- 1Department of Neurology, Zhuhai People’s Hospital, Zhuhai, Guangdong, China
- 2Department of Health Management, Zhuhai People’s Hospital, Zhuhai, Guangdong, China
Amidst rising Parkinson’s disease (PD) incidence in an aging global population, the need for non-invasive and reliable diagnostic methods is increasingly critical. This review evaluates the strategic role of transcranial sonography (TCS) in the early detection and monitoring of PD. TCS’s ability to detect substantia nigra hyperechogenicity offers profound insights into its correlation with essential neuropathological alterations—namely, iron accumulation, neuromelanin depletion, and glial proliferation—fundamental to PD’s pathophysiology. Our analysis highlights TCS’s advantages, including its non-invasiveness, cost-effectiveness, and ease of use, positioning it as an invaluable tool for early diagnosis and continual disease progression monitoring. Moreover, TCS assists in identifying potential risk and protective factors, facilitating tailored therapeutic strategies to enhance clinical outcomes. This review advocates expanding TCS utilization and further research to maximize its diagnostic and prognostic potential in PD management, contributing to a more nuanced understanding of the disease.
Introduction
Parkinson’s disease (PD) is a common central nervous system disease in middle-aged and elderly people and is the second-most degenerative disease of the nervous system after Alzheimer’s disease. According to Wirdefeldt et al. (1), 1% of people over 60 years of age are diagnosed with PD. An epidemiological survey showed that its prevalence in the population over 65 years was close to 1.7% (2). The age of onset of PD is mostly over 50 years, and the incidence is slightly higher incidence in males than females. The main pathological features of PD are the loss of dopaminergic neurons and the formation of Lewy bodies in the substantial nigra (SN) pars compacta (SNc) (3). At present, the diagnosis of PD still mainly depends on clinical manifestations. The assessment of disease stage and severity also mainly relies on the subjective evaluation of clinicians, with few objective auxiliary examination methods (4). The current diagnostic criteria for PD are based on the diagnostic criteria for PD in the UK Brain Bank and were developed with reference to the clinical diagnostic criteria for PD launched by the International Parkinson and Movement Disorder Society in 2015 (5). Since Becker et al. (6) first reported that transcranial sonography (TCS) through the temporal window could detect the characteristic manifestation of SN hyperechogenicity in patients with PD in 1995, an increasing number of scholars in China and other countries have carried out applied research on PD through TCS. In this paper, we focus on the mechanisms and clinical relevance of the emergence of SN hyperechogenicity arises in patients with PD.
TCS examination of patients with PD showed that SN hyperecho was related to iron deposition
It is not well understood how SN hyperechogenicity on TCS arises in patients with PD. Existing studies mainly focus on iron ion deposition, reductions in neuromelanin (NM), and glial cell hyperplasia (7–9). Patients with PD have abnormal iron deposition in the brain (10), and the accumulated iron undergoes the Fenton reaction (in which ferrous salts and hydrogen peroxide combine to form hydroxyl free radical, which has a powerful oxidative degradation effect) and the Haber-Weiss reaction (in which hydrogen peroxide is converted to hydroxyl free radicals under the action of ferrous ions) and will produce a large amount of strongly oxidizing hydroxyl free radical. This hydroxyl radical causes oxidation of neural membrane lipids, causing disruption of dopamine nerve cell membrane function and ultimately progressive loss of dopaminergic neurons (11). At the same time, pathological iron deposition can induce α-synuclein accumulation, over expression, and abnormal folding that can lead to the generation of Lewy bodies, giving rise to PD (12). Acosta-Cabronnero et al. (13) conducted a quantitative susceptibility mapping (QSM) (a recently developed magnetic resonance imaging technique based on susceptibility-weighted imaging sequences that can accurately quantify iron deposition in patients’ brains) study that included 25 patients with PD and 50 healthy controls (14). They showed that patients with PD had significantly more iron deposition in the SNc than the control group, and the abnormal region of iron deposition in the brain coincided with the region of ɑ-synuclein distribution. In recent years, QSM studies of patients with PD have found an abnormal increase in iron deposition in SN, suggesting a strong association between PD and iron deposition in the brain, especially in SN (15). Azuma et al. (16) applied QSM to compare 24 patients with PD and 24 healthy controls. After categorizing the degree of bilateral limb involvement in the disease course, found that the magnetic susceptibility (an indicator of QSM that shows a linear relationship with iron deposition in the brain) of the SN on the severely affected side was higher than the mildly affected side and control group, and the iron deposition in the SN of PD patients was significantly higher compared to the healthy control group. Another study showed that this change in iron deposition has already occurred by the early stages of PD. In a study that included 60 patients with PD and 40 healthy controls, according to the Hoehn & Yahr (H-Y) stage, Guan et al. (17) divided patients with PD into the early-stage group (H-Y stage ≤2.5) and the late-stage group (H-Y stage ≥3). The iron deposition in SN was significantly higher in both early-stage PD and late-stage PD groups compared to the control group. Additionally, in the late-stage PD group, the iron deposition level in the SNc was higher compared to both the control and early-stage PD group. In addition, studies by He et al. (18) and An et al. (19) found that the Unified Parkinson’s Disease Rating Scale (UPDRS) total score (UPDRS score indicates a total of 199 points) and UPDRS-III score (UPDRS-III scores a total of 56 points) were significantly higher in late-stage patients with PD than early-stage patients with PD, and UPDRS-III score was positively correlated with iron deposition in SN. That is, the more pronounced the motor symptoms in patients with PD, the higher the iron deposition in SN, which again confirmed that PD is closely related to iron deposition in SN and that there is a correlation between iron deposition and disease severity.
