- 1Birmingham Medical School, University of Birmingham, Birmingham, United Kingdom
- 2Birmingham Women’s and Children’s NHS Foundation Trust, Birmingham, United Kingdom
- 3Department of Rehabilitation and Sport Sciences, Faculty of Health and Social Sciences, Bournemouth University, Bournemouth, United Kingdom
- 4Retired Physiotherapist, Cambridge, United Kingdom
- 5School of Pharmacy, Aston University, Birmingham, West Midlands, United Kingdom
Background: Children and young people (CYP) with acquired brain injury (ABI) require early and effective neurorehabilitation to improve long-term functional outcomes. Non-invasive brain stimulation (NIBS), including transcranial magnetic stimulation (TMS) and transcranial direct current stimulation (tDCS), have been used to improve motor and sensory skills for children with cerebral palsy. However, there is limited evidence supporting its use in CYP with ABI.
Objective: To systematically review the TMS and tDCS intervention effects on motor, sensory and other functional issues in CYP with ABI as reported in the literature.
Methods: A comprehensive online bibliographic databases search was performed in various databases using keywords related to NIBS and CYP with ABI. Studies that examine the effect of NIBS intervention on motor function and other functional difficulties either as a primary or secondary objective were included in this review.
Results: Fourteen studies (10 single case reports, one retrospective analysis, one case series, one randomised and one quasi-randomised controlled trial) published between 2006 and 2023 were identified. These studies examined the use of NIBS to manage motor disorders, hearing, vision, headaches, speech and language and memory issues. Seventy-six children with mild to severe ABI had received NIBS. The session frequency (3–20), duration (10–45 min) was variable, and NIBS delivered between 3 and 28 days.
Conclusion: The literature describing NIBS interventions in CYP with ABI is scarce. An insufficient number of studies, inadequate information reported in them, and small sample sizes limit the ability to conclude how effective NIBS is in improving motor function and other functional issues in this cohort. Further studies are therefore necessary to examine the therapeutic effects of NIBS to manage various functional problems in the CYP with ABI.
1 Introduction
Acquired brain injury (ABI) is the term used to describe traumatic and non-traumatic brain injuries that occur after birth and a period of typical development (1). Children and young people (CYP) with severe ABI will often have movement difficulties caused by weakness, abnormal muscle tone, poor motor control, poor concentration, poor sensory regulation, fatigue and other comorbidities (2). They may also have difficulties with speech, swallowing, and cognitive impairment. During the acute and sub-acute phase, CYP with moderate to severe ABI frequently require a period of demanding medical and rehabilitative care to optimise their long-term capabilities and quality of life through neuroplasticity (3).
Early and effective neurorehabilitation provision promotes a good long-term functional outcome for CYP with ABI (4). Recent advances in technology enable clinicians to use functional electrical stimulation, virtual reality (5), and non-invasive brain stimulation (NIBS), which includes transcranial magnetic stimulation (TMS), transcranial direct current stimulation (tDCS), transcranial alternating current stimulation (tACS), transcranial ultrasound stimulation, vagus nerve stimulation, and galvanic vestibular stimulation (6) to help improve motor skills, tone management, and sensory problems of children with central nervous system disorders (7, 8).
TMS is a non-invasive, safe treatment technique. It delivers repetitive magnetic pulses directly to specifically targeted brain areas through electromagnetic induction. Low-frequency TMS reduces cortical excitability, but high-frequency increases it (9). TMS stimulates neurons in the brain through depolarisation of myelinated axons, excites inhibitory interneurons in the stimulated brain area, and propagates along axons and synapses (10). Navigated repetitive TMS is delivered to a targeted brain area to change polarisation, influencing cortical excitability many minutes after initial stimulation (11). This will help to facilitate, inhibit or interrupt the cortical network depending upon the frequency and intensity of the stimulus, thus promoting a cortical function change through neuroplasticity (12). TMS has been used to treat children with cerebral palsy (CP) (13) and neuropsychiatric disorders, including children on the autistic spectrum and those with attention deficit hyperactivity disorder, obsessive-compulsive disorder, and tics (7). It has been shown to improve gait and upper limb functions due to changes in body structure and function, and activities in CYP with CP (14).
tDCS is another type of NIBS in which a low-amplitude current stimulates the brain by modulating neural activities (15). In tDCS, the electrodes are placed on the scalp, and the current enters the brain by passing between electrodes. The electrical activities within the brain either inhibit or facilitate neural activities to produce therapeutic effects (16). Neuronal depolarisation occurs in anodal stimulation and hyper-polarisation in cathodal stimulation by changing the membrane potential. tDCS has been used in adults and CYP with various neurological disorders including multiple sclerosis, stroke, and CP, improving motor function and fatigue, thereby improving their participation (14, 17). tDCS influences the regulatory mechanism on motor and cognitive functions through changes in the neurotransmission system, synaptic microenvironment and neural connectivity (18).
