- 1Jiangxi Medical College, Nanchang University, Nanchang, China
- 2Jiangxi Provincial People's Hospital, The First Affiliated Hospital of Nanchang Medical College, Nanchang, China
- 3Department of Ophthalmology, Jiangxi Provincial People’s Hospital, The First Affiliated Hospital of Nanchang Medical College, Nanchang, China
Objective: Bronchial Asthma (BA) is a common chronic respiratory disease worldwide. Earlier research has demonstrated abnormal functional connectivity (FC) in multiple cognition-related cortices in asthma patients. The thalamus (Thal) serves as a relay center for transmitting sensory signals, yet the modifications in the thalamic FC among individuals with asthma remain uncertain. This research employed the resting-state functional connectivity (rsFC) approach to explore alterations in thalamic functional connectivity among individuals with BA.
Patients and methods: After excluding participants who did not meet the criteria, this study finally included 31 patients with BA, with a gender distribution of 16 males and 15 females. Subsequently, we recruited 31 healthy control participants (HC) matched for age, gender, and educational background. All participants underwent the Montreal Cognitive Assessment (MoCA) and the Hamilton Depression Rating Scale (HAMD) assessment. Following this, both groups underwent head magnetic resonance imaging scans, and resting-state functional magnetic resonance imaging (rs-fMRI) data was collected. Based on the AAL (Automated Anatomical Labeling) template, the bilateral thalamic regions were used as seed points (ROI) for subsequent rsFC research. Pearson correlation analysis was used to explore the relationship between thalamic functional connectivity and neuropsychological scales in both groups. After controlling for potential confounding factors such as age, gender, intelligence, and emotional level, a two-sample t-test was further used to explore differences in thalamic functional connectivity between the two groups of participants.
Result: Compared to the HC group, the BA group demonstrated heightened functional connectivity (FC) between the left thalamus and the left cerebellar posterior lobe (CPL), left postcentral gyrus (PCG), and right superior frontal gyrus (SFG). Concurrently, there was a decrease in FC with both the Lentiform Nucleus (LN) and the left corpus callosum (CC). Performing FC analysis with the right thalamus as the Region of Interest (ROI) revealed an increase in FC between the right thalamus and the right SFG as well as the left CPL. Conversely, a decrease in FC was observed between the right thalamus and the right LN as well as the left CC.
Conclusion: In our study, we have verified the presence of aberrant FC patterns in the thalamus of BA patients. When compared to HCs, BA patients exhibit aberrant alterations in FC between the thalamus and various brain areas connected to vision, hearing, emotional regulation, cognitive control, somatic sensations, and wakefulness. This provides further confirmation of the substantial role played by the thalamus in the advancement of BA.
Introduction
Asthma is a condition marked by recurrent wheezing, breathlessness, and coughing. Over 400 million individuals across the globe are affected by asthma. Currently, asthma affects 6.3% of the youth population in China (1). Previous research on the pathological mechanisms of asthma has primarily focused on airway inflammation and airway remodeling (2). However, peripheral inflammatory substances can also impact the central nervous system via various pathways (3). In a prior study, brain MRI scans revealed abnormal changes in the brains of asthma patients (4). Furthermore, markers associated with central nervous degeneration and neuroinflammation have been detected in the blood of asthma patients, suggesting potential consequences of asthma on the central nervous system (5).
The mechanisms through which asthma affects the central nervous system are not yet fully understood. However, it is currently believed that the long-term chronic hypoxia induced by asthma may initiate and exacerbate certain pathophysiological processes, such as reduced perfusion, endothelial dysfunction, and neuroinflammation. In addition to these factors, chemotactic factors produced by airway inflammation can directly traverse the blood–brain barrier, leading to increased release of reactive oxygen species by microglial cells, inducing neuronal apoptosis (6). A recent study found that allergens can reduce communication activity between the amygdala and the respiratory control cortex, exacerbating respiratory difficulties (7). Furthermore, asthma exhibits an overall suppression of the HPA axis, and long-term corticosteroid use also inhibits the HPA axis. This alteration is associated with a reduction in hippocampal volume, thereby impacting working memory (8). Additionally, asthma-related nocturnal sleep disturbances are associated with poorer cognitive function (9). Inherent difficulties in respiratory regulation, cognitive deficits, and emotional control disturbances all contribute to the duration and severity of asthma attacks.
When neuronal metabolic activity is ongoing, local changes in cerebral oxygen content and blood supply occur. fMRI is capable of detecting magnetic field fluctuations resulting from the mismatch between local oxygen consumption and cerebral blood flow in various brain areas, thereby reflecting the metabolic activity of brain areas. Rs-fMRI has increasingly been employed to investigate brain activity alterations in BA patients. Li et al. (10) found abnormal activity in the angular gyrus, prefrontal cortex, temporal gyrus, superior frontal gyrus, and occipital lobe when using fMRI to analyze brain region activity and neural networks in asthma patients. Ritz et al. (11) conducted an fMRI study on individuals with poorly controlled asthma and found increased activation in their dorsal anterior cingulate cortex (dACC). A study revealed abnormalities in these metrics in the frontal and superior lobes of the brains of asthmatic children, closely associated with attention deficits (12). Most of these studies focus on regional activity in the asthmatic brain cortex or changes in connectivity at the network level. In previous research, we found a substantial reduction in bilateral thalamic connectivity in asthma individuals (13). On the other hand, recent research has revealed an inverse relationship between the length of time an individual has had asthma, the level of asthma management, and the frequency of asthma attacks and the volume of the thalamus (14).
The thalamus is often described as an intermediary station for sensory information, playing a pivotal role in not only transmitting sensory input but also participating in cognitive processes. Additionally, it exerts significant control over visceral functions, motor coordination, and the maintenance of cerebral arousal (15). The thalamus acts as a gatekeeper for information directed to the cerebral cortex, selectively enhancing or inhibiting the activation of specific information pathways based on the behavioral state (16). With extensive and global connections to numerous brain regions, the thalamus and its constituent nuclei are likely major hubs within multiple brain networks (17). Consequently, conducting a comprehensive investigation into the functional connections between the thalamus and various other cerebral areas is deemed essential. The rs-FC primarily assesses the statistical associations among signals throughout the entire brain and particular brain areas, demonstrating the potential for exploring how different brain regions coordinate their operations. In this study, we will be the first to employ rs-FC to uncover evidence of abnormal functional connections within the thalamus in asthma patients. We hypothesize that thalamic FC differs from that of the healthy population, and this difference may be implicated in potential neurobiological mechanisms underlying cognitive impairments and emotional dysregulation in asthma patients.
Materials and methods
Clinical data
The criteria for selecting asthma subjects are as follows: (1) Adults under the age of 60; (2) presence of recurrent wheezing, with the forced expiratory volume in 1 s (FEV1) falling between 45 and 80% of the predicted normal value; (3) a positive bronchodilator test (FEV1 reversibility of at least 12% and 200 mL after the administration of 200 to 400 μg of salbutamol sulfate); and (4) individuals in a non-acute phase of asthma.
The criteria that are not suitable for the research object are as follows: (1) The presence of other respiratory system diseases; (2) Psychiatric disorders or other chronic diseases that may affect brain structure and function; (3) Drug dependence or adverse habits; (4) Lack of necessary MRI data or clinical assessment information; (5) Maximum head displacement exceeding 2.5 mm in the x, y, and/or z directions, or angle rotation exceeding 2.5 degrees around any axis; (6) Contraindications related to MRI examinations; (7) Absence of claustrophobia and the ability to tolerate MRI examinations. In the end, a total of 31 patients diagnosed with BA participated, comprising 16 males and 15 females. Simultaneously, We selected 31 HCs (16males and 15 females) with basic information matching.
The situations in HCs that are inappropriate for participation are as follows: (1) Presence of asthma or other diseases; (2) Brain or psychological disorders; (3) Completing an MRI examination carries risks; (4) Maximum head displacement exceeding 2.5 mm in the x, y, and/or z directions, or angle rotation exceeding 2.5 degrees around any axis; (5) Incomplete relevant data.
