- 1Department of Rehabilitation, Tianjin Medical University General Hospital, Tianjin, China
- 2Department of Imaging, Tianjin Medical University General Hospital, Tianjin, China
Introduction: Repetitive transcranial magnetic stimulation (rTMS) can improve post stroke motor function. However, there is little research on targets. The purpose of this study is to investigate the effects of rTMS therapy with different targets on post stroke motor function and neural plasticity.
Methods: Fifty-four subjects were randomly divided into M1 (Primary motor area) group, SMA (supplementary motor area) group and Sham group, and were given 10 Hz on the affected M1 area, SMA area and sham stimulation rTMS. The primary outcomes included Fugl-Meyer Assessment Upper Extremity Scale (FMA-UE), Fugl-Meyer Assessment Lower Extremity Scale (FMA-LE) and Berg balance scale (BBS). Secondary outcomes: amplitude of low frequency fluctuation (ALFF), regional homogeneity (ReHo) and functional connectivity (FC) were analyzed by functional magnetic resonance imaging (fMRI) to evaluate brain functional activation and functional connectivity changes.
Results: The 2-way repeated-measures ANOVA revealed a significant group × time interaction (F = 23.494, p < 0.001; F = 10.801, p < 0.001; F = 17.812, p < 0.001) in the FMA-UE, FMA-LE and BBS scores. Post hoc analysis indicated that 4 weeks of SMA rTMS resulted in an increase in FMA-UE, FMA-LE and BBS scores compared with Sham group (p = 0.006; p = 0.033; p = 0.012), SMA group was significantly increased in BBS compared with M1 group (p = 0.034). Moreover, there were significant effects of time in all 3 groups in the FMA-UE, FMA-LE and BBS scores (p < 0.001). In addition, the increase of ALFF in the supramarginal gyrus on the affected side was correlated with better FMA-UE recovery, the increase of ALFF in the middle temporal gyrus and the middle frontal gyrus on the affected side was positively correlated with the improvement of BBS, and the ALFF in the cerebellum on the healthy side was negatively correlated with the improvement of BBS. There was a positive correlation between FC (SMA – ipsilateral cerebellum) changes and BBS changes in SMA group.
Discussion: In conclusion, SMA-rTMS intervention has a better recovery effect on motor dysfunction after stroke than Sham-rTMS. SMA-rTMS led to similar improvement on motor function but significantly greater improvement on balance compared to M1-rTMS, and this may pave a new way for stroke rehabilitation.
Clinical trial registration: Registration number: ChiCTR2200060955, https://www.chictr.org.cn/.
1 Introduction
Stroke has become the main cause of morbidity and mortality and is showing a trend toward younger ages of onset (1, 2). Approximately 70 to 80% of patients with stroke experience varying degrees of functional impairment, with 80% of patients experiencing motor dysfunction, which is the main cause of disability (3). Research shows that the rate of cerebral nerve tissue repair is the fastest from a few hours to approximately 10 weeks after stroke and then gradually slows down and reaches a plateau 3 months after stroke (4, 5). The early stage of subacute phase is the golden period for functional recovery, and most clinical rehabilitation patients are also in this stage. Therefore, the first step is to clarify the changes in brain function of patients in this stage, which is the basis for subsequent interventions. Personalized rehabilitation interventions (including physical and occupational therapy) are the gold standard for promoting poststroke motor function recovery (2).
The nervous system forms a large and complex neural network through self-regulation and repair. The neural network can continuously undergo functional reorganization through external stimuli or intrinsic regulation, triggering a series of cellular and other biological processes after stroke, leading not only to necrosis but also to synaptogenesis and axon sprouting (6), laying the foundation for rehabilitation. Recently, transcranial magnetic stimulation (TMS) has become a new safe method for regulating cortical excitability, improving motor function in stroke patients by increasing motor output. As one of the modes, rTMS regulates neural plasticity and promotes neural repair by modulating the excitability and neurobiological function of the cerebral cortex. It has obtained “A-level evidence” in the neurorehabilitation application of motor function after stroke (7). However, there is still a lack of evidence for targeted stratification and individual treatment. In previous studies, M1 and cerebellum were the main targets for treating post-stroke motor function (8–10). The supplementary motor cortex (SMA), as an important motor related brain area, plays an important role in movement preparation, control and coordination (11), and is involved in continuous movement pattern programming, connecting primary motor cortex and spinal cord, and participating in movement execution (12). Previous studies have found that SMA plays an important role in the recovery of balance function and gait after stroke (13, 14). However, few studies have considered SMA as the target of rTMS (15), especially in the systematic comparison between M1 rTMS and SMA rTMS.
In recent years, the widespread application of functional magnetic resonance imaging (fMRI) has further advanced clinical research on brain function. As a non-invasive brain imaging method, it can detect the functional connections between various brain regions connected by synapses, and detect the plasticity between networks (11), which is expected to become a biomarker for precision medicine. In this study, we measured three rs-fMRI-based markers to predict local brain neural activity and functional connectivity; these measures comprised amplitude of low-frequency fluctuation (ALFF), regional homogeneity (ReHo), and functional connectivity (FC).
In condition, previous studies have shown that rTMS is helpful in improving motor dysfunction after stroke (16–18), but the possible mechanisms of stimulation parameters, targets, and neural remodeling are still unclear (19–21). In this study, based on the classic “interhemispheric competition model”, we administered high-frequency stimulation of 10 Hz to the affected side to correct the imbalance of excitability between hemispheres and promote brain function remodeling. The focus of this study is to use fMRI to assess the differences between M1 and SMA as different stimulation targets, aiming to identify more precise and optimized action targets, further optimize rehabilitation treatment plans, and refine rehabilitation treatment.
2 Methods
2.1 Study population
In this randomized controlled trial, 54 patients with cerebral infarction admitted to the Department of Rehabilitation Medicine of Tianjin Medical University General Hospital from June 27, 2022, to June 30, 2023, were selected as the study subjects. The inclusion criteria were as follows: ① Patients with subcortical ischemic stroke who met the diagnostic criteria specified in the “Key Diagnostic Points for Various Major Cerebrovascular Diseases in China 2019” formulated by the Neurology Branch of the Chinese Medical Association and have been confirmed by head spiral CT or head magnetic resonance imaging; ② First stroke, with motor dysfunction (FMA-UE<32, FMA-LE<18, BBS<20); ③ Course of disease: 2–12 weeks; ④ Right-handed; ⑤ No gender restrictions, age: 40–80 years; ⑥ Stable vital signs, clear consciousness; ⑦ MMSE (mini-mental state examination) > 21 points; and ⑧ Signed informed consent.
The exclusion criteria (15) were as follows: ① Unstable condition and secondary stroke; ② Previous peripheral nerve injury or peripheral neuropathy on the affected side; ③ Previous individua history or family history of epilepsy; ④ Severe anxiety or depression symptoms requiring control with medication; ⑤ Severe heart, lung, liver, kidney or other organ diseases; ⑥ Presence of a pacemaker, cochlear implant, or skull repair plate in the body; and ⑦ Claustrophobia.
The discontinuation and dropout criteria were as follows: ① Progressive worsening of condition or other newly diagnosed diseases rendering the patient unable to continue treatment; ② Severe adverse reactions or inability to tolerate rTMS during treatment; and ③ Requests by subjects to withdraw for their own reasons.
All participants provided written informed consent according to the Declaration of Helsinki before the experiment, as approved by the ethics committee of the hospital (approval number: IRB2022-YX-054-01), and registered in the Chinese Clinical Trial Registry (registration number: ChiCTR2200060955 at 2022-06-14).
