- Department of Biology, SDSU Electron Microscopy Facility, San Diego State University, San Diego, CA, United States
Many neurodegenerative diseases (NDD) appear to share commonality of origin, chronic ER stress. The endoplasmic reticulum (ER) is a dynamic organelle, functioning as a major site of protein synthesis and protein posttranslational modifications, required for proper folding. ER stress can occur because of external stimuli, such as oxidative stress or neuroinflammatory cytokines, creating the ER luminal environment permissive for the accumulation of aggregated and misfolded proteins. Unresolvable ER stress upregulates a highly conserved pathway, the unfolded protein response (UPR). Maladaptive chronic activation of UPR components leads to apoptotic neuronal death. In addition to other factors, physiological responses to stressors are emerging as a significant risk factor in the etiology and pathogenesis of NDD. Owned cats share a common environment with people, being exposed to many of the same stressors as people and additional pressures due to their “quasi” domesticated status. Feline Cognitive Dysfunction Syndrome (fCDS) presents many of the same disease hallmarks as human NDD. The prevalence of fCDS is rapidly increasing as more people welcome cats as companions. Barely recognized 20 years ago, veterinarians and scientists are in infancy stages in understanding what is a very complex disease. This review will describe how cats may represent an unexplored animal mimetic phenotype for human NDD with stressors as potential triggering mechanisms. We will consider how multiple variations of stressful events over the short-life span of a cat could affect neuronal loss or glial dysfunction and ultimately tip the balance towards dementia.
Introduction
Despite the original hypothesis proposed by Chambers et al. (1), the domestic cat is an underutilized model for NDD. When compared to transgenic rodent models or primates (2), felines are the only species extensively studied in which β-amyloid plaques (Aβ) and neurofibrillary hyperphosphorylated tau tangles (NFT) are found naturally comorbid (1, 3–6). Finding a translational animal model where early dementia-like behaviors and correlative biochemical signatures can predict final cognitive decline is a big leap forward in our understanding of the etiology of these devastating diseases. Cats, with significantly shorter life spans than humans, can bridge our gap in understanding the temporal progression in NDD and in our current futility in finding clinically efficacious therapies that go beyond simple temporary cognitive gains.
Modern human and animal medicine has greatly lengthened lifespans with a staggering increase in reported cognitive issues and dementia in people and cats alike. There is an emerging consensus within veterinary medicine on the need to understand senile dementia in our pet population (7–11). fCDS is currently a diagnosis of exclusion and like human counterparts; there are limited treatment options. In a seminal paper published in 2007, Dr. Gunn-Moore and Dr. Head review the lab evidence, behavioral changes and neuropathology unique to fCDS in geriatric cats (12). Recent studies confirm and extend the human-like neuropathology phenotype as ever closer to many human NDD (13, 14). Much like humans in early stages of NDD, the initial clinical feline characteristics present as disorientation, short-term memory loss, sleep/wake disturbances, incontinence, anxiety and increased vocalizations (15).
The unfolded protein response (UPR) is a highly conserved evolutionary pathway. Within the endoplasmic reticulum (ER), nascent polypeptide chains are synthesized on associated ribosomes or imported for post-translational modifications, and further folded into cellular proteins to be exported through Golgi-mediated pathways. Imbalances in this “conveyor belt” system cause backups and accumulation of defective or misfolded proteins, in essence blocking the carefully refined proteostasis between synthesis and degradation of cellular proteins; reviewed in (16–22). When the tipping point between accumulation and ER degradation of defective proteins in the ER lumen occurs, a condition called “ER stress” results. Resolving ER stress is critical to cell survival. Eukaryotic cells have evolved three distinct signaling pathways (UPR) to reprogram protein expression at multiple levels. Gene expression is regulated by activating transcription factor 6 (ATF6). Protein synthesis is regulated by eukaryotic initiation factor 2 (eIF2α) kinase (PERK), and modulation of protein folding through type I transmembrane protein inositol requiring 1 (IRE1α). These pathways are linked to ER-associated protein degradation (ERAD) and lysosomal degradation to remove problematic proteins (16).
Mild ER stress from temporary or mild physiological or pathological events is resolved through adaptive cytoprotective UPR mechanisms. However, continuous, or excessive protein synthesis of imperfect product or a slowing of the transport or degrading systems induces prolonged activation of UPR pathways, leading to maladaptive cellular signaling and ultimately, cell damage or death. Given the abundance of potential aggregating proteins associated with NDD, it is not surprising that links between ER stress and upregulation of UPR mechanisms is an emerging therapeutic area for all forms of NDD (18, 23–26).
Another critical factor upregulating maladaptive UPR pathways is the presence of neuroinflammation. Activated UPR components have been shown to increase known pro-inflammatory cytokines, IL-6, TNF-α, IL-1β, through the common transcription factor nuclear factor-κβ (NF-κβ) (27). Within the CNS, both microglia and astrocytes can synthesize and secrete pro-inflammatory proteins and cytokines, beginning a positive feedback loop and crosstalk between increasing ER stress, upregulation of UPR mechanisms and increasing levels of neuroinflammation. This prolonged activation of pro-apoptotic components of the UPR eventually leads to synaptic loss or neuronal cell death (28). Aβ accumulation may be an early triggering mechanism for this crosstalk loop initiation. When Aβ is administered to rats, an upregulation of ER stress and inflammatory markers is seen with a concomitant increase in cognitive impairment (29).
Oxidative stress is the third branch of a triumvirate with UPR components and neuroinflammation in the pathway to NDD. This state is an imbalance between reactive oxygen species (ROS) and antioxidant mediators (redox). Outside of mitochondrial oxidative phosphorylation, the ER generates about a quarter of total cellular ROS through protein folding (30). Disulfide bridging, required for some protein folding, occurs in the oxidizing environment of the ER lumen. Problems arise when oxidative stress overwhelms ER ROS defenses and protein transport is impeded (31). The UPR is then activated and if the excess ROS becomes chronic, neuronal apoptosis is likely (32).
