- 1Department of Neurology, Northwell, New Hyde Park, NY, United States
- 2Zucker School of Medicine at Hofstra/Northwell, Hempstead, NY, United States
- 3Department of Psychiatry, Northwell, New Hyde Park, NY, United States
- 4Departments of Neurology and Psychiatry, Donald and Barbara Zucker School of Medicine at Hofstra/Northwell, Hempstead, NY, United States
- 5Feinstein Institute for Medical Research, Northwell Health, Manhasset, NY, United States
- 6Department of Radiology, Northwell, New Hyde Park, NY, United States
Fluorodeoxyglucose F18 (FDG) positron emission tomography (PET) imaging can help clinicians pursue the differential diagnosis of various neurodegenerative diseases. It has become an invaluable diagnostic tool in routine clinical practice in conjunction with computed tomography (CT) imaging, magnetic resonance imaging (MRI), and biomarker studies. We present a single-institution case series and systematic literature review, showing how FDG-PET imaging has helped physicians diagnose neurodegenerative diseases and their mimickers and how patient care was amended. A single institution analysis and comprehensive literature search were completed following Preferred Reporting Items for Systematic Reviews and Meta-Analyses (PRISMA) guidelines. These medical subjects’ headings (MeSH) terms were used: “FDG-PET” AND “dementia” OR “Alzheimer’s” OR “neurodegeneration” OR “frontotemporal dementia” OR “atypical parkinsonian syndrome” OR “primary progressive aphasia” OR “lewy body dementia.” The inclusion criteria included studies with uncertain diagnoses of neurocognitive disease resolved with FDG-PET, PET/MRI, or PET/CT hybrid imaging. A literature search resulted in 3,976 articles. After considering inclusion and exclusion criteria, 14 case reports and 1 case series were selected, representing 19 patients. The average age of patients was 70.8 years (range: 54–83 years). Five of the 19 patients were females. Dementia with Lewy bodies (DLB) had the highest propensity for being misidentified as another neurodegenerative disease, followed by Alzheimer’s disease (AD) and frontotemporal dementia (FTD). Without accurate molecular imaging, neurodegenerative diseases may be missed or misdiagnosed. Our single-institution case series and literature review demonstrate how FDG-PET brain imaging can be used to correct and clarify preexisting clinical diagnoses of neurodegenerative disease.
Introduction
FDG-PET, or [18F]-fluorodeoxyglucose positron emission tomography, is a powerful tool in medical research. Since its introduction in Alzheimer’s disease (AD) in the 1980s, FDG-PET has complemented the clinical examination and cerebrospinal fluid (CSF) serology by providing additional information on the pathophysiology of many neurodegenerative diseases (1). With overlapping symptoms between various neurodegenerative diseases, FDG-PET can help to clarify diagnoses by revealing distinct metabolic patterns, making it a crucial diagnostic biomarker (2). In head-to-head studies, FDG-PET stereotactic surface projection (SSP) metabolic and statistical maps have been superior to clinical assessment regarding interrater reliability and diagnostic accuracy (3). It is a valuable complement to other diagnostic tools, as it enhances staging and monitoring of the extent and location of neurodegenerative disease, particularly tracking the progression of the disease over time (2, 4). In turn, earlier diagnosis with FDG-PET and confirmation with amyloid-PET and tau-PET can assist physicians in enrolling patients with clinical trials and pathology-specific disease-modifying therapies (5–7).
FDG-PET works by administering a radioactive tracer, [18F]-FDG, which, when taken up by metabolically active glial and neural cells, emits positrons that are detected by a scanner and computed into an image highlighting regions of the brain with increased or decreased glucose uptake (8). Diagnosing neurodegenerative diseases based on patterns of decreased glucose uptake was previously not possible using imaging techniques such as computed tomography (CT) or MRI, which mainly focused on detecting cortical atrophy (5). AD, the most common cause of dementia, is typically characterized by decreased glucose metabolism in the precuneus, posterior cingulate gyrus, and temporoparietal cortex, with sparing of the anterior cingulate, perirolandic region, visual cortex, basal ganglia, thalami, occipital lobes, brainstem and cerebellum (9, 10). FTD demonstrates a pattern of decreased glucose uptake in the frontal and anterior temporal lobes, with common involvement of the caudate nuclei and orbitofrontal region (11, 12). DLB is distinguished by decreased glucose metabolism in the parieto-occipital and temporal regions, including in the medial occipital lobes (cuneus and primary visual cortex), with sparing of the posterior cingulate (cingulate island sign) (12–21).
FDG-PET is typically used with clinical data, neuropsychological assessment, additional imaging modalities such as MRI, and cCSF measurements of β-amyloid and tau to differentiate between neurodegenerative diseases (22, 23). FDG-PET evidence with an Alzheimer’s pattern may indicate a lumbar puncture to determine a CSF Amyloid Tau Index (ATI), where reduced CSF levels of Aβ42-decreased Aβ42/40 ratio and increased CSF levels of tau, and phosphorylated tau can be used to confirm the diagnosis of AD (24, 25). Similarly, an Amyloid PET that detects cerebral Aβ deposition would help confirm amyloid pathology (26–28). These tests provide pathological evidence of disease and are necessary for inclusion in clinical trials and to assign patients to novel antiamyloid treatments (29). Similarly, when FTD is suspected, FDG-PET can trigger further investigations, such as the utilization of other radiotracers, including tau PET (30, 31).
PET radiotracers, such as [18F]-florbetapir, [18F]-florbetaben, and [18F]-flutemetamol, can be used to localize cortical amyloid deposition, whereas [18F]-flortaucipir can be used to localize regions of the brain with tau accumulation (7, 32, 33). In most studies, FDG is used to detect hypometabolism. In less frequent cases, patients affected by autoimmune encephalopathy (AEI), which is a known cause of rapidly progressing dementia, may display variable increased or decreased FDG uptake (34–37).
FDG-PET imaging is best combined with structural imaging such as MRI (PET/MRI), which can provide information on lobar-specific atrophy patterns unique to different types of dementia and can be particularly useful in defining the contribution of vascular pathology to ongoing clinical and metabolic phenotypes, as well as detecting co-pathologies such as cerebral amyloid angiopathy (CAA) (23). Notably, MRI provides precise and consistent information, helping overcome anatomic localization difficulties inherent to PET. The ease of acquiring PET and MRI in a single session on a hybrid system minimizes patient discomfort while maximizing clinical information and optimizes the co-registration of both modalities (38).
While FDG-PET is beneficial in helping to ascertain the diagnosis of neurodegenerative diseases, physicians and researchers should be aware of its limitations, leading to artifactual findings, and consider a patient’s underlying comorbidities and medications. Diabetic medications, such as insulin and metformin, can affect FDG biodistribution into skeletal and cardiac tissue (39). It is generally recommended to discontinue metformin 48 h before FDG-PET to standardize scan quality, though some studies suggest modifying the protocol based on the specific scan requirements (40). Brown fat is a potential confounder during FDG-PET as it exhibits high FDG uptake (41). β Blockers, such as propranolol, have been used to reduce brown adipose uptake of FDG, which has been helpful in the evaluation of tumors (42). Medications that can alter the cerebral metabolism of FDG include sedatives, narcotics, antipsychotic medications, and steroids (43). Therefore, a thorough evaluation of the patient’s medications should be completed to ensure reproducibility.
Ultimately, FDG-PET’s utility in distinguishing between dementia subtypes can help guide treatment options, potentially optimizing the therapeutic approach, enabling the enrollment of patients in appropriate clinical trials (44, 45), and, importantly, informing prognosis. The psychosocial implications of improving diagnostic accuracy should also be emphasized: the emotional frustration associated with false or uncertain diagnoses can be challenging for patients and caregivers alike, hence the need to improve the sensitivity and specificity of diagnosis. Here, we present a single-institution case series (Northwell Health, New York) and review pertinent literature wherein FDG-PET was utilized to obtain an accurate diagnosis of neurodegenerative disease, thereby allowing proper management. Patients were seen by a neurologist and, where applicable, a psychiatrist, both specialists in neurodegenerative diseases. Imaging was performed in the appropriate neuroradiology and nuclear medicine departments and read by a board-certified, fellowship-trained neuroradiologist with over a decade of clinical and research expertise focused on dementia and neurodegenerative disorders.