Neural plasticity (or neuroplasticity), a mechanism where the brain dynamically responds to the environment and the experience through changes in neural circuits, is critical to influencing cognitive and behavioural development (19). In ABI, the neural network is destructed or disrupted due to neuronal death, axonal tract damage, glia function, changes in the neurotransmitter system and poor cerebral perfusion. The destructed neurons and cell bodies are not replaced, and the injured axons grow slowly or minimally through regeneration. Glial responses to injury involve repairing and preserving the existing cell population through glial scar formation, and regenerating lost populations, including neurons and glia (20, 21). The molecular changes within the injured brain cells disrupt gene expression and protein phosphorylation, which are responsible for effective nerve conduction and regulation of neuron protein components. These changes are detrimental to the injured brain as they alter or interrupt normal brain development. Consequentially, children with ABI tend to have slower development or emerging long term functional problems (22). The brain’s response to environmental stimulation, a key component of neural plasticity, is limited in CYP with ABI due to the brain injury itself and the consequential impact this has on the CYP’s abilities to respond to environmental stimulation through participation. This then further restricts their recovery and development.
Interventions that promote neural plasticity in the developing brain may be advantageous in rehabilitation following ABI. It is considered that NIBS facilitates structural and functional neural plasticity through changes in regional volumes in brain cells or the formation of neural pathways through synaptogenesis, axonal or dendritic sprouting, and the creation of new neurons (23–25). If this is the case, NIBS combined with intensive rehabilitation appears to be a promising new interventional approach with broader future applications for CYP with ABI. There is, however, limited material supporting its use in CYP with ABI.
The intervention effect in rehabilitation research has been widely reported using the International Classification of Functioning, Disability and Health for Children and Youth (ICF-CY) framework (26). The ICF-CY domain consists of body structures and function, activity, participation, and contextual factors (environment and personal), which can be used to classify the level of functioning in childhood (13). This model can be applied to report the functional outcome of CYP with ABI who have impaired physical, cognitive and emotional difficulties and their impact on activity limitation and participation restriction following an intervention (13). To our knowledge, no review has examined the therapeutic effect of TMS and tDCS in CYP with ABI using the ICF-CY framework. This is a gap that should be addressed.
2 Methods
A brief method description is given here. The detailed methodology can be found in the protocol (27) and the deviations in the open-access framework.1 This review followed the Preferred Reporting Items for Systematic Reviews and Meta-Analysis extension for Scoping Reviews (PRISMA-ScR) checklist (28) (Supplementary File 1) and Arksey and O’Malley’s (29) Five stage scoping review approach.
2.1 Stage 1: identifying the research question
The purpose of this scoping review was to examine the literature relating to NIBS therapeutic effects in any functional domains such as motor, sensory and cognition. We have included only TMS and tDCS for this review to denote NIBS. The outcomes of this review were categorised according to the ICF-CY dimensions.
2.2 Stage 2: identifying relevant studies
Comprehensive online bibliographic searches were performed in Medline, Embase, Emcare, Cinahl, AMED and Cochrane Central databases by the professional librarian (DY). Results were screened for duplicate citations and remaining abstracts were uploaded in the Ryaan software (30). A copy of the full search strategy as run in Ovid Medline is provided in Appendix 1. Hand searches of citations, article references, conference proceedings identified by the searches, and trial registers checking were also carried out. The review team also contacted various TMS equipment manufacturers and distributors.
2.3 Stage 3: study selection
Two reviewers (CR and VM) scrutinised the collected titles and structured abstracts based on the following criteria. The searches were confined to CYP with ABI only (age group 2–18 years). All the subgroups of ABI, including traumatic, non-traumatic and brain tumour, were included, but children with CP were excluded. Research studies that include TMS for diagnostic purposes were excluded. No exclusion criteria were set for language or publication years, and these studies were considered if the title and abstracts had been written in English. Full articles that met the selection criteria from the above source were collected. If the full report and conference abstract were available for the same study, only the full report was included. Two reviewers decided which articles were suitable for the final review, and any disagreements were managed after discussion with the third reviewer (RG). A PRISMA flowchart was used to inform the selection process (Figure 1).
Our search yielded 1,234 titles and after removing duplicates, 774 studies were scrutinised. Fifty-four full-text reports (articles and abstracts) were collected, and 14 studies were included in the final review. Of these, 10 were single case reports, two were randomised controlled trials (RCT), one was a case series (n = 2), and one was a retrospective cohort analysis (n = 44). The total number of participants from all these studies was 76 (2–18 years). Five studies used TMS (n = 25), and nine used tDCS (n = 51).
2.4 Stage 4: charting the data
After the screening, two reviewers (CR and VM) independently extracted the data, and the details of which are given in Table 1. All the outcomes reported in the selected articles were classified under ICF-CY domains (Table 2).
