (squares bup-SR-LAB and circles bup-SR-HCl) indicate the percentage of pre-surgery body weight at 0, 1, 2, 3, 7, 14, 21, and 28 days post-surgery. Lines terminate at the animal’s final weight taken prior to euthanasia. Dashed red line indicates pre-determined humane weight-loss threshold endpoint, set at ≥80% body weight loss after surgery. Gray lines represent rats that survived as intended, reaching experimental endpoints. Orange lines represent rats that met criteria for humane endpoints.
Based on study designs conducted from 2019 to 2021, rats receiving either bup-SR-LAB or bup-SR-HCl were stratified into the five groups described in more detail previously: (1) Sham, (2) Sham + Implant, (3) TBI, (4) TBI + ICP + Implant, and (5) TBI + ICP or Implant. Upon stratifying bup-SR-LAB rats into these groups, it was found that the majority of humanely euthanized rats with significant weight loss concerns were given a cFPI paired with another insult, either osmotic pump implantation, secondary ICP elevation, or both (Figure 3A; Supplementary Figure S2A). Specifically, while 100% of Sham animals, 89% of Sham + Implant animals, and 82% of TBI animals survived until experimental endpoint, significantly fewer bup-SR-LAB rats within the TBI + ICP + Implant group (67%, p = 0.047 vs. Sham) or the TBI + ICP or Implant group (62%, p = 0.030 vs. Sham) survived to experimental endpoint (Figure 3A; Supplementary Figure S2A). This decrease in survival was not observed in animals administered bup-SR-HCl, as all groups maintained experimental endpoint survival rates at or above 75% (Figure 3B; Supplementary Figure S2B).
Figure 3. Lower percentage of rats administered bup-SR-LAB following TBI paired with an osmotic pump implantation and/or ICP elevation survived to experimental endpoints. Bar graphs representing the percentage of animals that survived to their experimental endpoint administered (A) bup-SR-LAB or (B) bup-SR-HCl. *p < 0.05 compared to sham.
In TBI research as well as in other fields, the opioid buprenorphine is a common analgesic choice given to model animals (21). Extended-release or slow-release formulations are preferred in many cases due to longer acting time courses, which are reported to last up to 72 h (22, 39, 40), thus allowing for the administration of fewer doses while still adequately addressing the animals’ postoperative pain. While many researchers use bup-SR in their studies, few published reports exist regarding the impact different bup-SR formulations May have on investigations of TBI. Due to alternations in our study design, in 2019 we began to administer a single veterinarian-recommended dose of bup-SR-LAB for pain relief. Specifically, the studies that the rats were involved in required the implantation of osmotic pumps into the lateral ventricles and artificial increases in ICP following sham injury or cFPI-induced TBI. From 2019 through the end of 2020, we observed that a higher-than-expected number of animals were reaching humane endpoints due to weight loss. After ensuring that protocols and procedures were appropriately being carried out by all surgeons, input from the overseeing veterinarian led the team to switch the post-operative analgesic from 1 mg/kg bup-SR-LAB to 0.9 mg/kg bup-SR-HCl in January of 2021. While some animals did still reach humane endpoints due to weight loss concerns, a notable decrease in amount was observed, prompting this study. Therefore, we conducted a retrospective analysis investigating the proportion of rats given bup-SR-LAB or bup-SR-HCl following varying TBI interventions that reached a humane endpoint due to weight loss.
Because the different formulations of bup-SR were given in sequence and not in parallel, we did not directly compare the groups of animals between given bup-SR formulations. Therefore, we binned animals into five experimental design groups to assess if increase in weight-related humane endpoints was related to a combination of the types of procedures the rats underwent and the bup-SR formulation given. Groupings were made as specific as possible given the experiments performed. We found that rats administered bup-SR-LAB post-TBI paired with ICP elevation and/or osmotic pump implantation were particularly vulnerable to weight-related humane endpoints (Figures 2A, 3A). This increased vulnerability was not observed in rats administered bup-SR-HCl (Figures 2B, 3B). The nature of secondary, retrospective analyses such as this imparts certain limitations. Since the animal studies were designed and powered for a variety of other experiments and not a head-to-head assessment between the two bup-SR formulations’ effects on animal weight, the number of animals within the five groups were not equal. As such, the analysis May not be adequately powered to robustly reveal all differences between groups.