Neuropsychological assessment
To ensure the reliability of the measured results, we employed the Montreal Cognitive Assessment (MoCA) scale as a cognitive function measurement tool. The maximum score on the scale is 30 points, with an additional point given if the participant has an education level of ≤12 years. Scores between 18 and 26 indicate mild cognitive impairment (MCI) (18). Furthermore, we utilized the 17-item Hamilton Depression Rating Scale (HAMD-17) to evaluate the emotional status of the two groups of participants (19). The test results from this scale will provide a basis for analyzing cognitive and emotional differences among the subjects.
fMRI data acquisition
Utilizing an 8-channel phased-array head coil, we acquired MRI data employing the Trio 3-Tesla MR scanner from Siemens, Germany. Participants were instructed to remain awake but refrain from engaging in any cognitive activities. Head motion was minimized using a foam cushion, and noise interference was mitigated through the use of earplugs. The parameters for obtaining fMRI images are shown in Table 1.
Data preprocessing
To begin, we validated the quality of the MRI data using the MRIcro software package (Montreal Neurological Institute, Canada) and excluded those with poor quality. Subsequently, we employed the DPABI V5.0 toolbox for brain imaging data processing and analysis to preprocess the fMRI data. This toolbox operates on the MatLab 2018b platform (Mathworks, Natick, MA, United States). We perform data preprocessing, including the following steps: (1) Converted the DICOM (Digital Imaging and Communications in Medicine) format to the NIFTI (Neuroimaging Informatics Technology Initiative) format (2) Discarding the first 10 time records (3) Correction of temporal differences between slices (4) Correction of head motion exceeding 2 millimeters or 2 degrees (5) Alignment and division of functional and structural images, normalized to the standard space, with Resampling to 3 mm x 3 mm x 3 mm Voxel Units (6) An isotropic Gaussian kernel with a Full-Width at Half-Maximum (FWHM) value of 6 mm was applied for spatial smoothing. Temporal filtering in the frequency range of 0.01–0.08 Hz was used to address linear drift (7) The regression covariates included the Friston-24 head motion parameters, as well as white matter, cerebrospinal fluid, and scale scores.
Functional connectivity analysis based on seed regions
To begin, we adopted a cubic size of 6 × 6 × 6 mm for the bilateral thalamus, designated as our region of interest (ROI). This choice ensured thorough coverage of critical thalamic regions while mitigating biases associated with oversized or undersized ROIs. Next, we established the thalamic ROI in the standardized MNI (Montreal Neurological Institute) space using the AAL (Automated Anatomical Labeling) template. This template, offering detailed anatomical partitioning of the brain, facilitated the creation of a uniform reference framework for consistent cross-individual comparisons. Subsequently, we employed affine transformation and non-linear transformation via SPM (Statistical Parametric Mapping) software to accurately map the ROI to individual subject space. This step was essential for accommodating anatomical variability across individuals and ensuring the precision of functional connectivity analyses. Once the thalamic ROI was accurately defined and mapped, we computed Pearson correlation coefficients between the bilateral thalamus and every voxel in the entire brain. These correlation coefficients underwent Fisher’s Z transformation to approximate a normal distribution. The resulting Z values represented the strength of functional connectivity, offering a quantitative assessment of the functional interactions between the thalamus and the broader brain network.
Statistical analysis
We conducted statistical analyses using SPSS 27 software, and continuous data were presented as means ± standard deviations. Group comparisons for age, level of education, and BMI were performed using independent sample t-tests. Additionally, a one-way ANOVA was conducted to compare the scores of psychological assessments and cognitive functions, adjusting for the effects of age, gender, and educational level. After controlling for potential confounders such as age, gender, intelligence, and emotional level, a two-sample t-test was further employed to explore the differences in thalamic functional connectivity between the two groups of participants. Subsequently, the DPABI software was employed to analyze intergroup differences while considering age, education level, and head motion as covariates. Utilizing AlphaSim for correction, p < 0.05. Finally, the xjView software was utilized to report the locations of brain regions with significant functional connectivity.
Results
Demographic statistics and clinical scales
In our investigation, there were no notable disparities in terms of age, gender, body weight, or BMI between the BA patients and HCs (p > 0.05). The findings are displayed as the mean value with its corresponding standard deviation (SD). The duration of asthma in our study was observed to be 24.12 years, with a standard deviation of 4.61 years. The HAMD scores for the BA group and the HC group are 8.13 ± 2.39 and 4.74 ± 1.77, respectively, while the MoCA scores for the BA group and the HC group are 25.52 ± 1.93 and 27.55 ± 1.34, respectively. The BA group shows mild cognitive impairment and emotional changes. Please refer to Table 2 for detailed results.
Differences in resting-state functional connectivity between the two groups
The results of inter-group rs-FC comparisons based on bilateral thalamic seed regions and voxels from other brain areas are presented in Table 3. In comparison to the HC group, the BA group exhibited increased FC between the left thalamus and the left cerebellar posterior lobe (CPL), left postcentral gyrus (PCG), and right superior frontal gyrus (SFG). Simultaneously, it demonstrated decreased FC with both the lentiform nucleus (LN) and the left corpus callosum (CC). The right thalamus showed increased FC with the right SFG and the left CPL, while FC with the right LN and the left CC decreased. The anatomical locations, voxel sizes, and corresponding MNI peak coordinates of differential brain regions between the two groups are detailed in Table 3. Figures 1, 2 depict the brain regions where notable alterations in bilateral thalamic rs-FC were observed in both groups.
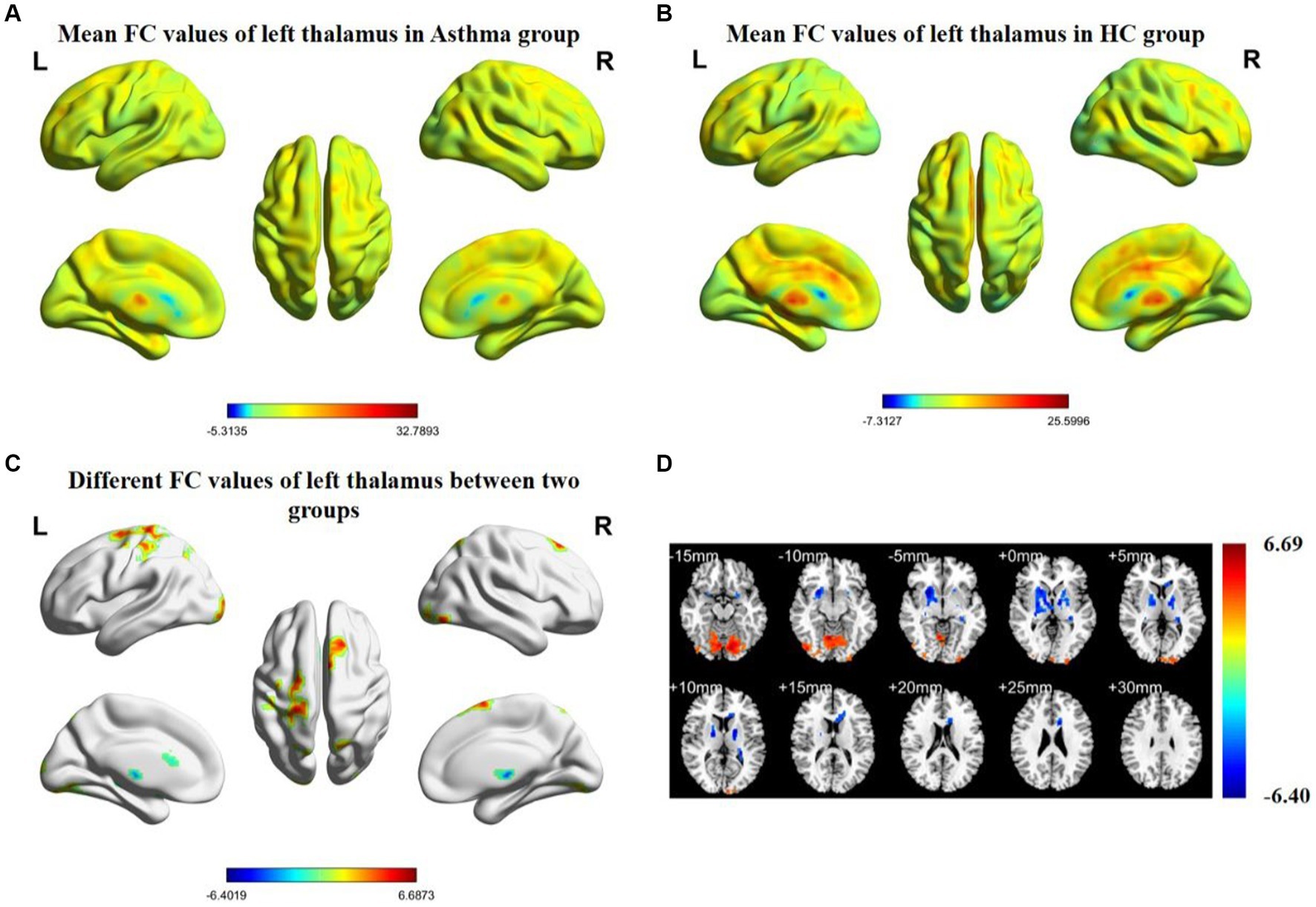
Figure 1. Spatial distribution maps of functional connectivity (FC) in the brain regions of the bronchial asthma group (A) and the healthy control group (B), based on the seed point of the left thalamus. (C, D) The brain regions where there are significant differences in FC between the two groups (with a voxel-level p-value less than 0.05, corrected using AlphaSim). Color bars indicate t-scores; cool colors indicate regions in BA with lower FC values compared to HC, while warm colors indicate the opposite.
