- 1School of Rehabilitation Medicine, Henan University of Chinese Medicine, Zhengzhou, China
- 2School of Clinical Medicine, Henan University, Zhengzhou, China
- 3Department of Rehabilitation Medicine, The First People’s Hospital of Zhengzhou, Zhengzhou, China
- 4Department of Rehabilitation, Henan Provincial People's Hospital, Zhengzhou, China
- 5Department of Rehabilitation, School of Rehabilitation Medicine, Henan Provincial People's Hospital, Henan University of Chinese Medicine, Zhengzhou, China
Objective: Executive dysfunction is a core symptom of vascular cognitive impairment (VCI), which seriously affects patients’ prognosis. This paper aims to investigate the effectiveness of rTMS on executive function in VCI.
Methods: The databases selected for this study included Pubmed, Embase, Cochrane Library, China National Knowledge Infrastructure (CNKI), Wanfang, China Science and Technology Journal Database (VIP), and China Biology Medicine Disc (CBM). The screening times were conducted from the time of library construction until August 23, 2023. The inclusion criteria for this meta-analysis were randomized controlled trials (RCTs) on rTMS for VCI, which include executive function scores. The primary metrics were executive subscale scores of the Cognitive Comprehensive Scale and total scores of the Executive Specificity Scale. The secondary metrics were subscale scores of the Executive Specificity Scale. The quality of each eligible study was assessed using the Cochrane Risk of Bias tool. Meta-analysis and bias analysis were performed using Stata (version 16.0) and RevMan (version 5.3).
Results: A total of 20 high-quality clinical RCTs with 1,049 samples were included in this paper. The findings from the primary outcomes revealed that within the rTMS group, there were significantly higher scores observed for the executive sub-item on the cognitive composite scale (SMD = 0.93, 95% CI = 0.77–1.08, p < 0.00001, I2 = 14%) and the total score on the executive specific scale (SMD = 0.69, 95% CI = 0.44–0.94, p < 0.00001, I2 = 0%) compared to the control group. As for the secondary outcome measures, as shown by the Trail Making Test-A (time) (MD = −35.75, 95% CI = −68.37 to −3.12, p = 0.03, I2 = 55%), the Stroop-C card (time) (SMD = −0.46, 95% CI = −0.86 to −0.06, p = 0.02, I2 = 0%) and the Stroop-C card (correct number) (SMD = 0.49, 95% CI = 0.04–0.94, p = 0.03, I2 = 0%), the experimental group shorts time and enhances accuracy of executive task in comparison to the control group. Subgroup analysis of the main outcome demonstrated that intermittent theta burst stimulation (iTBS), higher frequency, lower intensity, longer duration, and combined comprehensive therapy exhibited superior efficacy.
Conclusion: rTMS is effective in the treatment of the executive function of VCI. The present study has some limitations, so multi-center, large-sample, objective indicators and parameters are needed to further explore in the future.
Systematic review registration:https://www.crd.york.ac.uk/prospero/, CRD42023459669.
1 Introduction
Owing to the aging of the population, the number of patients with cognitive impairment is increasing year by year, and it is predicted that 81.1 million people will be affected by 2040. The trend increases the social and economic burden, which has become the public challenge of the times (1). Among the various causes of cognitive impairment, cerebrovascular disease stands out as the second, particularly in East Asia (2). Vascular cognitive impairment (VCI) refers to cognitive decline due to brain vessel damage, and it ranges from mild vascular cognitive impairment to vascular dementia (VaD) (3). Its symptoms mainly include four cognitive areas, among which executive dysfunction is its core symptom. Studies have also demonstrated that prefrontal subcortical function impairment is a prevalent neurophysiology of VCI, which significantly impairs executive function and negatively impacts the patient’s quality of life (2, 4, 5). At present, VCI is mainly treated by prevention and drugs. However, simple drug treatment is usually not effective and there is a lack of specific treatment. More and more scholars have begun to pay attention to its non-drug treatment, among which the commonest technologies for cognitive enhancement of stroke patients include computer cognitive training, virtual reality, non-invasive brain stimulation, and brain-computer interface (6).
Transcranial magnetic stimulation (TMS) is a non-invasive regulatory technique. Its mechanism entails the application of pulsed magnetic fields generated by a coil to selectively enhance or suppress the excitability of the cerebral cortex. This alters neuronal activity and modulates the functional connectivity within the brain network, thereby influencing cognitive and executive functions (7, 8). Most cognitive studies have used the dorsolateral prefrontal cortex (DLPFC) as a stimulating region, which is mainly related to executive and attention functions. In recent years, the therapeutic effects of rTMS on executive function have been investigated, and the possible mechanisms are related to increasing synaptic connections, promoting neuroplasticity, accelerating cerebral blood flow, facilitating neuronal cell growth, and improving local brain metabolism (9). Several studies have shown the potential of TMS in the recovery of executive function of diverse neuropsychiatric diseases. Moser et al. (10) found significant improvements in TMT-B tests in middle-aged and elderly patients with refractory depression after rTMS stimulation; Zheng et al. (11) found that improved executive functioning was associated with biochemical changes in cingulate neurochemistry after high-frequency rTMS in young depressed patients; Cricstancho et al. (12) obtained similarly beneficial results after stimulation of iTBS in depressed patients. Herremans et al. (13) treated alcohol-dependent patients with high-frequency rTMS and found that it stabilized cognitive performance in an executive control task; Ameis et al. (14) found improved executive functioning after rTMS in participants with autism who had more severe deficits in adaptive functioning. In particular, since Rektorova et al. (15) revealed the beneficial effects of high-frequency rTMS on executive function in a blinded crossover trial involving seven patients with post-stroke executive dysfunction, more researchers have begun to focus on the effects of rTMS on executive function with VCI. However, it is worth noting that existing these trials are generally constrained by limited sample sizes, geographical variations, and other factors, leading to non-uniform quality levels. Consequently, further research is imperative to validate these findings. According to the search, existing meta-analyses (16–20) have focused on rTMS for the treatment of cognitive impairment in stroke, with no meta-analysis of rTMS for vascular executive dysfunction now. Therefore, this paper aims to (1) demonstrate the effectiveness of rTMS for the executive function of patients with VCI and (2) further subgroup analyses to assess the effects of multiple factors on the treatment.