Iron plays a crucial role in many biological processes, including oxygen transportation, DNA synthesis, and electron transport in neurons. However, excessive iron accumulation can be toxic and is associated with oxidative stress and neuronal damage (20). This oxidative stress is thought to contribute to the degeneration of dopaminergic neurons observed in PD (20). In the study by Zecca et al. (14), TCS was utilized to measure the echogenic area of the SN region and analyze its correlation with the concentrations of iron, H-ferritin, and L-ferritin. The results indicated a significant positive correlation between the echogenic area of the SN and the concentrations of iron and its associated proteins (14). The hyperechogenicity observed could be partly due to the increased iron deposition which reflects ultrasound waves more than the surrounding tissues (21). Ceruloplasmin’s ferroxidase activity is essential for preventing iron accumulation in the brain (22). Lower activity leads to increased levels of ferrous iron within the neurons, promoting oxidative stress and contributing to the dopaminergic neuron loss characteristic of PD. TCS can sensitively detect pathological metal accumulation (23). Berg et al. (24) found that injection of iron and 6-hydroxydopamine (6-OHDA) (a destructive agent of dopaminergic cells that can release iron from ferritin) enhanced the echogenicity of SN in a dose-dependent manner. In 2006, their team autopsied the brain tissues of 60 patients with PD of different ages and confirmed that the echogenic characteristics of the SN were positively correlated with iron deposition in the SN and were not correlated with other heavy metal levels (metals with densities above 4.5 g/m3), such as copper (25).
SN hyperechogenicity is not unique to PD. SN hyperechogenicity can be detected in 90% of patients with PD as well as in approximately 8–14% of healthy individuals (26). In normal populations, iron deposition most often occurs during adolescence and early adulthood. The structures with the highest iron content in brain tissue are the globus pallidus and SN, which gradually decrease in adulthood. A study of 109 newborns showed that the SN region of most newborns exhibits significantly elevated echogenic on TCS, which diminish gradually with age. By around the age of 16, the echogenic level in the SN approaches that of adults. This suggests that iron metabolism and brain tissue development have reached maturity at this stage (27). A study of 330 healthy subjects showed that 8–9% of the subjects showed the phenomenon of SN hyperechogenicity. Positron-emission tomography of 10 subjects with SN hyperechogenicity revealed a significant decrease in striatal dopamine uptake, indicating that although there were no signs or symptoms of PD, the subjects with enhanced SN echogenicity may have subclinical or preclinical striatum damage (28). We speculate that the SN hyperechogenicity in adolescence and early adulthood may suggest an increased susceptibility to SN injury if the SN hyperechogenicity does not undergo a normal physiological decline.
TCS examination of patients with PD showed that SN hyperecho was associated with NM reduction
A multivariate analysis of TCS data (14) showed that SN echo intensity and area were negatively correlated with NM content. NM is an insoluble brown pigment composed of covalently bound melanin groups, lipids, and peptides. It is a byproduct of catecholamine oxidative catabolism and is present in a variety of neurons, mainly in the dopaminergic neurons of the SN (28). PD is a neurodegenerative disease characterized by selective loss of NM-containing neurons in SNc and the appearance of Lewy bodies. Pathological studies have confirmed that the NM of the SNC is significantly lower in patients with PD (29, 30). NM can reduce the oxidative damage of cells by removing excessive cytosolic dopamine and chelated iron and avoid the oxidative stress response in brain tissue (31, 32). In contrast, patients with PD have a significant loss of NM content in the SN compared to normal subjects (30). A decrease in the ability of NM to chelate iron leads to an accumulation of free iron in the cell, which in turn leads to an increase in the degree of oxidative damage in the cell.
TCS examination of patients with PD showed that SN hyperecho was associated with gliosis
In an autopsy study (33), Berg found that the echogenic properties of the SN were related to the activation of microglia after correction of iron and NM content, showing that microglial activation was an independent factor influencing the changes in SN echogenicity. Additionally, astrocytes also play an important role in SN echogenicity alterations. It is widely accepted that the PD-related glial cells are mainly astrocytes and microglia. Astrocytes are the most abundant cell type in the central nervous system, and play a dual role in the occurrence and progression of PD. On the one hand, glial-derived neurotrophic factors secreted by astrocytes can promote the survival and morphological differentiation of midbrain dopaminergic neurons, and increase their affinity for dopamine uptake, thereby mitigating the pathological progression of PD. On the other hand, in PD patients, astrocytes gathered a large number of α-synuclein proteins, these proteins are likely to be toll-like receptors and trigger inflammation upon recognition by molecular ligands, potentially leading to further damage and death of dopaminergic (34–37).
Microglia are innate immune cells that protect neurons from harmful stimuli (38). They account for 12% of the cells in the entire central nervous system. They are distributed throughout the brain parenchyma and form the first line of immune defense in the nervous system. After proliferation and activation, they can engulf cell debris and the myelin sheath of the lesion, eliminating dead cells and degenerative material and promoting tissue repair (39).
Cicchetti et al. (40) used a stereotactic technique to inject neurotoxin 6-OHDA into the right striatum of rats to create a PD animal model and found that the number of right SN dopaminergic neurons was significantly reduced. At the same time, the numbers of astrocytes and microglia significantly increased. Tumor necrosis factor-alpha (TNF-α) was positively expressed in the right SN and striatum of the model group, and it was mainly distributed on activated microglia. In the 1-methyl-4-phenyl-1,2,3,6-tetrahydropyridine (MPTP)-induced mouse PD model, the degree of loss of dopaminergic neurons was parallel to the activation of microglia at this site (40). An insecticide, rotenone, can cause symptoms and pathological changes similar to PD in rodents, and local microglial activation has been observed without exception in the rotenone-exposure animal model (41). Glial fibrillary acidic protein (GFAP) is a marker of astrocyte activation. GFAP expression was significantly increased in the autopsy of PD patients and in the brain tissue of PD animal models. This result suggests that many activated proliferative astrocytes are active in the pathological process of PD (42). The injection of lipopolysaccharide into the SNc of male mice can cause the activation of microglia and produce a large amount of interleukin-1β (IL-1β) (43). Injecting NM into the SN of rats, it was also observed that extracellular NM rapidly induced the activation of microglial. Activated microglia produced large amounts of oxidative stressors and pro-inflammatory factors, thereby inducing dopaminergic neurodegeneration in patients with PD (44). The above studies indicate that the neuroinflammatory response induced by the activation of microglia and astrocytes plays a decisive role in the occurrence and progression of PD.
