- 1Graduate School of Hunan University of Chinese Medicine, Changsha, China
- 2National TCM Master Liu Zuyi Inheritance Studio, The Affiliated Hospital of Hunan Academy of Chinese Medicine, Changsha, China
- 3The First Clinical College of Nanjing University of Chinese Medicine, Nanjing, China
Background: Parkinson’s disease (PD) is a chronic, progressive neurodegenerative disorder primarily affecting the elderly. Relevant studies suggest a significant connection between the mitochondria and PD. Publications exploring this connection have steadily increased in recent years. This study employs a bibliometric approach to comprehensively analyze the current status and future directions of the research on mitochondria and PD.
Method: We retrieved data from the Web of Science database and used CiteSpace, VOSviewer, and “Bibliometrix” software to visually analyze various aspects of the research field. These aspects included the number of published papers, contributing countries and institutions, authors, publishing journals, cited references, and keywords.
Results: Our analysis identified a total of 3,291 publications involving 14,670 authors from 2,836 organizations across 78 countries. The publication volume exhibited a continuous upward trend from 1999 to 2023. The United States emerged as the leading force in this research area, contributing the highest number of high-quality publications. Notably, the United States collaborated extensively with Germany and the United Kingdom. The University of Pittsburgh stood out as the most prolific institution. Harvard University had the highest academic influence and closely cooperated with the University of Pittsburgh, Juntendo University, and McGill University. Dr. Hattori Nobutaka was identified as the most prolific author, while Dr. Youle, Richard J emerged as the most influential author based on the highest average citation frequency. The Journal of Neurochemistry was the most published journal. The most co-cited paper was titled “Hereditary early-onset Parkinson’s disease caused by mutations in PINK1.” The major keywords included oxidative stress, alpha-synuclein, pink1, mitophagy, and mitochondrial dysfunction. Mitofusin 2, ubiquitin, and mitochondrial quality control have been identified as new research hotspots in recent years.
Conclusion: Mitochondria-PD research is experiencing a steady increase in activity, fueled by increasing close collaboration between countries and different institutions. However, there is a need to further strengthen collaboration and communication between developed and developing nations. Current research has focused on the specific mechanisms of mitochondrial dysfunction and their relationship with PD. Mitofusin 2, ubiquitin, and mitochondrial quality control are positioned to be the hotspots and future research directions.
1 Introduction
Parkinson’s disease (PD) is a chronic, progressive neurodegenerative disorder characterized by the progressive degeneration of dopaminergic neurons in the brain (1). This degeneration manifests as motor symptoms, such as bradykinesia, rigidity, resting tremor, and postural instability (2). These symptoms progressively worsen, interfering with daily activities and quality of life. PD is a complex condition with multiple contributing factors, including environmental exposures, genetic predisposition, and aging. Despite extensive research, the precise mechanisms underlying the pathogenesis of PD remain unclear, prompting ongoing exploration of new therapeutic targets and strategies.
Mitochondria, as energy-producing organelles, provide essential energy for neuronal activity (3). They are involved in a range of cellular processes, including oxidative phosphorylation (4), calcium signaling (5), and apoptosis (6). In neurons, mitochondria are particularly important as they fuel synaptic transmission and neuronal survival. Mitochondrial dysfunction has been implicated in numerous neurological diseases, with PD being a prime example (7). The relationship between mitochondria and PD has attracted significant attention in recent years. Mitochondrial dysfunction can result in dopaminergic neuronal degeneration (8), a key factor in PD pathogenesis. Additionally, PD patients exhibit abnormalities in mitochondrial morphology, function, and dynamics (9), further strengthening the link between mitochondrial dysfunction and PD. Targeting mitochondria for novel treatments holds promise as a potential effective therapeutic approach for PD. With the development of aging societies, research on the link between mitochondria and PD has gradually become a major focus. While numerous studies have been published, a comprehensive analysis of the current research status and development frontiers in the field of mitochondria and PD is lacking.
Bibliometric analysis, a systematic statistical analysis method (10), offers a powerful tool for effectively exploring knowledge structures and identifying research hotspots and future directions. This is made possible through the visualization of data and network collaboration maps (11). In this study, we utilized bibliometric analysis to systematically examine the relationship between mitochondria and PD. We aimed to identify influential authors, key research themes, and emerging trends within this rapidly evolving field. By doing so, we aimed to contribute to a more profound understanding of the role of mitochondria in the pathogenesis of PD.
2 Methods
2.1 Search strategy and data sources
We used the Web of Science (WoS) database, a comprehensive and authoritative literature retrieving tool encompassing literature from various scientific disciplines, for data retrieval. WoS is considered to be the most suitable database for bibliometric analysis (12). Data were collected from the WoS core collection. Specific filters were applied using the WoS topic search function (Topics, TS). The search formula was ((((TS = (mitochondria)) OR TS = (mitochondrion)) OR TS = (chondriosome)) OR TS = (plastosome)) AND TS = (Parkinson’s disease). To ensure that the most up-to-date data was included, the search timeframe was set before January 1, 2024. Additionally, the search was restricted to articles and reviews published in English. Meeting abstracts, proceedings papers, editorial material, and other types of publications were excluded. It was speculated that although the initial topic search may contain ostensibly related literature, it can also include marginally related or irrelevant literature. To address this, two members of the research team checked and analyzed the literature, manually screening to exclude irrelevant literature, resulting in a final dataset of 3,291 publications for analysis. The literature screening process is illustrated in Figure 1.
2.2 Data analysis
CiteSpace, VOSviewer, and “Bibliometrix” software were used for the bibliometric analysis. These tools hold much significance in analyzing literature visualization. They are also recognized and used by most scholars. CiteSpace is a practical literature software for quantitative analysis, which can present the structure and distribution of scientific knowledge (13). VOSviewer can visually analyze the knowledge in the research field and provides multiple types of view interpretation (14). Bibliometrix is an R package that collates relevant literature in the field and visualizes the results (15).
3 Results
3.1 Scientific publications related to PD and mitochondria
A total of 3,291 publications were involved in this study, including 2,363 articles (71.8%) and 928 reviews (28.2%). These publications were written by 14,670 authors from 2,836 organizations in 78 countries. They were published in 625 journals and cited 121,199 references from 7,996 journals.
3.2 Temporal trend of publication outputs
The number of publications reflected the research trends. In the investigated time frame, publications on mitochondria and PD increased significantly compared with publications on other PD-related research topics (Figure 2A). From 1999 to 2023, a total of 3,921 publications were published on mitochondria and PD, with the number of publications showing an upward trend (Figure 2B). The time trend change can be divided into two periods. In the first period, from 1999 to 2007, the number of publications increased slowly, with fewer than 100 publications each year. In the second period, from 2008 to 2023, the number of publications increased rapidly, with a peak of 264 publications in 2020.