2 Data and methods
2.1 Search strategy
The search time is from the establishment of the database to August 23, 2023, regardless of language, location, and other factors. The search databases included Pubmed, Embase, Cochrane Library, CBM, CNKI, and Wanfang. The search terms include transcranial magnetic stimulation, cognitive disorder, executive function, etc.
2.2 Inclusion and exclusion criteria
Inclusion criteria: (1) Randomized controlled trial (RCT); (2) the intervention method of the experimental group was transcranial magnetic stimulation combined with basic treatment, and the intervention method of the control group was non or sham-stimulation combined with the same basic treatment; (3) patients with VCI were treated; (4) outcome indicators include executive function scores. Exclusion criteria: (1) insufficient data; (2) publications; (3) the literature with original data still cannot be found after trying all methods.
2.3 Outcome indicators
There are two outcome indicators to evaluate executive function: one is a screening scale including executive function score, such as the Montreal Cognitive Assessment (MOCA), Mini-mental State Examination (MMSE), the Oxford Cognitive Screen (OCS), etc.; and the other is a specific scale to assess executive function, such as Frontal Assessment Battery (FAB), Trail Making Test (TMT), Stroop test, clock-drawing experiment (CDT), Behavioral Dysexecutive Syndrome (BADS), Tower of London test (TOL), etc. The primary indicators in this paper are the executive scores of the composite scale (designated as Indicator 1) and the total score of the specificity scale (designated as Indicator 2). Additionally, the secondary outcomes were the sub-scores of the specificity scale.
2.4 Literature screening, data extraction, and bias risk assessment
The study was collaboratively conducted by three authors. Two researchers independently screen, data extraction, and bias analysis. In case of disagreements, the third author was responsible for facilitating joint discussions. During the initial screening, we focused on the title and abstract. Subsequently, during the second screening, we read the original text to determine its compliance with the inclusion and exclusion criteria. The extracted data included: (1) basic information of the literature: author, year, etc. (2) subjects: disease type, group, number of cases, etc. (3) intervention methods: stimulating site, frequency, intensity, basic treatment, etc. (4) Outcome indicators: time, scale, data (5) risk of bias results. The risk of bias was assessed according to the Cochrane risk assessment tool (21). This involved seven different types of biases: attrition bias (incomplete outcome data), selection bias (unbiased sequence generation and allocation concealment), reporting bias (selective result reporting), blinding bias (unbiased performance and detection), and other biases. The risk of bias for each element was categorized as low, unknown, or high.
2.5 Statistical analysis
The results of the meta-analysis were processed by Revman 5.3 software (version 5.3; Cochrane Collaboration, Software Update, Oxford, UK). The continuous variables of the same indicator are compared with MD and 95% CI, and different indicators evaluating the same function can be treated with SMD and 95% CI (22). The heterogeneity of results was determined by I2: (1) The fixed-effect model was selected when I2 < 50%, and the random effect model was selected when I2 > 50%;(2) When I2 > 75%, it indicates that the heterogeneity is too high, and sensitivity analysis or subgroup analysis is needed to find the cause. If the heterogeneity cannot be reduced, a system description is performed. Stata software (version 16.01) was used to make funnel plots to evaluate publication bias. Finally, GRADE profiler software was used to evaluate the quality of the meta-analysis results.
3 Results of meta-analysis
3.1 Screening results and methodological quality evaluation results
The screening flow chart is shown in Figure 1. A total of 4,984 articles were retrieved based on the search strategy. After reading the abstract and title, 4,165 articles were excluded, leaving 239 articles for preliminary screening. Finally, after a thorough examination of the full text, 20 articles were included, encompassing a total of 1,049 patients. The risk of bias assessment (Figures 2, 3) unveiled that out of the 20 articles, 11 explicitly described the randomization procedure, while 9 only mentioned randomization without specifying the method. Additionally, 4 articles implemented double-blind, 1 employed a single-blind approach, while the rest did not mention their blinding strategy. Only 1 article among the 20 used allocation concealment. At present, no other risks have been identified. In conclusion, none of the articles manifested high-risk indicators.