TNF-α is mainly produced by activated macrophages, NK cells and T lymphocytes. TNF-α can stimulate cerebrovascular endothelial cells to express adhesion factors and activate glial cells to produce more inflammatory mediators, thus stimulating the body’s local disease response. Significantly elevated IL-1β and other inflammatory factors have been found in cerebrospinal fluid and serum of patients with PD, but the manifestations of different inflammatory factors are different in cerebrospinal fluid and serum, and the increase of serum IL-1β indicates the rapid development of PD (45). Studies have found that the injection of 6-OHDA into the striatum induced PD rat model can reduce the inflammation of injured striatal neurons by inhibiting the expression of IL-1β and other inflammatory cytokines (46). Some studies have also pointed out that lipopolysaccharide activates microglia and induces neuroinflammation by accelerating the expression of various pro-inflammatory cytokines such as IL-1β (47). Studies have shown that the occurrence and development of PD may be due to the cytotoxic effect caused by the increased expression of IL-1β, which leads to the chronic degeneration and even death of neurons (45). IL-6 is a cytokine. It acts on the blood–brain barrier, altering its permeability in such a way that peripheral immune cells can penetrate into the center, activate glial cells to produce inflammatory factors, and induce or exacerbate neurodegenerative disease (48, 49).
Studies on the mechanism of SN hyperechogenicity (shown in Table 1, Figure 1) have shown a close connection between SN hyperechogenicity and PD pathophysiology. Understanding the mechanism of SN hyperechogenicity and its relationship with the pathophysiological changes of PD may provide new ideas for the clinical treatment of PD. Reducing iron deposition in SN, upholding the intracellular levels of NM, and antagonizing glial cell proliferation may delay the pathological changes in PD and the occurrence of neurological deficit symptoms.
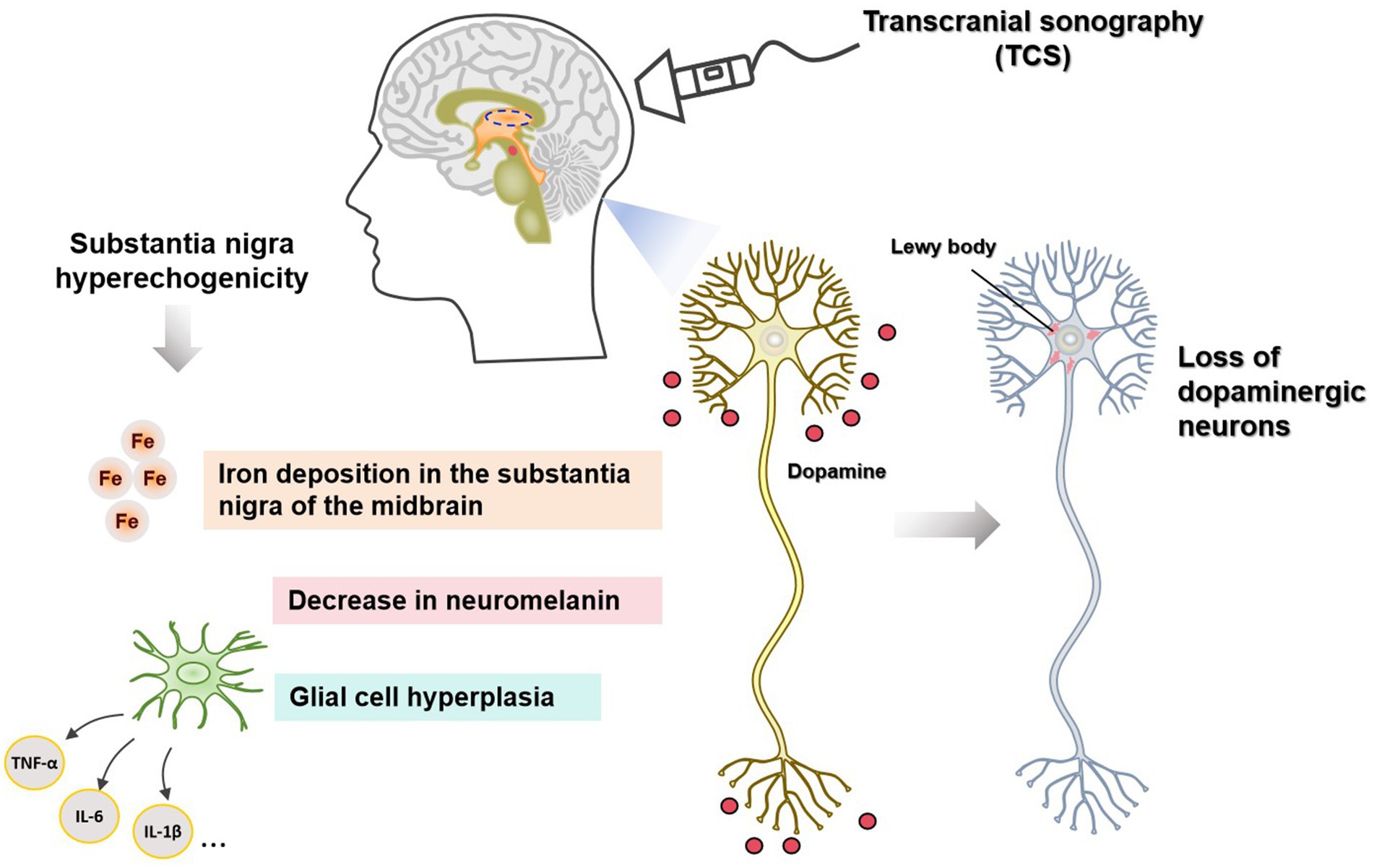
Figure 1. Mechanisms of substantial nigra (SN) hyperecho generation on the transcranial sonography (TCS) in patients with Parkinson’s disease.
The association between SN hyperechogenicity and PD subtypes
Recent research in the field of PD has increasingly focused on the varied manifestations of the disease, particularly the distinctions between the postural instability gait difficulty (PIGD) and tremor dominant (TD) subtypes (50, 51). Transcranial sonography (TCS), which detects hyperechogenicity of the substantia nigra (HSN), presents a valuable tool in exploring these differences. HSN has been correlated with various pathological and clinical features of PD, suggesting that it may play a role in distinguishing PD subtypes. Studies have indicated that HSN is more frequently observed and tends to be more pronounced in patients diagnosed with the PIGD subtype as compared to the TD subtype (52). This difference may reflect the underlying pathophysiological variations between these forms of PD. For example, patients with PIGD tend to exhibit a greater overall neurodegenerative burden, including more extensive loss of dopaminergic neurons and higher levels of iron deposition in the substantia nigra. These factors contribute to the increased echogenicity observed via TCS (53). Furthermore, the prevalence and intensity of HSN in PIGD patients have been associated with more severe motor dysfunctions and a faster disease progression, underscoring the clinical relevance of TCS as a diagnostic and prognostic tool (54). This imaging technique could potentially aid clinicians in more effectively anticipating disease trajectory and tailoring interventions. Conversely, the TD subtype shows a less consistent association with HSN. Some studies suggest a lower occurrence of HSN among TD patients, which may align with the generally slower progression rate and milder motor impairment observed in this group (55). The differential findings in HSN prevalence between PIGD and TD subtypes underscore the importance of considering subtype-specific pathologies in PD diagnosis and management. This emerging evidence suggests that TCS, through the assessment of HSN, may become an integral part of the diagnostic process, helping to differentiate between PD subtypes at an early stage. It also highlights the need for further research to elucidate the mechanisms driving the differences in substantia nigra hyperechogenicity and their implications for PD subtype diagnosis and treatment strategies.