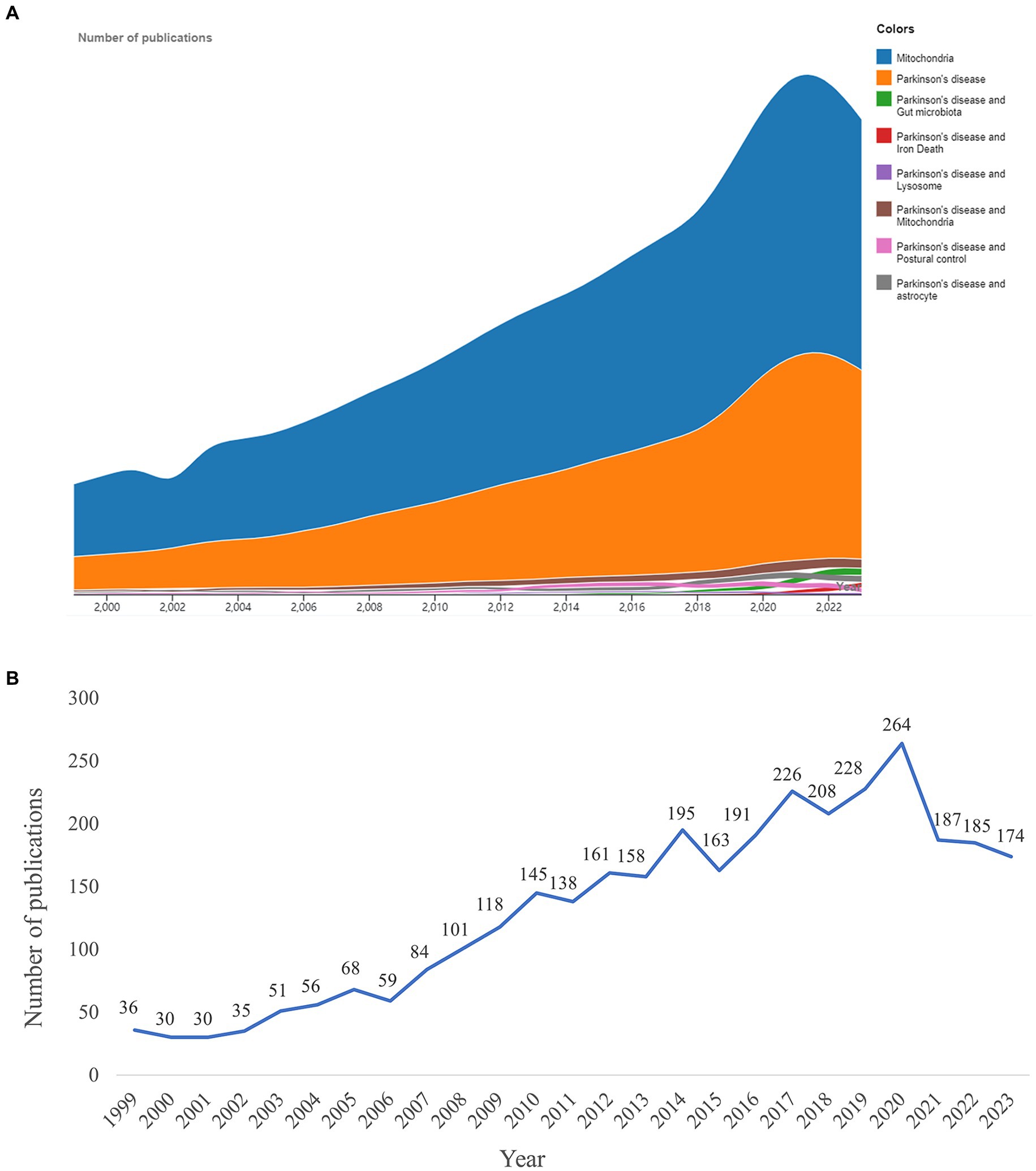
Figure 2. Research results. (A) Number of publications on mitochondria and Parkinson’s disease (PD) was compared with other topics. (B) Trend in the number of publications on mitochondria and PD from 1999 to 2023.
3.3 Geographic distribution and national collaboration
The heat map of the geographical distribution of publications revealed a concentration of research activity in North America, Asia, and Europe, with notable collaboration among these regions (Figure 3A). Researchers from 78 countries have contributed to the field of mitochondria and PD research. Among these countries, the United States ranked first (1,160 publications), followed by China (545 publications) and the United Kingdom (346 publications) (Figure 3B). The United States emerged as the leading force in this research area, contributing the highest number of high-quality publications and demonstrating strong collaborative ties with Germany and the United Kingdom (Figure 3C).
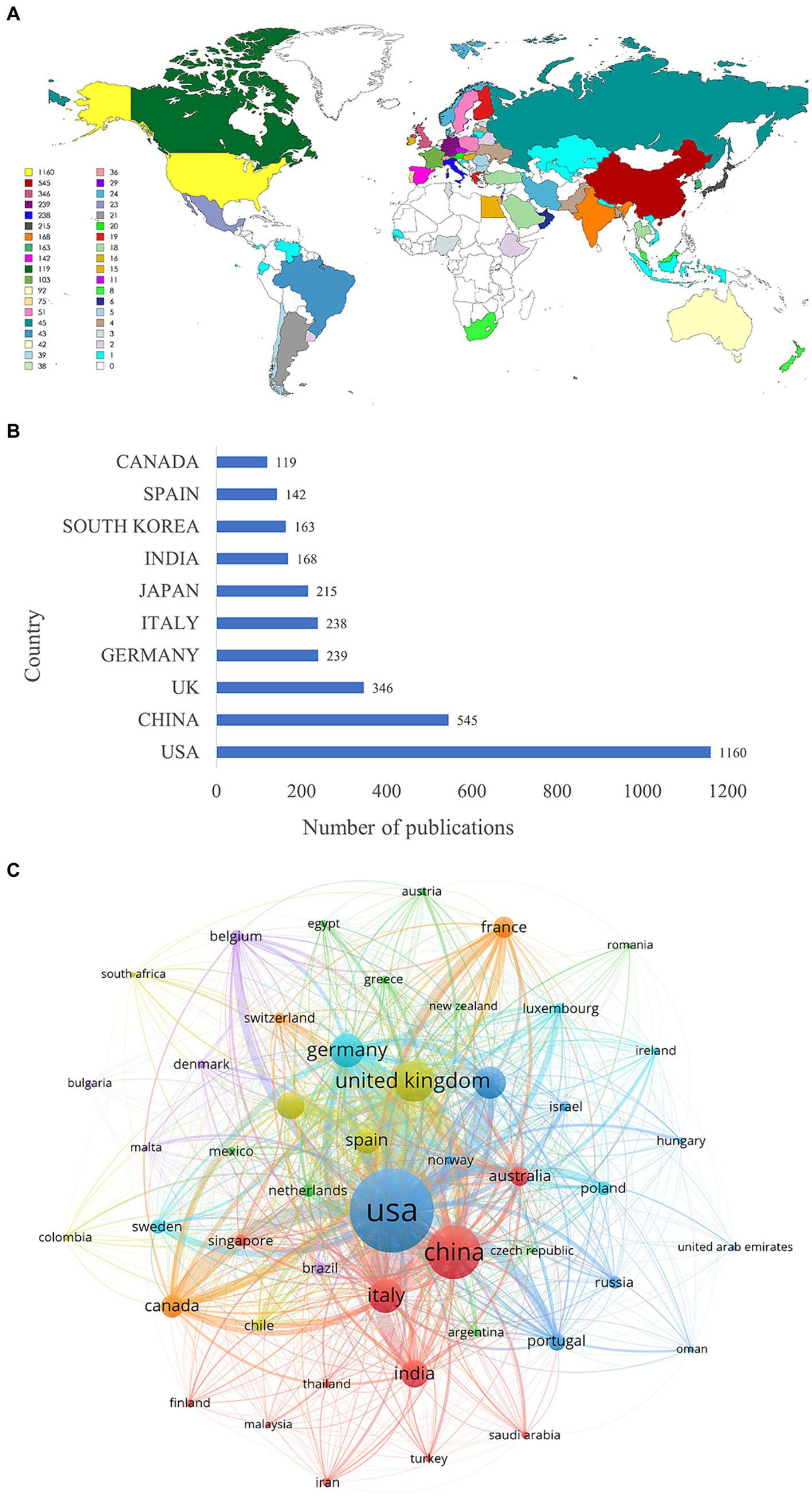
Figure 3. Analysis of countries. (A) Geographic distribution of countries. (B) Top 10 countries in terms of the number of publications. (C) The map of collaboration network between countries. The thicker the line, the closer the collaboration.