Cats are unique pets. Dogs have lost genetic components found in their wild counterparts, allowing for easier cohabitation with humans (33). Paleogenetic studies support the concept that cats, not humans, initiated their coexistence (34, 35). Modern feline DNA is nearly identical between the suspected wild ancestor of our modern housecat; Felis sylvestris lybica and current pet or feral felines (Felis sylvestris catus). However, key genetic signatures have been found in specific genes associated with behavior and reward systems (36) in cats living with humans, demonstrating the physical and social pressures cats may experience when dwelling amongst humans. Indoor only cats display enhanced problem-solving in a task that assesses social engagement with humans over mainly outdoor living cats (37). Actual feline facial morphology and expressions have been shaped by interactions with humans (38). Humans apparently like their housecats to appear “cute” over feral. Cats may be able to somewhat adapt to our world but the stressors can occasionally become overwhelming (39), with predictable behavioral issues.
There are few comparative studies of stressors between human and felines (40, 41). Funding for companion animals, cats in particular, is limited for pathologies such as fCDS. Given those constraints, chronic stressors must be viewed synergistically between humans and cats. Common environmental stressors are shared within a household and affect each species simultaneously (42). Many studies exist on effects of stressors on humans or model systems, like mice, rats, or dogs, but far fewer exist for felines alone. This review will breakdown what is known about the effects of predictable stressors on cats, categorized as environmental, social-behavioral, and physiological stress, and the potential for oxidative stress, neuroinflammation or increased expression of activated UPR from these stressors to induce cellular damage or cell death leading to an NDD-like pathology (Figure 1).
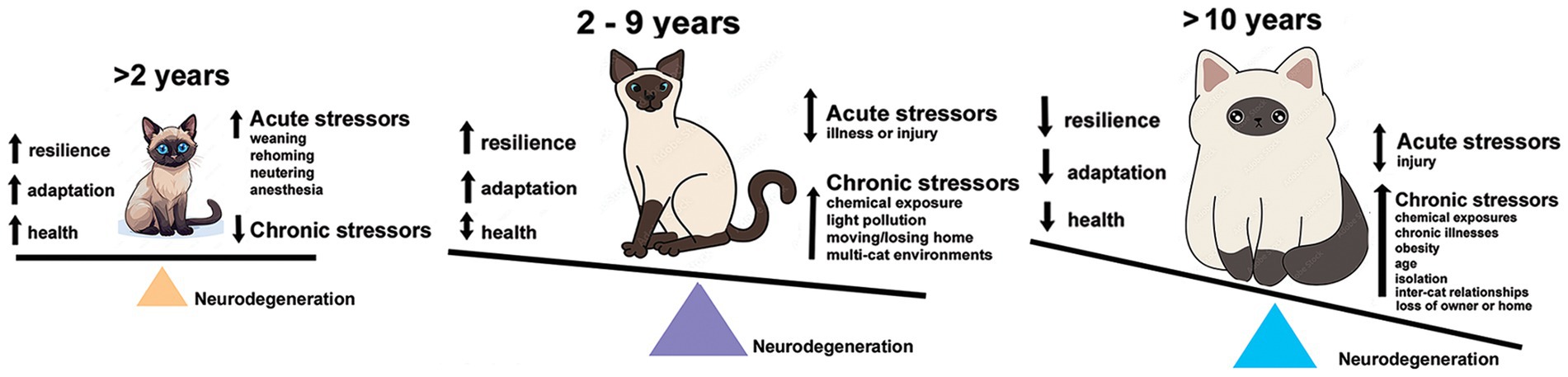
Figure 1. Tipping the scales over the lifespan of an owned cat towards neurodegeneration. Kittens and adolescent cats have capacity for resilience and adaptability to acute stresses and have fewer interactions with chronic stressors. By the time they reach adulthood, the burden of chronic stressors is accelerating and neural damage accumulating. Senior cats (10 years and older) are exposed to a multitude of stresses and increasing effects of health and age-related issues just as humans. Their capacity for resilience and adaptation is more limited, further increasing the potential for neural damage and loss.
Experimentally known effects of stressors on domestic cats
Environmental stressors
Although this review will mainly focus on research using client-owned cats, rather than feral individuals, many of the same environmental conditions exist for free-roaming or feral animals. Toxic chemicals, noises and excessive lighting exist as abundantly in outdoor environments as in our home settings. One of the most prominent indoor pollutants is tobacco smoke and the associated toxins from either cigarettes or vaping. Cats have highly developed olfactory systems, thus, even small amounts of second or third hand smoke evoke biochemical responses. Like human counterparts, cats respond to tobacco smoke with increased levels of pro-inflammatory cytokines, indicating states of enhanced oxidative stress (43). As neurons require significant oxygen to maintain high levels of oxidative phosphorylation, an imbalance between reactive oxygen species (ROS) and antioxidant compounds exists. Microglial phenotypes are found to be altered in human cell cultures following exposure to nicotine, further increasing localized ROS (44). The ER has a critical function in oxygen regulation and in situations of oxidative stress, the UPR is upregulated to mitigate the damage. Prolonged exposure to cigarette smoke for cats will therefore be considered a chronic stressor, with the potential to trigger the apoptotic wing of UPR over time in neurons by the generation of pro-inflammatory cytokines and increasing levels of oxidative stress in microglia and astrocytes.
Cats develop acute hearing at very early ages. They can discriminate ambient sounds outside of the human auditory range. Computer monitors, fluorescent light bulbs and other high frequency emitters are within their capabilities. Loud unpredictable sounds cause indoor cats stress when hiding places are limited. Resident and ambient noise is a consistent feature in homes. A typical range has been measured at 51–78.2 dB (45). White noise in the range of 5-20 kHz at 115 dB leads to auditory neuron degeneration in mice within hours of exposure (46). The same range of exposure in adult male cats resulted in synaptic dysfunction within the colliculus inferior over a period of 10 days exposure to 1 hour of noise daily (47). Whether synaptic dysfunction proceeds or precedes oxidative stress, the result is the same; neuronal loss and overall neurodegeneration (48). Thus, our noisy lives, with music, TV, traffic background and electronic white noise, has the potential to increase ROS, and begin the downward spiral of oxidative stress-UPR-neuroinflammation cycles.