Clinical relevance of the use of TCS for SN hyperechogenicity in patients with PD
In more than 90% of patients with PD, TCS indicates that SN hyperechogenicity is a typical and stable feature. Clinical correlation studies on SN echo characteristics (including echo intensity and area size) have lacked the support of large samples. Few clinical indicators were included in the study. In addition to the factors that have been discussed such as sex, age, onset mode, disease course, and disease severity, other clinical factors such as plasma uric acid, blood lipids, vitamin D, and homocysteine, may also be associated with SN hyperechogenicity. However, large-scale, multicenter studies are needed to be confirm theses associations.
Correlation with sex, age, onset pattern, disease course, and disease severity
Various studies have reached inconsistent conclusions on the correlation between SN hyperechoic characteristics and sex, age, mode of onset, and disease severity. Becker et al. (6) analyzed and compared 30 patients with PD and 30 healthy controls and found that SN hyperechogenicity was associated with disease severity. In 2010, a case–control study involving 115 subjects found that the SN hyperechoic area was larger in patients with onset of akinetic-rigid and bradykinesia, and the SN hyperechoic characteristics were correlated with age but not with sex (56). Joana et al. (57) showed that the SN hyperechogenicity was not associated with the severity of the disease. In 2017, a study of 373 patients with PD also found that akinetic-rigid or mixed PD had a larger SN hyperechoic area than tremor-dominant PD, but the SN hyperechogenicity was not correlated with the disease course or disease severity (55). Toomsoo et al. (58) analyzed 300 patients with PD and 200 healthy controls and found that the SN hyperechoic area of middle-aged and elderly patients with PD was smaller than that of young patients with PD, and the SN echo area was positively correlated with age in healthy people. In another cross-sectional study involving 374 patients with PD, SN echo characteristics were positively correlated with sex, age, age of onset, and disease severity (9). In summary, the correlation between SN hyperechogenicity and sex, age, onset pattern, and disease severity still require more study to draw true and reliable conclusions.
Correlation with plasma uric acid level
In a meta-analysis that included 2,379 patients with PD and 2,367 controls, Wen et al. (59) found that the plasma uric acid level of patients with PD was significantly lower than that of healthy people. Plasma uric acid levels were lower in patients with PD with intermediate to advanced H-Y stage, indicating a negative association between plasma uric acid levels and H-Y stage. Another study corroborated their findings (60). A randomized controlled trial applied the uric acid precursor inosine to patients with PD and found that oral administration of inosine effectively increased the plasma uric acid level and delayed the progression of PD, further verifying the above conclusions (43). Uric acid is an important antioxidant and free radical scavenger in the human body that chelates iron. It lowers specific iron deposition in the SN region of patients with PD and plays a protective role in dopaminergic neurons. Therefore, a decrease in serum uric acid level may affect iron deposition and increase iron deposition in the SN area, thereby showing high echogenicity in TCS (61). Uric acid also acts as a scavenger of peroxynitrite, blocking nitrite-mediated nitration and preventing damage to cells caused by nitration of the protein tyrosine. It can be seen that a certain level of uric acid can reduce the oxidative stress damage of cells to a certain extent. Oxidative stress is one of the important pathogeneses of PD, and uric acid is likely to exert its neuroprotective effect by reducing the level of oxidative stress in PD (62).
Abnormal blood lipid metabolism (63, 64), plasma 25-hydroxyvitamin D levels (65–67), and plasma homocysteine levels (59, 68, 69) are associated with the occurrence and progression of PD. There have been few studies on whether these biochemical indicators are related to the characteristic SN echogenicity. The relevant factors affecting SN echogenicity in PD patients were analyzed and summarized to identify risk factors and protective factors. The results provide a theoretical basis for early intervention and individualized treatment of relevant risk factors in clinical practice.
Discussion and summary
The review explored TCS as a non-invasive method to detect abnormal hyperechogenicity in the substantia nigra of patients with PD, offering a comparison with other imaging techniques like MRI and CT scans, and discussed how the hyperechoic features of the substantia nigra, as revealed by TCS, correlate with various clinical indicators such as age, disease progression, severity, and certain biochemical markers. The review concluded that TCS can serve as a valuable tool for early intervention and personalized treatment strategies by identifying risk factors and protective factors for PD.
In analyzing the factors contributing to SN hyperechogenicity detected by TCS, we first emphasized the crucial role of iron deposition in the pathogenesis of PD. The echogenic properties of the SN are closely associated with iron deposition (15), highlighting its potential as a diagnostic and monitoring marker in TCS. Additionally, NM protects dopaminergic neurons through its antioxidant properties and ability to chelate iron ions. In PD, the reduction of NM leads to the accumulation of iron ions and increased oxidative stress, exacerbating neuronal degeneration. The echogenic characteristics of the SN reflect changes in iron deposition and NM content (30). Furthermore, we observed that the activation of microglial cells plays a key role in the pathological process of PD. Activated microglia release a substantial amount of oxidative stress products and pro-inflammatory factors, contributing to neuronal damage. While microglia can promote neuronal repair by phagocytosing cellular debris and removing damaged substances, dysregulated activation may result in chronic inflammation and neurotoxicity, thereby accelerating the progression of PD (34, 44).