Since the surgeries were all performed by the same group of surgeons in the same laboratory using the same equipment, methods, and general protocols, the likelihood of such factors significantly influencing data are minimal. All surgeons were trained to levels of proficiency and the lab has extensive experience using the cFPI model. An additional factor to consider with potential to contribute to survival differences is the seasonal effect on laboratory animals, with effects previously shown (41–45). However, given the inclusion criteria dates spanned entire calendar years and animals were operated on consistently throughout the year, such potential seasonal biases are minimized. An additional consideration was the decrease in dose from 1 mg/kg bup-SR-LAB to 0.9 mg/kg bup-SR-HCl. Based on pre-surgical weights of the heaviest (694 g) and lightest (339 g) rats, the maximum administration difference between bup-SR-HCl and bup-SR-LAB was between 0.03 and 0.07 mg. This 10% difference in the dose was not assessed to be a significant enough contributor to result in the overall differences in humane endpoints observed (46–49). Other factors that May influence outcomes differences observed are surgical complications and/or infections. While a few rats incurred surgical complications requiring humane euthanasia, these complications were distinct from weight loss concerns and these animals were removed from analysis. Due to maintained consistency across the majority of study components, data presented here are valid and appropriate for use in the types of analyses reported.
While experiments were appropriately powered for the original, unfortunately we do not have the appropriate samples or data collected to more vigorously investigate the potential underlying reasons for the increased number of rats reaching weight-loss-induced humane endpoints with the bup-SR-LAB formulation versus the bup-SR-HCl formulation. While previous studies in our laboratory indicated a positive impact on weight with administration of bup-SR-LAB when compared with saline (30), these studies did not include groups with TBI and a secondary surgical insult, which were the ones found to be at-risk in this analysis. As such, those results are not contrary but rather additive to the ones reported here. Additionally, we do not know which compounds that exist in the bup-SR-LAB are not present in the bup-SR-HCl, and therefore which compounds May be contributing to the increased humane endpoints we observed. Overall, studies such as this help further inform the ever-growing field of TBI researchers on how analgesics could potentially (and unintentionally) influence data.
Anesthesia and analgesia are administered to laboratory animals to control their perioperative pain for humane animal welfare reasons as well as for reducing the likelihood of introducing confounding factors brought on by uncontrolled pain and distress that May influence the studies (21). In the context of multifaceted traumatic brain injury research using animal models, the type of analgesic administered is also a consideration due to potential effects on behavior, central nervous system cell types, and post-surgical recovery (25–31). As such, using the most appropriate analgesics for the species and study type is a prime concern when designing and executing animal research studies. While reducing animals’ pain is critical, the formulation of the chosen analgesic is also an important decision point. Historically, particularly with rodent studies, analgesic practices have not been well-documented in literature, so with recent advances in transparency, more potential insights May be gleaned, and methods could be further refined (50, 51). The current study underscores the importance of appropriate and well-informed analgesic selection to limit unintended impacts on laboratory animals and, consequently, on the experiments as a whole.
The raw data supporting the conclusions of this article will be made available by the authors, without undue reservation.
The animal study was approved by Institutional Animal Care and Use Committee at Virginia Commonwealth University. The study was conducted in accordance with the local legislation and institutional requirements.
RL: Data curation, Formal analysis, Investigation, Validation, Visualization, Writing – original draft, Writing – review & editing. MH: Funding acquisition, Investigation, Methodology, Writing – review & editing. CK: Investigation, Writing – review & editing. AL: Conceptualization, Formal analysis, Funding acquisition, Supervision, Writing – review & editing.