2.1.1 Trial inclusion
Due to the epidemic, there were 2, 1, and 3 subjects in the three groups who did not complete the entire study process. Finally, 16 cases, 17 cases, and 15 cases from the three groups completed this study (Figure 1).
2.2 Research design
2.2.1 Randomization and blinding
After enrollment, a simple randomization method was used, and the sample size was input into a simple statistical software program to obtain a random number table. According to the order in which the participants entered the experiment and the corresponding groups of characters in the random number table, they were randomly divided into the M1 group, SMA group, and Sham group in a 1:1:1 ratio. The intervention was transferred to the ipsilateral M1 (10 Hz) or SMA (10 Hz), or sham stimulation, respectively. The results of randomization were kept in randomly arranged opaque envelopes. Outcome measures were assessed by a physical therapist who was unaware of patient assignments. The therapists who performed physical therapy were also blinded. However, the therapist operating the magnetic stimulator was not blinded.
All three groups received the same routine rehabilitation training, including acupuncture, PT, and OT. Each rTMS intervention was preceded by routine rehabilitation as described above.
2.2.2 rTMS protocol determination of simulation regions
The intervention was provided by a physiotherapist who was well trained in TMS. All subjects received rTMS treatment or sham stimulation therapy using a Mag TD 100 Hz magnetic field stimulator (YIRUIDE Medical Co., Wuhan, China). Before the first treatment, determine the resting motion threshold (RMT) of the healthy abductor pollicis brevis muscle (22, 23): the minimum stimulation intensity that causes slight contraction of the healthy abductor pollicis brevis muscle in 5 out of 10 single pulse transcranial magnetic stimulation in the brain (24). Using the 10–10 international EEG standard to locate stimulation targets (M1: midpoint of the line connecting CPz to C3 or C4; SMA: FC1 or FC2; Sham: Midpoint of Pz and C3/C4) (25). During treatment, the subjects were placed in a comfortable sitting or supine position, and a “8 coil” was placed on the stimulation target. During treatment, the coil was tangent to the surface of the skull, and the parameters were as follows: stimulation frequency: 10 Hz, intensity: 100% RMT, stimulation time: 2.5 s, interval time: 10 s, total duration: 20 min, total pulse: 2400, once a day, 5 days a week, lasting for 4 weeks. The sham group received rTMS sham stimulation.
2.3 Outcome
2.3.1 Primary outcome
FMA-UE (26) assesses the motor function of the upper limbs (a total of 33 items, a total score of 66 points, and the lower the score, the more severe the injury).
FMA-LE (27) assesses the motor function of the lower limbs (a total of 17 items, a total score of 34 points, and the lower the score, the more severe the injury).
BBS (28) is used to assess activity limitations in daily life that require balance (a total of 14 items with a total score of 56, with higher scores indicating better balance. 0–20 points: poor balance and need to use a wheelchair; 21–40 points: some balance ability, walking with assistance; 41–56: good balance and able to walk independently).
2.3.2 Secondary outcomes
fMRI scans were performed before and after intervention. ALFF was used to evaluate the changes in spontaneous activity of brain neurons (the higher the ALFF value, the stronger the spontaneous activity of brain neurons, and vice versa). ReHo was used to evaluate the synchronicity of neuronal activity in functional areas of the brain (the higher the ReHo value, the greater the synchronicity of neuronal activity in the brain region, and vice versa, the synchronicity tends to be disordered). FC was used to evaluate changes in functional connectivity between brain regions (the higher the FC value, the stronger the functional connectivity between brain regions, and vice versa, the weaker).
2.3.2.1 MRI image acquisition and data processing
rs-fMRI data were obtained using the 3.0 T Siemens MRI scanner and 64 channel magnetic head coils. All subjects’ heads were fixed with customized foam pads to reduce head movement. High resolution gradient-echo echo-planar imaging (GRE-EPI) was used to scan functional imaging in resting-state fMRI to observe the spontaneous functional activity of the brain in the resting state and the functional connectivity between brain regions. The parameters were as follows: repetition time: 800 ms; echo time: 30 ms; flip angle: 56; FoV 100× 100 mm2; matrix size: 104 × 104; slice thickness: 1 mm; number of slices: 72; total time: 8′39″. During the functional magnetic resonance imaging scan, the subjects were instructed to relax, remain stationary, and close their eyes.
MATLAB (R2014b, MathWorks, Natick, MA, United States) software and DPARSF1 were used to preprocess the data. The data from the first 20 time points for each participant were removed to avoid scanner instability and allow subjects to adapt to scanner noise, and the data from the remaining 430 time points were preprocessed. Before data preprocessing, the images of lesions located in the left hemisphere were reversed to the right, with the right hemisphere uniformly defined as the affected hemisphere and the left hemisphere as the healthy hemisphere. Pretreatment included slice-timing correction, head movement correction, spatial standardization, spatial smoothing, removal of linear drift, low-frequency filtering (0.01 ~ 0.1 Hz) and removal of physiological confounding factors (head movement, whole brain signal, white matter signal and cerebrospinal fluid signal). For a given voxel, the time-dependent data were converted to the frequency domain using a fast Fourier transform. ALFF and ReHo were calculated within the range of 0.01 to 0.1 Hz for each voxel. Finally, all ALFF and ReHo images were smoothed spatially using a 4 mm full width at half maximum Gaussian kernel.
Based on the selection of the M1 and SMA of the cerebral cortex as intervention targets, to observe the changes in functional connectivity with other brain regions after intervention, we selected the left and right M1 and SMA (Supplemental material S1) as seed points for FC analysis and analyzed the correlation between seed points and total brain elements at the voxel level. Finally, the FC values obtained from the correlation analysis were normalized using the Fisher z-transform, and a z-value map of the entire brain was created.
2.4 Statistical analysis
This study was a randomized controlled trial, and the three groups were M1 group, SMA group, and Sham group. The FMA-UE, FMA-LE, and BBS scales were used as the primary clinical outcome indicators for sample size estimation. According to the results of the preliminary experiment, let a = 0.05, 1-β = 80%, One-way analysis of Variance F-Tests under the Means menu of PASS 2021 software were used to calculate the FMA-UE, FMA-LE and BBS. The minimum total sample size for the three groups was N = 48. Taking into account the cases of loss to follow-up and refusal to follow-up according to 10%, 48 × (1 + 0.1) = 53 cases, so a total of 54 cases were drawn up in the three groups, 18 cases in each group.
SPSS 27.0 statistical analysis software was used for data processing, and (𝑥̅±𝑠) was used for quantitative data analysis. Paired sample t tests were used to compare pre- and post-treatment data within groups. A 2-way repeated-measures analysis of variance was used to analyze the group × time interaction. The least significant difference (LSD) test was used for the subsequent comparison between the two groups. When p < 0.05, differences were considered statistically significant. SPM12 software was used to perform dual sample t tests, paired t tests, and one-way ANOVA on ALFF, ReHo, and FC values to obtain significant differences (qFDR corr. < 0.05). Pearson correlation analysis was used to measure the relationship between primary outcomes and imaging markers. The results were visualized using Xjview software.
3 Results
3.1 Baseline clinical characteristics and demographic data
No significant differences were found at baseline in the demographic or clinical evaluation data of the three groups of patients (p > 0.05) (Table 1).