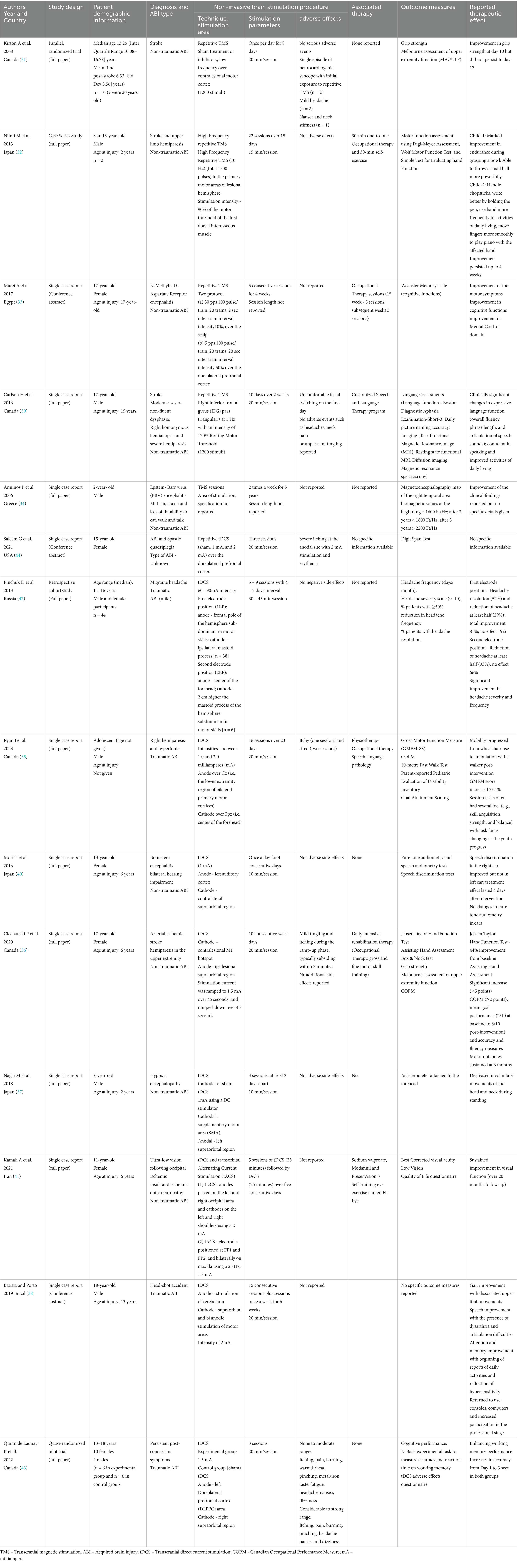
Table 1. Summary of the studies reporting TMS and TDS effects in children with acquired brain injury
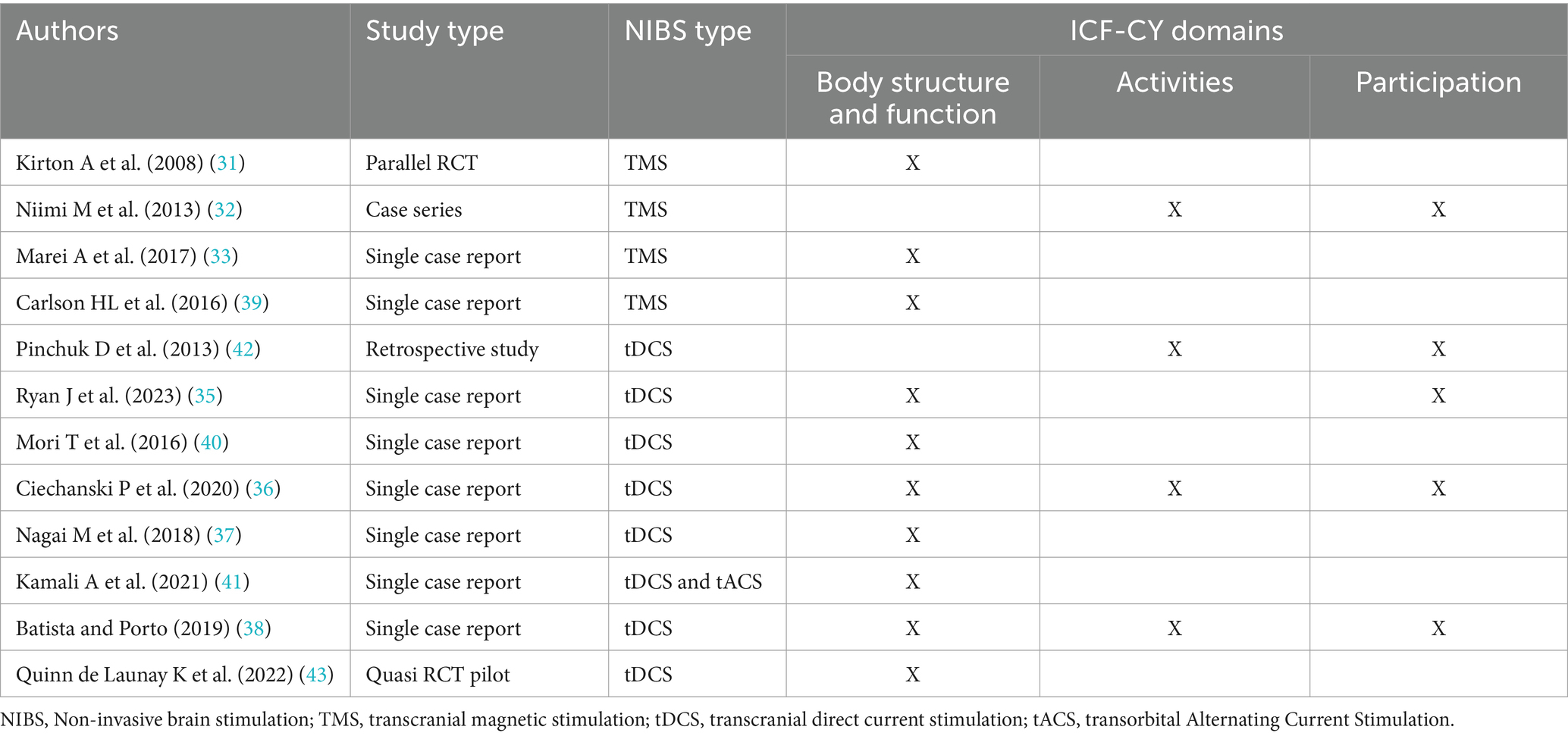
Table 2. International Classification of Functioning, Disability and Health—Children and Youth domains related outcome reported in the included studies.