In the final section of the review, we discussed the clinical significance of TCS and derived therapeutic insights based on the aforementioned detection mechanisms. These insights suggest that TCS could be a valuable tool not only for diagnosing PD but also for informing personalized treatment strategies. TCS can be used to demonstrate the characteristic structural changes of the SN in PD patients by using the principle of ultrasound imaging. The sensitivity of TCS in the diagnosis of PD is between 91 and 100% (50, 51). In 2013, the European Federation of Neurological Societies and the Movement Disorders Society recommended the finding of SN echogenic intensity > grade II and hyperechoic area > 0.25 cm2 on TCS as level A evidence for differentiating primary PD from atypical Parkinson’s syndrome, for early diagnosis of PD, and for screening patients at high risk for PD (52). A study on the diagnostic value of PD by Tao et al. (70) showed that the sensitivity and specificity of SN hyperechogenicity for PD were 84 and 85%, respectively. The sensitivity and specificity to distinguish PD from healthy people were 85 and 89%, respectively. The sensitivity and specificity to distinguish PD from other Parkinsonian’s syndromes were 82 and 74%, respectively. Our study, involving 97 patients with PD and 56 controls, found that TCS was 88.7% sensitive and 82.1% to the diagnosis of PD.
In conclusion, TCS has become an important auxiliary detection method for PD diagnosis and differential diagnosis due to its advantages of convenience, rapid detection, invasiveness, low cost, high reproducibility, and easy promotion. However, the mechanisms by which SN hyperechogenicity occurs, the factors that influence it, and its clinical relevance to TCS are not fully understood and therefore need to be investigated in more detail.
While TCS offers several advantages, such as being non-invasive, relatively low cost, and having the ability to provide immediate results, its limitations must be carefully considered. These include dependence on operator skills, challenges in image interpretation, limitations in the availability of acoustic windows, and issues with resolution and specificity. For clinical and research applications, these limitations suggest that TCS should be used in conjunction with other diagnostic tools to provide a more comprehensive assessment of neurological conditions. Future technological advances and standardized training may help mitigate some of these limitations, enhancing the reliability and applicability of TCS in clinical neurology.
Author contributions
Y-yZ: Conceptualization, Project administration, Writing – original draft, Writing – review & editing. X-hJ: Conceptualization, Project administration, Writing – original draft, Writing – review & editing. P-pZ: Data curation, Formal analysis, Writing – review & editing. W-yZ: Investigation, Methodology, Writing – review & editing. L-bL: Conceptualization, Project administration, Writing – original draft, Writing – review & editing.
Funding
The author(s) declare that financial support was received for the research, authorship, and/or publication of this article. This study is supported by the Clinical Research Promotion Project of Zhuhai People’s Hospital (No.2023LCTS-23).
Conflict of interest
The authors declare that the research was conducted in the absence of any commercial or financial relationships that could be construed as a potential conflict of interest.
Publisher’s note
All claims expressed in this article are solely those of the authors and do not necessarily represent those of their affiliated organizations, or those of the publisher, the editors and the reviewers. Any product that may be evaluated in this article, or claim that may be made by its manufacturer, is not guaranteed or endorsed by the publisher.
References
1. Wirdefeldt, K, Adami, HO, Cole, P, Trichopoulos, D, and Mandel, J. Epidemiology and etiology of Parkinson's disease: a review of the evidence. Eur J Epidemiol. (2011) 26 Suppl 1:1–58. doi: 10.1007/s10654-011-9581-6
2. ZHANG, Z, ROMAN, G, HONG, Z, WU, C, QU, Q, HUANG, J, et al. Parkinson's disease in China: prevalence in Beijing, Xian, and Shanghai. Lancet. (2005) 365:595–7. doi: 10.1016/S0140-6736(05)70801-1
3. Ye, H, Robak, LA, Yu, M, Cykowski, M, and Shulman, JM. Genetics and pathogenesis of Parkinson's syndrome. Annu Rev Pathol. (2023) 18:95–121. doi: 10.1146/annurev-pathmechdis-031521-034145
4. Postuma, RB, and Lang, AE. The clinical diagnosis of Parkinson's disease-we are getting better. Mov Disord. (2023) 38:515–7. doi: 10.1002/mds.29319
5. Postuma, RB, Berg, D, Stern, M, Poewe, W, Olanow, CW, Oertel, W, et al. MDS clinical diagnostic criteria for Parkinson's disease. Mov Disord. (2015) 30:1591–601. doi: 10.1002/mds.26424
6. Becker, G, Seufert, J, Bogdahn, U, Reichmann, H, and Reiners, K. Degeneration of substantia nigra in chronic Parkinson's disease visualized by transcranial color-coded real-time sonography. Neurology. (1995) 45:182–4. doi: 10.1212/WNL.45.1.182
7. Jiang, H, Wang, J, Rogers, J, and Xie, J. Brain Iron metabolism dysfunction in Parkinson's disease. Mol Neurobiol. (2017) 54:3078–101. doi: 10.1007/s12035-016-9879-1