3.2 Functional evaluation
The 2-way repeated-measures ANOVA revealed a significant group × time interaction (F = 23.494, p < 0.001; F = 10.801, p < 0.001; F = 17.812, p < 0.001) in the FMA-UE, FMA-LE and BBS scores. Post hoc analysis indicated that 4 weeks of SMA rTMS resulted in an increase in FMA-UE, FMA-LE and BBS scores compared with Sham group (p = 0.006; p = 0.033; p = 0.012). The SMA group also showed significantly increased in BBS compared with M1 group (p = 0.034). Moreover, there were significant effects of time in all 3 groups in the FMA-UE, FMA-LE and BBS scores (pa,b,c < 0.001) (Figure 2).
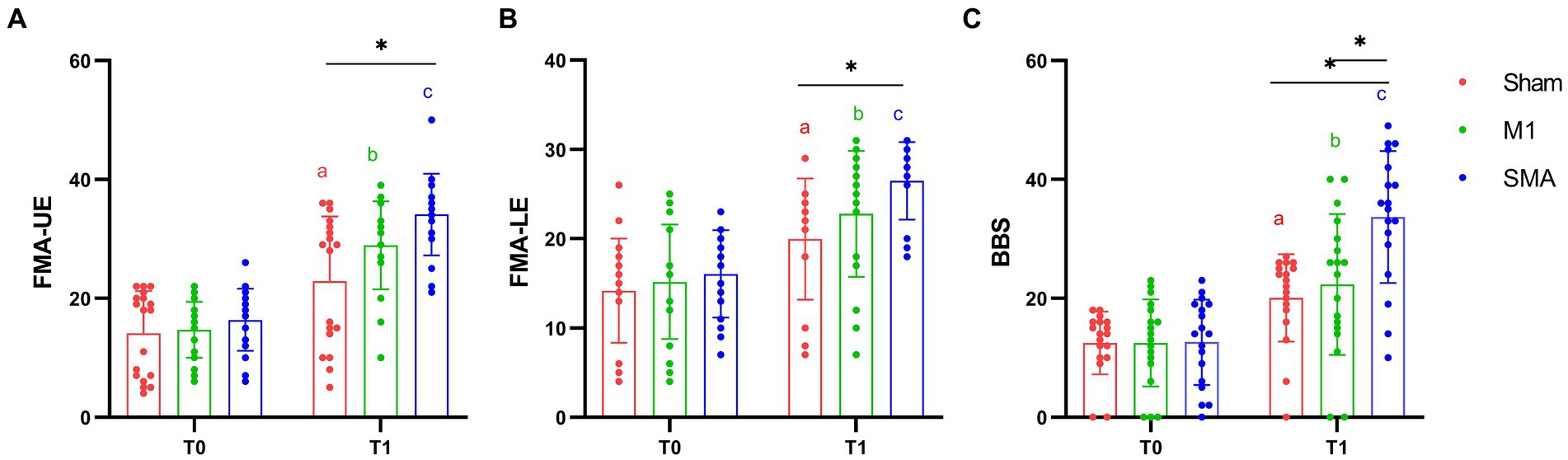
Figure 2. Motor function assessment. (A) FMA-UE assessment; (B) FMA-LE assessment; (C) BBS assessment. T0: baseline, T1:4W. *: p < 0.05, **: p < 0.01. a, b, and c: Significance of paired t-test before and after intervention (Pa,b,c < 0.001).
3.3 Local activation of brain networks
3.3.1 Comparison of three groups of ALFF and ReHo before and after treatment
Compared ALFF before and after treatment, the M1 group showed an increase in the right superior marginal gyrus, anterior cuneiform lobe, and postcentral gyrus. The difference between the right superior marginal gyrus and anterior cuneiform lobe was statistically significant (qFDR corr. < 0.05); the left rectus gyri, the right dorsolateral superior frontal gyrus, and the middle occipital gyrus decreased, with significant differences in the right dorsolateral superior frontal gyrus (qFDR corr. < 0.05) (Figure 3A). The SMA group showed an increase in the right middle temporal gyrus, postcentral gyrus, inferior parietal angular gyrus, middle frontal gyrus, precentral gyrus, left anterior cuneiform lobe, and left and right supplementary motor areas, with significant differences in the right middle temporal gyrus, inferior parietal angular gyrus, and middle frontal gyrus (qFDR corr. < 0.05);There was a decrease in the left cerebellum, superior temporal gyrus, precentral gyrus, postcentral gyrus, fusiform gyrus, insula, cortex around the right calcarine fissure, and the right anterior cingulate and paracingulate gyrus, with significant differences in the left cerebellum, superior temporal gyrus, fusiform gyrus, and insula (qFDR corr. < 0.05) (Figure 3B). No significant changes were found in the brain regions of the Sham group. The change of ALFF in the right supramargial gyrus (affected side) was positively correlated with the change of FMA-UE in the M1 group (Figure 3C) and SMA group (Figure 3D); and the increase of ALFF in the right middle temporal gyrus (affected side) was positively correlated with the improvement of BBS in the SMA group (Figure 3E). There was a negative correlation between the change of ALFF in the left (contralateral) cerebellum and the improvement of BBS in the SMA group (Figure 3F).
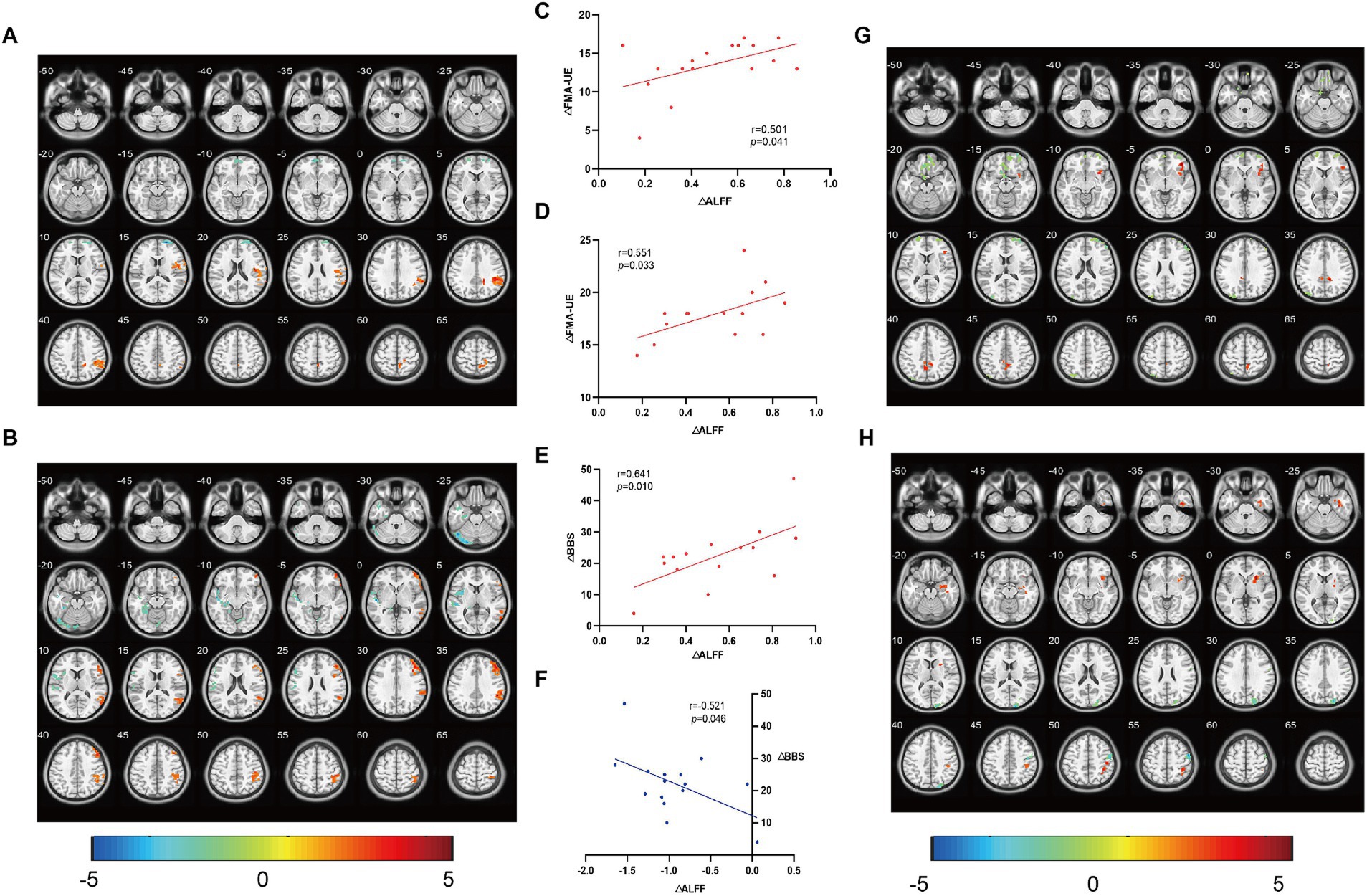
Figure 3. Comparison of ALFF/ReHo and correlation analysis. The changes of ALFF in brain regions before and after treatment and their correlation with clinical evaluation were analyzed. (A,G) brain regions with ALFF and ReHo changes in M1 group, and (B,H) brain regions with ALFF and ReHo changes in SMA group. (C) The change of ALFF in the right supramargial gyrus (affected side) was positively correlated with the change of FMA-UE in the M1 group, (D) the change of ALFF in the right supramargial gyrus (affected side) was positively correlated with the change of FMA-UE in the SMA group, and (E) the increase of ALFF in the right middle temporal gyrus (affected side) was positively correlated with the improvement of BBS in the SMA group. (F) There was a negative correlation between the change of ALFF in the left (contralateral) cerebellum and the improvement of BBS in the SMA group. The color bar represents the intensity of ALFF/ReHo changes in a given brain area.