3 Results
3.1 Stage 5: collating, summarising, and reporting the results
The TMS intervention had been utilised only in CYP with non-traumatic ABI, and the participants had undergone 8 to 22 TMS sessions over 8 to 28 days (15–20 min/session). The tDCS intervention had been utilised in both traumatic and non-traumatic ABI populations, and they had 3 to 16 sessions over 3 to 23 days, with the length of each session ranging between 10 and 45 min. Variable treatment parameters were used for TMS and tDCS, the details of which are given in Table 1.
3.2 Motor function
Three of the four studies reported improved motor function using TMS (31–33). Kirton et al. (31) stimulated the contralateral motor cortex with low-frequency TMS, but Niimi et al. (32) stimulated the ipsilateral primary motor cortex with high-frequency TMS. Both studies had shown improved upper limb motor functions. The reviewers could not extract specific details from the remaining two studies (33, 34). Four single case studies reported improved motor functions following tDCS intervention (35–38). Ryan et al. (35) and Ciechanski et al. (36) study participants had received intensive physiotherapy and occupational therapy along with TMS and tDCS, respectively. Therefore, it is not possible to determine the actual effectiveness of TMS and tDCS in improving motor function.
3.3 Speech and language function
Two case studies reported improved speech and language function using NIBS. TMS was used in one of the studies, and the authors attributed combined TMS with intensive speech and language therapy for the observed improvement (39). Batista and Porto (38) reported improved speech articulation using tDCS, but no specific information is available to describe the patient’s baseline speech level and how the authors measured the improvement.
3.4 Sensory (hearing and vision)
Mori et al. (40) noted improved hearing in the right ear using tDCS targeting the auditory cortex but found no change in hearing in the left ear. Kamali et al. (41) used combined tDCS and transorbital alternating current stimulation along with visual rehabilitation therapy to treat cerebral visual impairment. The authors noted improved visual acuity and suggested that tDCS is an alternative or adjunct therapeutic approach to managing visual impairment.
3.5 Headache
Pinchuk et al. (42) retrospective study examined the effectiveness of tDCS in post-lesional migraine headaches in adults and children. In this review, only data related to CYP was retrieved. The authors used two electrode positions (1EP and 2EP—description in Table 1) to treat headaches. They found that 52% of children in the 1EP group had entirely resolved headaches, and 28% of children’s headaches had reduced by at least 50% after 4.5 months. The authors noted no effect of tDCS for the remaining 20% of participants. This study observed a marked reduction in headache, tiredness, and irritability, and the children had better sleep following tDCS treatment.
3.6 Cognitive function
In a single case report, Carlson et al. (39) treated cognitive function with TMS, but no specific information is available to inform the baseline and the level of improvement made. Similarly, Batista and Porto (38) reported improved attention and memory following tDCS intervention, but no specific details are available. Quinn de Launay et al. (43) examined the effect of tDCS on the adolescent population’s cognitive function and noted improved working memory through an interaction with a consolidation mechanism.
3.7 ICF-CY domains
The included studies did not explicitly report changes under ICF-CY domains, and the reviewers inferred the appropriate domains through discussion and consensus. Following this process, only two studies reported changes in body structure and function, activities, and participation in ICF-CY domains (36, 38). Most studies reported changes in the body structure and function (31, 33, 35–41, 43); two reported changes in the activities (32, 42), and three studies in the participation domains (32, 33, 35). The reviewers could not comment on the ICF-CY components for a few of the included studies (34, 44) due to the limited availability of information.
4 Discussion
This scoping review aims to understand the effectiveness of NIBS in CYP with ABI. Feasibility and exploratory studies suggest that motor function in this population does improve with the use of NIBS. However, it is not possible to determine the actual effectiveness of NIBS in this population due to the variation in study types, small sample size, and the heterogeneity of the patient groups in terms of age of insult, type of ABI, variations in treatment parameters and the outcome measures used. Thus, the findings require cautious interpretation. The authors hypothesised different possible neurophysiological mechanisms for improvements in motor functions, for example, Kirton et al. (31) suggested that the improved motor function was due to TMS influence on the normal ipsilateral hemisphere, creating an imbalance of interhemispheric inhibition and motor cortex inhibition. Nagai et al. (37) noted improved motor control through decreased involuntary movement and proposed that tDCS inhibits the supplementary motor area through the modulation of abnormal excitation of the corticobasal ganglia network, thus potentially influencing the modulation of the corticospinal tract (37). Ryan et al. (35) and Ciechanski et al. (36) reported no specific mechanism apart from neural plasticity for improved motor function using tDCS. The remaining studies (32–34, 38–44) did not suggest any specific mechanism for improvement.
NIBS has been shown to be effective in treating expressive language difficulties, cognition issues and sensory problems, including hearing and vision. However, for similar reasons already discussed, it is impossible to determine the actual effectiveness of NIBS in this area and no specific neurophysiological mechanisms for improvements seen were suggested.
All the studies except one, reported change related to ICF-CY’s body structure and function domain. None of the studies reported how the CYP’s activities and participation changed after TMS or tDCS intervention. For example, Pinchuk et al. study reported a marked reduction in post-lesional migraine headaches, but no specific outcome measures were used to indicate how participation and activities had improved and the associated changes in quality of life (42). It should be noted that the absence of findings related to ICF-CY domains in the included studies is not necessarily linked to the use of NIBS but rather to the lack of its utilisation in guiding rehabilitation outcomes. The reviewers suggest incorporating all ICF-CY domains will help to demonstrate functional improvement in future studies.