8. Zhu, Y, Wang, B, Tao, K, Yang, H, Wang, Y, Zhou, T, et al. Iron accumulation and microglia activation contribute to substantia nigra hyperechogenicity in the 6-OHDA-induced rat model of Parkinson's disease. Parkinsonism Relat Disord. (2017) 36:76–82. doi: 10.1016/j.parkreldis.2017.01.003
9. Yu, SY, Cao, CJ, Zuo, LJ, Chen, ZJ, Lian, TH, Wang, F, et al. Clinical features and dysfunctions of iron metabolism in Parkinson disease patients with hyper echogenicity in substantia nigra: a cross-sectional study. BMC Neurol. (2018) 18:9. doi: 10.1186/s12883-018-1016-5
10. Zeng, W, Cai, J, Zhang, L, and Peng, Q. Iron deposition in Parkinson's disease: a Mini-review. Cell Mol Neurobiol. (2024) 44:26. doi: 10.1007/s10571-024-01459-4
11. Ward, RJ, Zucca, FA, Duyn, JH, Crichton, RR, and Zecca, L. The role of iron in brain ageing and neurodegenerative disorders. Lancet Neurol. (2014) 13:1045–60. doi: 10.1016/S1474-4422(14)70117-6
12. Zhao, Q, Tao, Y, Zhao, K, Ma, Y, Xu, Q, Liu, C, et al. Structural insights of Fe(3+) induced alpha-synuclein fibrillation in Parkinson's disease. J Mol Biol. (2023) 435:167680. doi: 10.1016/j.jmb.2022.167680
13. Acosta-Cabronero, J, Cardenas-Blanco, A, Betts, MJ, Butryn, M, Valdes-Herrera, JP, Galazky, I, et al. The whole-brain pattern of magnetic susceptibility perturbations in Parkinson's disease. Brain. (2017) 140:118–31. doi: 10.1093/brain/aww278
14. Zecca, L, Berg, D, Arzberger, T, Ruprecht, P, Rausch, WD, Musicco, M, et al. In vivo detection of iron and neuromelanin by transcranial sonography: a new approach for early detection of substantia nigra damage. Mov Disord. (2005) 20:1278–85. doi: 10.1002/mds.20550
15. Riederer, P, Sofic, E, Rausch, WD, Schmidt, B, Reynolds, GP, Jellinger, K, et al. Transition metals, ferritin, glutathione, and ascorbic acid in parkinsonian brains. J Neurochem. (2010) 52:515–20. doi: 10.1111/j.1471-4159.1989.tb09150.x
16. Azuma, M, Hirai, T, Yamada, K, Yamashita, S, Ando, Y, Tateishi, M, et al. Lateral asymmetry and spatial difference of Iron deposition in the substantia Nigra of patients with Parkinson disease measured with quantitative susceptibility mapping. AJNR Am J Neuroradiol. (2016) 37:782–8. doi: 10.3174/ajnr.A4645
17. Guan, X, Xuan, M, Gu, Q, Huang, P, Liu, C, Wang, N, et al. Regionally progressive accumulation of iron in Parkinson's disease as measured by quantitative susceptibility mapping. NMR Biomed. (2017) 30:10. doi: 10.1002/nbm.3489
18. He, N, Ling, H, Ding, B, Huang, J, Zhang, Y, Zhang, Z, et al. Region-specific disturbed iron distribution in early idiopathic Parkinson's disease measured by quantitative susceptibility mapping. Hum Brain Mapp. (2015) 36:4407–20. doi: 10.1002/hbm.22928
19. An, H, Zeng, X, Niu, T, Li, G, Yang, J, Zheng, L, et al. Quantifying iron deposition within the substantia nigra of Parkinson's disease by quantitative susceptibility mapping. J Neurol Sci. (2018) 386:46–52. doi: 10.1016/j.jns.2018.01.008
20. Mahoney-Sánchez, L, Bouchaoui, H, Ayton, S, Devos, D, Duce, JA, and Devedjian, JC. Ferroptosis and its potential role in the physiopathology of Parkinson's disease. Prog Neurobiol. (2021) 196:101890. doi: 10.1016/j.pneurobio.2020.101890
21. Garcia-Malo, C, Wanner, V, Miranda, C, Romero Peralta, S, Agudelo, L, Cano-Pumarega, I, et al. Quantitative transcranial sonography of the substantia nigra as a predictor of therapeutic response to intravenous iron therapy in restless legs syndrome. Sleep Med. (2020) 66:123–9. doi: 10.1016/j.sleep.2019.09.020
22. Wang, J, Bi, M, and Xie, J. Ceruloplasmin is involved in the Nigral Iron accumulation of 6-OHDA-lesioned rats. Cell Mol Neurobiol. (2015) 35:661–8. doi: 10.1007/s10571-015-0161-2
23. Becker, G, and Berg, D. Neuroimaging in basal ganglia disorders: perspectives for transcranial ultrasound. Mov Disord. (2001) 16:23–32. doi: 10.1002/1531-8257(200101)16:1<23::AID-MDS1003>3.0.CO;2-2
24. Berg, D, Grote, C, Rausch, WD, Mäurer, M, Wesemann, W, Riederer, P, et al. Iron accumulation in the substantia nigra in rats visualized by ultrasound. Ultrasound Med Biol. (1999) 25:901–4. doi: 10.1016/S0301-5629(99)00046-0
25. Berg, D. In vivo detection of iron and neuromelanin by transcranial sonography – a new approach for early detection of substantia nigra damage. J Neural Transm (Vienna). (2006) 113:775–80. doi: 10.1007/s00702-005-0447-5
26. Bor-Seng-Shu, E, Paschoal, FM, Almeida, KJ, de Lima Oliveira, M, Nogueira, RC, Teixeira, MJ, et al. Transcranial brain sonography for parkinsonian syndromes. J Neurosurg Sci. (2019) 63:441–9. doi: 10.23736/S0390-5616.19.04696-4
27. Berg, D, Merz, B, Reiners, K, Naumann, M, and Becker, G. Five-year follow-up study of hyperechogenicity of the substantia nigra in Parkinson's disease. Mov Disord. (2005) 20:383–5. doi: 10.1002/mds.20311
28. Zecca, L, Bellei, C, Costi, P, Albertini, A, Monzani, E, Casella, L, et al. New melanic pigments in the human brain that accumulate in aging and block environmental toxic metals. Proc Natl Acad Sci USA. (2008) 105:17567–72. doi: 10.1073/pnas.0808768105
29. Nagatsu, T, Nakashima, A, Watanabe, H, Ito, S, and Wakamatsu, K. Neuromelanin in Parkinson's disease: tyrosine hydroxylase and Tyrosinase. Int J Mol Sci. (2022) 23:785–91. doi: 10.3390/ijms23084176
30. Zecca, L, Fariello, R, Riederer, P, Sulzer, D, Gatti, A, and Tampellini, D. The absolute concentration of nigral neuromelanin, assayed by a new sensitive method, increases throughout the life and is dramatically decreased in Parkinson's disease. FEBS Lett. (2002) 510:216–20. doi: 10.1016/S0014-5793(01)03269-0