Methods
Literature search strategy
A comprehensive literature search was completed following the Preferred Reporting Items for Systematic Reviews and Meta-Analyses (PRISMA) guidelines. Titles and abstracts in English were searched across the PubMed citation database. No date limit was used, and articles were updated until April 2023. The medical subjects’ headings (MeSH) terms were used: “FDG-PET” AND “dementia” OR “Alzheimer’s” OR “neurodegeneration” OR “frontotemporal dementia” OR “atypical parkinsonian syndrome” OR “primary progressive aphasia” OR “lewy body dementia.”
Selection criteria
The inclusion criteria were (1) studies with uncertain diagnoses of dementia that were resolved using FDG-PET, and (2) studies published in a peer-reviewed journal. The exclusion criteria consisted of studies with non-human subjects, reviews, editorials, expert opinion, abstract-only articles, articles unable to be obtained, and articles not relevant to the inclusion criteria. The search resulted in 3,976 results, of which 1,927 were removed for being duplicates. The remaining 2,049 articles were screened using the exclusion criteria, resulting in 16 relevant articles. One full-text article was unable to be obtained. In total, 15 reports were included, comprising 14 case reports and 1 case series of 5 patients (Figure 1; Table 1).
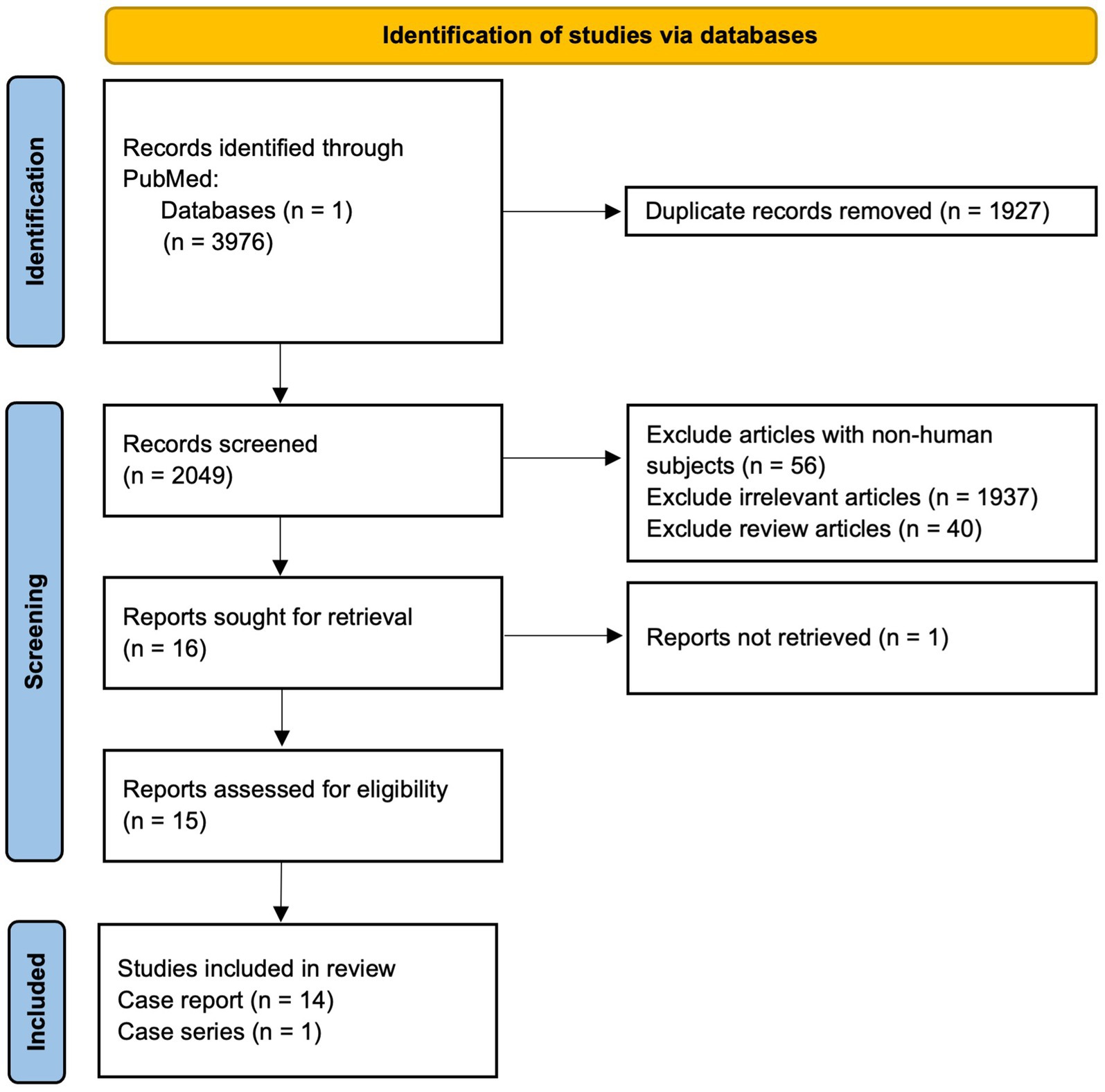
Figure 1. Preferred Reporting Items for Systematic Reviews and Meta-Analyses (PRISMA) schemata: diagrammatic representation of literature searches utilizing PubMed database.
Case presentations
Patient 1
A 67-year-old woman with baseline dementia, depression, and urinary incontinence presented for acute-on-chronic cognitive impairment. She was working as a high-functioning secretary until 2 years prior, after which cognitive issues prevented her from working further. She was noted to lose track of tasks and demonstrated difficulties with driving. The patient’s mood and sleep patterns had suffered during her illness. In the outpatient setting, the patient was empirically administered increasing doses of donepezil, with no improvement. She was diagnosed with mild cognitive impairment by her former neurologist and depression by her primary care physician.
When evaluated for a second opinion, she demonstrated deficits in clock drawing, trail-making, and remembering presidents. She was oriented to person, place, and time. She demonstrated neither signs of motor, dynamic, ideomotor, and ideational nor dressing apraxia. Her language ability was intact. She displayed adequate knowledge of personal and past history. She scored 24/30 on her mini-mental status examination (MMSE). There were no recorded deficits in motor strength, coordination, or sensation. Serum studies demonstrated normal antinuclear antibodies (ANA), thiamine, anti-Ro, anti-La, thyroid stimulating hormone (TSH), free T3/free T4 (fT3/T4), thyroid peroxidase (TPO) and thyroglobulin (TG) antibodies, syphilis screen, borrelia IgG/IgM antibodies, C-reactive protein (CRP). Brain MRI showed mild atrophy in the cortical and subcortical structures in the temporal lobe but not immediately indicative of AD. Brain FDG-PET showed moderate hypometabolism in the parietotemporal regions (right greater than left), which extended into the frontal lobes. There was accompanying mild hypometabolism in the precuneus and posterior cingulate gyri, especially on the right side, regions typically involved early in the setting of AD (Figure 2). This pattern suggested moderately advanced AD. An amyloid PET supported a pathological diagnosis by demonstrating significant cortical β-amyloid deposition. The patient was successfully screened and enrolled in an AD clinical trial while her mood changes were addressed and managed pharmacologically and behaviorally.