The author(s) declare that financial support was received for the research, authorship, and/or publication of this article. This work was funded by NINDS grants R01 NS096143 and F99 NS125825.
The authors would like to thank Dr. Krista Scoggins, DMV, for her guidance regarding the switch to bup-SR-HCl.
The authors declare that the research was conducted in the absence of any commercial or financial relationships that could be construed as a potential conflict of interest.
All claims expressed in this article are solely those of the authors and do not necessarily represent those of their affiliated organizations, or those of the publisher, the editors and the reviewers. Any product that may be evaluated in this article, or claim that may be made by its manufacturer, is not guaranteed or endorsed by the publisher.
The Supplementary material for this article can be found online at: https://www.frontiersin.org/articles/10.3389/fneur.2024.1467419/full#supplementary-material
1. James, SL, Theadom, A, Ellenbogen, RG, Bannick, MS, Montjoy-Venning, W, Lucchesi, LR, et al. Global, regional, and national burden of traumatic brain injury and spinal cord injury, 1990–2016: a systematic analysis for the Global Burden of Disease Study 2016. Lancet Neurol. (2019) 18:56–87. doi: 10.1016/S1474-4422(18)30415-0
2. Dewan, MC, Rattani, A, Gupta, S, Baticulon, RE, Hung, Y-C, Punchak, M, et al. Estimating the global incidence of traumatic brain injury. J Neurosurg. (2019) 130:1080–97. doi: 10.3171/2017.10.JNS17352
3. Guan, B, Anderson, DB, Chen, L, Feng, S, and Zhou, H. Global, regional and national burden of traumatic brain injury and spinal cord injury, 1990–2019: a systematic analysis for the Global Burden of Disease Study 2019. BMJ Open. (2023) 13:e075049. doi: 10.1136/bmjopen-2023-075049
4. McAllister, TW . Neurobehavioral sequelae of traumatic brain injury: evaluation and management. World Psychiatry. (2008) 7:3–10. doi: 10.1002/j.2051-5545.2008.tb00139.x
5. Bombardier, CH . Rates of major depressive disorder and clinical outcomes following traumatic brain injury. JAMA. (2010) 303:1938–45. doi: 10.1001/jama.2010.599
6. Mavroudis, I, Ciobica, A, Bejenariu, AC, Dobrin, RP, Apostu, M, Dobrin, I, et al. Cognitive impairment following mild traumatic brain injury (mTBI): a review. Medicina. (2024) 60:380. doi: 10.3390/medicina60030380
7. Arciniegas, DB, and Wortzel, HS. Emotional and behavioral dyscontrol after traumatic brain injury. Psychiatr Clin N Am. (2014) 37:31–53. doi: 10.1016/j.psc.2013.12.001
8. Starkstein, SE, and Pahissa, J. Apathy following traumatic brain injury. Psychiatr Clin N Am. (2014) 37:103–12. doi: 10.1016/j.psc.2013.10.002
9. Howlett, JR, Nelson, LD, and Stein, MB. Mental health consequences of traumatic brain injury. Biol Psychiatry. (2022) 91:413–20. doi: 10.1016/j.biopsych.2021.09.024
10. Nampiaparampil, DE . Prevalence of chronic pain after traumatic brain injury: a systematic review. JAMA. (2008) 300:711. doi: 10.1001/jama.300.6.711
11. Williams, GP, Schache, AG, and Morris, ME. Mobility after traumatic brain injury: relationships with ankle joint power generation and motor skill level. J Head Trauma Rehabil. (2013) 28:371–8. doi: 10.1097/HTR.0b013e31824a1d40