3.4 Research organizations
In terms of the number of publications, four of the top 10 organizations were located in the United States. The University of Pittsburgh topped the list with 76 publications, followed by University College London (69 publications), Harvard University (52 publications), and Johns Hopkins University (52 publications) (Table 1). Harvard University had the highest academic influence and maintained close collaboration with the University of Pittsburgh, Juntendo University, and McGill University (Table 1; Figure 4A). In organizational research outbreaks, the University of Cambridge, the University of Luxembourg, and the University of Lubeck have been identified as emerging and active organizations in recent years (Figure 4B).
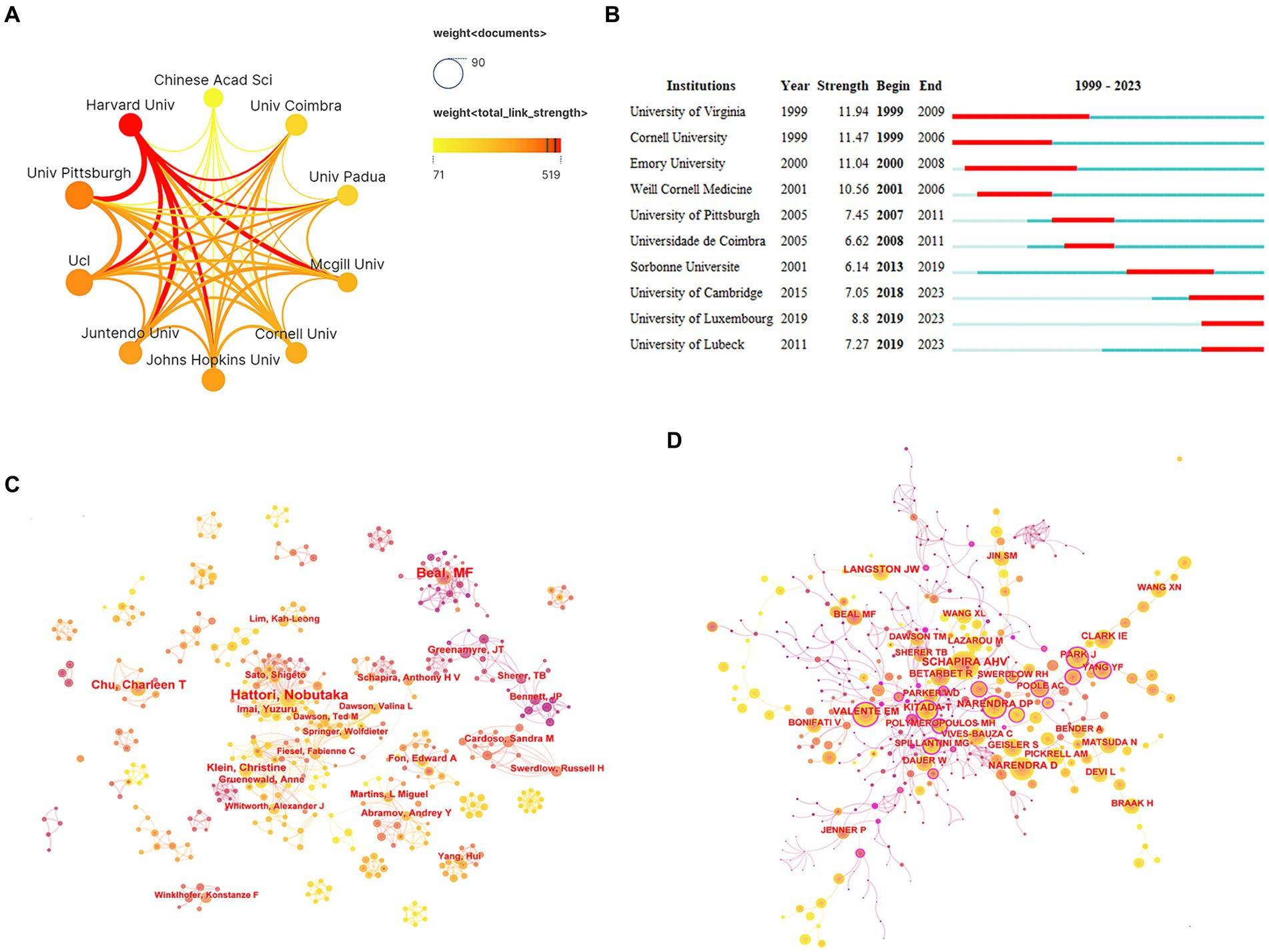
Figure 4. Analysis of organizations and authors. (A) Network collaboration map of top 10 organizations. (B) Top 10 organizations with the strongest citation bursts. (C) Author collaboration network diagram. (D) Co-cited author collaboration network diagram.
3.5 Authors and co-cited authors
An analysis of the authors associated with publications can identify prominent scholars and key forces within the research field. Dr. Hattori Nobutaka emerged as the most prolific author with 42 papers, followed by Dr. Beal, M. Flint (40 papers) and Dr. Chu, Charleen T (28 papers). These highly productive authors all benefited from stable collaboration networks and research teams (Figure 4C). Dr. Youle, Richard J stood out as the most influential author based on the highest average citation frequency (879.67 citations/literature) (Table 2). Notably, two teams—one comprised of Dr. Cardoso, Sandra M and Dr. Swerdlow, Russell H, and the other comprised of Dr. Greenamyre, JT, and Dr. Sherer, TB—demonstrated close collaboration and have published high-quality articles.
Co-cited authors refer to instances where two or more authors are cited together in the same publications. Among the top 10 most-cited authors, seven authors were cited over 500 times. Dr. Schapira, Ahv was the most cited author (1,401 times), followed by Dr. Narendra, Derek P (1,109 times) and Dr. Beal, M. Flint (610 times) (Table 3; Figure 4D).
3.6 Journals and co-cited journals
A Bradford’s law analysis (16) identified 23 core journals in the field of mitochondria and PD research (Figure 5A). These core journals were primarily concentrated in neurology and biology fields (Figure 5B), with 1,107 publications, accounting for 33.64% of total publications. The top three core journals with the most publications were the Journal of Neurochemistry (102 publications, 3.1%), International Journal of Molecular Sciences (99 publications, 3.01%), and Neurobiology of Disease (60 publications, 1.8%) (Table 4). It is noteworthy that the Journal of Neurochemistry has the highest quality of literature with an average citation frequency of 106.22.