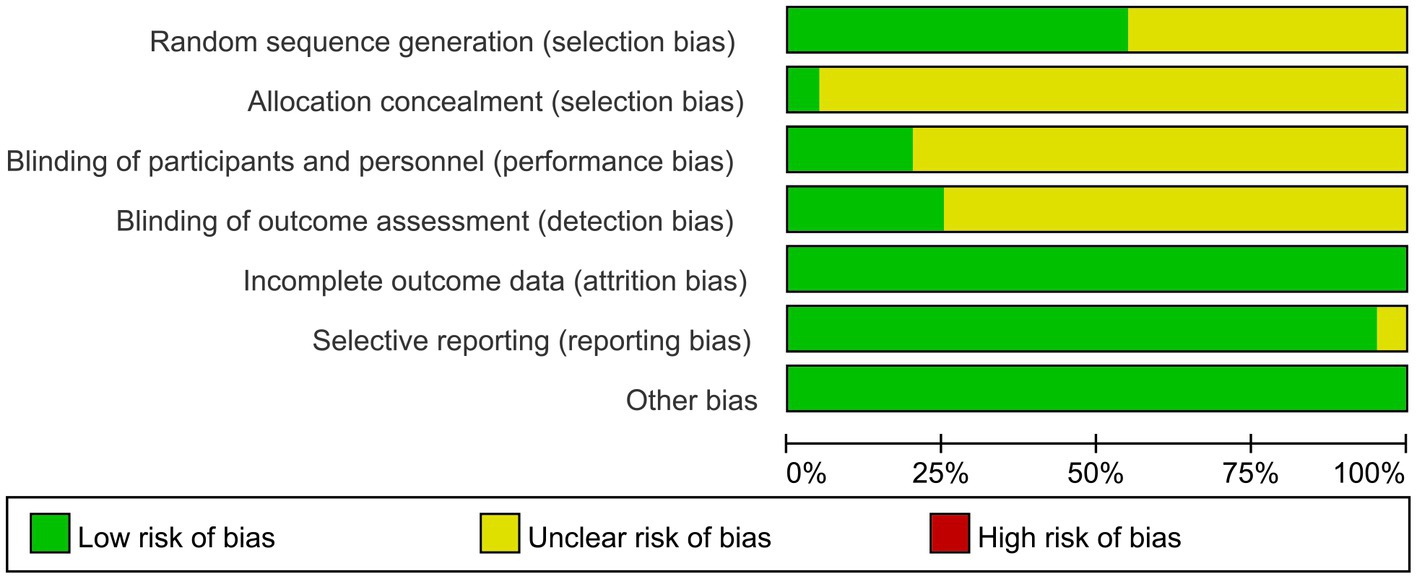
Figure 2. Risk of bias graph: review authors’ judgments about each risk of bias item presented as percentages across all included studies.
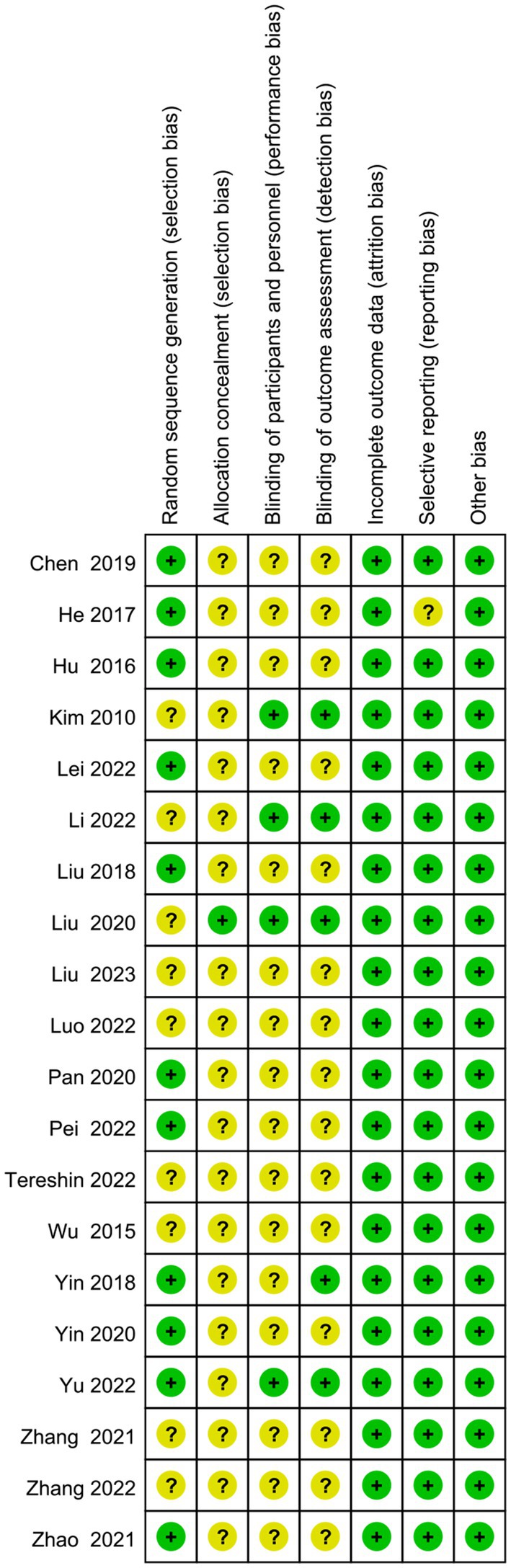
Figure 3. Risk of bias summary: review authors’ judgments about each risk of bias item for each included study.