Humans have disrupted the natural cycles of light and dark with artificial lighting. We have even stretched that further with bright LED illumination, increasing blue light (λ = 460–480 nm) and electronics left on 24 h. Light cycles are a critical aspect of circadian rhythms that govern wakefulness, sleep pattern and daily functioning. All cats sleep a typical 12–20 h per day, with peak wakefulness at sunrise or sunset to coordinate with prey activity. Light can affect the sleep of domestic cats, as it does in humans or rodents, by depressing expression of melatonin, interrupting normal intensity of sleep patterns or extend diurnal or nocturnal activity (49). Nighttime light exposure is shown to increase depression in humans (50, 51) and in rodent models (52–54). An unexplored aspect of sleep disruption is a recently recognized impairment of the brain waste disposal system; the astrocyte-mediated glymphatic system (55). Sleep disturbances increase CSF levels of toxic Aβ (56, 57), which accumulates into extracellular plaques. Hyperphosphorylated tau is usually found intracellularly but a recent study has found extracellular tau in the glymphatic system, indicating clearance could also be impaired with altered sleep (58). As cats present with both senile Aβ plaques and NFT tau isoforms (1, 3–6), stress of nighttime light may contribute to developing NDD.
Further increasing stress in a domestic cat are human expectations. Humans typically sleep between 10 PM – 6 AM and dislike nighttime disturbances such as wandering or pouncing cats. Playtimes are shifted towards daytime when cats would naturally be inactive. Domestic cats have had to suppress typical behaviors to maintain their human-cat bonds. Therefore, their ingrained sleep patterns may become more fragmented. In mice, fragmented sleep increases Aβ (40 and 42) in the hippocampus and increases microglial activation and secretion of pro-inflammatory cytokines (59). Dim light exposure of pig retinal organotypic cultures up-regulates markers of ER stress and electron microscopic analysis reveals photoreceptor degeneration (60). Our continual use of artificial light may be a major triggering mechanism over a lifespan of a cat towards NDD.
Social - behavioral stressors
Traditionally considered solitary hunters (61), modern feral cats frequently reside in large colonies (62). Although, there is limited research on domestic cats and social structures (63, 64), studies exist on larger cat species from zoos suggesting housing with unrelated conspecifics, and a lack of hiding spaces contributes to captive large cat stress (65, 66). Within a household, solitary cats do not have the daily stress of feline hierarchical conflicts, but in multi-cat homes, such daily stressors can have a profound effect on individual cats. A 2020 survey found that almost 74% of owners reported inter-cat conflict signals early in stages of introduction, with 50% reporting physical contact. There was a correlation between the number of resident cats and the reporting of conflicts (67). Some earlier studies show conflicting data. Urinary or fecal cortisol levels were not significantly different in multi-cat, single cat or sheltering housing conditions (68, 69), indicating that circulating cortisol levels do not fully reflect the full spectrum of behavioral aspects of feline stress. A more realistic assessment for feline stress relies on observational scores for behavioral demeanor for individual cats (70).
Individual cats frequently respond differently to stimuli regardless of their social ranking (71). Cats can and do live cooperatively. Yet, many feel and react to the stress of a lack of hiding resources, limited litter resources and especially the presence of a dominant bully cat. The aggressor cat and the bullied cat are at risk for oxidative stress and neuroinflammation (72). When aggressive mice are treated with antioxidants, biomarkers for oxidative stress, pro-inflammatory cytokines and aggressive behaviors decreased (73). The less dominant cat is at risk for depression, just as their human counterparts (74–76). Major depressive disorder (MDD) has its pathological roots in dysfunctional neurotransmission. Yet, there is increasing evidence that MDD further triggers neuroinflammation and concomitantly induces ER stress and upregulation of activated UPR components (77–79).
More pet parents are bowing to increasing public pressure to protect cat’s lives by restricting or eliminating their access to the outdoors. Although their impacts to wildlife are overblown (80), the dangers to owned pets by cars, coyotes, theft, or injury is real. For many cats, restricting their movements is a real stressor (81). In rodent models of restraint, rats display depressive like behaviors; reviewed by (82) and have increased expression of UPR components (83). Living in close contact with humans creates social stresses in our territorial pet cats that earlier generations of felines never experienced. Multi-cat homes, less range, and more restrictions over fundamental physiological needs has provided a seeding ground for psychological issues that could progress to actual neurological pathologies.
Physiological stressors
A major human risk factor for development of NDD is obesity (84, 85). Obesity is not a typical problem for feral cats, as they rely on prey availability. The story is quite different for owned domestic cats. Worldwide, an estimated 11–63% of household living cats are overweight (86) and a third considered obese. Extra weight is a “heavy” physiological stressor, and for cats, a direct consequence of human control over nutritional choices for their cats. Hematological oxidative stress markers are elevated in obese cats over control and overweight cats (87), predisposing animals to neuroinflammation. Compelling evidence from human obesity studies, mainly using advanced imaging techniques, demonstrate significant structural changes in brains of obese individuals (88–91), with atrophy of key areas such as the hippocampus and subcortical structures. Hand in hand with obesity, is the specter of physiological malnutrition of domestic cats. As obligate carnivores, commercial foods high in fat, consisting of unnatural plant-derived proteins, and carbohydrate additives, such as corn, soy or grains, combined with low levels of omega-3 and omega-6 polyunsaturated fatty acids (PUFA), are not adequate substitutes for pure animal proteins (92). Insufficient intake of animal-derived essential amino acids (taurine for cats), vitamin D3 and cholesterol can impede neuronal development or effect feline cognition (93, 94). Examples of diet-related cognitive dysfunction are found in other animal models. Thiamine deficiency (vitamin B1) increases oxidative stress and is associated with neuroinflammation leading to potentially irreversible hippocampal damage in an organotypic culture experiment (95). Oxidative stress and impaired spatial memory are a feature of a mouse model of protein malnutrition (96). Combining overfeeding our cats, and the physiological stress of a nutrient restrictive diet, both of which have the potential to upregulate maladaptive UPR mechanisms, humans are creating conditions appropriate for development of NDD feedback loops.
Age is cited as the most prominent risk factor in human NDD. It is a stressor that both humans and cats share. We have expanded human life with advanced medicine. Bringing our cats inside and providing steady diets, effective vaccinations and life-saving care has greatly extended previous definitions of feline lifespans. It is not usual for owned cats to live well beyond 16 years, while feral cats struggle to <5 years. With this increase in life expectancy comes a staggering increase in the incidence of feline cognitive dysfunction (fCDS) (11, 14, 15, 97–99). Extrapolating from human studies of aging and neuroinflammation, cats are expected to display similar increasing markers of neuroinflammation (100), but direct studies are lacking. Aged brains have increased levels of oxidized proteins and changes in membrane fatty acid composition with increased amounts of ROS susceptible monosaturated fatty acids and arachidonic acid (AA) (101). Systemic oxidative stress is reported in aging cats, with male cats at greater risks (102). Currently, there is more research on canine brain health than cats.