This scoping review found that the stimulation time was limited to an average of 20 min in most studies. This was presumably due to convenience and tolerance for CYP being static when receiving NIBS. Pinchuck et al. study used 30–45 min of tDCS stimulation, and they considered the increased exposure time led to a greater stimulation intensity which produced the desired therapeutic response (42). The treatment frequency, intensity, and duration differed for the remaining studies, and in the majority of studies, the rationale for the chosen treatment parameters was unclear. Therefore, deciding the treatment parameters through consensus and different co-production methods with all stakeholders (45), including parents, CYP, neurophysiologists, therapists, and neurologists, should be considered for future studies.
Many studies considered NIBS a useful adjunct, but it was hard to identify the effectiveness due to other confounding factors, including intensive therapy sessions, which may have resulted in neural plasticity. Evidence from children with CP indicates that NIBS promotes rehabilitation effectiveness when combined with intensive interventions such as constraint-induced movement therapy, hand-arm bimanual intensive therapy, treadmill walking, and gait training (46). To induce neural plasticity, repetitive training in conjunction with NIBS is required, and future studies need to consider this to optimise neurorehabilitation outcomes.
Both RCTs included in this review showed that applying NIBS is feasible in the ABI population during the chronic stage (31, 43). Ryan et al. (47) were unsuccessful in recruiting sufficient participants for their feasibility RCT study during the subacute stage of ABI rehabilitation. The authors suggested improved eligibility and retention by approaching children after discharge and providing tDCS on an outpatient basis. The findings of the above studies suggested that acceptability and willingness to try NIBS may be more effective during the later stage of rehabilitation when all other means of rehabilitation have been tried during and after the subacute stage.
Some studies noted minimal side effects (31, 35, 36, 39, 43) and no significant adverse effects were reported. All participants tolerated NIBS intervention well. Our review agrees with other studies that NIBS appears safe for children (48), and this can be considered as an intervention in future studies. Despite having apparently good safety profiles, our review has highlighted that the use of NIBS in the CYP with ABI population is understudied.
There are recognised anatomical and physiological differences between CYP and adults. Skull thickness (46), brain-scalp distances and corticospinal fluid levels are smaller in young children than in adults (49). Therefore, the average current intensity required in the cortex to produce voltage and dosimetry changes is smaller in children than in adults. Due to smaller head sizes, the difference between the anatomical landmarks varies up to 50% in children compared to the adult population (49). Therefore, a smaller TMS coil size is required to stimulate children, and the tDCS montage size needs to be smaller to avoid the shunting effect. The included studies did not indicate whether these differences were considered when stimulating the CYP with ABI using NIBS, and these should be considered in future studies.
It is not currently possible to determine if NIBS has a different therapeutic effect in different types of ABI. For instance, our search found no studies on brain tumour-related ABI, and it may be possible that no studies have been done in this population.
Similarly, it is not currently possible to determine whether NIBS is effective in treating specific symptoms of ABI. For instance, fatigue is a common symptom in CYP with ABI, and it is linked with sleep disturbance, emotional regulation, memory, attention deficiency, and poor academic performance (50, 51). However, there are no studies available which examine NIBS effectiveness in fatigue management.
Given the significant impact of ABI on CYP and their families, more appropriately designed research is required to investigate NIBS effectiveness in the management of various functional difficulties in CYP with ABI.
4.1 Deviation from protocol
This scoping review’s initial focus was to assess the effect of TMS primarily on motor function, but the review team subsequently amended this to include tDCS by taking advantage of the already included tDCS-related terms used in the database search, and included other functional difficulties of CYP with ABI, to develop a wider understanding of how NIBS may benefit CYP with ABI. A hand search was planned, but no journals fulfilled the criteria to be considered. Therefore, this was not conducted. Specific details about the NIBS equipment used in the reviewed studies were not included due to the limited availability of information. Comparator/control parameters are not applicable for most of the studies. Therefore, this information was not reported here. The review team had attempted to contact the study authors to verify the collected data through triangulation, which was another deviation from the protocol.
This review has certain limitations. TMS manufacturers were contacted to seek studies using their equipment, but this was not the case for tDCS manufacturers. The review team did not assess the quality of the evidence due to variations in the included study types. The review team could not contact three of the corresponding authors of the conference abstracts to seek further information; therefore, there was no scope to triangulate their findings. It is therefore possible that some of the potential studies that have not been published were excluded. The lack of availability of sufficient data in the studies considered did not allow an in-depth analysis on an individual patient basis.
5 Conclusion
The literature describing NIBS interventions in CYP with ABI is scarce. Most available studies have reported positive therapeutic effects, mainly in body structure and functions and activity domains of the ICF-CY. A few studies reported minimal transient adverse effects, but these were tolerated well. An insufficient number of studies, inadequate information reported in them, and small sample sizes limit the ability to conclude how effective NIBS is in improving motor function and other functional parameters in CYP with ABI. Further studies are needed in this area.
Author contributions
CR: Conceptualization, Data curation, Formal analysis, Methodology, Project administration, Software, Validation, Writing – original draft, Writing – review & editing. VM: Conceptualization, Data curation, Formal analysis, Methodology, Software, Validation, Writing – original draft, Writing – review & editing. DY: Methodology, Resources, Writing – review & editing. PB: Writing – review & editing. JP: Conceptualization, Writing – review & editing. RG: Writing – review & editing.