3.2 Basic features of included studies
The basic characteristics of the 20 literatures are shown in Table 1. Regarding the disease type, 16 articles centered on patients suffering from poststroke cognitive dysfunction. Two articles explored cognitive impairment resulting from small vessel disease, and one article investigated vascular cognitive impairment. Concerning the control group, 10 articles were subjected to sham stimulation, while another 10 articles did not receive any stimulation. Of the stimulus plan, 12 underwent rTMS, coupled with medication and rehabilitation. Eight articles underwent rTMS, along with either medication or rehabilitation. The stimulation intensity ranged from 80 to 100% of the motor threshold (MT), and only one article utilized low-frequency (1 Hz) stimulation. All other articles employed high-frequency stimulation (5 Hz or 10 Hz). Regarding outcomes, 10 articles integrated screening scales that assessed executive function through subitem scores, while 12 articles employed specific scales to evaluate executive function.
3.3 Results of a meta-analysis
3.3.1 Key indicator: executive subitems of the generality scale (indicator 1)
Ten articles (23–32) had executive function scores on the screening scales (Figure 4). Eight (24–28, 30–32) used MOCA-visual–spatial and executive scales, one (29) used MOCA-executive scales, and one (23) employed OCS-executive scales. Following a comprehensive analysis with an I2 value of 14%, a fixed-effects model was employed. The results indicated that the experimental group exhibited superior enhancements in executive function compared to the control group (SMD = 0.93, 95% CI = 0.77–1.08, p < 0.00001).
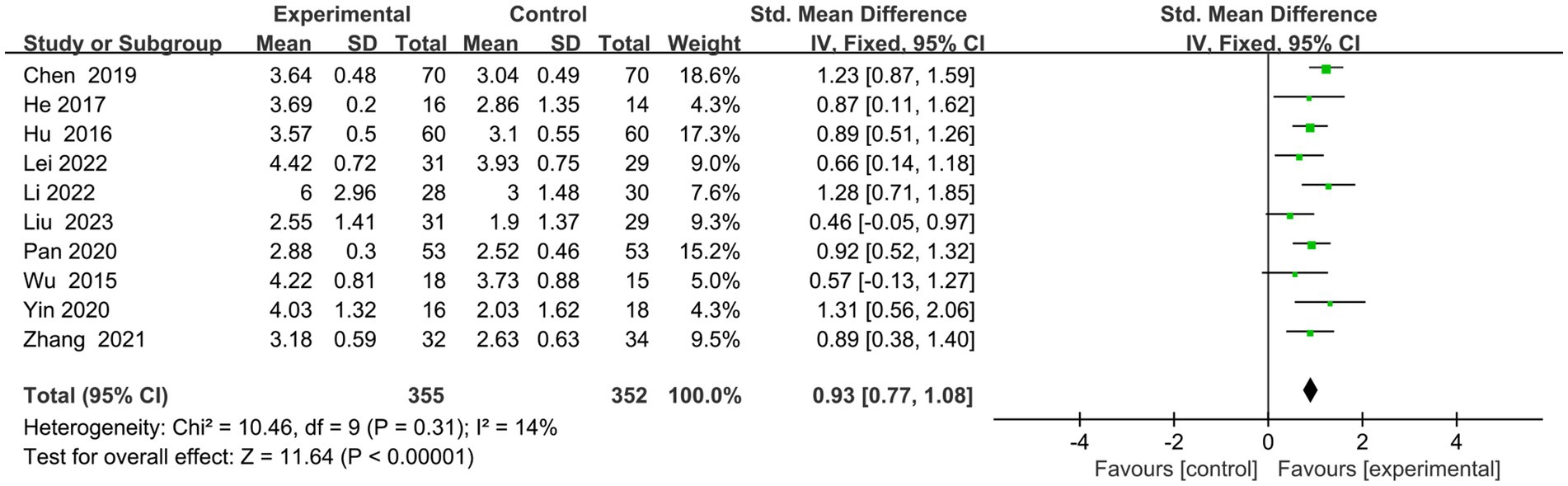
Figure 4. Indicator 1: Frost plot of the executive subscale scores of the Cognitive Comprehensive Scale.
3.3.2 Key indicator: specificity scale for executive functioning (indicator 2)
Five articles (24, 33–36) employed specialized scales to evaluate executive performance (Figure 5). Out of these, two (34, 35) utilized FAB, one (33) utilized the TOL, another (24) involved the CDT, and the last one (36) utilized the BADS. The combined analysis using a random effects model yielded an I2 value of 0%. The findings revealed a significant enhancement in executive function among the transcranial magnetic stimulation group in contrast to the control group (SMD = 0.69, 95% CI = 0.44–0.94, p < 0.00001).
3.3.3 Sub-indicators: sub-items of the specificity indicator
Here we discuss the subitems of two scales (TMT test and Stroop test). (1) The TMT is one of the most commonly used scales for assessing executive function. The TMT-A represents the right cerebral hemisphere’s function, indicating rapid visual search, visuospatial ordering, and perceptual-motor speed abilities. The TMT-B represents the left hemisphere’s function, indicating overall visuospatial scanning, perceptual-motor speed, and stereotypical switching abilities. Four articles (26, 36–39) evaluated the time on TMT-A, and meta results showed in Figure 6A that the rTMS group spent less time on A test (MD = −35.75, 95% CI = −68.37 to −3.12, I2 = 55%, p = 0.03). Two papers (36, 39) evaluated the time of TMT-B. However, owing to high heterogeneity (I2 = 90%) (Figure 6B), both Zhang et al. (39) (MD = −106.15, 95% CI = −157.07 to −55.23) and Zhao et al. (36) (MD = −10.23.95% CI = −40.42 to 19.96)’s experimental group took less time than the control group for qualitative analysis. However, owing to significant heterogeneity (I2 = 90%) (Figure 6B), both Jingjing et al. (39) (MD = −106.15, 95% CI = −157.07 to −55.23) and Jingjing et al. (39) (MD = −10.23.95% CI = −40.42 to 19.96)’s experimental group took less time than the control group for qualitative analysis. (2) There exist various versions of the Stroop test, such as the Symbol Color Word Test (SCWT) and the Visual Stroop Test (VST), which primarily assesses the ability of the execution function to resist interference. Two articles (40, 41) evaluated the correct number of Stroop scale, and the combined analysis found that the correction of the experimental group was better than the control group (SMD =0.49, 95% CI = 0.04 to 0.94, I2 = 0%, p = 0.03) (Figure 6C). Three articles (25, 39, 42) assessed the time to complete card C. meta-analysis showed that the rTMS group took less time than the control group (SMD = −0.46.95% CI = −0.86 to −0.06, I2 = 0%, p = 0.02) (Figure 6D).