31. Sulzer, D, Bogulavsky, J, Larsen, KE, Behr, G, Karatekin, E, Kleinman, MH, et al. Neuromelanin biosynthesis is driven by excess cytosolic catecholamines not accumulated. Proc Natl Acad Sci U S A. (2000) 97:11869–74. doi: 10.1073/pnas.97.22.11869
32. Zecca, L, and Swartz, HM. Total and paramagnetic metals in human substantia nigra and its neuromelanin. J Neural Transm Park Dis Dement Sect. (1993) 5:203–13. doi: 10.1007/BF02257675
33. Berg, D, Godau, J, Riederer, P, Gerlach, M, and Arzberger, T. Microglia activation is related to substantia nigra echogenicity. J Neural Transm (Vienna). (2010) 117:1287–92. doi: 10.1007/s00702-010-0504-6
34. Stefanova, N, Fellner, L, Reindl, M, Masliah, E, Poewe, W, and Wenning, GK. Toll-like receptor 4 promotes alpha-synuclein clearance and survival of nigral dopaminergic neurons. Am J Pathol. (2011) 179:954–63. doi: 10.1016/j.ajpath.2011.04.013
35. Fellner, L, Irschick, R, Schanda, K, Reindl, M, Klimaschewski, L, Poewe, W, et al. Toll-like receptor 4 is required for alpha-synuclein dependent activation of microglia and astroglia. Glia. (2013) 61:349–60. doi: 10.1002/glia.22437
36. Gu, XL, Long, CX, Sun, L, Xie, C, Lin, X, and Cai, H. Astrocytic expression of Parkinson's disease-related A53T alpha-synuclein causes neurodegeneration in mice. Mol Brain. (2010) 3:12. doi: 10.1186/1756-6606-3-12
37. Sun, H, Liang, R, Yang, B, Zhou, Y, Liu, M, Fang, F, et al. Aquaporin-4 mediates communication between astrocyte and microglia: implications of neuroinflammation in experimental Parkinson's disease. Neuroscience. (2016) 317:65–75. doi: 10.1016/j.neuroscience.2016.01.003
38. Chai, M, Su, G, Gao, J, Chen, W, Wu, Q, Dong, Y, et al. Molecular mechanism of the protective effects of M2 microglia on neurons: a review focused on exosomes and secretory proteins. Neurochem Res. (2022) 47:3556–64. doi: 10.1007/s11064-022-03760-4
39. Block, ML, Zecca, L, and Hong, JS. Microglia-mediated neurotoxicity: uncovering the molecular mechanisms. Nat Rev Neurosci. (2007) 8:57–69. doi: 10.1038/nrn2038
40. Cicchetti, F, Brownell, AL, Williams, K, Chen, YI, Livni, E, and Isacson, O. Neuroinflammation of the nigrostriatal pathway during progressive 6-OHDA dopamine degeneration in rats monitored by immunohistochemistry and PET imaging. Eur J Neurosci. (2002) 15:991–8. doi: 10.1046/j.1460-9568.2002.01938.x
41. Sherer, TB, Betarbet, R, Kim, JH, and Greenamyre, JT. Selective microglial activation in the rat rotenone model of Parkinson's disease. Neurosci Lett. (2003) 341:87–90. doi: 10.1016/S0304-3940(03)00172-1
42. Scuderi, C, Stecca, C, Iacomino, A, and Steardo, L. Role of astrocytes in major neurological disorders: the evidence and implications. IUBMB Life. (2013) 65:957–61. doi: 10.1002/iub.1223
43. Schwarzschild, MA, Ascherio, A, Beal, MF, Cudkowicz, ME, Curhan, GC, Hare, JM, et al. Inosine to increase serum and cerebrospinal fluid urate in Parkinson disease: a randomized clinical trial. JAMA Neurol. (2014) 71:141–50. doi: 10.1001/jamaneurol.2013.5528
44. Zhang, W, Phillips, K, Wielgus, AR, Liu, J, Albertini, A, Zucca, FA, et al. Neuromelanin activates microglia and induces degeneration of dopaminergic neurons: implications for progression of Parkinson's disease. Neurotox Res. (2011) 19:63–72. doi: 10.1007/s12640-009-9140-z
45. Karpenko, MN, Vasilishina, AA, Gromova, EA, Muruzheva, ZM, Miliukhina, IV, and Bernadotte, A. Interleukin-1beta, interleukin-1 receptor antagonist, interleukin-6, interleukin-10, and tumor necrosis factor-alpha levels in CSF and serum in relation to the clinical diversity of Parkinson's disease. Cell Immunol. (2018) 327:77–82. doi: 10.1016/j.cellimm.2018.02.011
46. Dallé, E, Daniels, WMU, and Mabandla, MV. Fluvoxamine maleate normalizes striatal neuronal inflammatory cytokine activity in a parkinsonian rat model associated with depression. Behav Brain Res. (2017) 316:189–96. doi: 10.1016/j.bbr.2016.08.005
47. Zhang, FX, and Xu, RS. Juglanin ameliorates LPS-induced neuroinflammation in animal models of Parkinson's disease and cell culture via inactivating TLR4/NF-kappaB pathway. Biomed Pharmacother. (2018) 97:1011–9. doi: 10.1016/j.biopha.2017.08.132
48. YAN, J, FU, Q, CHENG, L, ZHAI, M, WU, W, HUANG, L, et al. Inflammatory response in Parkinson's disease (review). Mol Med Rep. (2014) 10:2223–33. doi: 10.3892/mmr.2014.2563
49. Chen, X, Lan, X, Roche, I, Liu, R, and Geiger, JD. Caffeine protects against MPTP-induced blood-brain barrier dysfunction in mouse striatum. J Neurochem. (2008) 107:1147–57. doi: 10.1111/j.1471-4159.2008.05697.x
50. Alonso-Cánovas, A, López-Sendón, JL, Buisán, J, deFelipe-Mimbrera, A, Guillán, M, García-Barragán, N, et al. Sonography for diagnosis of Parkinson disease-from theory to practice: a study on 300 participants. J Ultrasound Med. (2014) 33:2069–74. doi: 10.7863/ultra.33.12.2069
51. Toomsoo, T, Liepelt-Scarfone, I, Kerner, R, Kadastik-Eerme, L, Asser, T, Rubanovits, I, et al. Substantia Nigra Hyperechogenicity: validation of transcranial sonography for Parkinson disease diagnosis in a large Estonian cohort. J Ultrasound Med. (2016) 35:17–23. doi: 10.7863/ultra.14.12069
52. Berardelli, A, Wenning, GK, Antonini, A, Berg, D, Bloem, BR, Bonifati, V, et al. EFNS/MDS-ES/ENS [corrected] recommendations for the diagnosis of Parkinson's disease. Eur J Neurol. (2013) 20:16–34. doi: 10.1111/ene.12022