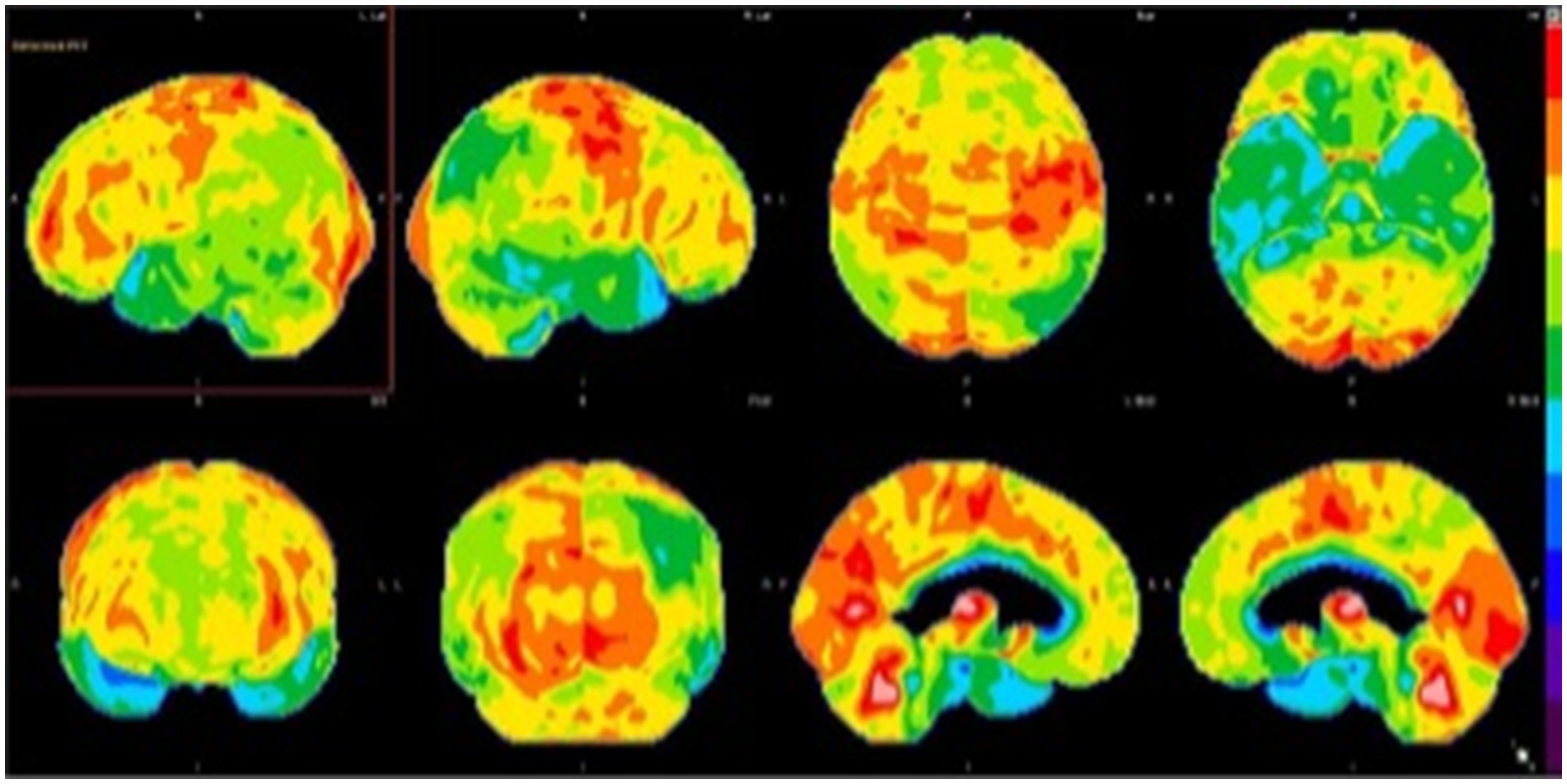
Figure 2. Brain FDG-PET imaging: 3D stereotactic surface projection maps demonstrate moderate hypometabolism in the parietotemporal regions (right greater than left), which extends into the frontal lobes. There is accompanying mild hypometabolism in the precuneus and posterior cingulate gyri, especially on the right side, regions typically involved early in the setting of Alzheimer’s disease.
Patient 2
A 55-year-old man presented to the clinic with noted changes in behavior. He worked as an architect but lost his job due to cognitive decline. He complained of losing interest in many of his hobbies and forgetting basic tasks such as paying bills. On physical exam, the patient demonstrated full orientation, and difficulties with short-term memory and attention span but intact registration and concentration. There were neither language difficulties nor deficits in recalling current events. He scored 24/30 on his Montreal Cognitive Assessment (MoCA). Cranial nerve, motor, sensory, coordination, and reflexes were intact. Brain MRI demonstrated frontal atrophy and significant atrophy of the precuneus. Combined with apathy and depressed mood, the patient was diagnosed with a possible behavioral variant of frontotemporal dementia (bvFTD) and started on rivastigmine. Later, brain FDG-PET demonstrated severe hypometabolism in the parietotemporal regions, left greater than right, with accompanying hypometabolism in the precuneus and posterior cingulate gyri bilaterally (Figure 3). These findings were compatible with advanced AD rather than bvFTD. Subsequently, the patient was referred to and successfully enrolled in an AD clinical trial, with pathological confirmation supported by amyloid PET.
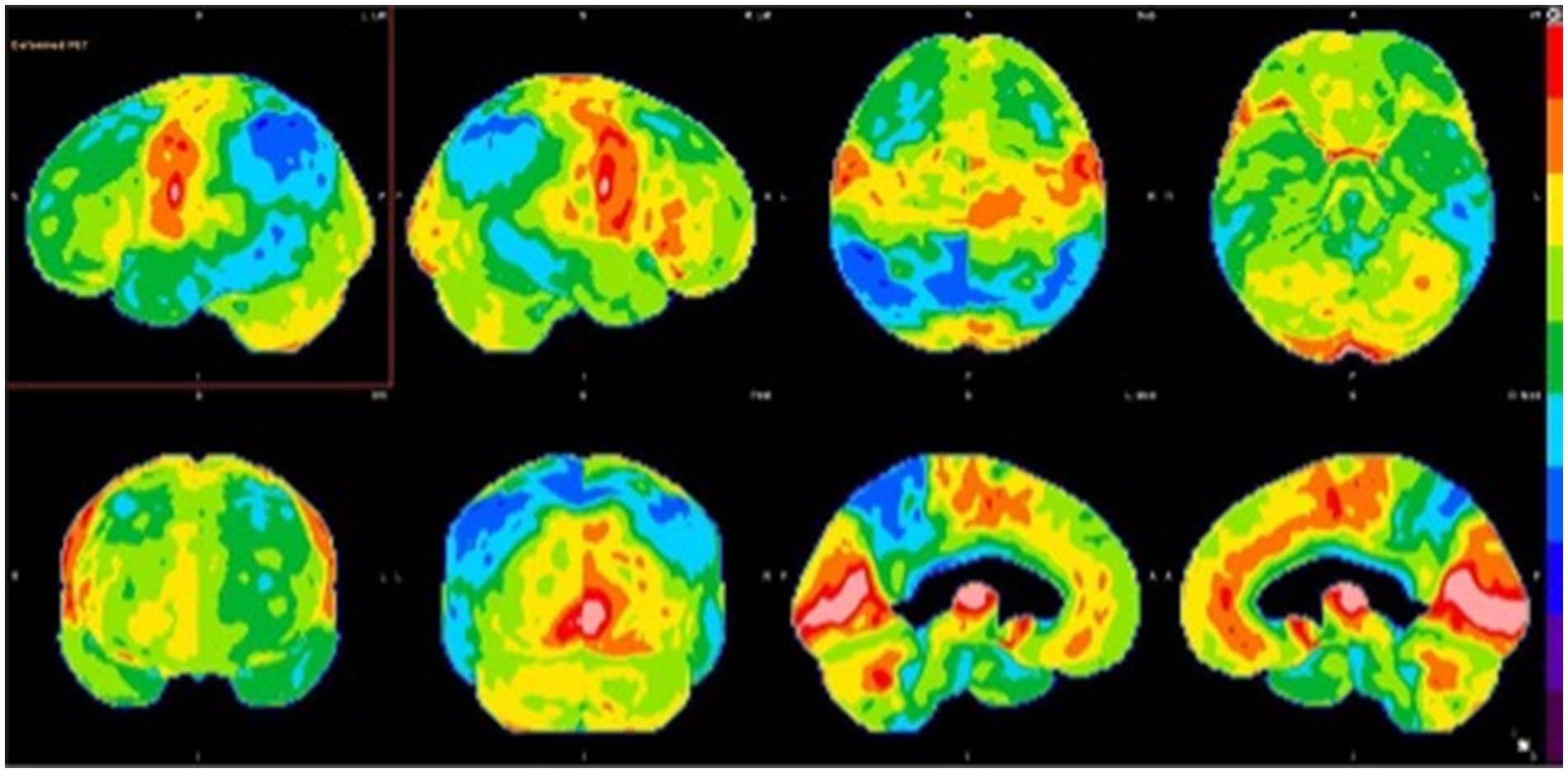
Figure 3. Brain FDG-PET imaging: 3D stereotactic surface projection maps demonstrate severe hypometabolism in the parietotemporal regions, left greater than right, with accompanying hypometabolism in the precuneus and posterior cingulate gyri bilaterally. There is extension of hypometabolism into the frontal lobes, indicating advanced neurodegenerative disease.