Compared ReHo before and after treatment, the M1 group showed an increase in the left and right cerebellum, right insula, supramarginal gyrus, anterior cuneiform gyrus, and left inferior parietal angular gyrus. Among them, the difference between the right insula and anterior cuneiform gyrus was significant (qFDR corr. < 0.05); The right inferior temporal gyrus, left rectus gyrus, right superior frontal gyrus, and left postcentral gyrus decreased, while the left rectus gyrus and right superior frontal gyrus showed significant differences compared to before (qFDR corr. < 0.05) (Figure 3G); The SMA group showed an increase in the right hippocampus, putamen, insular inferior frontal gyrus, inferior parietal lobule, precentral gyrus, postcentral gyrus, left and right supplementary motor area, left middle frontal gyrus, and anterior cuneus, with a significant increase in the right hippocampus, putamen, and inferior parietal lobule (qFDR corr. < 0.05); The left cerebellum and right occipital gyrus decreased, while the difference in the right occipital gyrus was significant (qFDR corr. < 0.05) (Figure 3H). No significant differences were found in the Sham group. No correlation was found between ReHo changes and functional improvement.
3.3.2 Comparison of ALFF and ReHo values among the M1 group, SMA group, and Sham group after treatment
The ALFF in the M1 group was significantly higher in the right marginal gyrus than in the Sham group (Figure 4A); the SMA group showed significant elevation in the right middle temporal gyrus (Figure 4B). After treatment, compared with the M1 group, the SMA group showed a significant decrease in ALFF in the left Precuneus, while the left superior marginal gyrus and middle frontal gyrus showed a significant increase (Figure 4C), and ReHo decreased in the cingulate gyrus and increased in the left parietal lobe and angular gyrus (Figure 4D) (post hoc t test, p < 0.05, FDR corrected at cluster level). Correlation analysis showed that the ALFF of the right supramarginal gyrus was positively correlated with FMA-UE (M1 group and Sham group, r = 0.653, p < 0.000, Figure 4E), the right middle temporal gyrus was positively correlated with BBS (SMA group and Sham group, r = 0.530, p = 0.002, Figure 4F), and the left middle frontal gyrus was positively correlated with BBS (M1group and SMA group, r = 0.407, p = 0.019, Figure 4G).
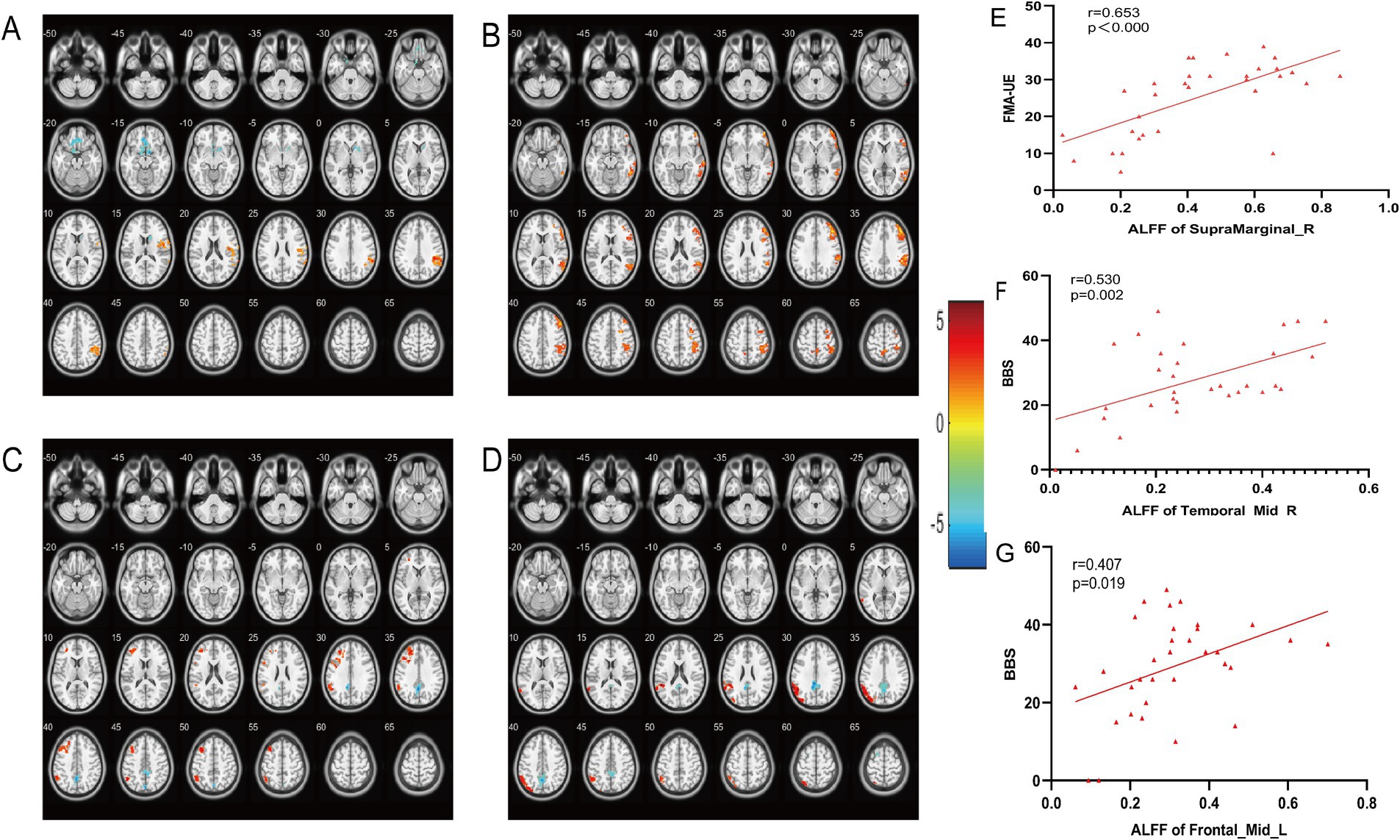
Figure 4. Comparison of brain regions with changes in ALFF and ReHo among the three groups after treatment. (A) Changing Brain changes in ALFF after treatment in (M1 group vs. Sham group). (B) Changing Brain changes in ALFF after treatment in (SMA group vs. Sham group). (C) Changing Brain changes in ALFF after treatment in (M1 group vs. SMA group). (D) Changing Brain changes in ReHo after treatment in (M1 group vs. SMA group). The color bar on the right represents the activation intensity of brain regions. (E–G) Correlation analysis between ALFF changes in brain regions and motor function scores.