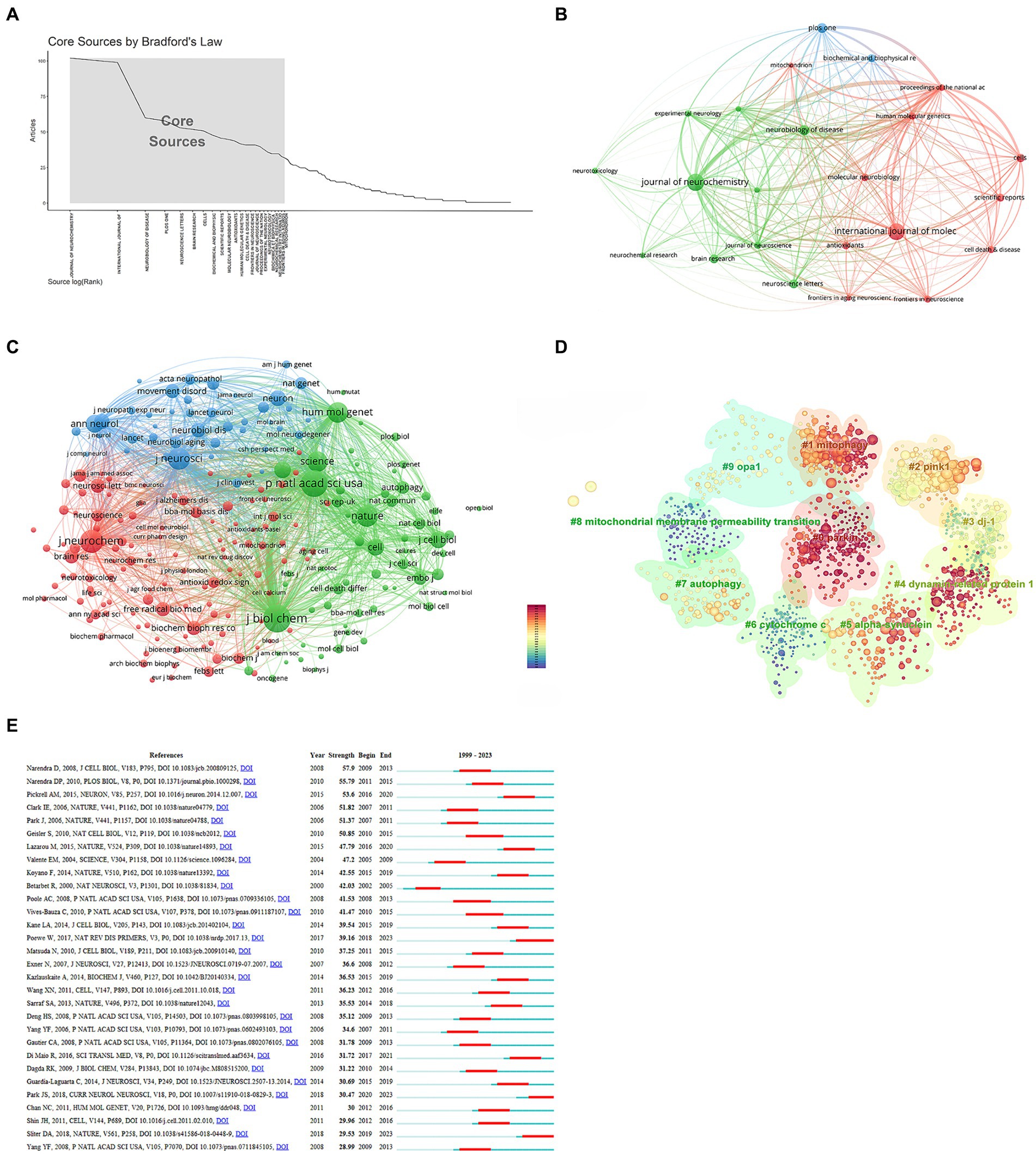
Figure 5. Analysis of journals and co-cited references. (A) Core journals. (B) Interconnections of core journals. (C) Network map of co-cited journals. (D) Cluster analysis of co-cited literature. (E) Top 30 references with the strongest citation bursts.
A co-cited journal network analysis is presented in Figure 5C. The effect of a journal is closely related to the number of citations. The top three co-cited journals were the Journal of Biological Chemistry (12,086 citations), Proceedings of the National Academy of Sciences of the United States of America (10,868 citations), and the Journal of Neurochemistry (8,054 citations) (Table 5).
3.7 Co-cited references and reference bursts
Co-cited references formed the basis of the research field. The top 10 cited references are listed in Table 6. The most co-cited paper (503 citations) was “Hereditary early-onset Parkinson’s disease caused by mutations in PINK1,” published in the journal Science with an impact factor of 56.9. This was followed by “Parkin is recruited selectively to impaired mitochondria and promotes their autophagy” (455 citations) and “Mutations in the parkin gene cause autosomal recessive juvenile parkinsonism” (433 citations). A cluster analysis of co-cited literature was performed using CiteSpace. The color of nodes in the cluster from dark blue to yellow and red reveals the shift in research focus over time (Figure 5D). While “mitochondrial membrane permeability transition” and “cytochrome” received attention in the past, recent research has shifted toward “parkin,” “dynamin-related protein 1,” and “mitophagy”.
Reference bursts reflect the number of citations within a specific timeframe. Among the strongest reference bursts, the most highly cited articles in recent years were “Parkinson’s disease,” “Parkin and PINK1 mitigate STING-induced inflammation,” and “Mitochondrial dysfunction in Parkinson’s disease: New mechanistic insights and therapeutic perspectives” (Figure 5E).
3.8 Keywords
Keyword analysis helps identify research hotspots and key areas within a research field. In the field of mitochondria and PD, frequently occurring keywords included oxidative stress (1,188 times), alpha-synuclein (677 times), neurodegeneration (520 times), apoptosis (436 times), pink1 (403 times), mitophagy (390 times), autophagy (352 times), and mitochondrial dysfunction (361 times) (Figure 6A). Keyword cluster analysis revealed that “pink1,” “apoptosis,” and “Parkinson’s disease” clusters were prominent research topics attracting significant scholarly attention in recent years (Figure 6B).
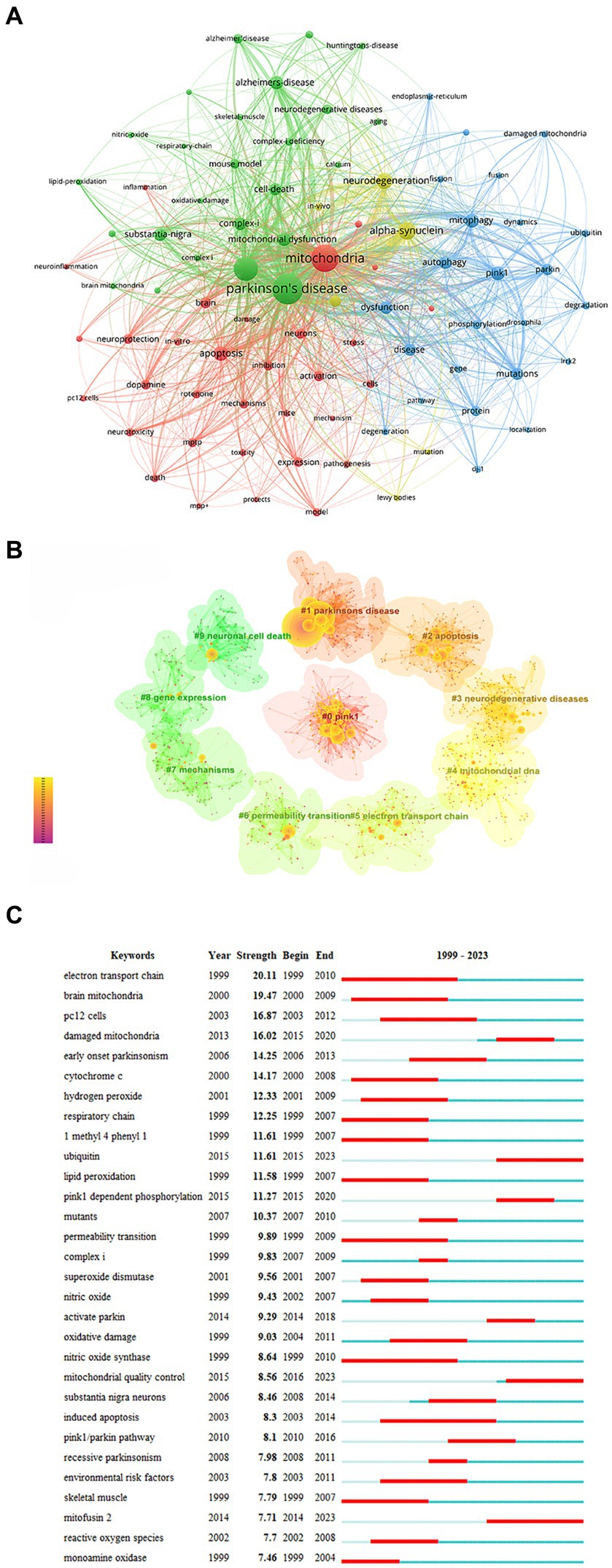
Figure 6. Analysis of keywords. (A) Network map of keywords. (B) Cluster analysis of keywords. (C) Top 30 keywords with the strongest citation.