Discussion
Finding a translational animal model where early dementia-like behaviors and correlative biochemical signatures can predict final cognitive decline is a big leap forward in our understanding of the etiology of NDD. Homed or sheltered cats may fill this current void, benefitting cats and humans. That we have this ideal model sitting in our living rooms today, makes this idea even more novel and exciting. Human-influenced stressors in cat’s lives have the potential to prime the cycle of neuronal damage, synaptic dysfunction, and maladaptive glial function, all leading up to neuron death and degeneration. Prevalence of fCDS is on the rise as our cat population ages. Whereas canine CDS is well defined, feline CDS remains mysterious due to lack of awareness by owners and veterinarians and the ability of cats to hide symptoms. Like human NDD, there are no effective pharmacological interventions to stop the neurodegeneration once it begins. Thus, understanding potential triggers of neuronal damage may hold keys to identifying at risk feline populations and finding efficacious treatments. Triggers may be multi-factorial, combining throughout the life of a cat with the endpoint being NDD. With a shorter course of disease, assessing therapies that control cognitive loss and brain loss become attainable goals. Importantly, the ability to find the earliest biochemical signatures of disease becomes possible. At risk cats, individuals with a combination of identified life stresses along with careful owner observation, can be tracked over time looking for changes in biochemistry and behaviors that eventually overlap with fCDS signalment (98) [Basepaws Aging survey]1. Thus, we advocate for more funding and more translational grants for feline research. Why else would the National Center for Advancing Translational Sciences be called NCATS?
Author contributions
IN: Conceptualization, Investigation, Writing – original draft, Writing – review & editing.
Funding
The author(s) declare that no financial support was received for the research, authorship, and/or publication of this article.
Acknowledgments
I wish to thank Ingrid King, author, and publisher of “Purrs of Wisdom” for encouraging me to continue writing about this important topic in feline health and medicine, despite a lack of funding.
Conflict of interest
The author declares that the research was conducted in the absence of any commercial or financial relationships that could be construed as a potential conflict of interest.
Publisher’s note
All claims expressed in this article are solely those of the authors and do not necessarily represent those of their affiliated organizations, or those of the publisher, the editors and the reviewers. Any product that may be evaluated in this article, or claim that may be made by its manufacturer, is not guaranteed or endorsed by the publisher.
Footnotes
1. ^Feline Longevity Research at Basepaws.
References
1. Chambers, JK, Tokuda, T, Uchida, K, Ishii, R, Tatebe, H, Takahashi, E, et al. The domestic cat as a natural animal model of Alzheimer's disease. Acta Neuropathol Commun. (2015) 3:78. doi: 10.1186/s40478-015-0258-3
2. Youssef, SA, Capucchio, MT, Rofina, JE, Chambers, JK, Uchida, K, Nakayama, H, et al. Pathology of the aging brain in domestic and laboratory animals, and animal models of human neurodegenerative diseases. Vet Pathol. (2016) 53:327–48. doi: 10.1177/0300985815623997
3. Vite, CH, and Head, E. Aging in the canine and feline brain. Vet Clin North Am Small Anim Pract. (2014) 44:1113–29. doi: 10.1016/j.cvsm.2014.07.008
4. Chambers, JK, Uchida, K, Harada, T, Tsuboi, M, Sato, M, Kubo, M, et al. Neurofibrillary tangles and the deposition of a beta amyloid peptide with a novel N-terminal epitope in the brains of wild Tsushima leopard cats. PLoS One. (2012) 7:e46452. doi: 10.1371/journal.pone.0046452
5. Gunn-Moore, DA, McVee, J, Bradshaw, JM, Pearson, GR, Head, E, and Gunn-Moore, FJ. Ageing changes in cat brains demonstrated by beta-amyloid and AT8-immunoreactive phosphorylated tau deposits. J Feline Med Surg. (2006) 8:234–42. doi: 10.1016/j.jfms.2006.01.003
6. Head, E, Moffat, K, das, P, Sarsoza, F, Poon, WW, Landsberg, G, et al. Beta-amyloid deposition and tau phosphorylation in clinically characterized aged cats. Neurobiol Aging. (2005) 26:749–63. doi: 10.1016/j.neurobiolaging.2004.06.015