Patient 3
A 60-year-old man with ongoing severe bipolar disorder presented for 1-year cognitive decline with acute exacerbation over the prior 2 months. Previously, he was a high-functioning individual but started to complain of difficulties following commands and staying organized with his thoughts.
On exam, the patient could recall four out of the previous five presidents. Upon neurocognitive testing, he was observed to have no difficulties with clock drawing, spiral drawing, and alternating patterns; he demonstrated noticeable errors in trail-making and fluency. During dynamic testing, testing for praxis was generally normal, with only difficulty in the left hand. He scored 26/30 on MMSE. Orientation, attention, language, fund of knowledge, cranial nerves, motor, coordination, and reflexes were normal or unimpaired. The sensory exam was generally intact with reduced vibration and position sense in the distal lower and upper extremities. Laboratory tests demonstrated negative encephalopathy antibody panel in serum (ENS1, Mayo Clinic Laboratories) and CSF (ENC1, Mayo Clinic Laboratories), syphilis screening, p-ANCA, c-ANCA, cryoglobulin, anti SS-A, SS-B, anti-Smith, erythrocyte sedimentation rate (ESR), antiribonucleoprotein and borrelia screening, and indeterminate atypical ANCA; normal levels of methylmalonic acid, homocysteine, vitamins B1, B2, B5, B6, B9, and B12, arsenic, lead, mercury, cadmium, TPO and TG antibodies, C-reactive protein, thyroid stimulating hormone (TSH), and fT3/T4. A brain MRI demonstrated no abnormal parenchymal or leptomeningeal enhancement and an incidental pituitary microadenoma. An FDG-PET, performed in the context of the acute changes, hospitalization, and significant medication regimen, demonstrated abnormal hypometabolism in the parietal–temporal regions with involvement of the precuneus and posterior cingulate gyri, extending into the frontal lobes (Figure 4A). Given the imaging findings, the patient was initially diagnosed with AD. The patient was started on donepezil with graduating doses. In the following months, the patient developed significant Parkinsonism and was noted to be significantly bradykinetic and rigid with asterixis. Upon further evaluation in our clinic for a second opinion, we conducted a full medication overhaul, with a reduction of anti-D2 and sedating medications, preferring targeted antidepressants and low-dose atypical antipsychotics, among others. He was followed for several months, and we noticed a significant improvement in his cognitive and motor status, while his behavioral issues remained persistent, to the point of being institutionalized for a brief period (also in relation to a lack of stable home and care by family). A repeated FDG-PET brain scan (18 months after the first FDG-PET) demonstrated interval improvement in metabolic deficits compared to the previous FDG-PET (Figure 4B). This confirmed the suspicion of pseudodementia, likely due to overmedication in the setting of bipolar disorder. Further psychiatric care is underway, with significant improvement in the patient’s behavioral status.
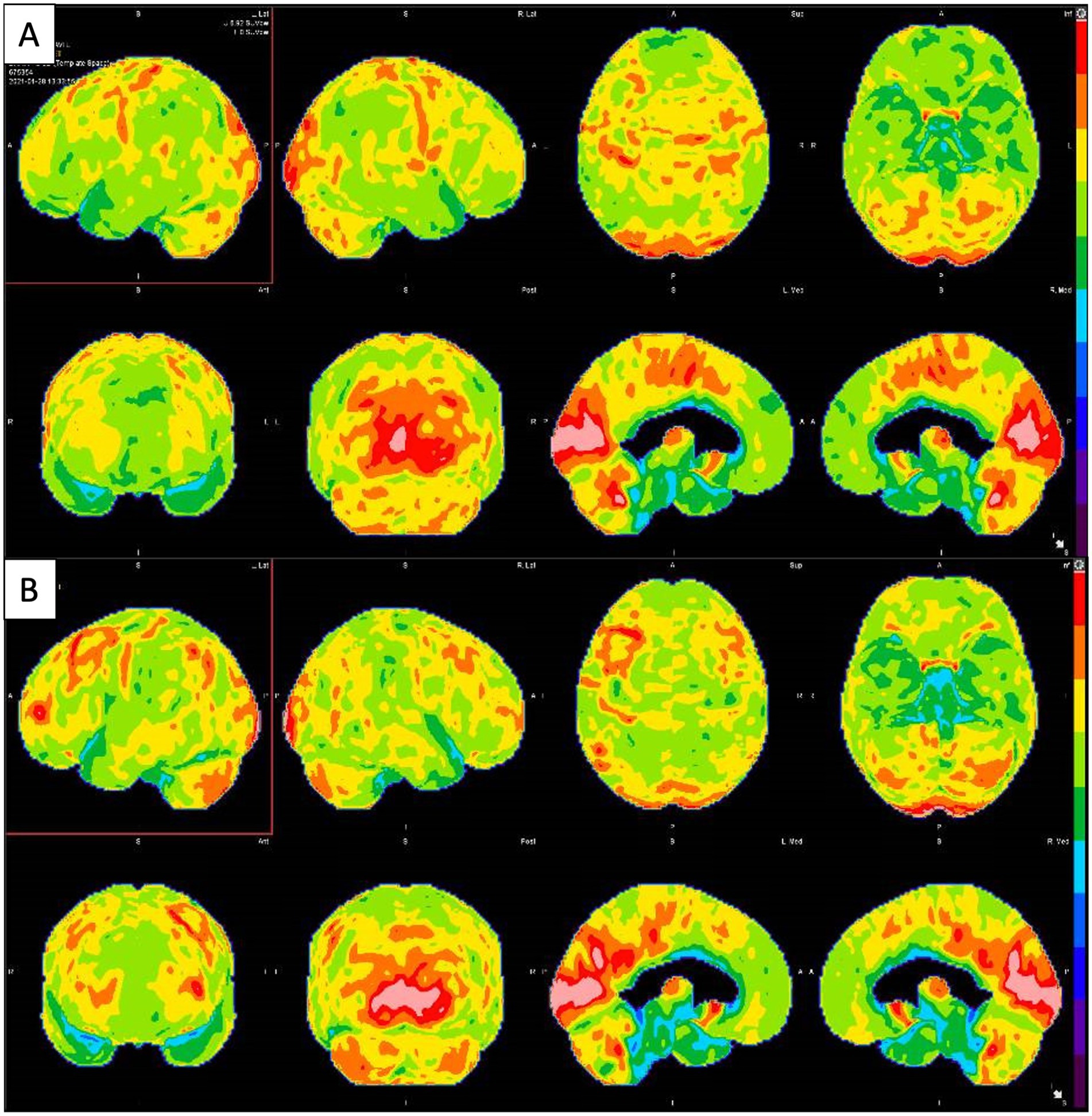
Figure 4. (A) Brain FDG-PET imaging: 3D stereotactic surface projection maps demonstrate mild to moderate hypometabolism in the bilateral parietotemporal regions (slightly more pronounced on the left side), which extends into the frontal lobes. There is accompanying mild hypometabolism in the precuneus and posterior cingulate gyri, regions typically involved early in the setting of Alzheimer’s disease. (B) Second Brain FDG-PET imaging taken 18 months after the first showing improvement in metabolic deficits.