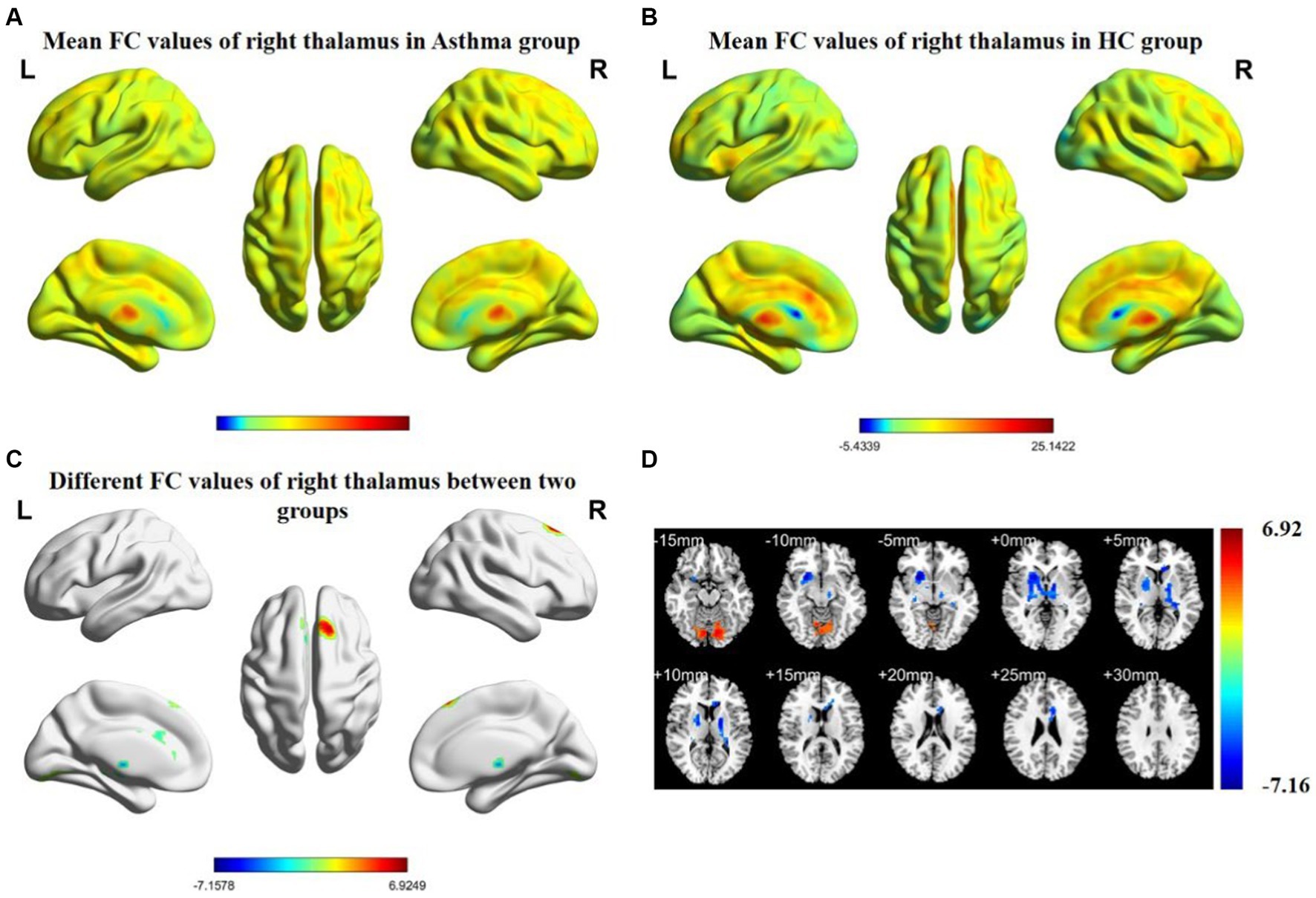
Figure 2. Spatial distribution maps of functional connectivity (FC) in the brain regions of the bronchial asthma group (A) and the healthy control group (B), based on the seed point of the right thalamus. (C, D) The brain regions where there are significant differences in FC between the two groups (with a voxel-level p-value less than 0.05, corrected using AlphaSim). Color bars indicate t-scores; cool colors indicate areas in BA with lower FC values compared to HC, while warm colors indicate the opposite.
Correlation analysis
Partial correlation analysis revealed a negative correlation between the FC values of the left thalamus (L-Thal) and right superior frontal gyrus (R-SFG) in the BA group and MoCA scores (r = −0.7437, p < 0.0001, Figure 3A), as well as a positive correlation with HAMD scores (r = 0.7232, p < 0.0001, Figure 3C). Furthermore, the FC values of L-Thal and left cerebellar posterior lobe (L-CPL) showed a negative correlation with MoCA scores (r = −0.6701, p < 0.0001, Figure 3B) and a positive correlation with HAMD scores (r = 0.7449, p < 0.0001, Figure 3D). The FC values between the bilateral thalamus and other differential brain regions did not show significant correlations with neuro-psychological measurement assessments.