Keywords with the strongest bursts identify phrases that appear frequently within a short period, reflecting shifts in research focus over time. The top three bursts were “electron transport chain” (strength = 20.11), “brain mitochondria” (strength = 19.47), and “damaged mitochondria” (strength = 16.87) (Figure 6C). “Electron transport chain,” “nitric oxide synthase,” and “induced apoptosis” have been the longest-lasting hot spots in the last two decades. The keywords that broke out in the past 3 years primarily focus on “mitofusin 2,” “ubiquitin,” and “mitochondrial quality control.”
4 Discussion
In recent years, the relationship between mitochondria and PD has gradually attracted considerable attention from the scientific community. Furthermore, publications on mitochondria and PD have grown significantly compared with publications in other PD-related research areas within the investigated time frame. Therefore, it is necessary to comprehensively analyze the progress and hotspot changes in this research field to provide an intuitive understanding to researchers. To analyze publications related to mitochondria and PD, we used bibliometric analysis, aiming to further explore the development of this research field and predict emerging trends.
The number of publications can reflect the development of the current research field. From 1999 to 2023, publications on mitochondria and PD steadily increased. This upward trend suggests a potential link between the development of this research field and the acceleration of the aging population. As societies age, exploration of the mechanisms of geriatric diseases such as PD has attracted increasing attention. A geographical analysis revealed a concentration of research activity in North America, Asia, and Europe, with notable collaborations among these regions. The top three countries in terms of the number of publications were the United States, China, and the United Kingdom. The United States was the main force in research with the highest quality of publications. Four of the top 10 organizations were from the United States. Harvard University had the highest academic influence and closely collaborated with the University of Pittsburgh, Juntendo University, and McGill University. The main research forces were concentrated in developed countries, so it is particularly important to strengthen academic communication and collaboration between developed and developing countries to promote the development of this research field.
In terms of authors, Dr. Hattori Nobutaka was the most prolific author (42 papers), followed by Dr. Beal, M. Flint (40 papers), and Dr. Chu, Charleen T (28 papers). Dr. Hattori Nobutaka comes from Juntendo University. His primary research area is Neurosciences & Neurology. In 2010, Dr. Hattori Nobutaka and his research team discovered that PINK1 recruited Parkin to damaged mitochondria for mitochondrial degradation in a membrane potential-dependent manner (17). Afterward, in 2020, they found that loss of Parkin led to mitochondrial accumulation and that the impairment of mitochondrial clearance may be the cause of dopaminergic neuron loss (18). In the same year, they discovered that mitochondrial UQCRC1 mutations contributed to familial parkinsonism (19). In recent years, their main research has focused on the gene PRKN (20) and proteins related to mitochondrial function, such as FBXO7 (21) and CHCHD2 (22). It is noteworthy that Dr. Youle, Richard J is the most influential author with the highest average citation frequency of literature (879.67 citations/literature). Dr. Youle, Richard J comes from NIH’s National Institute of Neurological Disorders and Stroke. His main research areas are cell biology, neuroscience, and neurology. In 2011, Dr. Youle, Richard J and his research team elaborated on the mechanism of mitophagy and its link with PD, which attracted great attention in the academic community (23). In 2012, they explored mitochondrial fission, fusion, and stress, while also further describing the association of mitochondrial fission, fusion, and stress with PD (24). Subsequently, in 2018, they found that PINK1 and Parkin mitigated inflammation in PD by mediating mitophagy (25). With regards to group collaboration, the team of Dr. Cardoso, Sandra M and Dr. Swerdlow, Russell H explored the effect of mitochondrial dysfunction on α-synuclein (26, 27). Dr. Greenamyre, JT and Dr. Sherer, TB focused on rotenone models of PD concerning mitochondrial dysfunction (28–30). Their findings not only provided important insights into the role of mitochondria in the pathogenesis of PD but also provided new ideas for treatment and drug development. These research efforts have significantly influenced the research direction of mitochondria and PD, which has promoted the progress and development of the entire research field.
Concerning journals, the Journal of Neurochemistry was the most widely published (102 articles, 3.1%), with the highest average number of citations (106.22), followed by the International Journal of Molecular Sciences (99 articles, 3.01%) and Biology of Neurological Diseases (60 articles, 1.8%). The top three co-cited journals were the Journal of Biological Chemistry (12,086 citations), followed by Proceedings of the National Academy of Sciences of the United States of America (10,868 citations) and the Journal of Neurochemistry (8,054 citations). Among the top 10 cited journals, seven were located in JCR Q1 with high quality. Cited journals comprised the basis of citing journals, which provided references to support the current research.
Co-cited references formed the basis of the research field. The literature “Hereditary early-onset Parkinson’s disease caused by mutations in PINK1” was the most co-cited paper (503 citations), followed by “Parkin is recruited selectively to impaired mitochondria and promotes their autophagy” (455 citations) and “Mutations in the parkin gene cause autosomal recessive juvenile parkinsonism” (433 citations). These top-cited articles focusing on mutations in PINK1 and Parkin genes and their interactions represent the foundational research on mitochondria in PD.
Keywords represent the core research content of the literature. Keyword analysis can quickly reflect the hotspot distribution and focus in the research field. Beyond PD and mitochondria, frequently occurring keywords included oxidative stress, alpha-synuclein, neurodegeneration, PINK1, mitophagy, and mitochondrial dysfunction. Oxidative stress is a key player in PD pathogenesis. It elevates reactive oxygen species (ROS) within cells, which can directly damage mitochondria and contribute to the degeneration of dopaminergic neurons (31). Alpha-synuclein, the major component of Lewy bodies, a hallmark of PD pathology, accumulates abnormally (32). This aggregation can exacerbate mitochondrial oxidative stress (33) and increase ROS production (31), which leads to neurodegeneration. Mutations in the PINK1 gene are a frequent cause of autosomal recessive PD (34). Localized to the mitochondria, PINK1 regulates the balance between mitochondrial fission and fusion (35). Additionally, PINK1 acts upstream of Parkin, recruiting Parkin to the mitochondria to initiate autophagy in damaged mitochondria (36). Senescent and damaged mitochondria are eliminated by mitophagy, and the PINK1/Parkin pathway plays a crucial role in this process (37). Mitochondrial dysfunction manifests primarily as abnormalities in mitochondrial morphological structure and function, including mitochondrial number abnormalities, mitochondrial DNA damage, and mitochondrial-related protein abnormalities. Research has revealed a close association between mitochondrial DNA variations, complex I deficiency, and PD (38). Moreover, a related study has revealed that genetic abnormalities In Parkin, PINK1, DJ-1, and LRRK2 could lead to mitochondrial dysfunction and were associated with monogenic PD (39). These keywords reflected that the focus of research was concentrated on the specific mechanisms of mitochondrial dysfunction and their relationship with PD. The study of genes, proteins, and pathways related to mitochondrial function remains the mainstream direction of the present research.