12. O’Neil, J, Egan, M, Marshall, S, Bilodeau, M, Pelletier, L, and Sveistrup, H. Remotely supervised exercise programmes to improve balance, mobility, and activity among people with moderate to severe traumatic brain injury: description and feasibility. Physiother Can. (2023) 75:146–55. doi: 10.3138/ptc-2021-0039
13. Gooijers, J, Chalavi, S, Beeckmans, K, Michiels, K, Lafosse, C, Sunaert, S, et al. Subcortical volume loss in the thalamus, putamen, and pallidum, induced by traumatic brain injury, is associated with motor performance deficits. Neurorehabil Neural Repair. (2016) 30:603–14. doi: 10.1177/1545968315613448
14. McMillan, TM, and Teasdale, GM. Death rate is increased for at least 7 years after head injury: a prospective study. Brain. (2007) 130:2520–7. doi: 10.1093/brain/awm185
15. Hukkelhoven, CWPM, Steyerberg, EW, Rampen, AJJ, Farace, E, Habbema, JDF, Marshall, LF, et al. Patient age and outcome following severe traumatic brain injury: an analysis of 5600 patients. J Neurosurg. (2003) 99:666–73. doi: 10.3171/jns.2003.99.4.0666
16. Seal, KH, Bertenthal, D, Barnes, DE, Byers, AL, Strigo, I, and Yaffe, K. Association of traumatic brain injury with chronic pain in Iraq and Afghanistan veterans: effect of comorbid mental health conditions. Arch Phys Med Rehabil. (2017) 98:1636–45. doi: 10.1016/j.apmr.2017.03.026
17. Russell, WMS, and Burch, RL. The Principles of Humane Experimental Technique. London: Methuen & Co. Ltd (1959). 289 p.
18. Flecknell, PA . The relief of pain in laboratory animals. Lab Anim. (1984) 18:147–60. doi: 10.1258/002367784780891226
19. Berry, SH . Analgesia in the perioperative period. Vet Clin N Am Small Anim Pract. (2015) 45:1013–27. doi: 10.1016/j.cvsm.2015.04.007
20. Ahmadi-Noorbakhsh, S, Farajli Abbasi, M, Ghasemi, M, Bayat, G, Davoodian, N, Sharif-Paghaleh, E, et al. Anesthesia and analgesia for common research models of adult mice. Lab Anim Res. (2022) 38:40. doi: 10.1186/s42826-022-00150-3
21. Flecknell, PA, and Thomas, AA. Comparative anesthesia and analgesia of laboratory animals In: Veterinary Anesthesia and Analgesia. Hoboken, New Jersey: John Wiley & Sons, Ltd (2015). 754–63.
22. Foley, PL, Liang, H, and Crichlow, AR. Evaluation of a sustained-release formulation of buprenorphine for analgesia in rats. J Am Assoc Lab Anim Sci. (2011) 50:198–204.
23. Herrmann, K, and Flecknell, P. Retrospective review of anesthetic and analgesic regimens used in animal research proposals. ALTEX. (2019) 36:65–80. doi: 10.14573/altex.1804011
24. Lewis, JW . Buprenorphine. Drug Alcohol Depend. (1985) 14:363–72. doi: 10.1016/0376-8716(85)90067-5
25. Avsaroglu, H, van der Sar, AS, van Lith, HA, van Zutphen, LFM, and Hellebrekers, LJ. Differences in response to anaesthetics and analgesics between inbred rat strains. Lab Anim. (2007) 41:337–44. doi: 10.1258/002367707781282811
26. Anderson, LM, Samineni, S, Wilder, DM, Lara, M, Eken, O, Urioste, R, et al. The neurobehavioral effects of buprenorphine and meloxicam on a blast-induced traumatic brain injury model in the rat. Front Neurol. (2021) 12:746370. doi: 10.3389/fneur.2021.746370
27. Reifenrath, J, Heider, M, Kempfert, M, Harting, H, Weidemann, F, Strauss, S, et al. Buprenorphine in rats: potent analgesic or trigger for fatal side effects? Acta Vet Scand. (2022) 64:37. doi: 10.1186/s13028-022-00661-y