53. Chen, J, Cai, T, Li, Y, Chi, J, Rong, S, He, C, et al. Different iron deposition patterns in Parkinson's disease subtypes: a quantitative susceptibility mapping study. Quant Imaging Med Surg. (2020) 10:2168–76. doi: 10.21037/qims-20-285
54. Zhu, S, Wang, Y, Jiang, Y, Gu, R, Zhong, M, Jiang, X, et al. Clinical features in Parkinson's disease patients with Hyperechogenicity in substantia Nigra: a cross-sectional study. Neuropsychiatr Dis Treat. (2022) 18:1593–601. doi: 10.2147/NDT.S374370
55. Sheng, AY, Zhang, YC, Sheng, YJ, Wang, CS, Zhang, Y, Hu, H, et al. Transcranial sonography image characteristics in different Parkinson's disease subtypes. Neurol Sci. (2017) 38:1805–10. doi: 10.1007/s10072-017-3059-6
56. Bártová, P, Školoudík, D, Ressner, P, Langová, K, Herzig, R, and Kaňovský, P. Correlation between substantia nigra features detected by sonography and Parkinson disease symptoms. J Ultrasound Med. (2010) 29:37–42. doi: 10.7863/jum.2010.29.1.37
57. Jesus-Ribeiro, J, Freire, A, Sargento-Freitas, J, Sousa, M, Silva, F, Moreira, F, et al. Transcranial sonography and DaTSCAN in early stage Parkinson's disease and essential tremor. Eur Neurol. (2016) 76:252–5. doi: 10.1159/000452216
58. Toomsoo, T, Liepelt-Scarfone, I, Berg, D, Kerner, R, Pool, AH, Kadastik-Eerme, L, et al. Effect of age on substantia Nigra hyper-echogenicity in Parkinson's disease patients and healthy controls. Ultrasound Med Biol. (2019) 45:122–8. doi: 10.1016/j.ultrasmedbio.2018.09.018
59. Wen, M, Zhou, B, Chen, YH, Ma, ZL, Gou, Y, Zhang, CL, et al. Serum uric acid levels in patients with Parkinson's disease: a meta-analysis. PLoS One. (2017) 12:e0173731. doi: 10.1371/journal.pone.0173731
60. Zhong, LL, Song, YQ, Tian, XY, Cao, H, and Ju, KJ. Level of uric acid and uric acid/creatinine ratios in correlation with stage of Parkinson disease. Medicine (Baltimore). (2018) 97:e10967. doi: 10.1097/MD.0000000000010967
61. Moccia, M, Picillo, M, Erro, R, Vitale, C, Longo, K, Amboni, M, et al. Presence and progression of non-motor symptoms in relation to uric acid in de novo Parkinson's disease. Eur J Neurol. (2015) 22:93–8. doi: 10.1111/ene.12533
62. Picillo, M, Santangelo, G, Moccia, M, Erro, R, Amboni, M, Prestipino, E, et al. Serum uric acid is associated with apathy in early, drug-naive Parkinson's disease. J Neural Transm (Vienna). (2016) 123:371–7. doi: 10.1007/s00702-015-1502-5
63. Li, J, Gu, C, Zhu, M, Li, D, Chen, L, and Zhu, X. Correlations between blood lipid, serum cystatin C, and homocysteine levels in patients with Parkinson's disease. Psychogeriatrics. (2020) 20:180–8. doi: 10.1111/psyg.12483
64. Wei, Q, Wang, H, Tian, Y, Xu, F, Chen, X, and Wang, K. Reduced serum levels of triglyceride, very low density lipoprotein cholesterol and apolipoprotein B in Parkinson's disease patients. PLoS One. (2013) 8:e75743. doi: 10.1371/journal.pone.0075743
65. Sleeman, I, Aspray, T, Lawson, R, Coleman, S, Duncan, G, Khoo, TK, et al. The role of vitamin D in disease progression in early Parkinson's disease. J Parkinsons Dis. (2017) 7:669–75. doi: 10.3233/JPD-171122
66. Zhou, Z, Zhou, R, Zhang, Z, and Li, K. The association between vitamin D status, vitamin D supplementation, sunlight exposure, and Parkinson's disease: a systematic review and Meta-analysis. Med Sci Monit. (2019) 25:666–74. doi: 10.12659/MSM.912840
67. Suzuki, M, Yoshioka, M, Hashimoto, M, Murakami, M, Kawasaki, K, Noya, M, et al. 25-hydroxyvitamin D, vitamin D receptor gene polymorphisms, and severity of Parkinson's disease. Mov Disord. (2012) 27:264–71. doi: 10.1002/mds.24016
68. Sleeman, I, Lawson, RA, Yarnall, AJ, Duncan, GW, Johnston, F, Khoo, TK, et al. Urate and homocysteine: predicting motor and cognitive changes in newly diagnosed Parkinson's disease. J Parkinsons Dis. (2019) 9:351–9. doi: 10.3233/JPD-181535
69. Licking, N, Murchison, C, Cholerton, B, Zabetian, CP, Hu, SC, Montine, TJ, et al. Homocysteine and cognitive function in Parkinson's disease. Parkinsonism Relat Disord. (2017) 44:1–5. doi: 10.1016/j.parkreldis.2017.08.005
Keywords: transcranial sonography, abnormal hyperechogenicity, mechanisms, clinical relevance, Parkinson
Citation: Zhang Y-y, Jiang X-h, Zhu P-p, Zhuo W-y and Liu L-b (2024) Advancements in understanding substantia nigra hyperechogenicity via transcranial sonography in Parkinson’s disease and its clinical implications. Front. Neurol. 15:1407860. doi: 10.3389/fneur.2024.1407860
Edited by:
Gargi Mahapatra, University of California, San Diego, United StatesReviewed by:
Félix Javier Jiménez-Jiménez, Hospital Universitario del Sureste, SpainLuigi Zecca, National Research Council (CNR), Italy
Copyright © 2024 Zhang, Jiang, Zhu, Zhuo and Liu. This is an open-access article distributed under the terms of the Creative Commons Attribution License (CC BY). The use, distribution or reproduction in other forums is permitted, provided the original author(s) and the copyright owner(s) are credited and that the original publication in this journal is cited, in accordance with accepted academic practice. No use, distribution or reproduction is permitted which does not comply with these terms.
*Correspondence: Li-bin Liu, 277812928@qq.com
†These authors have contributed equally to this work and share first authorship