The core themes and domain structure can be quickly identified from the massive literature through the keyword cluster analysis of the literature, and the research and development of this field can be further understood. In recent years, “pink1,” “apoptosis,” and “Parkinson's disease” clusters have been important research topics in this field with regard to cluster analysis. Dr. Klein, Christine, Dr. Valente, Enza Maria, and Dr. Muqit, Miratul M K contributed to the “pink1” research topic. Dr. Maruyama, Wakako and Dr. Naoi, Makoto made many contributions to the topic of “apoptosis.”
Animal models of PD mainly included the mouse model, rat model, Caenorhabditis elegans model, drosophila model, zebrafish model, and monkey model. Rotenone, 6-OHDA, and MPTP were the commonly used drugs in the process of construction of animal models of PD (40–42). The SH-SY5Y neuroblastoma cells and primary neuronal cultures are widely used for the cell model (43). Mitochondria-targeted biomimetic nanoparticles can promote neuronal mitochondrial biogenesis by regulating the NAD/SIRT1/PGC-1α/PPARγ/NRF1/TFAM pathway, thereby ameliorating mitochondrial dysfunction and alleviating PD symptoms (44). Clinical studies are also exploring gene therapy, drug therapy, and other methods to improve mitochondrial function to further treat PD. Gene therapy has been used to treat mitochondrial dysfunction in PD, mainly including enhancing dopamine synthesis (45), improving neurotrophic factors expression (46), and in the regulation of the human basal ganglia circuits (47). Coenzyme Q10, with its antioxidant properties, has demonstrated potential in reducing oxidative stress by protecting the mitochondrial membrane (48). Studies have suggested that PD patients with enrichment of mitochondrial gene risk variants may benefit from mitochondrial enhancer of coenzyme Q10 treatment (49). Oral administration of nicotinamide riboside (NR) can increase the level of nicotinamide adenine dinucleotide (NAD), thereby mitigating mitochondrial dysfunction and alleviating PD symptoms (50). Clinical trials have demonstrated great tolerability for NR treatment, with evidence of elevated levels of NAD in the brains of patients (51). The development of drugs that specifically target mitochondria holds promise as a potential treatment strategy for PD and warrants further investigations in larger clinical trials.
In recent years, popular research topics have mainly included mitofusin 2, ubiquitin, and mitochondrial quality control. These research hotspots may serve both as the latest research trends and as future research directions. Mitofusin 2 is a mitochondrial outer membrane protein, which is involved in the mitochondrial dynamic process (52). Loss of mitofusin 2 can lead to damage of the mitochondrial respiratory chain (53). It has also been demonstrated that mitofusin 2 had an imperative role in axonal mitochondrial transport (54) and axonal projections of dopaminergic neurons (55). Ubiquitination targets mitochondrial proteins for degradation through mitophagy (56). Parkin, for instance, can ubiquitinate certain candidate substrate proteins, tagging them for proteasomal degradation (57). Mitochondrial fission, fusion, mitophagy, and mitochondrial biogenesis are involved in mitochondrial quality control, and they coordinate with each other to regulate the quantity and quality of mitochondria (58). Defective mitochondrial quality control has been implicated in the development of PD (59). The identification of these emerging research hotspots has helped to reveal the research dynamics of mitochondria and PD, paving the way for future advancements in our understanding and treatment of the disease.
This study has some limitations. First, the analysis was limited to review articles and original research publications in English. This approach may have excluded relevant research published in other languages or formats. Second, with the continuous update of the WoS database, the included publications may differ slightly from the actual number of publications.
5 Conclusion
The bibliometric analysis of mitochondria and PD provides valuable insight into the current research status and development trend. Research related to mitochondria and PD is expanding considerably. There is growing collaboration among different countries, institutions, and authors. However, the collaboration and exchange between developed and developing countries needs to be further strengthened. Current research has focused on the specific mechanisms of mitochondrial dysfunction and their relationship with PD. Emerging research hotspots will likely include mitofusin 2, ubiquitin, and mitochondrial quality control, which are likely to shape future research directions in this field.
Data availability statement
The original contributions presented in the study are included in the article/supplementary material, further inquiries can be directed to the corresponding authors.
Author contributions
Y-JC: Software, Visualization, Writing – original draft. M-RX: Software, Writing – original draft. S-QZ: Writing – review & editing. FL: Software, Visualization, Writing – review & editing.
Funding
The author(s) declare financial support was received for the research, authorship, and/or publication of this article. This work was supported by the Academic Inheritance and Communication Project of China Academy of Chinese Medical Sciences (Grant No. CI2022E014XB), the Natural Science Foundation of Hunan Province (Grant No. 2023JJ60458, 2023JJ30367), the Clinical Medical Technology Innovation Guidance Project of Hunan Province (Grant No. 2021SK51010), the Scientific Research Project of Hunan Provincial Health Commission (Grant No. D202319019427), the Scientific Research Project of Hunan Provincial Administration of Traditional Chinese Medicine (Grant No. C2022011), and the Natural Science Foundation Project of Changsha City (Grant No. kq2208144).
Acknowledgments
We are grateful to all researchers who have published articles related to Parkinson’s disease and mitochondria research.
Conflict of interest
The authors declare that the research was conducted in the absence of any commercial or financial relationships that could be construed as a potential conflict of interest.
Publisher’s note
All claims expressed in this article are solely those of the authors and do not necessarily represent those of their affiliated organizations, or those of the publisher, the editors and the reviewers. Any product that may be evaluated in this article, or claim that may be made by its manufacturer, is not guaranteed or endorsed by the publisher.
Abbreviations
PD, Parkinson’s disease; WoS, Web of Science; ROS, Reactive Oxygen Species; NR, Nicotinamide Riboside; NAD, Nicotinamide Adenine Dinucleotide.