Patient 4
An 82-year-old man with a previous diagnosis of pseudodementia due to depression presented with subacute onset of mild–moderate motor aphasia, initially ascribed to a possible stroke. Upon evaluation, brain MRI did not show significant vascular lesions. He was also noted to have unintentionally lost a significant amount of weight in relation to poor appetite and side effects of neurotropic medications; he was also in recent depressive episodes. His orientation, memory, attention, language, and fund of knowledge were intact in testing his mental status. He scored 27/30 on MMSE. He recalled 4/5 previous presidents. Testing for alternating patterns, spirals, and repetitions was normal. The clock drawing was abnormal as the patient drew numbers counterclockwise. He did not demonstrate apraxia though slow processing was noticeable.
During neuropsychological testing, the patient understood the test and maintained adequate engagement. His thought processes were logical and goal directed. His speech was hesitant and notable for slow prosody and hypophonia. No spastic speech was elicited, but it was noticeable for being hesitant, halting with effort, and more limitation in gutturals. Brain FDG-PET demonstrated asymmetric hypometabolism in the left frontal lobe, including in the left dorsal frontal, peri-insular/perisylvian regions, with involvement of the left frontal operculum (pars triangularis and pars opercularis of the left inferior frontal gyrus—expected Broca’s area) and left supplementary motor area. (Figure 5A) Imaging also revealed right greater than left cerebellar hypometabolism, indicating a component of crossed cerebral diaschisis. Given neuropsychological testing and imaging, the patient’s condition was suggestive of corticobasal degeneration (CBD) with a non-fluent/agrammatic primary progressive aphasia (nfvPPA) component. The patient was started on several trials of cholinesterase inhibitors, which he did not tolerate; similarly, dopaminergic medications were started, with the benefit only from selegiline but also poorly tolerated. A year later, repeated PET-MRI demonstrated similar findings (Figure 5B). At this time, the patient’s clinical picture had progressed to CBD, with a profound nfvPPA phenotype.
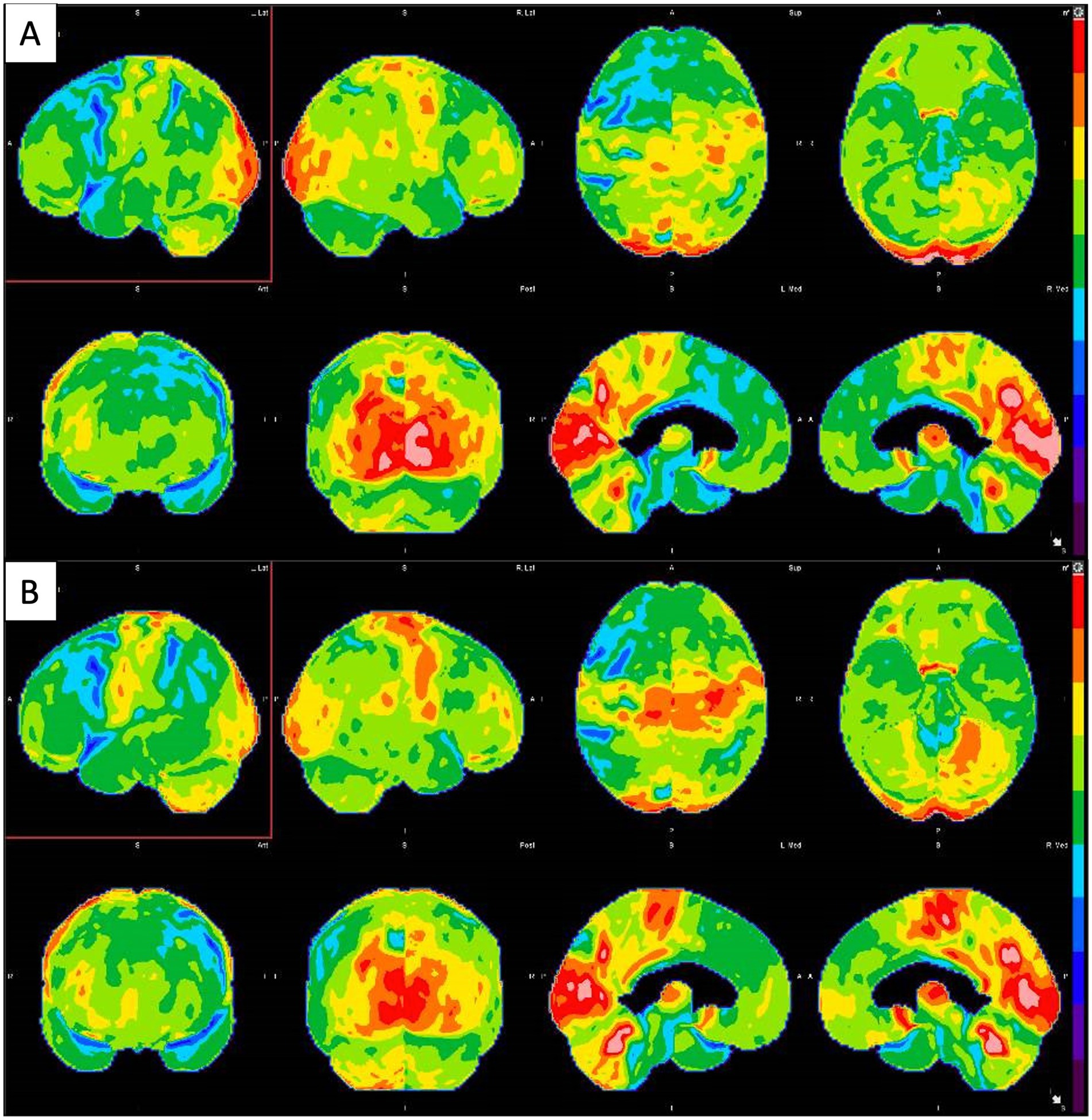
Figure 5. (A) Brain FDG-PET imaging: Three-dimensional stereotactic surface projection (SSP) maps demonstrate severe asymmetric hypometabolism in the left frontal lobe, particularly involving the left dorsolateral and fronto-insular region, including the left frontal operculum (pars opercularis and pars triangularis of the left inferior frontal gyrus, expected Broca’s area). There is accompanying crossed cerebellar diaschisis, with right greater than left cerebellar hypometabolism. (B) Second Brain FDG-PET imaging taken 12 months after the first showed similar hypometabolism distributions.
Results
Four patients were studied as part of our single-institution case series (Table 2). Nineteen patients were included as part of our literature review (Table 1). Six of these 23 patients were female. Two patients were diagnosed with AD before the age of 65 and, thus, qualified as early onset AD (10). Sixteen out of 23 patients had a brain MRI before FDG-PET for initial diagnosis. One patient had a CT scan (12). Six patients did not have an initial structural scan (18, 21). DLB was the most common neurodegenerative disorder that was initially misdiagnosed and then corrected through imaging (12, 15–19, 21). Most studies involved patients who were initially diagnosed with an alternative neurodegenerative disease and, through FDG-PET, were able to shift to the correct diagnosis. Some studies described patients with neurological symptoms, where the utilization of FDG-PET clarified the correct diagnosis (10, 17, 18).