28. Rasouli, HR, Mohammadian Salim, M, Talebi, S, Eslamian, M, and Ahmadpour, F. The efficacy of buprenorphine on moderate traumatic brain injury in the rat model. Neurol Res. (2023) 45:1055–62. doi: 10.1080/01616412.2023.2257447
29. Faulkner, MB, Rizk, M, Bazzi, Z, Dysko, RC, and Zhang, Z. Sex-specific effects of buprenorphine on endoplasmic reticulum stress, abnormal protein accumulation, and cell loss after pediatric mild traumatic brain injury in mice. Neurotrauma Rep. (2023) 4:573–85. doi: 10.1089/neur.2023.0051
30. Ryu, J, Stone, P, Lee, S, Payne, B, Gorse, K, and Lafrenaye, A. Buprenorphine alters microglia and astrocytes acutely following diffuse traumatic brain injury. Sci Rep. (2021) 11:8620. doi: 10.1038/s41598-021-88030-z
31. Ryu, J, Jeizan, P, Ahmed, S, Ehsan, S, Jose, J, Regan, S, et al. Post-injury buprenorphine administration is associated with long-term region-specific glial alterations in rats. Pharmaceutics. (2022) 14:2068. doi: 10.3390/pharmaceutics14102068
32. Percie du Sert, N, Hurst, V, Ahluwalia, A, Alam, S, Avey, MT, Baker, M, et al. The ARRIVE guidelines 2.0: Updated guidelines for reporting animal research. PLoS Biol. (2020) 18:e3000410. doi: 10.1371/journal.pbio.3000410
33. National Research Council (U.S.), Institute for Laboratory Animal Research (U.S.), National Academies Press (U.S.) ed. Guide for the care and use of laboratory animals. 8th ed. Washington, DC: National Academies Press (2011). 220 p.
34. Hernandez, ML, Marone, M, Gorse, KM, and Lafrenaye, AD. Cathepsin B Relocalization in Late Membrane Disrupted Neurons Following Diffuse Brain Injury in Rats. ASN Neuro. (2022) 14:17590914221099112. doi: 10.1177/17590914221099112
35. Hernandez, M, Regan, S, Ansari, R, Logan-Wesley, A, Lilova, R, Levi, C, et al. The effects of cathepsin B inhibition in the face of diffuse traumatic brain injury and secondary intracranial pressure elevation. Biomedicines. (2024) 12:1612. doi: 10.3390/biomedicines12071612
36. Dixon, CE, Lyeth, BG, Povlishock, JT, Findling, RL, Hamm, RJ, Marmarou, A, et al. A fluid percussion model of experimental brain injury in the rat. J Neurosurg. (1987) 67:110–9. doi: 10.3171/jns.1987.67.1.0110
37. Lafrenaye, AD, Krahe, TE, and Povlishock, JT. Moderately elevated intracranial pressure after diffuse traumatic brain injury is associated with exacerbated neuronal pathology and behavioral morbidity in the rat. J Cereb Blood Flow Metab. (2014) 34:1628–36. doi: 10.1038/jcbfm.2014.122
38. R Core Team . R: A Language and Environment for Statistical Computing. (2024). Available at: https://www.R-project.org/.
39. Catbagan, DL, Quimby, JM, Mama, KR, Rychel, JK, and Mich, PM. Comparison of the efficacy and adverse effects of sustained-release buprenorphine hydrochloride following subcutaneous administration and buprenorphine hydrochloride following oral transmucosal administration in cats undergoing ovariohysterectomy. Am J Vet Res. (2011) 72:461–6. doi: 10.2460/ajvr.72.4.461
40. Chum, HH, Jampachairsri, K, McKeon, GP, Yeomans, DC, Pacharinsak, C, and Felt, SA. Antinociceptive effects of sustained-release buprenorphine in a model of incisional pain in rats (Rattus norvegicus). J Am Assoc Lab Anim Sci. (2014) 53:193–7.