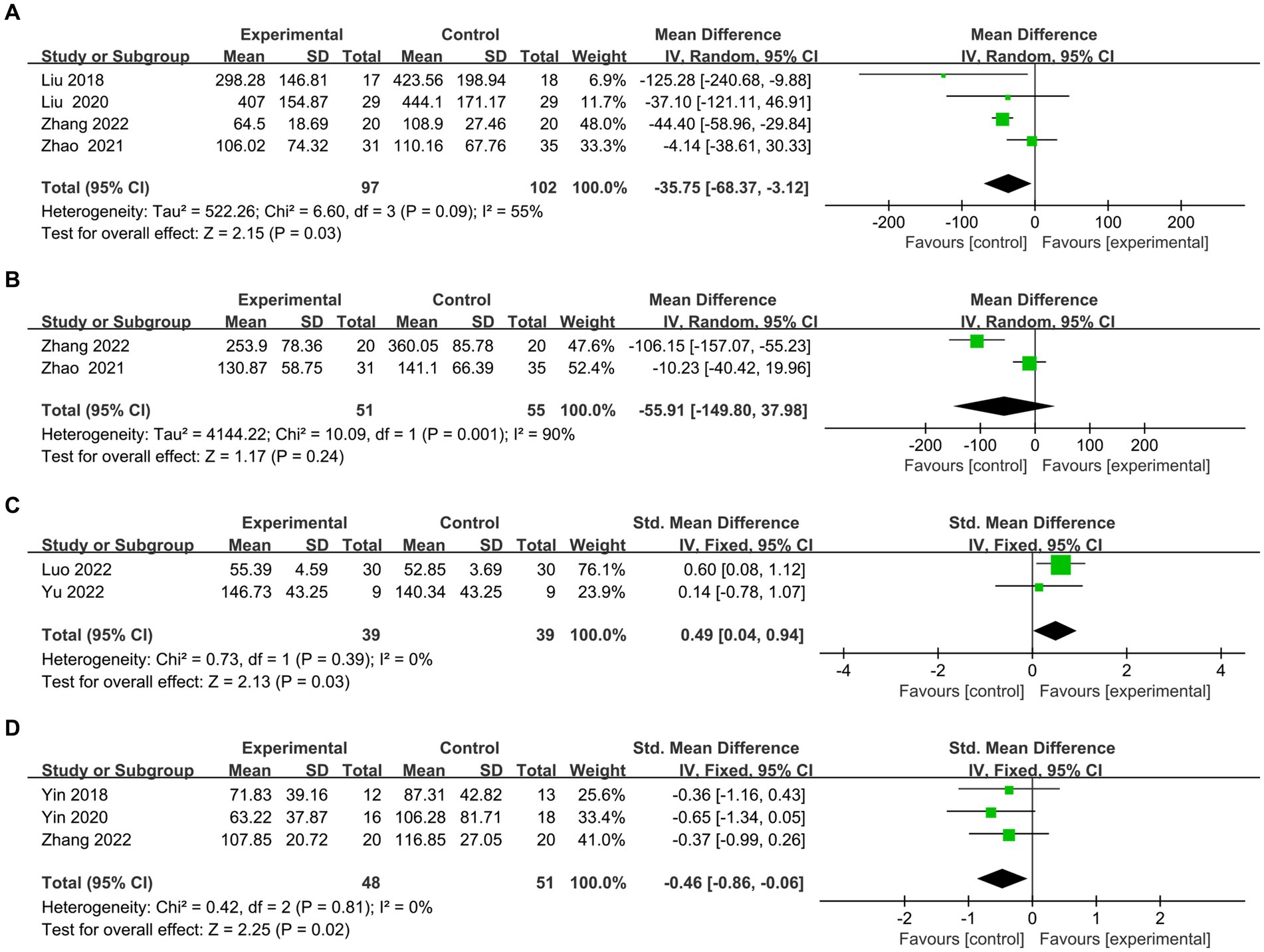
Figure 6. Frost plot of subscale scores of the Executive Specificity Scale. (A) Frost plot of TMT-A. (B) Frost plot of TMT-B. (C) Frost plot of the Stroop-C(n). (D) Frost plot of Stroop-C(t). TMT-A, Trail Making Test-A test; TMT-B, Trail Making Test-B test; Stroop-C(n), correction number of the C card of the Stroop test; Stroop-C(n), time of the C card of the Stroop test.