Funding
The author(s) declare that no financial support was received for the research, authorship, and/or publication of this article.
Conflict of interest
The authors declare that the research was conducted in the absence of any commercial or financial relationships that could be construed as a potential conflict of interest.
Publisher’s note
All claims expressed in this article are solely those of the authors and do not necessarily represent those of their affiliated organizations, or those of the publisher, the editors and the reviewers. Any product that may be evaluated in this article, or claim that may be made by its manufacturer, is not guaranteed or endorsed by the publisher.
Supplementary material
The Supplementary material for this article can be found online at: https://www.frontiersin.org/articles/10.3389/fneur.2024.1388718/full#supplementary-material
Footnotes
References
1. Wales, L, Davis, K, Kelly, G, and Lynott, H. Long term participation outcomes for severe acquired brain injury in childhood—an expanded scoping review. Dev Neurorehabil. (2021) 24:379–87. doi: 10.1080/17518423.2021.1886191
2. Hypher, RE, Brandt, AE, Risnes, K, Rø, TB, Skovlund, E, Andersson, S, et al. Paediatric goal management training in patients with acquired brain injury: study protocol for a randomised controlled trial. BMJ Open. (2019) 9:e029273. doi: 10.1136/bmjopen-2019-029273
3. McKinlay, A, Linden, M, DePompei, R, Aaro Jonsson, C, Anderson, V, Braga, L, et al. Service provision for children and young people with acquired brain injury: practice recommendations. Brain Inj. (2016) 30:1656–64. doi: 10.1080/02699052.2016.1201592
4. Shen, J, Johnson, S, Chen, C, and Xiang, H. Virtual reality for pediatric traumatic brain injury rehabilitation: a systematic review. Am J Lifestyle Med. (2020) 14:6–15. doi: 10.1177/1559827618756588
5. Rathinam, C, Mohan, V, Peirson, J, Skinner, J, Nethaji, KS, and Kuhn, I. Effectiveness of virtual reality in the treatment of hand function in children with cerebral palsy: a systematic review. J Hand Ther. (2018) 32:426–434.e1. doi: 10.1016/j.jht.2018.01.006
6. Bhattacharya, A, Mrudula, K, Sreepada, SS, Sathyaprabha, TN, Pal, PK, Chen, R, et al. An overview of noninvasive brain stimulation: basic principles and clinical applications. Can J Neurol Sci. (2022) 49:479–92. doi: 10.1017/cjn.2021.158
7. Bejenaru, AM, and Malhi, NK. Use of repetitive Transcranial magnetic stimulation in child psychiatry. Innov Clin Neurosci. (2022) 19:11–22.
8. Dobney, DM, Miller, MB, and Tufts, E. Non-pharmacological rehabilitation interventions for concussion in children: a scoping review. Disabil Rehabil. (2019) 41:727–39. doi: 10.1080/09638288.2017.1400595
9. Kubis, N . Non-invasive brain stimulation to enhance post-stroke recovery. Front Neural Circuits. (2016) 10:56. doi: 10.3389/fncir.2016.00056
10. Siebner, HR, Funke, K, Aberra, AS, Antal, A, Bestmann, S, Chen, R, et al. Transcranial magnetic stimulation of the brain: what is stimulated?—a consensus and critical position paper. Clin Neurophysiol. (2022) 140:59–97. doi: 10.1016/j.clinph.2022.04.022
11. Schambra, HM . Repetitive Transcranial magnetic stimulation for upper extremity motor recovery: does it help? Curr Neurol Neurosci Rep. (2018) 18:97. doi: 10.1007/s11910-018-0913-8
12. Lefaucheur, J-P . Transcranial magnetic stimulation In: KH Levin and PBT-H Chauvel, editors. Clinical neurophysiology: Basis and technical aspects : Elsevier (2019). 559–80.