41. Mock, EJ, Kamel, F, Wright, WW, and Frankel, AI. Seasonal rhythm in plasma testosterone and luteinising hormone of the male laboratory rat. Nature. (1975) 256:61–3. doi: 10.1038/256061a0
42. Perissin, L, Facchin, P, and Porro, CA. Tonic pain response in mice: effects of sex, season and time of day. Life Sci. (2003) 72:897–907. doi: 10.1016/S0024-3205(02)02344-5
43. Kiank, C, Koerner, P, Keler, W, Traeger, T, Maier, S, Heidecke, C-D, et al. Seasonal variations in inflammatory responses to sepsis and stress in mice*. Crit Care Med. (2007) 35:2352–8. doi: 10.1097/01.CCM.0000282078.80187.7F
44. Ferguson, SA, and Maier, KL. A review of seasonal/circannual effects of laboratory rodent behavior. Physiol Behav. (2013) 119:130–6. doi: 10.1016/j.physbeh.2013.06.007
45. Suckow, MA, and Tirado-Muñiz, N. Seasonal variation of laboratory animals as a consideration for research reproducibility. Comp Med. (2023) 73:255–9. doi: 10.30802/AALAS-CM-23-000033
46. Cowan, A, Doxey, J, and Harry, E. The animal pharmacology of buprenorphine, an oripavine analgesic agent. Brit J Pharmacol. (1977) 60:547–54. doi: 10.1111/j.1476-5381.1977.tb07533.x
47. Shannon, HE . Stimulation of avoidance behavior by buprenorphine in rats. Psychopharmacology. (1983) 80:19–23. doi: 10.1007/BF00427487
48. Paronis, CA, and Holtzman, SG. Sensitization and tolerance to the discriminative stimulus effects of mu-opioid agonists. Psychopharmacology. (1994) 114:601–10. doi: 10.1007/BF02244991
49. Pechnick, RN, George, R, and Poland, RE. The effects of the acute administration of buprenorphine hydrochloride on the release of anterior pituitary hormones in the rat: Evidence for the involvement of multiple opiate receptors. Life Sci. (1985) 37:1861–8. doi: 10.1016/0024-3205(85)90002-5
50. Richardson, CA, and Flecknell, PA. Anaesthesia and post-operative analgesia following experimental surgery in laboratory rodents: are we making progress? Altern Lab Anim. (2005) 33:119–27. doi: 10.1177/026119290503300207
Keywords: buprenorphine slow-release LAB, buprenorphine slow-release HCl, traumatic brain injury, rats, weight loss, humane endpoints
Citation: Lilova RL, Hernandez M, Kelliher C and Lafrenaye A (2024) Increased incidence of weight-loss-associated humane endpoints in rats administered buprenorphine slow-release LAB formulation following traumatic brain injury: a retrospective study. Front. Neurol. 15:1467419. doi: 10.3389/fneur.2024.1467419
Received: 19 July 2024; Accepted: 11 September 2024;
Published: 23 September 2024.
Edited by:
Deborah Shear, Walter Reed Army Institute of Research, United StatesReviewed by:
Bruce G. Lyeth, University of California, Davis, United StatesCopyright © 2024 Lilova, Hernandez, Kelliher and Lafrenaye. This is an open-access article distributed under the terms of the Creative Commons Attribution License (CC BY). The use, distribution or reproduction in other forums is permitted, provided the original author(s) and the copyright owner(s) are credited and that the original publication in this journal is cited, in accordance with accepted academic practice. No use, distribution or reproduction is permitted which does not comply with these terms.
*Correspondence: Audrey Lafrenaye, QXVkcmV5LkxhZnJlbmF5ZUB2Y3VoZWFsdGgub3Jn
Disclaimer: All claims expressed in this article are solely those of the authors and do not necessarily represent those of their affiliated organizations, or those of the publisher, the editors and the reviewers. Any product that may be evaluated in this article or claim that may be made by its manufacturer is not guaranteed or endorsed by the publisher.
Research integrity at Frontiers
Learn more about the work of our research integrity team to safeguard the quality of each article we publish.