3.4 Functional connection
After treatment, the functional connection between the right supplementary motor area and the right cerebellar hemisphere in the SMA group was enhanced compared to that in the M1 group (voxel: 96, T = 5.0161, qFDR corr. < 0.05); FC in the ipsilateral parietal lobe weakened (voxel: 97, T = -4.1696, qFDR corr. < 0.05). Correlational analysis showed that changes in resting-state functional connectivity between the right supplementary motor area and the right cerebellum were correlated with improvements in BBS scores (r = 0.530, p = 0.029) (Figure 5). No significant correlation was observed between resting-state functional connectivity and other clinical evaluations.
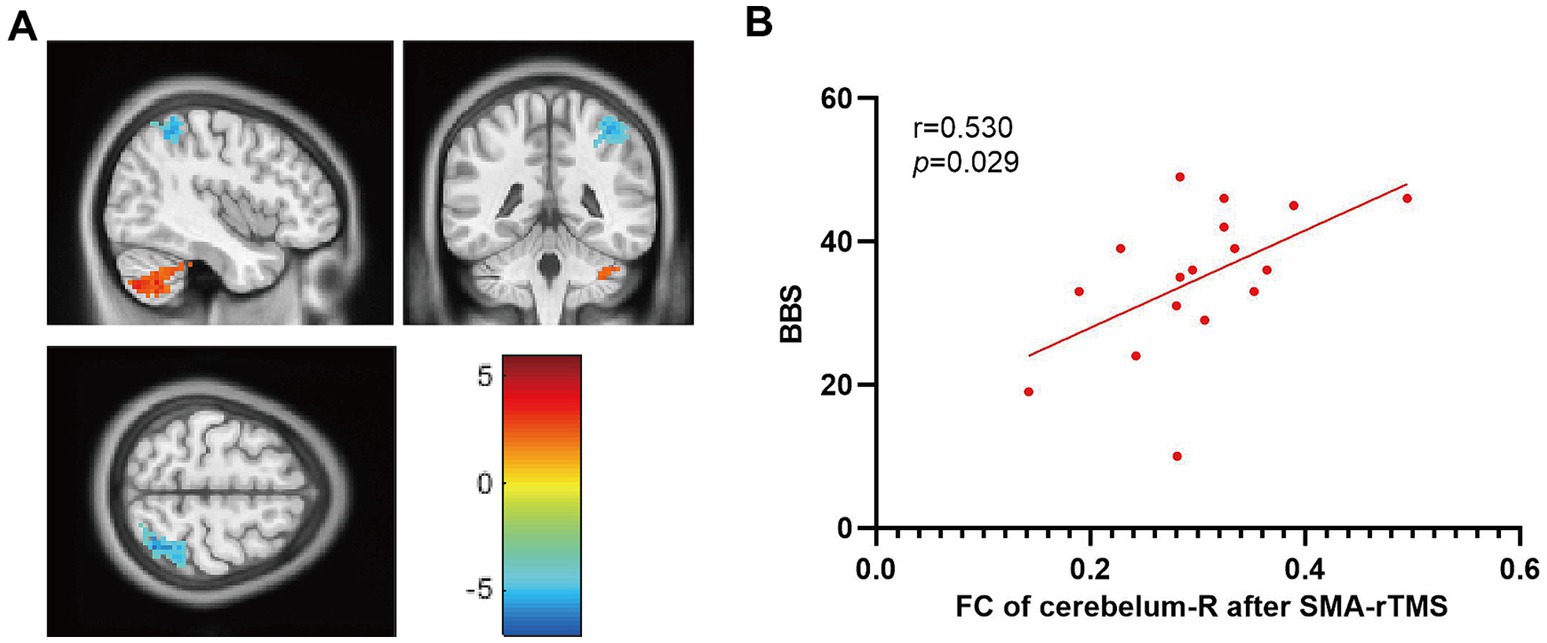
Figure 5. Comparison of functional connectivity between the M1 and SMA groups after treatment. (A) FC changes in brain regions, (B) Correlation between FC changes and BBS. The color bar on the right represents the intensity of brain activation.
4 Discussion
This randomized, double-blind, controlled clinical trial is the first to systematically compare the effects of high-frequency rTMS targeting the M1 and SMA in the affected cerebral hemisphere on motor function after stroke. Our results showed significant improvements in limb motor function (FMA-UE and FMA-LE scores) and balance function (BBS) in all three groups. Our findings further demonstrate that SMA-rTMS significantly enhances motor function in patients, encompassing improvements in limb movement and balance, when compared to the Sham group. Notably, the recovery of balance function following 4 weeks of SMA-rTMS intervention is markedly superior to that observed with M1-rTMS. Additionally, we identified a positive correlation between local activation in the middle temporal gyrus (SMA and Sham groups) and middle frontal gyrus (M1 and SMA groups) with the recovery of balance function; conversely, local activation in the contralateral cerebellum (SMA group) was found to hinder this recovery. The functional connectivity between SMA and the ipsilateral cerebellum (SMA group) appears to facilitate balance recovery. Furthermore, local activation within the supramarginal gyrus (three groups) positively correlates with enhancements in upper limb functionality.
4.1 Effect of rTMS on clinical motor function in stroke patients
Research has shown (29–32)that TMS has a significant effect on the improvement of motor function after stroke; for example, it can significantly improve the recovery of motor functions such as upper and lower limb movement, balance function, and gait after stroke. This study obtained similar results, with significant improvements in limb movement (FMA-UE, FMA-LE) and balance function (BBS) in stroke patients after high-frequency (10 Hz) rTMS intervention compared to the control group and before intervention. This study is the first to conduct a randomized controlled study on different targets (M1, SMA), The results showed that compared to the sham rTMS, both M1-rTMS and SMA-rTMS significantly improved motor function and balance measured by FM and BBS. Further, SMA-rTMS led to similar improvement on motor function but significantly greater improvement on balance compared to M1-rTMS.