Discussion
Brain FDG-PET is a desirable imaging modality that aids in the diagnosis of neurodegenerative disease. However, its cost may contribute to its limited use and availability, regardless of whether its radioisotopes are purchased or produced on-site (46). Our study highlights how FDG-PET can supplement or alter a preexisting clinical diagnosis. With an accurate diagnosis and in conjunction with pathological confirmation with amyloid and tau PET, patients may be started on treatment with resulting improvements seen through clinical symptoms and imaging (6, 15, 19, 47), or to access appropriate clinical trials (29, 48). FDG-PET should not be the sole study used to diagnose neurodegenerative disease. However, other imaging and biomarker studies are also fallible, especially when parsing through neurodegenerative diseases with similar initial presentations. FTD diagnosis can be challenging since its neurological symptoms can overlap with other neurodegenerative diseases, and its behavioral symptoms can mimic psychiatric disorders. Similarly, early presentation of DLB can be confused with those of AD, FTD, psychosis, and Parkinson’s disease dementia. The ability of FDG-PET to clarify some neurological diagnoses is becoming increasingly recognized, especially in the setting of emerging disease-modifying therapies that can lead to improved patient outcomes (27, 29, 48, 49). Of note, the combination of FDG-PET and clinical judgment can also lead to reversing the diagnosis of neurodegenerative dementia, as discussed in the subsection, “Patient 3.” FDG-PET diagnostic accuracy can be improved with several semi-quantitative methods. Z-scores measure a patient’s FDG update about the general population mean in terms of standard deviations and have been shown to improve the accuracy of readings (50). An abnormal finding would be reflected in a higher average Z-score (51). Centiloid scores are a semiquantitative method to improve accuracy in amyloid PET interpretation. The score quantifies the amyloid burden in the brain and normalizes the findings across different scanners and protocols, improving interstudy and intercenter interpretation (52, 53). The limitations of the current study lie in its retrospective nature. Thirteen patients in our study did not have an MMSE, which made it difficult to characterize the baseline cognitive impairment. In addition, only a few studies had a repeat MMSE following treatment (54). The patients described by Caffarra et al. (55) and Schönecker et al. (10) had clear symptoms of neurological decline but without a formal diagnosis. One patient underwent only computer tomography imaging but no MRI (12). The future studies can explore the use of other PET radiotracers, such as radiopharmaceuticals targeting tau (30), amyloid (32), FDOPA (56–58), TSPO (59, 60), and α-synuclein (61), in helping to diagnose neurodegenerative disorders accurately. In our current study, only a few patients were scanned with FDG and another tracer, such as amyloid PET (10, 17, 19). Others utilized single-photon emission computer tomography (SPECT) and dopamine active transporter (DaT) scans to supplement existing FDG-PET data (12, 17, 19, 20, 55).
In conclusion, FDG-PET can be a useful tool for diagnosing different subtypes of dementia with challenging clinical presentations. Additional investigation into the role of FDG-PET, for example, in psychiatric disorders, would be welcomed and may help improve the diagnosis and management of patients with dementia.
Conclusion
The differential diagnosis of neurodegenerative disorders and dementia subtypes, including Alzheimer’s disease, FTD, dementia with Lewy bodies, atypical Parkinsonism, and pseudodementia can be challenging in patients with an unclear clinical presentation or inconclusive biomarkers. Dementia subtypes have unique FDG uptake patterns that can be used to aid in establishing an accurate diagnosis. Our case series and literature review highlight using FDG-PET brain imaging to correct and clarify preexisting clinical diagnoses of neurodegenerative disease.
Author contributions
BH: Conceptualization, Investigation, Methodology, Writing – original draft, Writing – review & editing. SS: Data curation, Formal analysis, Investigation, Methodology, Writing – original draft, Writing – review & editing. CH: Data curation, Formal analysis, Investigation, Methodology, Writing – original draft, Writing – review & editing. PM: Writing – review & editing. MG: Writing – review & editing. AF: Visualization, Writing – review & editing. LG: Conceptualization, Formal analysis, Methodology, Supervision, Writing – review & editing.
Funding
The author(s) declare that no financial support was received for the research, authorship, and/or publication of this article.
Acknowledgments
The authors acknowledge Emma Kaye, NP, DNP, for providing care to patients as part of the neurological team.
Conflict of interest
The authors declare that the research was conducted in the absence of any commercial or financial relationships that could be construed as a potential conflict of interest.
Publisher’s note
All claims expressed in this article are solely those of the authors and do not necessarily represent those of their affiliated organizations, or those of the publisher, the editors and the reviewers. Any product that may be evaluated in this article, or claim that may be made by its manufacturer, is not guaranteed or endorsed by the publisher.
References
1. Chételat, G . How to use neuroimaging biomarkers in the diagnosis framework of neurodegenerative diseases? Rev Neurol (Paris). (2022) 178:490–7. doi: 10.1016/j.neurol.2022.03.006
2. Chételat, G, Arbizu, J, Barthel, H, Garibotto, V, Law, I, Morbelli, S, et al. Amyloid-PET and 18F-FDG-PET in the diagnostic investigation of Alzheimer’s disease and other dementias. Lancet Neurol. (2020) 19:951–62. doi: 10.1016/S1474-4422(20)30314-8
3. Foster, NL, Heidebrink, JL, Clark, CM, Jagust, WJ, Arnold, SE, Barbas, NR, et al. FDG-PET improves accuracy in distinguishing frontotemporal dementia and Alzheimer’s disease. Brain. (2007) 130:2616–35. doi: 10.1093/brain/awm177
4. Grothe, MJ, Barthel, H, Sepulcre, J, Dyrba, M, Sabri, O, Teipel, SJ, et al. In vivo staging of regional amyloid deposition. Neurology. (2017) 89:2031–8. doi: 10.1212/WNL.0000000000004643
5. Burkett, BJ, Babcock, JC, Lowe, VJ, Graff-Radford, J, Subramaniam, RM, and Johnson, DR. PET imaging of dementia: update 2022. Clin Nucl Med. (2022) 47:763–73. doi: 10.1097/RLU.0000000000004251
6. Rabinovici, GD, Gatsonis, C, Apgar, C, Chaudhary, K, Gareen, I, Hanna, L, et al. Association of amyloid positron emission tomography with subsequent change in clinical management among Medicare beneficiaries with mild cognitive impairment or dementia. JAMA. (2019) 321:1286–94. doi: 10.1001/jama.2019.2000
7. Cassinelli Petersen, G, Roytman, M, Chiang, GC, Li, Y, Gordon, ML, and Franceschi, AM. Overview of tau PET molecular imaging. Curr Opin Neurol. (2022) 35:230–9. doi: 10.1097/WCO.0000000000001035
8. Kapoor, M, and Kasi, A. PET scanning. StatPearls Publishing; (2022). Available at: https://www.ncbi.nlm.nih.gov/books/NBK559089/
9. Marcus, C, Mena, E, and Subramaniam, RM. Brain PET in the diagnosis of Alzheimer’s disease. Clin Nucl Med. (2014) 39:e413–26. doi: 10.1097/RLU.0000000000000547
10. Schönecker, S, Brendel, M, Huber, M, Vollmar, C, Huppertz, H-J, Teipel, S, et al. Applied multimodal diagnostics in a case of presenile dementia. BMC Neurol. (2016) 16:131. doi: 10.1186/s12883-016-0647-7
11. Di Battista, ME, Dell’Acqua, C, Baroni, L, Fenoglio, C, Galimberti, D, and Gallucci, M. Frontotemporal dementia misdiagnosed for post-treatment Lyme disease syndrome or vice versa? A Treviso dementia (TREDEM) registry case report. J Alzheimers Dis. (2018) 66:445–51. doi: 10.3233/JAD-180524
12. Gallucci, M, Dell’Acqua, C, Boccaletto, F, Fenoglio, C, Galimberti, D, and Di Battista, ME. Overlap between frontotemporal dementia and dementia with Lewy bodies: a Treviso dementia (TREDEM) registry case report. J Alzheimers Dis. (2019) 69:839–47. doi: 10.3233/JAD-181298
13. Mak, E, Su, L, Williams, GB, and O’Brien, JT. Neuroimaging characteristics of dementia with Lewy bodies. Alzheimers Res Ther. (2014) 6:18. doi: 10.1186/alzrt248
14. Jeong, Y, Cho, SS, Park, JM, Kang, SJ, Lee, JS, Kang, E, et al. 18F-FDG PET findings in frontotemporal dementia: an SPM analysis of 29 patients. J Nucl Med. (2005) 46:233–9. Available at: https://www.ncbi.nlm.nih.gov/pubmed/15695781.