13. Gmelig Meyling, C, Verschuren, O, Rentinck, IR, Engelbert, RHH, and Gorter, JW. Physical rehabilitation interventions in children with acquired brain injury: a scoping review. Dev Med Child Neurol. (2022) 64:40–8. doi: 10.1111/dmcn.14997
14. Elbanna, ST, Elshennawy, S, and Ayad, MN. Noninvasive brain stimulation for rehabilitation of pediatric motor disorders following brain injury: systematic review of randomized controlled trials. Arch Phys Med Rehabil. (2019) 100:1945–63. doi: 10.1016/j.apmr.2019.04.009
15. Yamada, Y, and Sumiyoshi, T. Neurobiological mechanisms of Transcranial direct current stimulation for psychiatric disorders; neurophysiological, chemical, and anatomical considerations. Front Hum Neurosci. (2021) 15:631838. doi: 10.3389/fnhum.2021.631838
16. Thair, H, Holloway, AL, Newport, R, and Smith, AD. Transcranial direct current stimulation (tDCS): a Beginner’s guide for design and implementation. Front Neurosci. (2017) 11:641. doi: 10.3389/fnins.2017.00641
17. Jagadish, A, Shankaranarayana, AM, Natarajan, M, and Solomon, JM. Transcranial direct current stimulation for fatigue in neurological conditions: a systematic scoping review. Physiother Res Int J Res Clin Phys Ther. (2023) 29:e2054. doi: 10.1002/pri.2054
18. Dadgar, H, Majidi, H, and Aghaei, S. Biological and neurobiological mechanisms of Transcranial direct current stimulation. Iran J Psychiatry. (2022) 17:350–5. doi: 10.18502/ijps.v17i3.9735
19. Fandakova, Y, and Hartley, CA. Mechanisms of learning and plasticity in childhood and adolescence. Dev Cogn Neurosci. (2020) 42:100764. doi: 10.1016/j.dcn.2020.100764
20. Nicaise, AM, D’Angelo, A, Ionescu, R-B, Krzak, G, Willis, CM, and Pluchino, S. The role of neural stem cells in regulating glial scar formation and repair. Cell Tissue Res. (2022) 387:399–414. doi: 10.1007/s00441-021-03554-0
21. Gallo, V, and Deneen, B. Glial development: the crossroads of regeneration and repair in the CNS. Neuron. (2014) 83:283–308. doi: 10.1016/j.neuron.2014.06.010
22. Anderson, V, Spencer-Smith, M, and Wood, A. Do children really recover better? Neurobehavioural plasticity after early brain insult. Brain. (2011) 134:2197–221. doi: 10.1093/brain/awr103
23. Dan, B . Neuroscience underlying rehabilitation: what is neuroplasticity? Dev Med Child Neurol. (2019) 61:1240. doi: 10.1111/dmcn.14341
24. King, ES, and Tang, AD. Intrinsic plasticity mechanisms of repetitive Transcranial magnetic stimulation. Neuroscientist. (2022) 30:260–74. doi: 10.1177/10738584221118262
25. Johnston, MV . Plasticity in the developing brain: implications for rehabilitation. Dev Disabil Res Rev. (2009) 15:94–101. doi: 10.1002/ddrr.64
26. Martinuzzi, A, De Polo, G, Bortolot, S, and Pradal, M. Pediatric neurorehabilitation and the ICF. NeuroRehabilitation. (2015) 36:31–6. doi: 10.3233/NRE-141188
27. Rathinam, C, Mohan, V, Bill, P, Yates, D, Gupta, R, and Peirson, J. Impact of transcranial magnetic stimulation on motor function in children with acquired brain injury: a scoping review protocol. BMJ Paediatr Open. (2023) 7:e001885. doi: 10.1136/bmjpo-2023-001885
28. Tricco, AC, Lillie, E, Zarin, W, O’Brien, KK, Colquhoun, H, Levac, D, et al. PRISMA extension for scoping reviews (PRISMA-ScR): checklist and explanation. Ann Intern Med. (2018) 169:467–73. doi: 10.7326/M18-0850
29. Arksey, H, and O’Malley, L. Scoping studies: towards a methodological framework. Int J Soc Res Methodol. (2005) 8:19–32. doi: 10.1080/1364557032000119616
30. Ouzzani, M, Hammady, H, Fedorowicz, Z, and Elmagarmid, A. Rayyan-a web and mobile app for systematic reviews. Syst Rev. (2016) 5:210. doi: 10.1186/s13643-016-0384-4
31. Kirton, A, Chen, R, Friefeld, S, Gunraj, C, Pontigon, A-M, and Deveber, G. Contralesional repetitive transcranial magnetic stimulation for chronic hemiparesis in subcortical paediatric stroke: a randomised trial. Lancet Neurol. (2008) 7:507–13. doi: 10.1016/S1474-4422(08)70096-6
32. Niimi, M, Takekawa, T, Ito, H, Kameda, Y, Kakuda, W, and Abo, M. Therapeutic application of high-frequency rtms combined with intensive occupational therapy for pediatric stroke patients with upper limb hemiparesis: a case series study. In: Neurorehabilitation neural Repair Conference 7th world Congr NeuroRehabilitation, WCNR 2012 Melbourne, VIC Australia Publishing. (2012);26:735–736.
33. Marei, A, Rashed, H, and Sabra, F. Repetitive transcranial magnetic stimulation (rTMS) and occupational therapy (OT) can improve cognitive deficits due to anti n-methyl-d-aspartate receptor (NMDA) encephalitis in 4 weeks: case study. In: Brain Stimulation 2nd International Brain Stimulation Conference Spain. (2017);10:518–519.
34. Anninos, P, Kotini, A, Tamiolakis, D, and Tsagas, N. Transcranial magnetic stimulation. A case report and review of the literature. Acta Neurol Belg. (2006) 106:26–30.