References
1. Lang, AE, and Lozano, AM. Parkinson's disease. First of two parts. N Engl J Med. (1998) 339:1044–53. doi: 10.1056/nejm199810083391506
2. Jankovic, J . Parkinson's disease: clinical features and diagnosis. J Neurol Neurosurg Psychiatry. (2008) 79:368–76. doi: 10.1136/jnnp.2007.131045
3. Nunnari, J, and Suomalainen, A. Mitochondria: in sickness and in health. Cell. (2012) 148:1145–59. doi: 10.1016/j.cell.2012.02.035
4. Acin-Perez, R, Salazar, E, Kamenetsky, M, Buck, J, Levin, LR, and Manfredi, G. Cyclic AMP produced inside mitochondria regulates oxidative phosphorylation. Cell Metab. (2009) 9:265–76. doi: 10.1016/j.cmet.2009.01.012
5. Rizzuto, R, De Stefani, D, Raffaello, A, and Mammucari, C. Mitochondria as sensors and regulators of calcium signalling. Nat Rev Mol Cell Biol. (2012) 13:566–78. doi: 10.1038/nrm3412
6. Desagher, S, and Martinou, JC. Mitochondria as the central control point of apoptosis. Trends Cell Biol. (2000) 10:369–77. doi: 10.1016/s0962-8924(00)01803-1
7. Beal, MF . Mitochondria take center stage in aging and neurodegeneration. Ann Neurol. (2005) 58:495–505. doi: 10.1002/ana.20624
8. Trinh, D, Israwi, AR, Arathoon, LR, Gleave, JA, and Nash, JE. The multi-faceted role of mitochondria in the pathology of Parkinson's disease. J Neurochem. (2021) 156:715–52. doi: 10.1111/jnc.15154
9. Schapira, AH . Mitochondria in the aetiology and pathogenesis of Parkinson's disease. Lancet Neurol. (2008) 7:97–109. doi: 10.1016/s1474-4422(07)70327-7
10. Ninkov, A, Frank, JR, and Maggio, LA. Bibliometrics: methods for studying academic publishing. Perspect Med Educ. (2022) 11:173–6. doi: 10.1007/s40037-021-00695-4
11. Durieux, V, and Gevenois, PA. Bibliometric indicators: quality measurements of scientific publication. Radiology. (2010) 255:342–51. doi: 10.1148/radiol.09090626
12. Ding, X, and Yang, Z. Knowledge mapping of platform research: a visual analysis using VOSviewer and CiteSpace. Electron Commer Res. (2022) 22:787–809. doi: 10.1007/s10660-020-09410-7
13. Chen, C . Searching for intellectual turning points: progressive knowledge domain visualization. Proc Natl Acad Sci. (2004) 101:5303–10. doi: 10.1073/pnas.0307513100
14. Van Eck, N, and Waltman, L. Software survey: VOSviewer, a computer program for bibliometric mapping. Scientometrics. (2010) 84:523–38. doi: 10.1007/s11192-009-0146-3
15. Aria, M, and Cuccurullo, C. Bibliometrix: an R-tool for comprehensive science mapping analysis. J Informet. (2017) 11:959–75. doi: 10.1016/j.joi.2017.08.007
17. Matsuda, N, Sato, S, Shiba, K, Okatsu, K, Saisho, K, Gautier, CA, et al. PINK1 stabilized by mitochondrial depolarization recruits Parkin to damaged mitochondria and activates latent Parkin for mitophagy. J Cell Biol. (2010) 189:211–21. doi: 10.1083/jcb.200910140
18. Noda, S, Sato, S, Fukuda, T, Tada, N, Uchiyama, Y, Tanaka, K, et al. Loss of Parkin contributes to mitochondrial turnover and dopaminergic neuronal loss in aged mice. Neurobiol Dis. (2020) 136:104717. doi: 10.1016/j.nbd.2019.104717
19. Lin, CH, Tsai, PI, Lin, HY, Hattori, N, Funayama, M, Jeon, B, et al. Mitochondrial UQCRC1 mutations cause autosomal dominant parkinsonism with polyneuropathy. Brain. (2020) 143:3352–73. doi: 10.1093/brain/awaa279
20. Yokota, M, Yoshino, Y, Hosoi, M, Hashimoto, R, Kakuta, S, Shiga, T, et al. Reduced ER-mitochondrial contact sites and mitochondrial ca(2+) flux in PRKN-mutant patient tyrosine hydroxylase reporter iPSC lines. Front Cell Dev Biol. (2023) 11:1171440. doi: 10.3389/fcell.2023.1171440
21. Noda, S, Sato, S, Fukuda, T, Ueno, S, Tada, N, and Hattori, N. Impaired mitochondrial accumulation and Lewy pathology in neuron-specific FBXO7-deficient mice. Mol Brain. (2022) 15:54. doi: 10.1186/s13041-022-00936-5
22. Ikeda, A, Imai, Y, and Hattori, N. Neurodegeneration-associated mitochondrial proteins, CHCHD2 and CHCHD10-what distinguishes the two? Front Cell Dev Biol. (2022) 10:996061. doi: 10.3389/fcell.2022.996061
23. Youle, RJ, and Narendra, DP. Mechanisms of mitophagy. Nat Rev Mol Cell Biol. (2011) 12:9–14. doi: 10.1038/nrm3028
24. Youle, RJ, and van der Bliek, AM. Mitochondrial fission, fusion, and stress. Science. (2012) 337:1062–5. doi: 10.1126/science.1219855
25. Sliter, DA, Martinez, J, Hao, L, Chen, X, Sun, N, Fischer, TD, et al. Parkin and PINK1 mitigate STING-induced inflammation. Nature. (2018) 561:258–62. doi: 10.1038/s41586-018-0448-9
26. Esteves, AR, Arduíno, DM, Swerdlow, RH, Oliveira, CR, and Cardoso, SM. Oxidative stress involvement in alpha-synuclein oligomerization in Parkinson's disease cybrids. Antioxid Redox Signal. (2009) 11:439–48. doi: 10.1089/ars.2008.2247
27. Esteves, AR, Arduíno, DM, Swerdlow, RH, Oliveira, CR, and Cardoso, SM. Microtubule depolymerization potentiates alpha-synuclein oligomerization. Front Aging Neurosci. (2010) 1:5. doi: 10.3389/neuro.24.005.2009
28. Sherer, TB, Betarbet, R, Stout, AK, Lund, S, Baptista, M, Panov, AV, et al. An in vitro model of Parkinson's disease: linking mitochondrial impairment to altered alpha-synuclein metabolism and oxidative damage. J Neurosci. (2002) 22:7006–15. doi: 10.1523/jneurosci.22-16-07006.2002
29. Sherer, TB, Betarbet, R, Testa, CM, Seo, BB, Richardson, JR, Kim, JH, et al. Mechanism of toxicity in rotenone models of Parkinson's disease. J Neurosci. (2003) 23:10756–64. doi: 10.1523/jneurosci.23-34-10756.2003
30. Sherer, TB, Kim, JH, Betarbet, R, and Greenamyre, JT. Subcutaneous rotenone exposure causes highly selective dopaminergic degeneration and alpha-synuclein aggregation. Exp Neurol. (2003) 179:9–16. doi: 10.1006/exnr.2002.8072
31. Dias, V, Junn, E, and Mouradian, MM. The role of oxidative stress in Parkinson's disease. J Parkinsons Dis. (2013) 3:461–91. doi: 10.3233/jpd-130230
32. Goedert, M, Spillantini, MG, Del Tredici, K, and Braak, H. 100 years of Lewy pathology. Nat Rev Neurol. (2013) 9:13–24. doi: 10.1038/nrneurol.2012.242
33. Dryanovski, DI, Guzman, JN, Xie, Z, Galteri, DJ, Volpicelli-Daley, LA, Lee, VM, et al. Calcium entry and α-synuclein inclusions elevate dendritic mitochondrial oxidant stress in dopaminergic neurons. J Neurosci. (2013) 33:10154–64. doi: 10.1523/jneurosci.5311-12.2013
34. Valente, EM, Abou-Sleiman, PM, Caputo, V, Muqit, MM, Harvey, K, Gispert, S, et al. Hereditary early-onset Parkinson's disease caused by mutations in PINK1. Science. (2004) 304:1158–60. doi: 10.1126/science.1096284
35. Scarffe, LA, Stevens, DA, Dawson, VL, and Dawson, TM. Parkin and PINK1: much more than mitophagy. Trends Neurosci. (2014) 37:315–24. doi: 10.1016/j.tins.2014.03.004