4.2 Clinical function and brain activity
rs-fMRI is widely used and has become a source of biomarkers for the study of complex brain networks after stroke (33–36). ALFF (Amplitude of Low Frequency Fluctuations) is a method to measure local spontaneous brain activity, which reflects the activity intensity of local brain regions by analyzing the amplitude of low-frequency oscillation signals in gray matter at rest. ALFF mainly focuses on the amplitude of low-frequency oscillations in voxels, reflecting the local spontaneous activity in the resting state. In addition, ReHo (Regional Homogeneity) is a method to measure the homogeneity of neural activity in a local brain region, which evaluates the activity pattern of a local brain region by analyzing the similarity of neural activity between adjacent voxels. ReHo reflects the regional homogeneity of neural activity between adjacent voxels and is often used to assess concordant activity in local brain regions. Previous studies (19, 37, 38) have found that the excitability (ALFF, ReHo) of the lesion hemisphere after stroke was significantly reduced compared to that of healthy controls, while that of the contralateral hemisphere was significantly increased. This may be due to the delayed effects of injury on the affected hemisphere, which leads to a reduction in the inhibitory effect of the affected hemisphere on the healthy hemisphere and the enhancement of excitability on the healthy side. In turn, the excessive inhibitory effect of the healthy side on the affected side via the corpus callosum further aggravates the injury of the affected side (39, 40). After rTMS intervention, we observed that the excitability of the affected hemisphere was significantly increased and that of the contralateral hemisphere was decreased, indicating that rTMS played an important role in regulating the functional balance of the cerebral hemisphere.
On this basis, we conducted a correlation study between brain area activation and motor function score and found that there was a negative correlation between the changes in the activation level of the contralateral cerebellum (ALFF) and balance function score (BBS) after stroke. We hypothesized that too strong activation of unilateral cerebellum may be detrimental to the recovery of balance function after stroke. Considering that balance is dominated by the bilateral cerebellum, the activation of the bilateral cerebellum tending to a steady state may be more conducive to the recovery of balance function. The activation level of the affected cerebellum was not significantly enhanced compared with before, but the activation level of the whole affected hemisphere was generally increased after the intervention. It is speculated that the affected cerebellum may not show significant changes due to the problem of sample size. In the future, we will expand the sample size to continue studying this interesting finding.
In addition, the activation and retention (ALFF) of the middle temporal gyrus on the affected side was positively correlated with balance function (BBS), suggesting the role of the middle temporal gyrus in balance function. The temporal lobe is surrounded by the frontal, parietal, and occipital lobes and has extensive connections with other brain regions, making its functions complex. The middle temporal gyrus is located in the middle of the temporal lobe and plays an important role in brain function. Some studies have shown (41) that the middle temporal gyrus has a greater impact on trunk ataxia, and this study also confirmed that the retention of middle temporal gyrus function has an impact on balance function.
The present study found significant activation of the supramarginal gyrus on the affected side in the M1, SMA and Sham groups, and it was found to correlate with the degree of recovery of upper limb dysfunction. The supramarginal gyrus is located in the front of the inferior parietal lobule. Some studies have shown that (42, 43) after TMS intervention, the local activity of the parietal cortex is related to improvements in motor function and cerebral cortex reorganization after stroke. The supramarginal gyrus, located in the secondary somatosensory cortex, responds to physical stimuli and completes the task of structural differentiation. It is an important center responsible for fine motor coordination as well as complex athletic and occupational skills (42, 44). This study also confirmed its correlation with upper limb movement. In addition, the closer connection between the SMA and other brain regions has also been confirmed. Correlation analysis revealed that there was a positive correlation between the activity of the affected temporal lobe nerve and the preservation of balance function after stroke. After 10 Hz rTMS intervention on the affected side, the same results were found in the SMA group. The enhancement of neural activity in the cerebellum and middle temporal gyrus was positively correlated with the balance function score.
4.3 Clinical function and brain functional connectivity
Seed-based FC (seed-based FC) is a common method for functional connectivity analysis, which can be used to explain the functional connectivity correlation between the brain area of interest and other regions of the brain (45, 46). After intervention, the SMA group showed a significant increase in functional connectivity between the affected SMA brain area and the ipsilesional cerebellum compared to the M1 group, and it was found to be positively correlated with the recovery of balance function (BBS). This indicates that SMA-targeted rTMS stimulation can regulate the coupling between the SMA and the ipsilateral cerebellum and once again confirms that the cerebellum is more closely connected to the SMA than to M1.
The reorganization of brain function after rTMS intervention is a key step in rehabilitation (47). In this analysis of resting state functional connectivity, we observed a significant enhancement in SMA cerebellar functional connectivity, which is correlated with improvement in balance function. In theory, there is also a widespread association between M1 and cerebellar functional connectivity, but unfortunately, we did not observe this phenomenon in this study. Considering that SMA and cerebellum may have a broader connection through the cortical pontine cerebellar tract or cerebellar thalamic cortical pathway (48, 49). M1 may mainly affect motor function through the corticospinal tract, involving limb muscle strength and endurance. The FC between M1 and cerebellum showed an increasing trend in this study, but no significant difference was found. The sample size will be further expanded to verify this result.
4.4 Limitations of this study
Due to limited time and the COVID-19 the collected samples are not large enough, and the results may be biased. In addition, the image reversal process may have allowed lateralized features of left and right hemisphere stroke injuries to go unnoticed. Therefore, in future research, we need to compare studies conducted on diverse scales, at various centers, and with multiple time points to verify or correct the results of the present study.
5 Conclusion
Our findings demonstrate that SMA-rTMS significantly enhances motor function in patients, encompassing improvements in limb movement and balance, when compared to the Sham group. Notably, the recovery of balance function following 4 weeks of SMA-rTMS intervention is markedly superior to that observed with M1-rTMS. Therefore, SMA-rTMS may provide a new way for the rehabilitation of motor function in patients after stroke.
Data availability statement
The raw data supporting the conclusions of this article will be made available by the authors, without undue reservation.
Ethics statement
The studies involving humans were approved by Ethics Committee of Tianjin Medical University General Hospital (Approval number: IRB2022-YX-054-01). The studies were conducted in accordance with the local legislation and institutional requirements. The participants provided their written informed consent to participate in this study. Written informed consent was obtained from the individual(s) for the publication of any potentially identifiable images or data included in this article.
Author contributions
LZ: Data curation, Investigation, Methodology, Validation, Visualization, Writing – original draft. LC: Investigation, Writing – original draft. CYW: Methodology, Writing – original draft. SL: Investigation, Writing – original draft. CXW: Conceptualization, Funding acquisition, Project administration, Resources, Supervision, Writing – review & editing.
Funding
The author(s) declare that financial support was received for the research, authorship, and/or publication of this article. This work was supported by the Key Discipline of Tianjin Health Science and Technology Project (TJWJ2022XK007), Tianjin Key Medical Discipline (Specialty) Construction Project (TJYXZDXK-060B), and “Double First-Class” Discipline Construction Project.
Acknowledgments
We would like to thank all the participants of this project and investigators for collecting the data.
Conflict of interest
The authors declare that the research was conducted in the absence of any commercial or financial relationships that could be construed as a potential conflict of interest.
Publisher’s note
All claims expressed in this article are solely those of the authors and do not necessarily represent those of their affiliated organizations, or those of the publisher, the editors and the reviewers. Any product that may be evaluated in this article, or claim that may be made by its manufacturer, is not guaranteed or endorsed by the publisher.