3.3.4 Subgroup analysis results
Table 2 shows the results of the subgroup analysis of the main indicators from all aspects. The results are as follows: (1) The improvement of rTMS in combination with comprehensive therapy (Indicator 1: SMD, 95% CI = 0.92, 0.72–1.12; Indicator 2: SMD, 95% CI = 1.03, 0.48–1.57) is superior to that in combination with monotherapy (Indicator 1: SMD, 95% CI =0.85, 0.56–1.14; Indicator 2: SMD, 95% CI = 0.60, 0.32–0.88); (2) the effect of intervention time for ≥4 w (Indicator 1: SMD, 95% CI = 0.95, 0.78–1.12; Indicator 2: SMD, 95% CI = 0.74, 0.47–1.00) was superior to that for <4 w (Indicator 1: p = 0.08; Indicator 2: p = 0.22). (3) The application of intermittent theta burst stimulation (iTBS) (SMD, 95% CI = 1.28, 0.71–1.85), a novel therapeutic modality of rTMS, may be superior to traditional rTMS (SMD, 95% CI = 0.90, 0.74–1.06) in improving executive function. (4) Higher frequencies (10 Hz) (p < 0.0001) may demonstrate greater efficacy in executing functions when compared to both low frequency (p = 0.11) and high frequencies of 5 Hz (p = 0.08). (5) Lower-intensity (80%) rTMS (SMD, 95% CI = 0.90, 0.49–1.32) may be better than higher-intensity (SMD, 95% CI = 0.87.0.65–1.08) for improving executive functioning. Overall, it is possible that a combination of comprehensive therapy, intervention for a longer period (>4w), higher frequency (10 Hz), lower intensity (80%), and a specific type (iTBS), may have a better effect on improving the executive function of VCI.
3.4 Publication bias and grade quality evaluation
To evaluate publication bias, we conducted an analysis using funnel plots (Figure 7) and the Egger test. The results demonstrated a symmetrical distribution on both sides of the funnel plots and a p-value of 0.635 on the Egger test, indicating the absence of publication bias. Furthermore, a sensitivity analysis was carried out for each indicator, and the results consistently demonstrated stability. Ultimately, the quality assessment of the meta-analysis conducted in this study employed the GRADE approach. The evaluation revealed that our primary outcomes, namely the screening scale sub-score (indicator 1) and specificity scale total score (indicator 2), exhibited high quality. Meanwhile, the remaining outcomes, such as TMT-A and Stroop scales (time and correction number), displayed a moderate quality.
4 Discussion
Executive function is a high-level cognitive function with core components of abstract thinking, working memory, stereotype transfer, and reflective inhibition (43, 44). Executive dysfunction is the core symptom of VCI, which seriously affects the prognosis of stroke and poses a significant clinical challenge. Scholars are increasingly focused on this issue, highlighting the critical need to find an effective remedy (45). Studies have found that rTMS shows great potential in the treatment of executive function in VCI. The pulsed magnetic field of rTMS influences brain tissues (46), affecting synaptic structure (47–49), neurotransmitters (50, 51), brain-derived trophic factors (50, 52, 53), regulating neuronal excitatory gene expression (52, 54, 55), and altering neuronal membrane potentials (56, 57). The neurobiological mechanisms underlying the cognitive-enhancing effects of rTMS are not fully understood. The primary mechanism involves cortical plasticity, specifically the change of long-term potentiation (LTP) and long-term depression (LTD) (58–60). Stroke recovery relies on two competing neuroplastic remodeling mechanisms: interhemispheric competition and compensation. rTMS can enhance excitability in the affected hemisphere or modulate activity in the unaffected hemisphere (61). Animal studies employing rTMS for VCl have revealed changes in hippocampal synaptic morphologies, increased LTD induction, and heightened synaptic plasticity (52, 56, 62). The second manifestation involves an impact on the cholinergic system (63, 64). Acetylcholine (Ach) serves as an excitatory neurotransmitter crucial for learning and memory functions. Zhang et al. (50) applied rTMS (0.5 Hz, 1.33 T) to rats with vascular dementia (VD) over 30 days. They noted a substantial rise in the activities of acetylcholinesterase (AchE) and choline acetyltransferase (ChAT), an augmentation in neuronal cholinergic density, and a notable amelioration of learning and memory impairments in VD rats. Furthermore, apoptosis stands as the predominant cause of neuronal demise, notably in ischemic regions. Animal studies have demonstrated that rTMS exhibits anti-apoptotic and neuroprotective properties. By stimulating the PI3K/Akt/mTOR pathway, rTMS hinders apoptosis in hippocampal neurons (53). Guo et al. (65) identified significantly elevated levels of BDNF, TrkB, and the anti-apoptotic protein Bcl-2 in the ischemic hippocampus. In addition, astrocytes possess the capacity to shield brain tissue and mitigate disability stemming from ischemic brain disorders. rTMS (10 Hz) can enhance cognitive function by mitigating white matter lesions, reducing pro-inflammatory cytokine levels, elevating anti-inflammatory cytokine levels, and inducing the transformation of microglial cells into the M2 phenotype (66). These process influences the activity and functional connectivity of specific brain regions, potentially serving as one of the mechanisms to regulate functional brain activity and enhance executive function.