35. Ryan, JL, Beal, DS, Levac, DE, Fehlings, DL, and Wright, FV. Integrating Transcranial direct current stimulation into an existing inpatient physiotherapy program to enhance motor learning in an adolescent with traumatic brain injury: a case report. Phys Occup Ther Pediatr. (2023) 43:463–81. doi: 10.1080/01942638.2022.2163214
36. Ciechanski, P, Carlson, HL, Herrero, M, Lane, C, FP, MM, and Kirton, A. A case of Transcranial direct-current stimulation for childhood stroke hemiparesis: a brief report [internet]. Dev Neurorehabil. (2020) 23:133–6. doi: 10.1080/17518423.2019.1655678
37. Nagai, M, Tanaka, N, Oouchida, Y, and Izumi, SI. Effect of cathodal Transcranial direct current stimulation on a child with involuntary movement after hypoxic encephalopathy. Case Rep Med. (2018) 2018:1–5. doi: 10.1155/2018/8934253
38. Batista, J, and Porto, H. Abstract #131: tDCS in post firearm brain injury—case report. Brain Stimul. (2019) 12:e45. doi: 10.1016/j.brs.2018.12.138
39. Carlson, HL, Jadavji, Z, Mineyko, A, Damji, O, Hodge, J, Saunders, J, et al. Treatment of dysphasia with rTMS and language therapy after childhood stroke: multimodal imaging of plastic change. Brain Lang. (2016) 159:23–34. doi: 10.1016/j.bandl.2016.05.008
40. Mori, T, Takeuchi, N, Suzuki, S, Miki, M, Kawase, T, and Izumi, S-I. Anodal transcranial direct current stimulation over the auditory cortex improved hearing impairment in a patient with brainstem encephalitis. J Int Med Res. (2016) 44:760–4. doi: 10.1177/0300060516630843
41. Kamali, AM, Gholamzadeh, MJ, Mousavi, SZ, Gharamaleki, MV, and Nami, M. Improved visual function in a case of ultra-low vision following ischemic encephalopathy using Transcranial electrical stimulation; a brief report. Dev Neurorehabil. (2021) 24:68–72. doi: 10.1080/17518423.2020.1839978
42. Pinchuk, D, Pinchuk, O, Sirbiladze, K, and Shugar, O. Clinical effectiveness of primary and secondary headache treatment by transcranial direct current stimulation. Front Neurol. (2013) 4:25. doi: 10.3389/fneur.2013.00025
43. Quinn de Launay, K, Cheung, ST, Riggs, L, Reed, N, and Beal, DS. The effect of transcranial direct current stimulation on cognitive performance in youth with persistent cognitive symptoms following concussion: a controlled pilot study. Brain Inj. (2022) 36:39–51. doi: 10.1080/02699052.2022.2034179
44. Saleem, G, Slomine, B, and Suskauer, S. Transcranial direct current stimulation feasibility challenges in youth with severe brain injury: a case report. In: Neuromodulation Conference North American Neuromodulation Society Meeting 2021 Virtual. (2021);24:e159.
45. Smith, H, Budworth, L, Grindey, C, Hague, I, Hamer, N, Kislov, R, et al. Co-production practice and future research priorities in United Kingdom-funded applied health research: a scoping review. Health Res Policy Syst. (2022) 20:36. doi: 10.1186/s12961-022-00838-x
46. O’Leary, GH, Jenkins, DD, Coker-Bolt, P, George, MS, Kautz, S, Bikson, M, et al. From adults to pediatrics: a review noninvasive brain stimulation (NIBS) to facilitate recovery from brain injury. Prog Brain Res. (2021) 264:287–322. doi: 10.1016/bs.pbr.2021.01.019
47. Ryan, J, Beal, D, Fehlings, D, Levac, D, and Wright, V. The feasibility of Transcranial direct current stimulation as an adjunct to inpatient physiotherapy in children and youth with moderate to severe acquired brain injury. J Head Trauma Rehabil. (2022) 37:E544–5. doi: 10.3138/ptc-2023-0015
48. Zewdie, E, Ciechanski, P, Kuo, HC, Giuffre, A, Kahl, C, King, R, et al. Safety and tolerability of transcranial magnetic and direct current stimulation in children: prospective single center evidence from 3.5 million stimulations. Brain Stimul. (2020) 13:565–75. doi: 10.1016/j.brs.2019.12.025
49. Beauchamp, MS, Beurlot, MR, Fava, E, Nath, AR, Parikh, NA, Saad, ZS, et al. The developmental trajectory of brain-scalp distance from birth through childhood: implications for functional neuroimaging. PLoS One. (2011) 6:e24981. doi: 10.1371/journal.pone.0024981
50. Ortelli, P, Ferrazzoli, D, Sebastianelli, L, Engl, M, Romanello, R, Nardone, R, et al. Neuropsychological and neurophysiological correlates of fatigue in post-acute patients with neurological manifestations of COVID-19: insights into a challenging symptom. J Neurol Sci. (2021) 420:117271. doi: 10.1016/j.jns.2020.117271
51. Bogdanov, S, Brookes, N, Epps, A, Naismith, SL, Teng, A, and Lah, S. Fatigue in children with moderate or severe traumatic brain injury compared with children with orthopedic injury: characteristics and associated factors. J Head Trauma Rehabil. (2021) 36:E108–17. doi: 10.1097/HTR.0000000000000585
Keywords: acquired brain injury, children and young people, transcranial magnetic stimulation, transcranial direct current stimulation, motor function, TMS, tDCS
Citation: Rathinam C, Mohan V, Yates D, Bill P, Peirson J and Gupta R (2024) Effect of non-invasive brain stimulation in children with acquired brain injury—a scoping review. Front. Neurol. 15:1388718. doi: 10.3389/fneur.2024.1388718
Edited by:
Robson Luis Amorim, University of São Paulo, BrazilReviewed by:
Perin Cecilia, University of Milano-Bicocca, ItalyAlessio Faralli, University of Florence, Italy
Rui-zhe Zheng, Fudan University, China
Copyright © 2024 Rathinam, Mohan, Yates, Bill, Peirson and Gupta. This is an open-access article distributed under the terms of the Creative Commons Attribution License (CC BY). The use, distribution or reproduction in other forums is permitted, provided the original author(s) and the copyright owner(s) are credited and that the original publication in this journal is cited, in accordance with accepted academic practice. No use, distribution or reproduction is permitted which does not comply with these terms.
*Correspondence: Chandrasekar Rathinam, c.rathinam@bham.ac.uk