Supplementary material
The Supplementary material for this article can be found online at: https://www.frontiersin.org/articles/10.3389/fneur.2024.1454220/full#supplementary-material
Footnotes
References
1. Saini, V, Guada, L, and Yavagal, DR. Global epidemiology of stroke and access to acute ischemic stroke interventions. Neurology. (2021) 97:S6–S16. doi: 10.1212/WNL.0000000000012781
2. Collaborators, GBDN . Global, regional, and national burden of neurological disorders, 1990–2016: a systematic analysis for the global burden of disease study 2016. Lancet Neurol. (2019) 18:459–80. doi: 10.1016/S1474-4422(18)30499-X
3. Chohan, SA, Venkatesh, PK, and How, CH. Long-term complications of stroke and secondary prevention: an overview for primary care physicians. Singapore Med J. (2019) 60:616–20. doi: 10.11622/smedj.2019158
4. Traversa, R, Cicinelli, P, Oliveri, M, Giuseppina Palmieri, M, Filippi, MM, Pasqualetti, P, et al. Neurophysiological follow-up of motor cortical output in stroke patients. Clin Neurophysiol. (2000) 111:1695–703. doi: 10.1016/S1388-2457(00)00373-4
5. Krakauer, JW, Carmichael, ST, Corbett, D, and Wittenberg, GF. Getting neurorehabilitation right: what can be learned from animal models? Neurorehabil Neural Repair. (2012) 26:923–31. doi: 10.1177/1545968312440745
6. Cramer, SC . Repairing the human brain after stroke: I. Mechanisms of spontaneous recovery. Ann Neurol. (2008) 63:272–87. doi: 10.1002/ana.21393
7. Lefaucheur, JP, Aleman, A, Baeken, C, Benninger, DH, Brunelin, J, Di Lazzaro, V, et al. Evidence-based guidelines on the therapeutic use of repetitive transcranial magnetic stimulation (rTMS): an update (2014–2018). Clin Neurophysiol. (2020) 131:474–528. doi: 10.1016/j.clinph.2019.11.002
8. Luk, KY, Ouyang, HX, and Pang, MYC. Low-frequency rTMS over Contralesional M1 increases Ipsilesional cortical excitability and motor function with decreased interhemispheric asymmetry in subacute stroke: a randomized controlled study. Neural Plast. (2022) 2022:1–13. doi: 10.1155/2022/3815357
9. Liao, L-Y, Zhu, Y, Peng, Q-Y, Gao, Q, Liu, L, Wang, Q-H, et al. Intermittent Theta-burst stimulation for stroke: primary motor cortex versus cerebellar stimulation: a randomized sham-controlled trial. Stroke. (2024) 55:156–65. doi: 10.1161/STROKEAHA.123.044892
10. Edwards, DJ, Liu, CY, Dunning, K, Fregni, F, Laine, J, Leiby, BE, et al. Electric field navigated 1-Hz rTMS for Poststroke motor recovery: the E-FIT randomized controlled trial. Stroke. (2023) 54:2254–64. doi: 10.1161/STROKEAHA.123.043164
11. Cunnington, R, Windischberger, C, Deecke, L, and Moser, E. The preparation and execution of self-initiated and externally-triggered movement: a study of event-related fMRI. NeuroImage. (2002) 15:373–85. doi: 10.1006/nimg.2001.0976
12. Kim, JH, Lee, JM, Jo, HJ, Kim, SH, Lee, JH, Kim, ST, et al. Defining functional SMA and pre-SMA subregions in human MFC using resting state fMRI: functional connectivity-based parcellation method. NeuroImage. (2010) 49:2375–86. doi: 10.1016/j.neuroimage.2009.10.016
13. Xia, Y, Tang, X, Hu, R, Liu, J, Zhang, Q, Tian, S, et al. Cerebellum-cerebrum paired target magnetic stimulation on balance function and brain network of patients with stroke: a functional near-infrared spectroscopy pilot study. Front Neurol. (2022) 13:1071328. doi: 10.3389/fneur.2022.1071328
14. Manji, A, Amimoto, K, Matsuda, T, Wada, Y, Inaba, A, and Ko, S. Effects of transcranial direct current stimulation over the supplementary motor area body weight-supported treadmill gait training in hemiparetic patients after stroke. Neurosci Lett. (2018) 662:302305:302–5. doi: 10.1016/j.neulet.2017.10.049
15. Koch, PJ, and Hummel, FC. Toward precision medicine: tailoring interventional strategies based on noninvasive brain stimulation for motor recovery after stroke. Curr Opin Neurol. (2017) 30:388–97. doi: 10.1097/WCO.0000000000000462
16. Du, J, Tian, L, Liu, W, Hu, J, Xu, G, Ma, M, et al. Effects of repetitive transcranial magnetic stimulation on motor recovery and motor cortex excitability in patients with stroke: a randomized controlled trial. Eur J Neurol. (2016) 23:1666–72. doi: 10.1111/ene.13105
17. Hosomi, K, Morris, S, Sakamoto, T, Taguchi, J, Maruo, T, Kageyama, Y, et al. Daily repetitive transcranial magnetic stimulation for Poststroke upper limb paresis in the subacute period. J Stroke Cerebrovasc Dis. (2016) 25:1655–64. doi: 10.1016/j.jstrokecerebrovasdis.2016.02.024
18. Sasaki, N, Abo, M, Hara, T, Yamada, N, Niimi, M, and Kakuda, W. High-frequency rTMS on leg motor area in the early phase of stroke. Acta Neurol Belg. (2017) 117:189–94. doi: 10.1007/s13760-016-0687-1
19. Grefkes, C, and Fink, GR. Connectivity-based approaches in stroke and recovery of function. Lancet Neurol. (2014) 13:206–16. doi: 10.1016/S1474-4422(13)70264-3
20. Simonetta-Moreau, M . Non-invasive brain stimulation (NIBS) and motor recovery after stroke. Ann Phys Rehabil Med. (2014) 57:530–42. doi: 10.1016/j.rehab.2014.08.003
21. Raffin, E, and Hummel, FC. Restoring motor functions after stroke: multiple approaches and opportunities. Neuroscientist. (2018) 24:400–16. doi: 10.1177/1073858417737486
22. Abdelkader, AA, Afifi, LM, Maher, EA, Atteya, AA, and el Salmawy, DA. Comparison of bilateral versus unilateral 5 Hz or 1 Hz repetitive transcranial magnetic stimulation in subacute stroke: assessment of motor function in a randomized controlled study. J Clin Neurophysiol. (2024) 41:478–83. doi: 10.1097/WNP.0000000000000987
23. Yang, Y, Pan, H, Pan, W, Liu, Y, Song, X, Niu, CM, et al. Repetitive transcranial magnetic stimulation on the affected hemisphere enhances hand functional recovery in subacute adult stroke patients: a randomized trial. Front Aging Neurosci. (2021) 13:636184. doi: 10.3389/fnagi.2021.636184
24. Rossini, PM, Burke, D, Chen, R, Cohen, LG, Daskalakis, Z, Di Iorio, R, et al. Non-invasive electrical and magnetic stimulation of the brain, spinal cord, roots and peripheral nerves: basic principles and procedures for routine clinical and research application. An updated report from an I.F.C.N. Committee. Clin Neurophysiol. (2015) 126:1071–107. doi: 10.1016/j.clinph.2015.02.001
25. Mosayebi-Samani, M, Agboada, D, Mutanen, TP, Haueisen, J, Kuo, MF, and Nitsche, MA. Transferability of cathodal tDCS effects from the primary motor to the prefrontal cortex: a multimodal TMS-EEG study. Brain Stimul. (2023) 16:515–39. doi: 10.1016/j.brs.2023.02.010