At present, rTMS is still being explored for its therapeutic applicability in the executive function of VCI. While numerous RCTs have been conducted, a comprehensive meta-analysis on this topic is yet to be established. Based on this, this study aims to fill this research gap by incorporating a total of 20 RCTs that investigate the efficacy of rTMS in improving executive function following VCI. The conclusions of the meta-analysis and systematic review are as follows (1) rTMS can improve the executive function of VCI; (2) Subgroup analyses showed that higher frequency, lower intensity, iTBS, combined comprehensive therapy, and longer interventions yielded better gains. The meta-results are consistent with the meta-analysis results of Gao et al. (18) and Han et al. (20). However, unlike these studies, they only incorporated two articles as part of their cognitive outcome analysis without conducting a comprehensive analysis. This paper addresses this limitation by providing more extensive evidence with 20 RCTs and full indicators. Alongside the inclusion of the 20 RCTs, it is noteworthy to mention that Rectorova et al. (15) conducted a well-designed crossover, sham-controlled trial in 2005. This study focused on the application of rTMS for improving executive impairment following stroke (referred to as PSEI). The results indicated that stimulating the left DLPFC led to notable improvements in patients’ problem-solving ability, as assessed by the TMT. However, the efficacy in terms of memory function and verbal fluency was found to be insignificant. In 2008, Sedlackva et al. (67) expanded their study to include the MCl-V of seven patients as a before-and-after control trial, confirming that rTMS had an equivalent effect on this subgroup. Additionally, Aref (68) conducted a study involving 40 patients to investigate the efficacy of high-frequency rTMS in treating cerebral small-vessel disease. The results from their RCT indicated that the FAB scores of the true-stimulation group were significantly higher than those of the sham-stimulation group. However, the findings were not incorporated into the present meta-analysis due to the lack of post-treatment data. Furthermore, Yuanwen et al. (38) conducted another RCT, concluding that rTMS treatment for PSEI led to improved WCST scores. Nonetheless, this study was excluded from the meta-analysis due to inconsistencies in comparison to other RCTs included in this meta-analysis. With the help of functional near-infrared spectroscopy (fNIRS), a recent study by Liu et al. (69) observed individuals with post-stroke executive impairment following rTMS activation of the left DLPFC. The researchers discovered that the time and number of error scores of the Stroop test were significantly higher in these patients, which correlated with the enhanced functional connectivity between various brain regions. Notably, this activation involved the left DLPFC, the right PMC, and the right SM1 brain regions. These trials all further support the conclusions of this article.
The potential of rTMS in treating executive function after VCI is immense, and as the evidence for its effectiveness grows, the need for further optimization becomes increasingly crucial. Currently, there is no uniform clinical standard for the treatment mode. The most common stimulation site for rTMS is DLPFC, with an intensity of 80% ~ 120% motor threshold (MT), a frequency of 5 ~ 25 Hz, and time intervals of 20 ~ 30 s. The treatment duration typically extends 3 weeks or longer (70). Numerous factors, including stimulation site, combined modalities, intervention timing, and stimulation parameters, influence the therapeutic outcomes of rTMS. Hence, this paper focuses on subgroup analysis to deepen the primary findings. In terms of stimulation sites, it is known that functional changes in the DLPFC can lead to cognitive and executive deficits (71). Since the patient is right-handed, the current RCT primarily targets the left DLPFC, with only one article in this paper examining the healthy DLPFC, which reduces heterogeneity. In terms of the combination modality of rTMS, subgroup analyses showed that rTMS combined with comprehensive therapy was superior to combined with monotherapy, suggesting that rTMS can add to cognitive routine treatment. Previous studies (72, 73) have also shown that rTMS in combination with other therapies for treating executive dysfunction is a significant trend for the future. In terms of intervention time, the results for both primary indicators 1 and 2 showed better improvement in executive functioning of ≥4 weeks, probably due to the cumulative effect of stimuli. Regarding stimulation type, iTBS is a unique type of rTMS characterized by a 50 Hz pulse with 3 pulses administered every 200 ms (7). Previous studies have confirmed that iTBS has superior effects on cognitive function than conventional rTMS (60, 61), which is consistent with the results of the subgroup analyses in this paper. Concerning stimulation frequency, current clinical research results mainly focus on high-frequency rTMS (10 Hz) to treat the executive function of VCI. 5 Hz high frequency or 1 Hz low frequency may not be effective, but numerous studies have found that low-frequency rTMS can improve cognitive function and enhance neuroplasticity (45). This paper has fewer articles on lower frequencies (one at 5 Hz, one at 1 Hz). Due to the limitations of sample size, their effectiveness needs further verification through original experiments. Regarding stimulation intensity, subgroup analyses showed that lower intensities (80%MT) may be more effective. Zhang et al. (74) explored prefrontal optimal intensity with the fNLRS and found that intensity was nonlinear with prefrontal cortical blood flow and that a 70% MT effect may be better than a 100% effect. However, the interactions between the parameters are not yet clear and need to be further explored in the future.