15. Suantio, AM, Huang, HL, Kwok, CSN, Teo, DCH, and Nguyen, MH. FDG-PET in suspected dementia with Lewy bodies: a case report. BMC Geriatr. (2019) 19:150. doi: 10.1186/s12877-019-1166-3
16. Tun, MZ, Soo, WK, Wu, K, and Kane, R. Dementia with Lewy bodies presenting as probable epileptic seizure. BMJ Case Rep. (2017) 2017:1–3. doi: 10.1136/bcr-2017-221454
17. Van Der Gucht, A, Cleret de Langavant, L, Bélissant, O, Rabu, C, Cottereau, A-S, Evangelista, E, et al. Brain (18) F-FDG, (18) F-Florbetaben PET/CT, (123) I-FP-CIT SPECT and cardiac (123) I-MIBG imaging for diagnosis of a “cerebral type” of Lewy body disease. Nucl Med Mol Imaging. (2016) 50:258–60. doi: 10.1007/s13139-016-0394-0
18. Yoo, J, and Cheon, M. Differential diagnosis of patients with atypical parkinsonian syndrome using F-FDG and F-FP CIT PET: a report of five cases. Radiol Case Rep. (2022) 17:2765–70. doi: 10.1016/j.radcr.2022.05.007
19. Bouter, C, Hansen, N, Timäus, C, Wiltfang, J, and Lange, C. Case report: the role of neuropsychological assessment and imaging biomarkers in the early diagnosis of Lewy body dementia in a patient with major depression and prolonged alcohol and benzodiazepine dependence. Front Psych. (2020) 11:684. doi: 10.3389/fpsyt.2020.00684
20. Gallucci, M, Pallucca, C, Di Battista, ME, Bergamelli, C, Fiore, V, Boccaletto, F, et al. Anti-cholinergic derangement of cortical metabolism on 18F-FDG PET in a patient with frontotemporal lobar degeneration dementia: a case of the TREDEM registry. J Alzheimers Dis. (2020) 74:1107–17. Available at: https://www.medra.org/servlet/aliasResolver?alias=iospress&doi=10.3233/JAD-191290.
21. Al-Faham, Z, Zein, RK, and Wong, C-YO. 18F-FDG PET assessment of Lewy body dementia with cerebellar diaschisis. J Nucl Med Technol. (2014) 42:306–7. doi: 10.2967/jnmt.114.139295
22. Ahmed, RM, Paterson, RW, Warren, JD, Zetterberg, H, O’Brien, JT, Fox, NC, et al. Biomarkers in dementia: clinical utility and new directions. J Neurol Neurosurg Psychiatry. (2014) 85:1426–34. doi: 10.1136/jnnp-2014-307662
23. Dukart, J, Mueller, K, Horstmann, A, Barthel, H, Möller, HE, Villringer, A, et al. Combined evaluation of FDG-PET and MRI improves detection and differentiation of dementia. PLoS One. (2011) 6:e18111. doi: 10.1371/journal.pone.0018111
24. Jack, CR Jr, Bennett, DA, Blennow, K, Carrillo, MC, Dunn, B, Haeberlein, SB, et al. NIA-AA research framework: toward a biological definition of Alzheimer’s disease. Alzheimers Dement. (2018) 14:535–62. doi: 10.1016/j.jalz.2018.02.018
25. McDade, EM . Alzheimer disease. Continuum (Minneap Minn). (2022) 28:648–75. doi: 10.1212/CON.0000000000001131
26. Ivanidze, J, Nordvig, AS, Fajardo, AR, Tsiouris, AJ, Chiang, GC-Y, and Osborne, JR. Medicare coverage of amyloid PET: implications for clinical practice. AJNR Am J Neuroradiol. (2023) 44:E45. doi: 10.3174/ajnr.A8027
27. Verger, A, Yakushev, I, Albert, NL, van Berckel, B, Brendel, M, Cecchin, D, et al. FDA approval of lecanemab: the real start of widespread amyloid PET use? The EANM neuroimaging committee perspective. Eur J Nucl Med Mol Imaging. (2023) 50:1553–5. doi: 10.1007/s00259-023-06177-5
28. Pontecorvo, MJ, and Mintun, MA. PET amyloid imaging as a tool for early diagnosis and identifying patients at risk for progression to Alzheimer’s disease. Alzheimers Res Ther. (2011) 3:11. doi: 10.1186/alzrt70
29. Cummings, J, Apostolova, L, Rabinovici, GD, Atri, A, Aisen, P, Greenberg, S, et al. Lecanemab: appropriate use recommendations. J Prev Alzheimers Dis. (2023) 10:362–77. doi: 10.14283/jpad.2023.30
30. Beyer, L, and Brendel, M. Imaging of tau pathology in neurodegenerative diseases: an update. Semin Nucl Med. (2021) 51:253–63. doi: 10.1053/j.semnuclmed.2020.12.004
31. Mueller, A, Bullich, S, Barret, O, Madonia, J, Berndt, M, Papin, C, et al. Tau PET imaging with 18F-PI-2620 in patients with Alzheimer disease and healthy controls: a first-in-humans study. J Nucl Med. (2020) 61:911–9. doi: 10.2967/jnumed.119.236224
32. Suppiah, S, Didier, M-A, and Vinjamuri, S. The who, when, why, and how of PET amyloid imaging in Management of Alzheimer’s disease-review of literature and interesting images. Diagnostics (Basel). (2019) 9:1–18. doi: 10.3390/diagnostics9020065
33. Anand, K, and Sabbagh, M. Amyloid imaging: poised for integration into medical practice. Neurotherapeutics. (2017) 14:54–61. doi: 10.1007/s13311-016-0474-y
34. Banks, SA, Sechi, E, and Flanagan, EP. Autoimmune encephalopathies presenting as dementia of subacute onset and rapid progression. Ther Adv Neurol Disord. (2021) 14:1756286421998906. doi: 10.1177/1756286421998906
35. Wesley, SF, and Ferguson, D. Autoimmune Encephalitides and rapidly progressive dementias. Semin Neurol. (2019) 39:283–92. doi: 10.1055/s-0039-1678583
36. Hermann, P, and Zerr, I. Rapidly progressive dementias — aetiologies, diagnosis and management. Nat Rev Neurol. (2022) 18:363–76. doi: 10.1038/s41582-022-00659-0
37. Patil, A, Vishnu, V, Naheed, D, Pulikottil, V, Pandit, A, Goyal, M, et al. Rapidly progressive dementia: an eight year (2008-2016) retrospective study. J Neurol Sci. (2017) 381:117–8. doi: 10.1016/j.jns.2017.08.367
38. Sarikaya, I, Sarikaya, A, and Elgazzar, AH. Current status of 18F-FDG PET brain imaging in patients with dementia. J Nucl Med Technol. (2018) 46:362–7. doi: 10.2967/jnmt.118.210237
39. Kaiser, A, Davenport, MS, Frey, KA, Greenspan, B, and Brown, RKJ. Management of diabetes mellitus before 18F-fluorodeoxyglucose PET/CT: a nationwide patient-centered assessment of approaches to examination preparation. J Am Coll Radiol. (2019) 16:804–9. doi: 10.1016/j.jacr.2018.09.006
40. Zhang, X, Ogihara, T, Zhu, M, Gantumur, D, Li, Y, Mizoi, K, et al. Effect of metformin on 18F-fluorodeoxyglucose uptake and positron emission tomographic imaging. Br J Radiol. (2022) 95:20200810. doi: 10.1259/bjr.20200810
41. Steinberg, JD, Vogel, W, and Vegt, E. Factors influencing brown fat activation in FDG PET/CT: a retrospective analysis of 15, 000+ cases. Br J Radiol. (2017) 90:20170093. doi: 10.1259/bjr.20170093
42. Parysow, O, Mollerach, AM, Jager, V, Racioppi, S, San Roman, J, and Gerbaudo, VH. Low-dose oral propranolol could reduce brown adipose tissue F-18 FDG uptake in patients undergoing PET scans. Clin Nucl Med. (2007) 32:351–7. doi: 10.1097/01.rlu.0000259570.69163.04