36. Shiba-Fukushima, K, Imai, Y, Yoshida, S, Ishihama, Y, Kanao, T, Sato, S, et al. PINK1-mediated phosphorylation of the Parkin ubiquitin-like domain primes mitochondrial translocation of Parkin and regulates mitophagy. Sci Rep. (2012) 2:1002. doi: 10.1038/srep01002
37. Deas, E, Wood, NW, and Plun-Favreau, H. Mitophagy and Parkinson's disease: the PINK1-parkin link. Biochim Biophys Acta. (2011) 1813:623–33. doi: 10.1016/j.bbamcr.2010.08.007
38. Exner, N, Lutz, AK, Haass, C, and Winklhofer, KF. Mitochondrial dysfunction in Parkinson's disease: molecular mechanisms and pathophysiological consequences. EMBO J. (2012) 31:3038–62. doi: 10.1038/emboj.2012.170
39. Subramaniam, SR, and Chesselet, MF. Mitochondrial dysfunction and oxidative stress in Parkinson's disease. Prog Neurobiol. (2013) 106-107:17–32. doi: 10.1016/j.pneurobio.2013.04.004
40. Essam, RM, and Kandil, EA. P-CREB and p-DARPP-32 orchestrating the modulatory role of cAMP/PKA signaling pathway enhanced by Roflumilast in rotenone-induced Parkinson's disease in rats. Chem Biol Interact. (2023) 372:110366. doi: 10.1016/j.cbi.2023.110366
41. Uspalenko, NI, Mosentsov, AA, Khmil, NV, Pavlik, LL, Belosludtseva, NV, Khunderyakova, NV, et al. Uridine as a regulator of functional and Ultrastructural changes in the brain of rats in a model of 6-OHDA-induced Parkinson's disease. Int J Mol Sci. (2023) 24:14304. doi: 10.3390/ijms241814304
42. Vaidya, B, Polepalli, M, Sharma, SS, and Singh, JN. 2-Aminoethoxydiphenyl borate ameliorates mitochondrial dysfunctions in MPTP/MPP(+) model of Parkinson's disease. Mitochondrion. (2023) 69:95–103. doi: 10.1016/j.mito.2023.02.003
43. Pokusa, M, Hajdúchová, D, Menichová, V, Evinová, A, Hatoková, Z, and Kráľová-Trančíková, A. Vulnerability of subcellular structures to pathogenesis induced by rotenone in SH-SY5Y cells. Physiol Res. (2021) 70:89–99. doi: 10.33549/physiolres.934477
44. Zheng, Q, Liu, H, Zhang, H, Han, Y, Yuan, J, Wang, T, et al. Ameliorating mitochondrial dysfunction of neurons by biomimetic targeting nanoparticles mediated mitochondrial biogenesis to boost the therapy of Parkinson's disease. Adv Sci (Weinh). (2023) 10:e2300758. doi: 10.1002/advs.202300758
45. Hwu, PW, Kiening, K, Anselm, I, Compton, DR, Nakajima, T, Opladen, T, et al. Gene therapy in the putamen for curing AADC deficiency and Parkinson's disease. EMBO Mol Med. (2021) 13:e14712. doi: 10.15252/emmm.202114712
46. Behl, T, Kaur, I, Kumar, A, Mehta, V, Zengin, G, and Arora, S. Gene therapy in the Management of Parkinson's disease: potential of GDNF as a promising therapeutic strategy. Curr Gene Ther. (2020) 20:207–22. doi: 10.2174/1566523220999200817164051
47. Niethammer, M, Tang, CC, LeWitt, PA, Rezai, AR, Leehey, MA, Ojemann, SG, et al. Long-term follow-up of a randomized AAV2-GAD gene therapy trial for Parkinson's disease. JCI Insight. (2017) 2:e90133. doi: 10.1172/jci.insight.90133
48. Shults, CW, Oakes, D, Kieburtz, K, Beal, MF, Haas, R, Plumb, S, et al. Effects of coenzyme Q10 in early Parkinson disease: evidence of slowing of the functional decline. Arch Neurol. (2002) 59:1541–50. doi: 10.1001/archneur.59.10.1541
49. Prasuhn, J, Brüggemann, N, Hessler, N, Berg, D, Gasser, T, Brockmann, K, et al. An omics-based strategy using coenzyme Q10 in patients with Parkinson's disease: concept evaluation in a double-blind randomized placebo-controlled parallel group trial. Neurol Res Pract. (2019) 1:31. doi: 10.1186/s42466-019-0033-1
50. Lautrup, S, Sinclair, DA, Mattson, MP, and Fang, EF. NAD(+) in Brain Aging and Neurodegenerative Disorders. Cell Metab. (2019) 30:630–55. doi: 10.1016/j.cmet.2019.09.001
51. Brakedal, B, Dölle, C, Riemer, F, Ma, Y, Nido, GS, Skeie, GO, et al. The NADPARK study: a randomized phase I trial of nicotinamide riboside supplementation in Parkinson's disease. Cell Metab. (2022) 34:396–407.e396. doi: 10.1016/j.cmet.2022.02.001
52. Filadi, R, Pendin, D, and Pizzo, P. Mitofusin 2: from functions to disease. Cell Death Dis. (2018) 9:330. doi: 10.1038/s41419-017-0023-6
53. Mourier, A, Motori, E, Brandt, T, Lagouge, M, Atanassov, I, Galinier, A, et al. Mitofusin 2 is required to maintain mitochondrial coenzyme Q levels. J Cell Biol. (2015) 208:429–42. doi: 10.1083/jcb.201411100
54. Misko, A, Jiang, S, Wegorzewska, I, Milbrandt, J, and Baloh, RH. Mitofusin 2 is necessary for transport of axonal mitochondria and interacts with the Miro/Milton complex. J Neurosci. (2010) 30:4232–40. doi: 10.1523/jneurosci.6248-09.2010
55. Lee, S, Sterky, FH, Mourier, A, Terzioglu, M, Cullheim, S, Olson, L, et al. Mitofusin 2 is necessary for striatal axonal projections of midbrain dopamine neurons. Hum Mol Genet. (2012) 21:4827–35. doi: 10.1093/hmg/dds352
56. Gegg, ME, Cooper, JM, Chau, KY, Rojo, M, Schapira, AH, and Taanman, JW. Mitofusin 1 and mitofusin 2 are ubiquitinated in a PINK1/parkin-dependent manner upon induction of mitophagy. Hum Mol Genet. (2010) 19:4861–70. doi: 10.1093/hmg/ddq419
57. Kahle, PJ, and Haass, C. How does parkin ligate ubiquitin to Parkinson's disease? EMBO Rep. (2004) 5:681–5. doi: 10.1038/sj.embor.7400188
58. Franco-Iborra, S, Vila, M, and Perier, C. Mitochondrial quality control in neurodegenerative diseases: focus on Parkinson's disease and Huntington's disease. Front Neurosci. (2018) 12:342. doi: 10.3389/fnins.2018.00342
Keywords: Parkinson’s disease, mitochondria, bibliometrics, Web of Science, VOSviewer, CiteSpace
Citation: Chen Y-J, Xie M-R, Zhou S-Q and Liu F (2024) Mapping the research of mitochondria and Parkinson’s disease: a bibliometric analysis. Front. Neurol. 15:1413762. doi: 10.3389/fneur.2024.1413762
Edited by:
Liana Rosenthal, Johns Hopkins University, United StatesReviewed by:
Max Borsche, University of Lübeck, GermanyJulia C. Fitzgerald, University of Tübingen, Germany
Copyright © 2024 Chen, Xie, Zhou and Liu. This is an open-access article distributed under the terms of the Creative Commons Attribution License (CC BY). The use, distribution or reproduction in other forums is permitted, provided the original author(s) and the copyright owner(s) are credited and that the original publication in this journal is cited, in accordance with accepted academic practice. No use, distribution or reproduction is permitted which does not comply with these terms.
*Correspondence: Fang Liu, bXNsaXVmYW5nMjNAMTI2LmNvbQ==; Sheng-Qiang Zhou, NTQ5MTYwOTQxQHFxLmNvbQ==
†These authors have contributed equally to this work and share first authorship