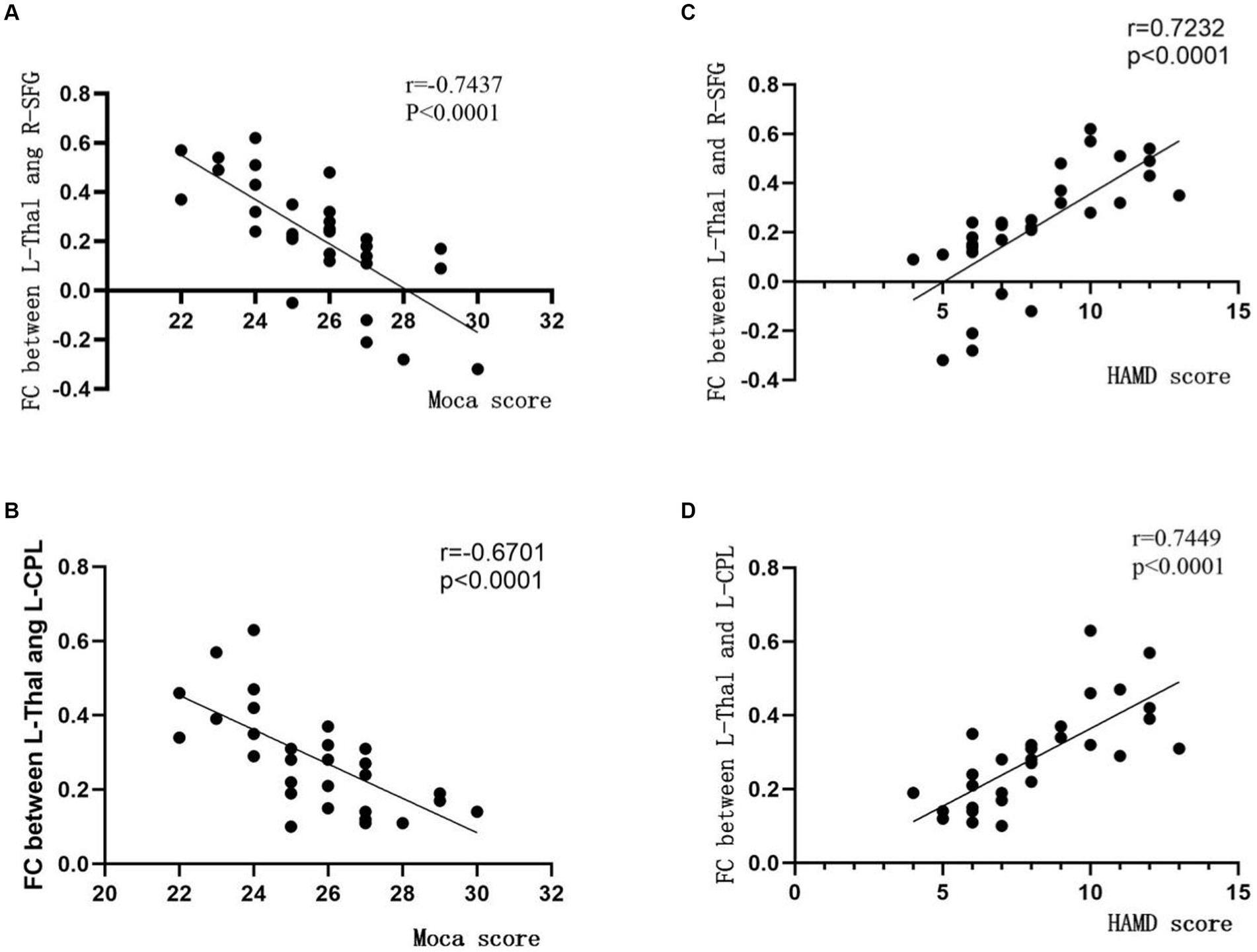
Figure 3. Correlation between inter-group differential brain regions based on thalamus functional connectivity analysis and scores on neuro-psychological assessment scales. The vertical axis represents the functional connectivity (FC) values, while the horizontal axis corresponds to the scores on the neuro-psychological assessment scales. MoCA, Montreal Cognitive Assessment; HAMD, Hamilton Depression; Thal, thalamus; SFG, superior frontal gyrus; CPL, cerebellar posterior lobe; L for Left; R for Right.
Discussion
Previous research has found a significant decrease in voxel-mirrored homotopic connectivity (VMHC) between the bilateral thalamus in asthma patients, reflecting specific changes in internal information exchange, integration, and coordination within the thalamus (13). However, unlike discussions on functional changes between homologous regions of the bilateral thalamus with VMHC, functional connectivity (FC) analysis focuses more on alterations in the interaction between the thalamus as a key relay station and different brain regions (20, 21). This study is the first to look into rs-FC in BA patients between the thalamus and other brain areas. The principal discoveries of this study demonstrate that individuals with asthma show extensive FC deficiencies in multiple brain regions associated with emotion regulation, cognitive control, somatic sensation, arousal, visual processing, and auditory processing. These findings align with our initial hypothesis. Despite statistical analyses revealing only mild cognitive impairment and emotional abnormalities in the BA group based on the scores from the two assessment scales, subsequent correlation analyses unveiled potential neurobiological indicators reflecting cognition and emotion. Specifically, the functional connectivity strength between the left thalamus (L-Thal) and the right superior frontal gyrus (R-SFG), as well as the left cerebellar posterior lobe (L-CPL), was identified.
The cerebellum posterior lobe (CPL) constitutes a significant portion of the human cerebellum (22). The thalamus conveys signals originating from the dentate nucleus of the CPL and subsequently projects them to the cerebral cortex, providing processed information back to the cerebellum (23). This neural circuit is typically associated with precision task execution, visual processing, speech expression, and emotional responses (24, 25). Similarly, we observed a significant correlation between increased functional connectivity (FC) between the thalamus and cerebellar posterior lobe (CPL) and the presence of cognitive impairment and emotional abnormalities. Aberrant FC results in a decline in functional interaction capacity between brain regions, thereby weakening the efficiency of information transmission. In research examining primary nocturnal enuresis in children, disturbances in arousal were linked to diminished functional connectivity between the thalamus and CPL (26). In the study conducted by Liu and colleagues (27), it was also observed that individuals suffering from sleep disorders exhibited elevated metabolic activity in both the thalamus and the CPL. Xie et al. (28) investigated the spontaneous brain activity in patients with obstructive sleep apnea (OSA) using the percentage of amplitude fluctuation (PerAF). Their findings revealed an increased PerAF in the CPL, which correlates with the presence of sleep disturbances. Another study also indicates enhanced regional activity in the left CPL in BA patients (29). In our study, we observed enhanced FC between the Thal and CPL. This may potentially amplify projections to the cortical areas of CPL, resulting in cortical excitation. This could possibly explain the higher prevalence of nocturnal awakenings and sleep disturbances in asthma patients. Prolonged insufficient sleep can lead to decreased vigilance, resulting in reduced responsiveness of individuals with asthma to external threats (30, 31). This may contribute to an increased risk of triggering asthma-related behaviors. Research indicates that, compared to non-depressed individuals with asthma, those with depression exhibit significantly increased cerebral blood flow in the CPL (32–34). The inclination toward depression is also related to CPL metabolism (35). Furthermore, as a region with a “proofreading” function for information, the increased signal strength in the cerebellum reflects abnormal control of respiratory muscles, and this change in asthmatic individuals may be associated with alterations in their respiratory rate (36, 37). It is worth noting that in the cerebellum-thalamus-cerebral cortex (CTC) ascending loop, we have only identified FC changes between the cerebellum and thalamus in BA patients. In the future, probabilistic fiber tractography can be used to determine the regions with strong fiber bundle connections between the thalamus and the cerebellum, serving as ROIs for the projection of the cerebellum onto the thalamus. This approach can be employed to construct the FC between the thalamus and the cortex within this circuit, thereby elucidating the specific changes within the CTC in individuals with asthma.