43. Guedj, E, Varrone, A, Boellaard, R, Albert, NL, Barthel, H, van Berckel, B, et al. EANM procedure guidelines for brain PET imaging using [18F] FDG, version 3. Eur J Nucl Med Mol Imaging. (2022) 49:632–51. doi: 10.1007/s00259-021-05603-w
44. Robinson, DM, and Keating, GM. Memantine: a review of its use in Alzheimer’s disease. Drugs. (2006) 66:1515–34. doi: 10.2165/00003495-200666110-00015
45. Munafò, A, Burgaletto, C, Di Benedetto, G, Di Mauro, M, Di Mauro, R, Bernardini, R, et al. Repositioning of Immunomodulators: a ray of Hope for Alzheimer’s disease? Front Neurosci. (2020) 14:614643. doi: 10.3389/fnins.2020.614643
46. Berger, M, Gould, MK, and Barnett, PG. The cost of positron emission tomography in six United States veterans affairs hospitals and two academic medical centers. AJR Am J Roentgenol. (2003) 181:359–65. doi: 10.2214/ajr.181.2.1810359
47. McKeith, I, Del Ser, T, Spano, P, Emre, M, Wesnes, K, Anand, R, et al. Efficacy of rivastigmine in dementia with Lewy bodies: a randomised, double-blind, placebo-controlled international study. Lancet. (2000) 356:2031–6. doi: 10.1016/S0140-6736(00)03399-7
48. van Dyck, CH, Swanson, CJ, Aisen, P, Bateman, RJ, Chen, C, Gee, M, et al. Lecanemab in early Alzheimer’s disease. N Engl J Med. (2023) 388:9–21. doi: 10.1056/NEJMoa2212948
49. Sims, JR, Zimmer, JA, Evans, CD, Lu, M, Ardayfio, P, Sparks, J, et al. Donanemab in early symptomatic Alzheimer disease: the TRAILBLAZER-ALZ 2 randomized clinical trial. JAMA. (2023) 330:512–27. doi: 10.1001/jama.2023.13239
50. Fällmar, D, Lilja, J, Danfors, T, Kilander, L, Iyer, V, Lubberink, M, et al. Z-score maps from low-dose 18F-FDG PET of the brain in neurodegenerative dementia. Am J Nucl Med Mol Imaging. (2018) 8:239–46. Available at: https://www.ncbi.nlm.nih.gov/pmc/articles/PMC6146163/.
51. Wingo, TS, Liu, Y, Gerasimov, ES, Vattathil, SM, Wynne, ME, Liu, J, et al. Shared mechanisms across the major psychiatric and neurodegenerative diseases. Nat Commun. (2022) 13:4314. doi: 10.1038/s41467-022-31873-5
52. Klunk, WE, Koeppe, RA, Price, JC, Benzinger, TL, Devous, MD Sr, Jagust, WJ, et al. The Centiloid project: standardizing quantitative amyloid plaque estimation by PET. Alzheimers Dement. (2015) 11:1-15.e1-4. doi: 10.1016/j.jalz.2014.07.003
53. Bourgeat, P, Doré, V, Doecke, J, Ames, D, Masters, CL, Rowe, CC, et al. Non-negative matrix factorisation improves Centiloid robustness in longitudinal studies. NeuroImage. (2021) 226:117593. doi: 10.1016/j.neuroimage.2020.117593
54. Pozzi, FE, Licciardo, D, Musarra, M, Jonghi-Lavarini, L, Crivellaro, C, Basso, G, et al. Depressive Pseudodementia with reversible AD-like brain Hypometabolism: a case report and a review of the literature. J Pers Med. (2022) 12:1–12. doi: 10.3390/jpm12101665
55. Caffarra, P, Gardini, S, Cappa, S, Dieci, F, Concari, L, Barocco, F, et al. Degenerative jargon aphasia: unusual progression of logopenic/phonological progressive aphasia? Behav Neurol. (2013) 26:89–93. doi: 10.1155/2013/965782
56. Sipos, D, László, Z, Tóth, Z, Kovács, P, Tollár, J, Gulybán, A, et al. Additional value of 18F-FDOPA amino acid analog radiotracer to irradiation planning process of patients with glioblastoma Multiforme. Front Oncol. (2021) 11:699360. doi: 10.3389/fonc.2021.699360
57. Aboian, M, Barajas, R, Shatalov, J, Ravanfar, V, Bahroos, E, Tong, E, et al. Maximizing the use of batch production of 18F-FDOPA for imaging of brain tumors to increase availability of hybrid PET/MR imaging in clinical setting. Neurooncol Pract. (2021) 8:91–7. doi: 10.1093/nop/npaa065
58. Lizarraga, KJ, Allen-Auerbach, M, Czernin, J, DeSalles, AAF, Yong, WH, Phelps, ME, et al. 18F-FDOPA PET for differentiating recurrent or progressive brain metastatic tumors from late or delayed radiation injury after radiation treatment. J Nucl Med. (2014) 55:30–6. doi: 10.2967/jnumed.113.121418
59. Tournier, BB, Tsartsalis, S, Ceyzériat, K, Garibotto, V, and Millet, P. In vivo TSPO signal and Neuroinflammation in Alzheimer’s disease. Cells. (2020) 9:1–30. doi: 10.3390/cells9091941
60. Garland, EF, Dennett, O, Lau, LC, Chatelet, DS, Bottlaender, M, Nicoll, JAR, et al. The mitochondrial protein TSPO in Alzheimer’s disease: relation to the severity of AD pathology and the neuroinflammatory environment. J Neuroinflammation. (2023) 20:186. doi: 10.1186/s12974-023-02869-9
61. Alzghool, OM, van Dongen, G, van de Giessen, E, Schoonmade, L, and Beaino, W. α-Synuclein radiotracer development and in vivo imaging: recent advancements and new perspectives. Mov Disord. (2022) 37:936–48. doi: 10.1002/mds.28984
62. Michels, S, Buchholz, H-G, Rosar, F, Heinrich, I, Hoffmann, MA, Schweiger, S, et al. 18F-FDG PET/CT: an unexpected case of Huntington’s disease. BMC Neurol. (2019) 19:78. doi: 10.1186/s12883-019-1311-9
63. Obergassel, J, Lohmann, L, Meuth, SG, Wiendl, H, Grauer, O, and Nelke, C. An enigmatic case of cortical anopsia: Antemortem diagnosis of a 14-3-3 negative Heidenhain-variant MM1-sCJD. Prion. (2020) 14:24–8. doi: 10.1080/19336896.2019.1706703
Keywords: Alzheimer’s disease, lewy body dementia, FDG-PET, frontotemporal dementia, systematic review
Citation: Huang B, Sawicki S, Habiger C, Mattis PJ, Gordon ML, Franceschi AM and Giliberto L (2024) Memories and mimics: unveiling the potential of FDG-PET in guiding therapeutic approaches for neurodegenerative cognitive disorders. Front. Neurol. 15:1428036. doi: 10.3389/fneur.2024.1428036
Edited by:
Ahmed Negida, Virginia Commonwealth University, United StatesReviewed by:
Ayon Nandi, Johns Hopkins University, United StatesMatej Perovnik, University Medical Centre Ljubljana, Slovenia
Anat Biegon, Stony Brook Medicine, United States
Copyright © 2024 Huang, Sawicki, Habiger, Mattis, Gordon, Franceschi and Giliberto. This is an open-access article distributed under the terms of the Creative Commons Attribution License (CC BY). The use, distribution or reproduction in other forums is permitted, provided the original author(s) and the copyright owner(s) are credited and that the original publication in this journal is cited, in accordance with accepted academic practice. No use, distribution or reproduction is permitted which does not comply with these terms.
*Correspondence: Brendan Huang, Ymh1YW5nMUBub3J0aHdlbGwuZWR1