7. Crowell-Davis, SL. Cognitive dysfunction in senior pets. Compend Contin Educ Vet. (2008) 30:106–10.
8. Landsberg, G, and Araujo, JA. Behavior problems in geriatric pets. Vet Clin North Am Small Anim Pract. (2005) 35:675–98. doi: 10.1016/j.cvsm.2004.12.008
9. Landsberg, GM, Denenberg, S, and Araujo, JA. Cognitive dysfunction in cats: a syndrome we used to dismiss as 'old age'. J Feline Med Surg. (2010) 12:837–48. doi: 10.1016/j.jfms.2010.09.004
10. Landsberg, GM, Deporter, T, and Araujo, JA. Clinical signs and management of anxiety, sleeplessness, and cognitive dysfunction in the senior pet. Vet Clin North Am Small Anim Pract. (2011) 41:565–90. doi: 10.1016/j.cvsm.2011.03.017
11. Landsberg, GM, Nichol, J, and Araujo, JA. Cognitive dysfunction syndrome: a disease of canine and feline brain aging. Vet Clin North Am Small Anim Pract. (2012) 42:749–68. doi: 10.1016/j.cvsm.2012.04.003
12. Gunn-Moore, D, Moffat, K, Christie, LA, and Head, E. Cognitive dysfunction and the neurobiology of ageing in cats. J Small Anim Pract. (2007) 48:546–53. doi: 10.1111/j.1748-5827.2007.00386.x
13. Vidal-Palencia, L, Font, C, Rebollada-Merino, A, Santpere, G, Andrés-Benito, P, Ferrer, I, et al. Primary feline Tauopathy: clinical, morphological, Immunohistochemical, and genetic studies. Animals. (2023) 13:2985. doi: 10.3390/ani13182985
14. Sordo, L, Martini, AC, Houston, EF, Head, E, and Gunn-Moore, D. Neuropathology of aging in cats and its similarities to human Alzheimer's disease. Front Aging. (2021) 2:684607. doi: 10.3389/fragi.2021.684607
15. Sordo, L, and Gunn-Moore, DA. Cognitive dysfunction in cats: update on neuropathological and Behavioural changes plus clinical management. Vet Rec. (2021) 188:e3. doi: 10.1002/vetr.3
16. Read, A, and Schroder, M. The unfolded protein response: an overview. Biology. (2021) 10:384. doi: 10.3390/biology10050384
17. Kim, S, Kim, DK, Jeong, S, and Lee, J. The common cellular events in the neurodegenerative diseases and the associated role of endoplasmic reticulum stress. Int J Mol Sci. (2022) 23:5894. doi: 10.3390/ijms23115894
18. Singh, R, Kaur, N, Choubey, V, Dhingra, N, and Kaur, T. Endoplasmic reticulum stress and its role in various neurodegenerative diseases. Brain Res. (2024) 1826:148742. doi: 10.1016/j.brainres.2023.148742
19. Mahdi, AA, Rizvi, SH, and Parveen, A. Role of endoplasmic reticulum stress and unfolded protein responses in health and diseases. Indian J Clin Biochem. (2016) 31:127–37. doi: 10.1007/s12291-015-0502-4
20. Diaz-Villanueva, JF, Diaz-Molina, R, and Garcia-Gonzalez, V. Protein folding and mechanisms of Proteostasis. Int J Mol Sci. (2015) 16:17193–230. doi: 10.3390/ijms160817193
21. Sano, R, and Reed, JC. ER stress-induced cell death mechanisms. Biochim Biophys Acta. (2013) 1833:3460–70. doi: 10.1016/j.bbamcr.2013.06.028
22. Hetz, C, Zhang, K, and Kaufman, RJ. Mechanisms, regulation and functions of the unfolded protein response. Nat Rev Mol Cell Biol. (2020) 21:421–38. doi: 10.1038/s41580-020-0250-z
23. Valdés, P, Mercado, G, Vidal, RL, Molina, C, Parsons, G, Court, FA, et al. Control of dopaminergic neuron survival by the unfolded protein response transcription factor XBP1. Proc Natl Acad Sci USA. (2014) 111:6804–9. doi: 10.1073/pnas.1321845111
24. Naranjo, JR, Zhang, H, Villar, D, González, P, Dopazo, XM, Morón-Oset, J, et al. Activating transcription factor 6 derepression mediates neuroprotection in Huntington disease. J Clin Invest. (2016) 126:627–38. doi: 10.1172/JCI82670
25. Yu, Z, Sheng, H, Liu, S, Zhao, S, Glembotski, CC, Warner, DS, et al. Activation of the ATF6 branch of the unfolded protein response in neurons improves stroke outcome. J Cereb Blood Flow Metab. (2017) 37:1069–79. doi: 10.1177/0271678X16650218
26. Jeon, JH, Im, S, Kim, HS, Lee, D, Jeong, K, Ku, JM, et al. Chemical chaperones to inhibit endoplasmic reticulum stress: implications in diseases. Drug Des Devel Ther. (2022) 16:4385–97. doi: 10.2147/DDDT.S393816
27. Salminen, A, Kauppinen, A, Suuronen, T, Kaarniranta, K, and Ojala, J. ER stress in Alzheimer's disease: a novel neuronal trigger for inflammation and Alzheimer's pathology. J Neuroinflammation. (2009) 6:41. doi: 10.1186/1742-2094-6-41
28. Santos, LE, and Ferreira, ST. Crosstalk between endoplasmic reticulum stress and brain inflammation in Alzheimer's disease. Neuropharmacology. (2018) 136:350–60. doi: 10.1016/j.neuropharm.2017.11.016
29. Goswami, P, Akhter, J, Mangla, A, Suramya, S, Jindal, G, Ahmad, S, et al. Downregulation of ATF-4 attenuates the endoplasmic reticulum stress-mediated Neuroinflammation and cognitive impairment in experimentally induced Alzheimer's disease model. Mol Neurobiol. (2023). doi: 10.1007/s12035-023-03861-3
30. Tu, BP, and Weissman, JS. Oxidative protein folding in eukaryotes: mechanisms and consequences. J Cell Biol. (2004) 164:341–6. doi: 10.1083/jcb.200311055
31. Ong, G, and Logue, SE. Unfolding the interactions between endoplasmic reticulum stress and oxidative stress. Antioxidants. (2023) 12:981. doi: 10.3390/antiox12050981
32. Ekundayo, BE, Obafemi, TO, Adewale, OB, Obafemi, BA, Oyinloye, BE, and Ekundayo, SK. Oxidative stress, endoplasmic reticulum stress and apoptosis in the pathology of Alzheimer's disease. Cell Biochem Biophys. (2024). doi: 10.1007/s12013-024-01248-2
33. Hunter, P. The genetics of domestication: research into the domestication of livestock and companion animals sheds light both on their "evolution" and human history. EMBO Rep. (2018) 19:201–5. doi: 10.15252/embr.201745664