LN, including the pallidum and the putamen nucleus, is a critical part of the basal ganglia, projecting to the ventrolateral nucleus of the thalamus and the lateral geniculate body (38). The basal ganglia-thalamus-cortical loop (BTC) is involved in arousal and attention functions (39). It also mediates regulatory control over a wide range of cortical areas, playing a pivotal role in emotional processing, motor control, cognitive processing, and motivational behavior (40). In our study, we observed a reduction in FC between the left thalamus and bilateral LN, while the right thalamus exhibited decreased FC, specifically with the right LN. The altered FC patterns may contribute to an overall decrease in information transmission efficiency between the thalamus and the cerebral cortex. Previous fMRI studies have indicated that the pallidum and the putamen nucleus often exhibit abnormal spontaneous activity when individuals are experiencing emotions such as fear, anxiety, and sadness (41). Individuals with asthma are more likely to trigger anxious thoughts about the dire consequences of an asthma attack. Additionally, it is widely hypothesized that the aberrant neural activity in this circuit serves as a fundamental neural mechanism in individuals with obsessive-compulsive disorder (42). Decreased thalamic gating efficiency can lead to a greater projection of intrusive, distressing thoughts into the cortex (16). Under the influence of obsessive thoughts and anxious emotions, this can result in more frequent oral corticosteroid and bronchodilator use. Prolonged corticosteroid treatment is linked to a decrease in LN volume, which in turn leads to more severe anxiety occurrences (14, 43). Furthermore, damage to dopaminergic neurons in LN is closely associated with cognitive impairments in schizophrenia, including deficits in attention, working memory, reward processing, and executive functions (44). The LN is involved in the reward processing system and is responsible for linking different sensory cues with rewarding outcomes. In individuals suffering from severe depression, LN becomes abnormally activated during the occurrence of negative emotions, thereby intensifying the avoidance motivation to alleviate the experience of negative emotions (45, 46). Impairments in reward and executive functions in BA patients may lead to reduced proactiveness in seeking medication and a higher likelihood of experiencing negative emotions. In the future, it is also possible to integrate multimodal data from DTI and fMRI to segmentally construct a detailed profile of BTC changes in BA patients.
The SFG is situated in the anterior medial prefrontal cortex, a key region within the default mode network (DMN), and is primarily involved in functions such as attention selection, inhibitory control, stress perception, and working memory (47, 48). The DMN consumes a substantial amount of energy during rest, making it particularly susceptible to oxidative stress damage and the influence of diseases (49). Previous research has shown reduced activity in other brain regions of the DMN in individuals with asthma, such as the angular gyrus and the precuneus, confirming regional functional and network-level intrinsic activity abnormalities in the brains of BA patients (10). Hwang et al. (17) discovered robust functional connections between the DMN and various thalamic nuclei. Therefore, we hypothesize that, to compensate for the reduction in internal network activity, the thalamus and SFG’s FC will increase adaptively. The thalamus mediates top-down regulation and the filtering and integration of sensory information, as well as serving as a crucial hub for selective inhibition of external stimuli and focused attention (50). Meanwhile, the prefrontal cortex plays a central role in processing higher-level emotional and cognitive information. The enhanced functional connection between SFG and the thalamus is considered a neurofunctional characteristic of schizophrenia, often characterized by excessive attention to external stimuli and abnormal emotional reactions to these stimuli (51). Furthermore, these changes in BA patients may lead to a reduction in the use of adaptive strategy, potentially resulting in emotional regulation disorders (52, 53). Research indicates a positive correlation between spontaneous activity in SFG and perceived stress, with excessive perceived stress typically stemming from negative emotions such as anxiety and depression (54). The thalamus also plays a role in mediating noxious input to the cortex, and the functional reorganization of the SFG is crucial in top-down modulation of pain experiences, with strong FC between them potentially contributing to the sustained perception of pain (55). The thalamus and SFG’s FC serve as a shared pathway in the experiences of breathlessness and pain perception (56, 57). We hypothesize that asthma patients, in the long-term management of their breathing difficulties, gradually adapt to the stress associated with pain, transforming their adaptation to breathlessness into a tolerance for pain. This adaptation is manifested through increased spontaneous activity in the superior frontal gyrus (SFG). Therefore, the heightened functional connectivity (FC) between the thalamus and SFG in BA patients may be linked to emotional regulation disorders, perceived stress, and experiences related to pain and breathlessness.
PCG is a crucial region within the sensorimotor network responsible for processing various types of sensory perceptions. Sensory stimuli are conveyed through C-fibers to the spinal thalamic tract and are then relayed to the PCG via the thalamic ventral posterior nucleus (58). In their analysis of gender differences in chronic cough, Morice et al. (59) found that females exhibited higher sensitivity in their cough reflex, which was associated with increased activation in the PCG. Our research has revealed that, in comparison to HCs, asthma patients exhibit increased FC between the thalamus and PCG. This finding aligns with the tendency for asthma patients to experience coughing more frequently. Furthermore, damage to the PCG cortex could potentially affect respiratory motor control. Zhang et al. (60), in their fMRI analysis of COPD patients, observed enhanced low-frequency amplitude of fluctuations (ALFF) in the PCG, which was closely related to over-breathing. As the condition worsens, patients with asthma may gradually experience decreased sensitivity in perceiving asthma symptoms within the somatosensory cortex. This decline in perception sensitivity may hinder their ability to promptly address their condition due to reduced perception strength (61). Previous research has yielded varying results, showing reduced functional connectivity (FC) between the insular cortex and the PCG in BA patients (42). This reduction may be attributed to pathological activation in the PCG, which subsequently diminishes the FC between the insular cortex and PCG. Furthermore, early cognitive development often relies on essential sensory experiences, and excessive input from the thalamus can lead to aberrant sensory experiences, ultimately impacting cognitive function (58). Hence, the PCG is involved in the occurrence of bronchial hyperreactivity and respiratory overdrive in asthma, and it can serve as a potential biomarker for the severity of asthma symptoms.
Lastly, we have observed a decrease in FC between the CC and the thalamus, even though there is no apparent anatomical correlation between them. But functionally, they are interconnected, such as in the context of visual short-term memory capacity (62), visual processing (63), and speech formation (64). In fact, the input from the thalamus plays a highly instructive role in the maturation trend of the CC area, achieved through the regulation of neuronal projection, development, signal transduction, and activity-dependent plasticity (65, 66). The CC serves as an intermediary for interhemispheric communication (67) and indirectly contributes to cognitive functions like language, attention, working memory, and visual spatial memory (68–70). Previous studies have suggested that CC atrophy in BA patients might be mediated by allergens (71) and affect the cortical metabolism associated with cognitive functions (72). In BA patients, there is a reduction in the FC between the CC and the thalamus, which we hypothesize is associated with a decline in the coordinated processing of sensory stimuli across both brain hemispheres. This decline includes the ability to recognize odors from olfactory stimuli (73, 74). This might explain why asthma patients have difficulty identifying harmful gases, which can trigger asthma symptoms. In an fMRI study, Wang et al. (75) found that damage to the white matter integrity of the CC is related to the severity of anxiety. Changes in FC between the thalamus and these brain structures contribute to understanding the pathophysiological mechanisms underlying cognitive impairments, emotional dysregulation, and motor control failures in BA patients. In the future, inducing positive plastic changes may be considered in the treatment of BA to promote brain function recovery.
Limitations
Nonetheless, there are certain constraints associated with this study. In the first place, the sample size was rather limited owing to rigorous inclusion criteria. Furthermore, during the administration of the neuro-psychological assessment scales, it is noteworthy that the subjects may not have fully adhered to a conservative representation of their condition, introducing a potential bias to the test results. Thirdly, this study did not investigate how these FC differences change over time. Future research could employ dynamic FC analysis to elucidate this aspect. Hence, it is imperative to conduct longitudinal studies using a variety of analytical approaches for fMRI. This will enable us to investigate the evolving patterns in FC as time progresses. Fourthly, our study only identified brain regions where there were differences in FC between the BA group and the HCs concerning the thalamus and other cortical regions. In the future, machine learning methods such as support vector machine (SVM) classifiers can be employed to determine whether these brain regions can serve as discriminative features for distinguishing these groups.
Conclusion
In our study, we have verified the presence of aberrant FC patterns in the thalamus of BA patients. When compared to HCs, BA patients exhibit abnormal changes in functional connectivity between the thalamus and various brain regions associated with vision, hearing, emotional regulation, cognitive control, somatic sensations, and wakefulness. This provides further confirmation of the substantial role played by the thalamus in the progression of BA.
Data availability statement
The original contributions presented in the study are included in the article/supplementary material, further inquiries can be directed to the corresponding author.