26. Hernández, E, Galeano, CP, Barbosa, NE, Forero, S, Nordin, Å, Sunnerhagen, K, et al. Intra- and inter-rater reliability of Fugl-Meyer assessment of upper extremity in stroke. J Rehabil Med. (2019) 51:652–9. doi: 10.2340/16501977-2590
27. Hernández, ED, Forero, SM, Galeano, CP, Barbosa, NE, Sunnerhagen, KS, and Alt Murphy, M. Intra- and inter-rater reliability of Fugl-Meyer assessment of lower extremity early after stroke. Braz J Phys Ther. (2021) 25:709–18. doi: 10.1016/j.bjpt.2020.12.002
28. Sarasso, E, Gardoni, A, Tettamanti, A, Agosta, F, Filippi, M, and Corbetta, D. Virtual reality balance training to improve balance and mobility in Parkinson's disease: a systematic review and meta-analysis. J Neurol. (2022) 269:1873–88. doi: 10.1007/s00415-021-10857-3
29. Rossi, S, Hallett, M, Rossini, PM, and Pascual-Leone, ASafety of TMSCG. Safety, ethical considerations, and application guidelines for the use of transcranial magnetic stimulation in clinical practice and research. Clin Neurophysiol. (2009) 120:2008–39. doi: 10.1016/j.clinph.2009.08.016
30. Pellicciari, MC, Bonni, S, Ponzo, V, Cinnera, AM, Mancini, M, Casula, EP, et al. Dynamic reorganization of TMS-evoked activity in subcortical stroke patients. NeuroImage. (2018) 175:365–78. doi: 10.1016/j.neuroimage.2018.04.011
31. Koch, G, Bonni, S, Casula, EP, Iosa, M, Paolucci, S, Pellicciari, MC, et al. Effect of cerebellar stimulation on gait and balance recovery in patients with Hemiparetic stroke: a randomized clinical trial. JAMA Neurol. (2019) 76:170–8. doi: 10.1001/jamaneurol.2018.3639
32. Ni, J, Jiang, W, Gong, X, Fan, Y, Qiu, H, Dou, J, et al. Effect of rTMS intervention on upper limb motor function after stroke: a study based on fNIRS. Front Aging Neurosci. (2022) 14:1077218. doi: 10.3389/fnagi.2022.1077218
33. Bozkurt, B, Yagmurlu, K, Middlebrooks, EH, Karadag, A, Ovalioglu, TC, Jagadeesan, B, et al. Microsurgical and Tractographic anatomy of the supplementary motor area complex in humans. World Neurosurg. (2016) 95:99–107. doi: 10.1016/j.wneu.2016.07.072
34. Ward, NS, Brown, MM, Thompson, AJ, and Frackowiak, RS. Neural correlates of motor recovery after stroke: a longitudinal fMRI study. Brain. (2003) 126:2476–96. doi: 10.1093/brain/awg245
35. Grefkes, C, Nowak, DA, Wang, LE, Dafotakis, M, Eickhoff, SB, and Fink, GR. Modulating cortical connectivity in stroke patients by rTMS assessed with fMRI and dynamic causal modeling. NeuroImage. (2010) 50:233–42. doi: 10.1016/j.neuroimage.2009.12.029
36. Silasi, G, and Murphy, TH. Stroke and the connectome: how connectivity guides therapeutic intervention. Neuron. (2014) 83:1354–68. doi: 10.1016/j.neuron.2014.08.052
37. Palmer, JA, Wheaton, LA, Gray, WA, Saltao da Silva, MA, Wolf, SL, and Borich, MR. Role of interhemispheric cortical interactions in Poststroke motor function. Neurorehabil Neural Repair. (2019) 33:762–74. doi: 10.1177/1545968319862552
38. McDonnell, MN, and Stinear, CM. TMS measures of motor cortex function after stroke: a meta-analysis. Brain Stimul. (2017) 10:721–34. doi: 10.1016/j.brs.2017.03.008
39. Takechi, U, Matsunaga, K, Nakanishi, R, Yamanaga, H, Murayama, N, Mafune, K, et al. Longitudinal changes of motor cortical excitability and transcallosal inhibition after subcortical stroke. Clin Neurophysiol. (2014) 125:2055–69. doi: 10.1016/j.clinph.2014.01.034
40. Di Pino, G, Pellegrino, G, Assenza, G, Capone, F, Ferreri, F, Formica, D, et al. Modulation of brain plasticity in stroke: a novel model for neurorehabilitation. Nat Rev Neurol. (2014) 10:597–608. doi: 10.1038/nrneurol.2014.162
41. Rizzolatti, G, and Matelli, M. Two different streams form the dorsal visual system: anatomy and functions. Exp Brain Res. (2003) 153:146–57. doi: 10.1007/s00221-003-1588-0
42. Harrison, OK, Guell, X, Klein-Flugge, MC, and Barry, RL. Structural and resting state functional connectivity beyond the cortex. NeuroImage. (2021) 240:118379. doi: 10.1016/j.neuroimage.2021.118379
43. Hordacre, B, Moezzi, B, and Ridding, MC. Neuroplasticity and network connectivity of the motor cortex following stroke: a transcranial direct current stimulation study. Hum Brain Mapp. (2018) 39:3326–39. doi: 10.1002/hbm.24079
44. Potok, W, Maskiewicz, A, Króliczak, G, and Marangon, M. The temporal involvement of the left supramarginal gyrus in planning functional grasps: a neuronavigated TMS study. Cortex. (2019) 111:16–34. doi: 10.1016/j.cortex.2018.10.010
45. Schmahmann, JD, Rosene, DL, and Pandya, DN. Motor projections to the basis pontis in rhesus monkey. J Comp Neurol. (2004) 478:248–68. doi: 10.1002/cne.20286
46. Damoiseaux, JS, Rombouts, SA, Barkhof, F, Scheltens, P, Stam, CJ, Smith, SM, et al. Consistent resting-state networks across healthy subjects. Proc Natl Acad Sci USA. (2006) 103:13848–53. doi: 10.1073/pnas.0601417103
47. Guggisberg, AG, Koch, PJ, Hummel, FC, and Buetefisch, CM. Brain networks and their relevance for stroke rehabilitation. Clin Neurophysiol. (2019) 130:1098–124. doi: 10.1016/j.clinph.2019.04.004
48. Fernandez, L, Rogasch, NC, Do, M, Clark, G, Major, BP, Teo, WP, et al. Cerebral cortical activity following non-invasive cerebellar stimulation-a systematic review of combined TMS and EEG studies. Cerebellum. (2020) 19:309–35. doi: 10.1007/s12311-019-01093-7
Keywords: ischemic stroke, rTMS, SMA, rehabilitation, neural remodeling
Citation: Zhao L, Chen L, Wang C, Li S and Wan C (2024) Effects of repetitive transcranial magnetic stimulation at different targets on brain function in stroke patients: a randomized controlled trial. Front. Neurol. 15:1454220. doi: 10.3389/fneur.2024.1454220
Edited by:
Giorgio Scivoletto, Santa Lucia Foundation (IRCCS), ItalyReviewed by:
Joao Miguel Castelhano, University of Coimbra, PortugalAkhil Mohan, Cleveland Clinic, United States
Hui-Ting Goh, Texas Woman’s University, United States
Weronika Dębowska, Medical University of Warsaw, Poland
Copyright © 2024 Zhao, Chen, Wang, Li and Wan. This is an open-access article distributed under the terms of the Creative Commons Attribution License (CC BY). The use, distribution or reproduction in other forums is permitted, provided the original author(s) and the copyright owner(s) are credited and that the original publication in this journal is cited, in accordance with accepted academic practice. No use, distribution or reproduction is permitted which does not comply with these terms.
*Correspondence: Chunxiao Wan, cmVoYWJ0ZWFtb2Z3YW5AMTYzLmNvbQ==