34. Ottoni, C, Van Neer, W, De Cupere, B, Daligault, J, Guimaraes, S, Peters, J, et al. The palaeogenetics of cat dispersal in the ancient world. Nat Ecol Evol. (2017) 1:1–7. doi: 10.1038/s41559-017-0139
35. Natoli, E, Litchfield, C, and Pontier, D. Coexistence between humans and 'Misunderstood' domestic cats in the Anthropocene: exploring Behavioural plasticity as a gatekeeper of evolution. Animals. (2022) 12:1717. doi: 10.3390/ani12131717
36. Montague, MJ, Li, G, Gandolfi, B, Khan, R, Aken, BL, Searle, SMJ, et al. Comparative analysis of the domestic cat genome reveals genetic signatures underlying feline biology and domestication. Proc Natl Acad Sci USA. (2014) 111:17230–5. doi: 10.1073/pnas.1410083111
37. Scandurra, A, di Lucrezia, A, D’Aniello, B, and Pinelli, C. Home sweet home: the impact of lifestyle on a Cat's approach to impossible tasks in the home environment. Animals. (2023) 13:2679. doi: 10.3390/ani13162679
38. Hattori, M, Saito, A, Nagasawa, M, Kikusui, T, and Yamamoto, S. Changes in cat facial morphology are related to interaction with humans. Animals. (2022) 12:3493. doi: 10.3390/ani12243493
39. Zhang, L, Bian, Z, Liu, Q, and Deng, B. Dealing with stress in cats: what is new about the olfactory strategy? Front Vet Sci. (2022) 9:928943. doi: 10.3389/fvets.2022.928943
40. Lefman, SH, and Prittie, JE. Psychogenic stress in hospitalized veterinary patients: causation, implications, and therapies. J Vet Emerg Crit Care (San Antonio). (2019) 29:107–20. doi: 10.1111/vec.12821
41. Wallis, N, and Raffan, E. The genetic basis of obesity and related metabolic diseases in humans and companion animals. Genes (Basel). (2020) 11:1378. doi: 10.3390/genes11111378
42. Hegedus, C, Andronie, L, Uiuiu, P, Jurco, E, Lazar, EA, and Popescu, S. Pets, genuine tools of environmental pollutant detection. Animals. (2023) 13:2923. doi: 10.3390/ani13182923
43. Demirtas, B, Yanar, K, Koenhemsi, L, Erozkan Dusak, N, Guzel, O, and Akdogan Kaymaz, A. Tobacco smoke induces oxidative stress and alters pro-inflammatory cytokines and some trace elements in healthy indoor cats. Vet Res Forum. (2023) 14:301–8. doi: 10.30466/vrf.2022.545424.3357
44. Wu, SY, Xing, F, Sharma, S, Wu, K, Tyagi, A, Liu, Y, et al. Nicotine promotes brain metastasis by polarizing microglia and suppressing innate immune function. J Exp Med. (2020) 217:e20191131. doi: 10.1084/jem.20191131
45. Manukyan, AL. Noise as a cause of neurodegenerative disorders: molecular and cellular mechanisms. Neurol Sci. (2022) 43:2983–93. doi: 10.1007/s10072-022-05948-6
46. Gröschel, M, Manchev, T, Fröhlich, F, Jansen, S, Ernst, A, and Basta, D. Neurodegeneration after repeated noise trauma in the mouse lower auditory pathway. Neurosci Lett. (2024) 818:137571. doi: 10.1016/j.neulet.2023.137571
47. Gogokhia, N, Pochkhidze, N, Japaridze, N, Bikashvili, T, and Zhvania, M. The effect of high intensity white noise on the ultrastructure of Axo-dendritic synapses in colliculus inferior of adult male cats. Quantitative Electron microscopic study. Georgian Med News. (2021) 320:178–82.
48. Tonnies, E, and Trushina, E. Oxidative stress, synaptic dysfunction, and Alzheimer's disease. J Alzheimers Dis. (2017) 57:1105–21. doi: 10.3233/JAD-161088
49. Aulsebrook, AE, Jones, TM, Mulder, RA, and Lesku, JA. Impacts of artificial light at night on sleep: a review and prospectus. J Exp Zool A Ecol Integr Physiol. (2018) 329:409–18. doi: 10.1002/jez.2189
50. Min, JY, and Min, KB. Outdoor light at night and the prevalence of depressive symptoms and suicidal behaviors: a cross-sectional study in a nationally representative sample of Korean adults. J Affect Disord. (2018) 227:199–205. doi: 10.1016/j.jad.2017.10.039
51. Obayashi, K, Saeki, K, Iwamoto, J, Ikada, Y, and Kurumatani, N. Exposure to light at night and risk of depression in the elderly. J Affect Disord. (2013) 151:331–6. doi: 10.1016/j.jad.2013.06.018
52. Fonken, LK, and Nelson, RJ. Dim light at night increases depressive-like responses in male C3H/HeNHsd mice. Behav Brain Res. (2013) 243:74–8. doi: 10.1016/j.bbr.2012.12.046
53. Fonken, LK, Kitsmiller, E, Smale, L, and Nelson, RJ. Dim nighttime light impairs cognition and provokes depressive-like responses in a diurnal rodent. J Biol Rhythm. (2012) 27:319–27. doi: 10.1177/0748730412448324
54. Fonken, LK, Finy, MS, Walton, JC, Weil, ZM, Workman, JL, Ross, J, et al. Influence of light at night on murine anxiety-and depressive-like responses. Behav Brain Res. (2009) 205:349–54. doi: 10.1016/j.bbr.2009.07.001
55. Postnov, D, Semyachkina-Glushkovskaya, O, Litvinenko, E, Kurths, J, and Penzel, T. Mechanisms of activation of Brain's drainage during sleep: the nightlife of astrocytes. Cells. (2023) 12:2667. doi: 10.3390/cells12222667
56. Ooms, S, Overeem, S, Besse, K, Rikkert, MO, Verbeek, M, and Claassen, JAHR. Effect of 1 night of total sleep deprivation on cerebrospinal fluid beta-amyloid 42 in healthy middle-aged men: a randomized clinical trial. JAMA Neurol. (2014) 71:971–7. doi: 10.1001/jamaneurol.2014.1173
57. Shokri-Kojori, E, Wang, GJ, Wiers, CE, Demiral, SB, Guo, M, Kim, SW, et al. beta-amyloid accumulation in the human brain after one night of sleep deprivation. Proc Natl Acad Sci USA. (2018) 115:4483–8. doi: 10.1073/pnas.1721694115
58. Ishida, K, and Yamada, K. Detection of Glymphatic outflow of tau from brain to cerebrospinal fluid in mice. Methods Mol Biol. (2024) 2754:351–9. doi: 10.1007/978-1-0716-3629-9_19