Ethics statement
The studies involving humans were approved by Ethics Committee of Jiangxi Provincial People’s Hospital. The studies were conducted in accordance with the local legislation and institutional requirements. The participants provided their written informed consent in this study.
Author contributions
TW: Conceptualization, Data curation, Formal analysis, Investigation, Methodology, Software, Writing – original draft. JW: Funding acquisition, Supervision, Writing – review & editing. XH: Methodology, Resources, Software, Writing – review & editing. L-xD: Investigation, Methodology, Writing – review & editing. K-mZ: Investigation, Methodology, Writing – review & editing.
Funding
The author(s) declare financial support was received for the research, authorship, and/or publication of this article. This study was funded by the Jiangxi Provincial Natural Science Foundation General Project (20202BAB206003).
Conflict of interest
The authors declare that the research was conducted in the absence of any commercial or financial relationships that could be construed as a potential conflict of interest.
Publisher’s note
All claims expressed in this article are solely those of the authors and do not necessarily represent those of their affiliated organizations, or those of the publisher, the editors and the reviewers. Any product that may be evaluated in this article, or claim that may be made by its manufacturer, is not guaranteed or endorsed by the publisher.
References
1. Wong, QYA, Lim, JJ, Ng, JY, Malipeddi, P, Lim, YYE, Sio, YY, et al. An updated prevalence of asthma, its phenotypes, and the identification of the potential asthma risk factors among young Chinese adults recruited in Singapore. World Allergy Organ J. (2023) 16:100757. doi: 10.1016/j.waojou.2023.100757
2. Habib, N, Pasha, MA, and Tang, DD. Current understanding of asthma pathogenesis and biomarkers. Cells. (2022) 11:764. doi: 10.3390/cells11172764
3. Quan, N, and Banks, WA. Brain-immune communication pathways. Brain Behav Immun. (2007) 21:727–35. doi: 10.1016/j.bbi.2007.05.005
4. Parker, J, Wolansky, LJ, Khatry, D, Geba, GP, and Molfino, NA. Brain magnetic resonance imaging in adults with asthma. Contemp Clin Trials. (2011) 32:86–9. doi: 10.1016/j.cct.2010.09.006
5. Rosenkranz, MA, Dean, DC III, Bendlin, BB, Jarjour, NN, Esnault, S, Zetterberg, H, et al. Neuroimaging and biomarker evidence of neurodegeneration in asthma. J Allergy Clin Immunol. (2022) 149:589–598.e6. doi: 10.1016/j.jaci.2021.09.010
6. Parajuli, B, Horiuchi, H, Mizuno, T, Takeuchi, H, and Suzumura, A. CCL11 enhances excitotoxic neuronal death by producing reactive oxygen species in microglia. Glia. (2015) 63:2274–84. doi: 10.1002/glia.22892
7. Dehdar, K, Salimi, M, and Raoufy, MR. Allergen disrupts amygdala-respiration coupling. Respir Physiol Neurobiol. (2022) 297:103835. doi: 10.1016/j.resp.2021.103835
8. Kroll, JL, and Ritz, T. Asthma, the central nervous system, and neurocognition: current findings, potential mechanisms, and treatment implications. Neurosci Biobehav Rev. (2023) 146:105063. doi: 10.1016/j.neubiorev.2023.105063
9. Lavielle, P, Talavera, JO, Reynoso, N, González, M, Gómez-Díaz, RA, Cruz, M, et al. Prevalence of cognitive impairment in recently diagnosed type 2 diabetes patients: are chronic inflammatory diseases responsible for cognitive decline? PLoS One. (2015) 10:e0141325. doi: 10.1371/journal.pone.0141325
10. Li, S, Lv, P, He, M, Zhang, W, Liu, J, Gong, Y, et al. Cerebral regional and network characteristics in asthma patients: a resting-state fMRI study. Front Med. (2020) 14:792–801. doi: 10.1007/s11684-020-0745-1
11. Ritz, T, Kroll, JL, Patel, SV, Chen, JR, Yezhuvath, US, Aslan, S, et al. Central nervous system signatures of affect in asthma: associations with emotion-induced bronchoconstriction, airway inflammation, and asthma control. J Appl Physiol. (1985) 126:1725–36. doi: 10.1152/japplphysiol.01018.2018
12. Zhu, L, Zhao, J, Yang, Y, Shangguan, Q, Chen, Y, He, Y, et al. Brain network study of attentional cognitive impairment in children with bronchial asthma. Int J Dev Neurosci. (2023) 83:224–31. doi: 10.1002/jdn.10250
13. Wu, YJ, Rao, J, Huang, X, Wu, N, Shi, L, Huang, H, et al. Impaired interhemispheric synchrony in bronchial asthma. Int J Gen Med. (2021) 14:10315–25. doi: 10.2147/IJGM.S343269
14. Ritz, T, Kroll, JL, Aslan, S, Janssens, T, Khan, DA, Pinkham, AE, et al. Subcortical gray matter volumes in asthma: associations with asthma duration, control, and anxiety. Brain Imaging Behav. (2020) 14:2341–50. doi: 10.1007/s11682-019-00188-3
15. Roy, DS, Zhang, Y, Halassa, MM, and Feng, G. Thalamic subnetworks as units of function. Nat Neurosci. (2022) 25:140–53. doi: 10.1038/s41593-021-00996-1
16. Niu, Q, Yang, L, Song, X, Chu, C, Liu, H, Zhang, L, et al. Abnormal resting-state brain activities in patients with first-episode obsessive-compulsive disorder. Neuropsychiatr Dis Treat. (2017) 13:507–13. doi: 10.2147/NDT.S117510
17. Hwang, K, Bertolero, MA, Liu, WB, and D'Esposito, M. The human thalamus is an integrative hub for functional brain networks. J Neurosci. (2017) 37:5594–607. doi: 10.1523/JNEUROSCI.0067-17.2017
18. Bourgeois, JA, John, M, Zepf, R, Greene, M, Frankel, S, and Hessol, NA. Functional deficits and other psychiatric associations with abnormal scores on the Montreal cognitive assessment (MoCA) in older HIV-infected patients. Int Psychogeriatr. (2020) 32:105–18. doi: 10.1017/S1041610219000413
19. Ren, S, Chang, M, Yin, Z, Feng, R, Wei, Y, Duan, J, et al. Age-related alterations of white matter integrity in adolescents and young adults with bipolar disorder. Front Psych. (2019) 10:1010. doi: 10.3389/fpsyt.2019.01010
20. Kocar, TD, Müller, HP, and Kassubek, J. Differential functional connectivity in thalamic and dopaminergic pathways in restless legs syndrome: a meta-analysis. Ther Adv Neurol Disord. (2020) 13:1756286420941670. doi: 10.1177/1756286420941670
21. Donahue, EK, Venkadesh, S, Bui, V, Tuazon, AC, Wang, RK, Haase, D, et al. Physical activity intensity is associated with cognition and functional connectivity in Parkinson's disease. Parkinsonism Relat Disord. (2022) 104:7–14. doi: 10.1016/j.parkreldis.2022.09.005
22. Liu, WF, Shu, YQ, Zhu, PW, Li, B, Shi, WQ, Lin, Q, et al. The cerebellum posterior lobe associates with the exophthalmos of primary hyperthyroidism: a resting-state fMRI study. Int J Endocrinol. (2019) 2019:8135671. doi: 10.1155/2019/8135671
23. Qiao, J, Wu, ZM, Ye, QP, Dai, M, Dai, Y, He, ZT, et al. Characteristics of dysphagia among different lesion sites of stroke: a retrospective study. Front Neurosci. (2022) 16:944688. doi: 10.3389/fnins.2022.944688