59. Duncan, MJ, Guerriero, LE, Kohler, K, Beechem, LE, Gillis, BD, Salisbury, F, et al. Chronic fragmentation of the daily sleep-wake rhythm increases amyloid-beta levels and Neuroinflammation in the 3xTg-AD mouse model of Alzheimer's disease. Neuroscience. (2022) 481:111–22. doi: 10.1016/j.neuroscience.2021.11.042
60. Mohlin, C, Taylor, L, Ghosh, F, and Johansson, K. Autophagy and ER-stress contribute to photoreceptor degeneration in cultured adult porcine retina. Brain Res. (2014) 1585:167–83. doi: 10.1016/j.brainres.2014.08.055
61. Kleiman, DG, and Eisenberg, JF. Comparisons of canid and felid social systems from an evolutionary perspective. Anim Behav. (1973) 21:637–59. doi: 10.1016/S0003-3472(73)80088-0
62. Flockhart, DTT, Norris, DR, and Coe, JB. Predicting free-roaming cat population densities in urban areas. Anim Conserv. (2016) 19:472–83. doi: 10.1111/acv.12264
63. Bradshaw, J. Normal feline behaviour: … and why problem behaviours develop. J Feline Med Surg. (2018) 20:411–21. doi: 10.1177/1098612X18771203
64. Finka, LR, and Foreman-Worsley, R. Are multi-cat homes more stressful? A critical review of the evidence associated with cat group size and wellbeing. J Feline Med Surg. (2022) 24:65–76. doi: 10.1177/1098612X211013741
65. Marinath, L, Vaz, J, Kumar, D, Thiyagesan, K, and Baskaran, N. Drivers of stereotypic behaviour and physiological stress among captive jungle cat (Felis chaus Schreber, 1777) in India. Physiol Behav. (2019) 210:112651. doi: 10.1016/j.physbeh.2019.112651
66. Vaz, J, Narayan, EJ, Dileep Kumar, R, Thenmozhi, K, Thiyagesan, K, and Baskaran, N. Prevalence and determinants of stereotypic behaviours and physiological stress among tigers and leopards in Indian zoos. PLoS One. (2017) 12:e0174711. doi: 10.1371/journal.pone.0174711
67. Elzerman, AL, DePorter, TL, Beck, A, and Collin, JF. Conflict and affiliative behavior frequency between cats in multi-cat households: a survey-based study. J Feline Med Surg. (2020) 22:705–17. doi: 10.1177/1098612X19877988
68. Turner, MLAD. Influence of indoor-cat group size and dominance rank on urinary cortisol levels. Anim Welf. (2023) 17:215–37. doi: 10.1017/S0962728600032152
69. Ramos, D, Reche-Junior, A, Fragoso, PL, Palme, R, Yanasse, NK, Gouvêa, VR, et al. Are cats (Felis catus) from multi-cat households more stressed? Evidence from assessment of fecal glucocorticoid metabolite analysis. Physiol Behav. (2013) 122:72–5. doi: 10.1016/j.physbeh.2013.08.028
70. Klintip, W, Jarudecha, T, Rattanatumhi, K, Ritchoo, S, Muikaew, R, Wangsud, S, et al. First study on stress evaluation and reduction in hospitalized cats after neutering surgery. Vet World. (2022) 15:2111–8. doi: 10.14202/vetworld.2022.2111-2118
71. Durr, R, and Smith, C. Individual differences and their relation to social structure in domestic cats. J Comp Psychol. (1997) 111:412–8. doi: 10.1037/0735-7036.111.4.412
72. Gorlova, A, Svirin, E, Pavlov, D, Cespuglio, R, Proshin, A, Schroeter, CA, et al. Understanding the role of oxidative stress, Neuroinflammation and abnormal myelination in excessive aggression associated with depression: recent input from mechanistic studies. Int J Mol Sci. (2023) 24:915. doi: 10.3390/ijms24020915
73. Karim, A, Anwar, F, Saleem, U, Fatima, S, Ismail, T, Obaidullah, AJ, et al. Administration of alpha-lipoic acid and silymarin attenuates aggression by modulating endocrine, oxidative stress and inflammatory pathways in mice. Metab Brain Dis. (2023) 38:2255–67. doi: 10.1007/s11011-023-01258-8
74. Jang, Y, Park, J, Rhee, MK, Lee, HW, Park, NS, Kim, Y, et al. Mental health impact of bullying by ethnic peers in senior housing: a study with older Korean American residents in the greater Los Angeles area. J Gerontol Soc Work. (2024) 67:575–87. doi: 10.1080/01634372.2024.2338071
75. Ibrahim, FM, Rashad Dabou, EA, AbdelSamad, S, and Abuijlan, IAM. Prevalence of bullying and its impact on self-esteem, anxiety and depression among medical and health sciences university students in RAS Al Khaimah, UAE. Heliyon. (2024) 10:e25063. doi: 10.1016/j.heliyon.2024.e25063
76. Vveinhardt, J, and Kaspare, M. Connections of bullying experienced by Kyokushin karate athletes with the psychological state: is "a cure for bullying" safe? Front Sports Act Living. (2024) 6:1304285. doi: 10.3389/fspor.2024.1304285
77. Yoshino, Y, and Dwivedi, Y. Elevated expression of unfolded protein response genes in the prefrontal cortex of depressed subjects: effect of suicide. J Affect Disord. (2020) 262:229–36. doi: 10.1016/j.jad.2019.11.001
78. Fujii, C, Zorumski, CF, and Izumi, Y. Endoplasmic reticulum stress, autophagy, neuroinflammation, and sigma 1 receptors as contributors to depression and its treatment. Neural Regen Res. (2024) 19:2202–11. doi: 10.4103/1673-5374.391334
79. Timberlake, MA, and Dwivedi, Y. Altered expression of endoplasmic reticulum stress associated genes in Hippocampus of learned helpless rats: relevance to depression pathophysiology. Front Pharmacol. (2015) 6:319. doi: 10.3389/fphar.2015.00319
80. Wandesforde-Smith, G, Levy, JK, Lynn, W, Rand, J, Riley, S, Schaffner, JE, et al. Coping with human-cat interactions beyond the limits of domesticity: moral pluralism in the Management of Cats and Wildlife. Front Vet Sci. (2021) 8:682582. doi: 10.3389/fvets.2021.682582
81. Tan, SML, Stellato, AC, and Niel, L. Uncontrolled outdoor access for cats: an assessment of risks and benefits. Animals. (2020) 10:258. doi: 10.3390/ani10020258
82. Wang, Q, Timberlake, MA II, Prall, K, and Dwivedi, Y. The recent progress in animal models of depression. Prog Neuro-Psychopharmacol Biol Psychiatry. (2017) 77:99–109. doi: 10.1016/j.pnpbp.2017.04.008
83. Timberlake, M, Prall, K, Roy, B, and Dwivedi, Y. Unfolded protein response and associated alterations in toll-like receptor expression and interaction in the hippocampus of restraint rats. Psychoneuroendocrinology. (2018) 89:185–93. doi: 10.1016/j.psyneuen.2018.01.017
84. Kuneš, J, Hojná, S, Mráziková, L, Montezano, A, Touyz, RM, and Maletínská, L. Obesity, cardiovascular and neurodegenerative diseases: potential common mechanisms. Physiol Res. (2023) 72:S73–90. doi: 10.33549/physiolres.935109
85. Schmitt, LO, and Gaspar, JM. Obesity-induced brain Neuroinflammatory and mitochondrial changes. Meta. (2023) 13:86. doi: 10.3390/metabo13010086
86. Godfrey, H, Morrow, S, Abood, SK, and Verbrugghe, A. Identifying the target population and preventive strategies to combat feline obesity. J Feline Med Surg. (2024) 26:1098612X241228042. doi: 10.1177/1098612X241228042
87. Martins, TDO, Ramos, RC, Possidonio, G, Bosculo, MRM, Oliveira, PL, Costa, LR, et al. Feline obesity causes hematological and biochemical changes and oxidative stress - a pilot study. Vet Res Commun. (2023) 47:167–77. doi: 10.1007/s11259-022-09940-5
88. Guillemot-Legris, O, and Muccioli, GG. Obesity-induced Neuroinflammation: beyond the hypothalamus. Trends Neurosci. (2017) 40:237–53. doi: 10.1016/j.tins.2017.02.005
89. Dekkers, IA, Jansen, PR, and Lamb, HJ. Obesity, brain volume, and white matter microstructure at MRI: a cross-sectional UK biobank study. Radiology. (2019) 291:763–71. doi: 10.1148/radiol.2019181012
90. Ou, X, Andres, A, Pivik, RT, Cleves, MA, and Badger, TM. Brain gray and white matter differences in healthy normal weight and obese children. J Magn Reson Imaging. (2015) 42:1205–13. doi: 10.1002/jmri.24912
91. Kim, AY, Shim, JH, Choi, HJ, and Baek, HM. Comparison of volumetric and shape changes of subcortical structures based on 3-dimensional image between obesity and normal-weighted subjects using 3.0 T MRI. J Clin Neurosci. (2020) 73:280–7. doi: 10.1016/j.jocn.2019.12.052
92. Zoran, DL, and Buffington, CA. Effects of nutrition choices and lifestyle changes on the well-being of cats, a carnivore that has moved indoors. J Am Vet Med Assoc. (2011) 239:596–606. doi: 10.2460/javma.239.5.596
93. Wu, G. Roles of nutrients in the brain development, cognitive function, and mood of dogs and cats. Adv Exp Med Biol. (2024) 1446:177–202. doi: 10.1007/978-3-031-54192-6_8
94. Wu, G. Recent advances in the nutrition and metabolism of dogs and cats. Adv Exp Med Biol. (2024) 1446:1–14. doi: 10.1007/978-3-031-54192-6_1
95. Cassiano, LMG, Oliveira, MS, Pioline, J, Salim, ACM, and Coimbra, RS. Neuroinflammation regulates the balance between hippocampal neuron death and neurogenesis in an ex vivo model of thiamine deficiency. J Neuroinflammation. (2022) 19:272. doi: 10.1186/s12974-022-02624-6
96. Ferroni, NM, Chertoff, MJ, Alberca, CD, Berardino, BG, Gianatiempo, O, Brahamian, M, et al. Oxidative stress associated with spatial memory impairment and social olfactory deterioration in female mice reveals premature aging aroused by perinatal protein malnutrition. Exp Neurol. (2023) 368:114481. doi: 10.1016/j.expneurol.2023.114481
97. Denenberg, S, Machin, KL, and Landsberg, GM. Behavior and cognition of the senior cat and its interaction with physical disease. Vet Clin North Am Small Anim Pract. (2024) 54:153–68. doi: 10.1016/j.cvsm.2023.09.001
98. MacQuiddy, B, Moreno, J, Frank, J, and McGrath, S. Survey of risk factors and frequency of clinical signs observed with feline cognitive dysfunction syndrome. J Feline Med Surg. (2022) 24:e131–7. doi: 10.1177/1098612X221095680
99. Černá, P, Gardiner, H, Sordo, L, Tørnqvist-Johnsen, C, and Gunn-Moore, DA. Potential causes of increased vocalisation in elderly cats with cognitive dysfunction syndrome as assessed by their owners. Animals. (2020) 10:1092. doi: 10.3390/ani10061092
100. Scuderi, C, and Golini, L. Successful and unsuccessful brain aging in pets: pathophysiological mechanisms behind clinical signs and potential benefits from Palmitoylethanolamide nutritional intervention. Animals. (2021) 11:2584. doi: 10.3390/ani11092584
101. Castillo, C, and Hernandez, J. The role of oxidative stress in the development of cognitive dysfunction syndrome in cats. Importance of antioxidant prevention and therapy. Symbiosis. (2015) 1:1–12. doi: 10.15226/2381-2907/1/2/00110
Keywords: neurodegeneration, fCDS, ER stress, oxidative stress, neuroinflammation
Citation: Niesman IR (2024) Stress and the domestic cat: have humans accidentally created an animal mimic of neurodegeneration? Front. Neurol. 15:1429184. doi: 10.3389/fneur.2024.1429184
Edited by:
Juan Pablo Palavicini, The University of Texas Health Science Center at San Antonio, United StatesReviewed by:
M. Heather West Greenlee, Iowa State University, United StatesMartí Pumarola, Autonomous University of Barcelona, Spain
Copyright © 2024 Niesman. This is an open-access article distributed under the terms of the Creative Commons Attribution License (CC BY). The use, distribution or reproduction in other forums is permitted, provided the original author(s) and the copyright owner(s) are credited and that the original publication in this journal is cited, in accordance with accepted academic practice. No use, distribution or reproduction is permitted which does not comply with these terms.
*Correspondence: Ingrid R. Niesman, aW5pZXNtYW5Ac2RzdS5lZHU=