24. Zhu, B, Wang, Y, Zhang, G, Ouyang, H, Zhang, J, Zheng, Y, et al. Acupuncture at KI3 in healthy volunteers induces specific cortical functional activity: an fMRI study. BMC Complement Altern Med. (2015) 15:361. doi: 10.1186/s12906-015-0881-3
25. Schmahmann, JD . The cerebellum and cognition. Neurosci Lett. (2019) 688:62–75. doi: 10.1016/j.neulet.2018.07.005
26. Zhang, A, Zhang, L, Wang, M, Zhang, Y, Jiang, F, Jin, X, et al. Functional connectivity of thalamus in children with primary nocturnal enuresis: results from a resting-state fMRI study. Brain Imaging Behav. (2021) 15:355–63. doi: 10.1007/s11682-020-00262-1
27. Liu, X, Yu, T, Zhao, X, Yu, P, Lv, R, Wang, C, et al. Risk factors and brain metabolic mechanism of sleep disorders in autoimmune encephalitis. Front Immunol. (2021) 12:738097. doi: 10.3389/fimmu.2021.738097
28. Xie, W, Shu, Y, Liu, X, Li, K, Li, P, Kong, L, et al. Abnormal spontaneous brain activity and cognitive impairment in obstructive sleep apnea. Nat Sci Sleep. (2022) 14:1575–87. doi: 10.2147/NSS.S376638
29. Huang, H, Li, SY, Shi, L, Huang, X, and Wang, J. Altered spontaneous brain activity in patients with asthma: a resting-state functional MRI study using regional homogeneity analysis. NeuroReport. (2021) 32:1403–7. doi: 10.1097/WNR.0000000000001736
30. Liu, X, Yan, Z, Wang, T, Yang, X, Feng, F, Fan, L, et al. Connectivity pattern differences bilaterally in the cerebellum posterior lobe in healthy subjects after normal sleep and sleep deprivation: a resting-state functional MRI study. Neuropsychiatr Dis Treat. (2015) 11:1279–89. doi: 10.2147/NDT.S84204
31. Albakri, U, Drotos, E, and Meertens, R. Sleep health promotion interventions and their effectiveness: an umbrella review. Int J Environ Res Public Health. (2021) 18:5533. doi: 10.3390/ijerph18115533
32. Zhang, Y, Yang, Y, Wang, Z, Bian, R, Jiang, W, Yin, Y, et al. Altered regional cerebral blood flow of right cerebellum posterior lobe in asthmatic patients with or without depressive symptoms. Front Psych. (2018) 9:225. doi: 10.3389/fpsyt.2018.00225
33. Wildgruber, D, Riecker, A, Hertrich, I, Erb, M, Grodd, W, Ethofer, T, et al. Identification of emotional intonation evaluated by fMRI. NeuroImage. (2005) 24:1233–41. doi: 10.1016/j.neuroimage.2004.10.034
34. Fu, X, Zhang, F, Liu, F, Yan, C, and Guo, W. Editorial: brain and somatization symptoms in psychiatric disorders. Front Psych. (2019) 10:146. doi: 10.3389/fpsyt.2019.00146
35. Cheng, B, Roberts, N, Zhou, Y, Wang, X, Li, Y, Chen, Y, et al. Social support mediates the influence of cerebellum functional connectivity strength on postpartum depression and postpartum depression with anxiety. Transl Psychiatry. (2022) 12:54. doi: 10.1038/s41398-022-01781-9
36. Harper, RM, Macey, PM, Henderson, LA, Woo, MA, Macey, KE, Frysinger, RC, et al. fMRI responses to cold pressor challenges in control and obstructive sleep apnea subjects. J Appl Physiol. (1985) 94:1583–95. doi: 10.1152/japplphysiol.00881.2002
37. Pollok, B, Jurkiewicz, M, and Krause, V. Anodal transcranial direct current stimulation (atDCS) of the primary motor cortex (M1) facilitates nonconscious error correction of negative phase shifts. Neural Plast. (2022) 2022:9419154. doi: 10.1155/2022/9419154
38. Tang, S, Liu, X, Nie, L, Qian, F, Chen, W, and He, L. Three-dimensional pseudocontinuous arterial spin labeling perfusion imaging shows cerebral blood flow perfusion decline in attention-deficit/hyperactivity disorder children. Front Psych. (2023) 14:1064647. doi: 10.3389/fpsyt.2023.1064647
39. Zhang, J, Zhang, H, Yan, F, Zhang, H, Zhang, E, Wang, X, et al. Investigating the mechanism and prognosis of patients with disorders of consciousness on the basis of brain networks between the thalamus and whole-brain. Front Neurol. (2022) 13:990686. doi: 10.3389/fneur.2022.990686
40. Aravamuthan, BR, and Waugh, JL. Localization of basal ganglia and thalamic damage in Dyskinetic cerebral palsy. Pediatr Neurol. (2016) 54:11–21. doi: 10.1016/j.pediatrneurol.2015.10.005
41. Ashworth, E, Brooks, SJ, and Schiöth, HB. Neural activation of anxiety and depression in children and young people: a systematic meta-analysis of fMRI studies. Psychiatry Res Neuroimaging. (2021) 311:111272. doi: 10.1016/j.pscychresns.2021.111272
42. Zhang, Y, Yang, Y, Bian, R, Yin, Y, Hou, Z, Yue, Y, et al. Group cognitive behavior therapy reversed insula subregions functional connectivity in asthmatic patients. Front Aging Neurosci. (2017) 9:105. doi: 10.3389/fnagi.2017.00105
43. Liang, J, Yu, Q, Liu, Y, Qiu, Y, Tang, R, Yan, L, et al. Gray matter abnormalities in patients with major depressive disorder and social anxiety disorder: a voxel-based meta-analysis. Brain Imaging Behav. (2023) 17:749–63. doi: 10.1007/s11682-023-00797-z
44. Li, P, Zhao, SW, Wu, XS, Zhang, YJ, Song, L, Wu, L, et al. The association between Lentiform nucleus function and cognitive impairments in schizophrenia. Front Hum Neurosci. (2021) 15:777043. doi: 10.3389/fnhum.2021.777043
45. Wang, X, Zhou, X, Dai, Q, Ji, B, and Feng, Z. The role of motivation in cognitive reappraisal for depressed patients. Front Hum Neurosci. (2017) 11:516. doi: 10.3389/fnhum.2017.00516
46. Zhang, L, Li, Q, du, Y, Gao, Y, Bai, T, Ji, GJ, et al. Effect of high-definition transcranial direct current stimulation on improving depression and modulating functional activity in emotion-related cortical-subcortical regions in bipolar depression. J Affect Disord. (2023) 323:570–80. doi: 10.1016/j.jad.2022.12.007
47. Greicius, MD, Krasnow, B, Reiss, AL, and Menon, V. Functional connectivity in the resting brain: a network analysis of the default mode hypothesis. Proc Natl Acad Sci USA. (2003) 100:253–8. doi: 10.1073/pnas.0135058100
48. Briggs, RG, Khan, AB, Chakraborty, AR, Abraham, CJ, Anderson, CD, Karas, PJ, et al. Anatomy and white matter connections of the superior frontal gyrus. Clin Anat. (2020) 33:823–32. doi: 10.1002/ca.23523
49. Wang, Y, Chen, L, Cai, F, Gao, J, Ouyang, F, Chen, Y, et al. Altered functional connectivity of the thalamus in primary angle-closure glaucoma patients: a resting-state fMRI study. Front Neurol. (2022) 13:1015758. doi: 10.3389/fneur.2022.1015758
50. Yuan, HL, Lai, CYY, Wong, MNK, Kwong, TC, Choy, YS, Mung, SWY, et al. Interventions for sensory over-responsivity in individuals with autism Spectrum disorder: a narrative review. Children. (2022) 9:1584. doi: 10.3390/children9101584
51. Widmayer, S, Borgwardt, S, Lang, UE, Stieglitz, RD, and Huber, CG. Functional neuroimaging correlates of aggression in psychosis: a systematic review with recommendations for future research. Front Psych. (2018) 9:777. doi: 10.3389/fpsyt.2018.00777
52. Shi, J, Yao, Y, Zhan, C, Mao, Z, Yin, F, and Zhao, X. The relationship between big five personality traits and psychotic experience in a large non-clinical youth sample: the mediating role of emotion regulation. Front Psych. (2018) 9:648. doi: 10.3389/fpsyt.2018.00648
53. Guo, WB, Liu, F, Xue, ZM, Xu, XJ, Wu, RR, Ma, CQ, et al. Alterations of the amplitude of low-frequency fluctuations in treatment-resistant and treatment-response depression: a resting-state fMRI study. Prog Neuro-Psychopharmacol Biol Psychiatry. (2012) 37:153–60. doi: 10.1016/j.pnpbp.2012.01.011
54. Wang, S, Zhao, Y, Zhang, L, Wang, X, Wang, X, Cheng, B, et al. Stress and the brain: perceived stress mediates the impact of the superior frontal gyrus spontaneous activity on depressive symptoms in late adolescence. Hum Brain Mapp. (2019) 40:4982–93. doi: 10.1002/hbm.24752
55. Lee, JJ, Lee, S, Lee, DH, and Woo, CW. Functional brain reconfiguration during sustained pain. eLife. (2022) 11:463. doi: 10.7554/eLife.74463
56. von Leupoldt, A, Sommer, T, Kegat, S, Baumann, HJ, Klose, H, Dahme, B, et al. Dyspnea and pain share emotion-related brain network. NeuroImage. (2009) 48:200–6. doi: 10.1016/j.neuroimage.2009.06.015
57. Herigstad, M, Hayen, A, Evans, E, Hardinge, FM, Davies, RJ, Wiech, K, et al. Dyspnea-related cues engage the prefrontal cortex: evidence from functional brain imaging in COPD. Chest. (2015) 148:953–61. doi: 10.1378/chest.15-0416
58. Zhu, W, Tang, W, Liang, Y, Jiang, X, Li, Y, Chen, Z, et al. Aberrant functional connectivity of sensorimotor network and its relationship with executive dysfunction in bipolar disorder type I. Front Neurosci. (2021) 15:823550. doi: 10.3389/fnins.2021.823550
59. Morice, AH, Jakes, AD, Faruqi, S, Birring, SS, McGarvey, L, Canning, B, et al. A worldwide survey of chronic cough: a manifestation of enhanced somatosensory response. Eur Respir J. (2014) 44:1149–55. doi: 10.1183/09031936.00217813
60. Zhang, J, Chen, J, Yu, Q, Fan, C, Zhang, R, Lin, J, et al. Alteration of spontaneous brain activity in COPD patients. Int J Chron Obstruct Pulmon Dis. (2016) 11:1713–9. doi: 10.2147/COPD.S110089
61. Davenport, P W, Cruz, M, Stecenko, A A, and Kifle, Y. Respiratory-related evoked potentials in children with life-threatening asthma. Am J Respir Crit Care Med. (2000) 161:1830–5. doi: 10.1164/ajrccm.161.6.9903077
62. Menegaux, A, Meng, C, Neitzel, J, Bäuml, JG, Müller, HJ, Bartmann, P, et al. Impaired visual short-term memory capacity is distinctively associated with structural connectivity of the posterior thalamic radiation and the splenium of the corpus callosum in preterm-born adults. NeuroImage. (2017) 150:68–76. doi: 10.1016/j.neuroimage.2017.02.017
63. Zarkali, A, McColgan, P, Leyland, LA, Lees, AJ, Rees, G, and Weil, RS. Fiber-specific white matter reductions in Parkinson hallucinations and visual dysfunction. Neurology. (2020) 94:e1525–38. doi: 10.1212/WNL.0000000000009014
64. Schaltenbrand, G . The effects on speech and language of stereotactical stimulation in thalamus and corpus callosum. Brain Lang. (1975) 2:70–7. doi: 10.1016/S0093-934X(75)80055-1
65. Wilson, S, Pietsch, M, Cordero-Grande, L, Price, AN, Hutter, J, Xiao, J, et al. Development of human white matter pathways in utero over the second and third trimester. Proc Natl Acad Sci USA. (2021) 118:e2023598118. doi: 10.1073/pnas.2023598118
66. De León Reyes, NS, Bragg-Gonzalo, L, and Nieto, M. Development and plasticity of the corpus callosum. Development. (2020) 147:dev189738. doi: 10.1242/dev.189738
67. Mizutani, R, Saiga, R, Ohtsuka, M, Miura, H, Hoshino, M, Takeuchi, A, et al. Three-dimensional X-ray visualization of axonal tracts in mouse brain hemisphere. Sci Rep. (2016) 6:35061. doi: 10.1038/srep35061
68. Koo, DL, Cabeen, RP, Yook, SH, Cen, SY, Joo, EY, and Kim, H. More extensive white matter disruptions present in untreated obstructive sleep apnea than we thought: a large sample diffusion imaging study. Hum Brain Mapp. (2023) 44:3045–56. doi: 10.1002/hbm.26261
69. Roy, B, Sahib, AK, Kang, D, Aysola, RS, and Kumar, R. Brain tissue integrity mapping in adults with obstructive sleep apnea using T1-weighted and T2-weighted images. Ther Adv Neurol Disord. (2022) 15:17562864221137505. doi: 10.1177/17562864221137505
70. Kheirandish-Gozal, L, Sahib, AK, Macey, PM, Philby, MF, Gozal, D, and Kumar, R. Regional brain tissue integrity in pediatric obstructive sleep apnea. Neurosci Lett. (2018) 682:118–23. doi: 10.1016/j.neulet.2018.06.002
71. Dehdar, K, and Raoufy, MR. Effects of inhaled corticosteroids on brain volumetry, depression and anxiety-like behaviors in a rat model of asthma. Respir Physiol Neurobiol. (2023) 315:104121. doi: 10.1016/j.resp.2023.104121
72. Yamauchi, H, Fukuyama, H, Nagahama, Y, Katsumi, Y, Dong, Y, Konishi, J, et al. Atrophy of the corpus callosum associated with cognitive impairment and widespread cortical hypometabolism in carotid artery occlusive disease. Arch Neurol. (1996) 53:1103–9. doi: 10.1001/archneur.1996.00550110039011
73. Martinez-Nunez, AE, Soltanian-Zadeh, H, Latack, K, Ghazi, N, and Mahajan, A. Hyposmia and apathy in early, de novo Parkinson's disease: lessons from structural brain connectivity. J Neurol Sci. (2023) 452:120767. doi: 10.1016/j.jns.2023.120767
74. Eslinger, PJ, Damasio, AR, and Van Hoesen, GW. Olfactory dysfunction in man: anatomical and behavioral aspects. Brain Cogn. (1982) 1:259–85. doi: 10.1016/0278-2626(82)90028-8
Keywords: asthma, thalamus, fMRI, functional connectivity, cognitive impairment
Citation: Wang T, Huang X, Dai L-x, Zhan K-m and Wang J (2024) Functional connectivity alterations in the thalamus among patients with bronchial asthma. Front. Neurol. 15:1378362. doi: 10.3389/fneur.2024.1378362
Edited by:
Salem Hannoun, American University of Beirut, LebanonReviewed by:
Tomokazu Tsurugizawa, National Institute of Advanced Industrial Science and Technology (AIST), JapanJessica N. Kraft, The University of Texas at Dallas, United States
Copyright © 2024 Wang, Huang, Dai, Zhan and Wang. This is an open-access article distributed under the terms of the Creative Commons Attribution License (CC BY). The use, distribution or reproduction in other forums is permitted, provided the original author(s) and the copyright owner(s) are credited and that the original publication in this journal is cited, in accordance with accepted academic practice. No use, distribution or reproduction is permitted which does not comply with these terms.
*Correspondence: Jun Wang, d2FuZ2p1bjUwODdAMTYzLmNvbQ==