The therapeutic efficacy of rTMS is influenced by a variety of factors, such as age, sex, handedness, infarct area and size, cardiovascular risk factors, and education level (73). Age is an independent risk factor for executive dysfunction. The results of the study by Yaning et al. (75) showed that, in comparison to younger age groups, showed that, in comparison to younger age groups, older adults had poorer executive scores. Additionally, Mally et al. (76) reported executive function declines and rTMS therapy effects wear off faster in individuals 65 and up. Gender-specific prognoses have been observed in VCI (77). Cantone et al. (78) found that men with mild VCI had worse cognitive and functional status compared with women. This study is the first to emphasize gender-specific alterations in intracortical and corticospinal excitability following rTMS. Executive function primarily stimulates left DLPFC, as confirmed by related research. However, 21.4% of right-handed individuals have their DLPFC located mainly in the right hemisphere rather than the presumed left hemisphere, and 16.7% of left-handed individuals have their DLPFC located mainly in the left hemisphere (79). This shows that a scanner for localizing the potential DLPFC for precise stimulation also significantly impacts prognosis. Brain regions associated with executive regions include the frontal-striatal loops and the cerebellum, and injuries to these regions result in more severe impairments in executive function, which can affect the therapeutic efficacy of rTMS (80). There are no original studies on these relevant factors, and further exploration is necessary. Currently, RCT stimulation sites are mostly limited to the DLPFC, but stimulating the M1 area has been demonstrated to effectively enhance cognitive capabilities. Experiments involving rTMS stimulation of the bilateral DLPFC have yielded favorable outcomes (81). Diseases are characterized by damage to multiple networks and stimulation of other regions may also cause cognitive enhancement (82). The exact mechanism remains uncertain. It is proposed that targeting different stimulation sites such as the precuneus, parietal region, or cerebellum could improve executive dysfunction in the future.
In conclusion, this meta included 20 RCTs of executive function in patients with VCI treated by rTMS. It not only provides comprehensive evidence of its efficacy but also has some limitations: (1) the RCT has a range of subjective scales and no objective indications, which increases the heterogeneity of indicators; (2) there is less literature in foreign languages (7 foreign papers of 20 papers in this meta-analysis), which is probably related to the absence of rTMS in the latest guidelines for post-stroke cognitive dysfunction (83). So there is a greater need for further studies to promote the application of the study.
5 Conclusion
rTMS is effective in the treatment of the executive function of VCI. Higher frequency, lower intensity, iTBS, combined comprehensive therapies, and longer interventions are more effective. There are some limitations in this study, and further exploration requires more objective metrics and optimal parameters to enhance its application.
Data availability statement
The original contributions presented in the study are included in the article/supplementary material, further inquiries can be directed to the corresponding author.
Author contributions
XW: Data curation, Formal analysis, Methodology, Software, Writing – original draft. QD: Conceptualization, Software, Writing – review & editing. YuL: Resources, Supervision, Writing – review & editing. TL: Resources, Supervision, Writing – review & editing. YaL: Data curation, Investigation, Writing – review & editing. JY: Investigation, Project administration, Writing – review & editing. WZ: Funding acquisition, Project administration, Writing – review & editing.
Funding
The author(s) declare financial support was received for the research, authorship, and/or publication of this article. This paper was funded by the Henan Science and Technology Project (232102310266) and the Henan Province Medical Science and Technology Research Program (SBGJ202102037).
Acknowledgments
We would like to express our appreciation to the experts for editing and proofreading this manuscript.
Conflict of interest
The authors declare that the research was conducted in the absence of any commercial or financial relationships that could be construed as a potential conflict of interest.
Publisher’s note
All claims expressed in this article are solely those of the authors and do not necessarily represent those of their affiliated organizations, or those of the publisher, the editors and the reviewers. Any product that may be evaluated in this article, or claim that may be made by its manufacturer, is not guaranteed or endorsed by the publisher.
Footnotes
References
1. Ferri, CP, Prince, M, Brayne, C, Brodaty, H, Fratiglioni, L, Ganguli, M, et al. Global prevalence of dementia: a Delphi consensus study. Lancet. (2005) 366:2112–7. doi: 10.1016/S0140-6736(05)67889-0
2. Iadecola, C, Duering, M, Hachinski, V, Joutel, A, Pendlebury, ST, Schneider, JA, et al. Vascular Cognitive Impairment And dementia. J Am Coll Cardiol. (2019) 73:3326–44. doi: 10.1016/j.jacc.2019.04.034
3. van der Flier, WM, Skoog, I, Schneider, JA, Pantoni, L, Mok, V, Chen, CLH, et al. Vascular cognitive impairment. Nat Rev Dis Prim. (2018) 4:18003. doi: 10.1038/nrdp.2018.3
4. Hosoki, S, Hansra, GK, Jayasena, T, Poljak, A, Mather, KA, Catts, VS, et al. Molecular biomarkers for vascular cognitive impairment and dementia. Nat Rev Neurol. (2023) 19:737–53. doi: 10.1038/s41582-023-00884-1
5. Xiaodi, D, Binbin, D, Lijun, D, Jie, Q, Lei, Z, and Rubo, S. Changes of cerebellar white matter fiber bundles in vascular cognitive impairment patients. China Acad. J. Electr. Publish. House. (2021) 23:394–8.
6. Draaisma, LR, Wessel, MJ, and Hummel, FC. Neurotechnologies as tools for cognitive rehabilitation in stroke patients. Expert Rev Neurother. (2020) 20:1249–61. doi: 10.1080/14737175.2020.1820324
7. Lefaucheur, JP, Aleman, A, Baeken, C, Benninger, DH, Brunelin, J, Di Lazzaro, V, et al. Evidence-based guidelines on the therapeutic use of repetitive transcranial magnetic stimulation (rTMS): An update (2014–2018). Clin Neurophysiol. (2020) 131:474–528. doi: 10.1016/j.clinph.2019.11.002
8. Valero-Cabre, A, Amengual, JL, Stengel, C, Pascual-Leone, A, and Coubard, OA. Transcranial magnetic stimulation in basic and clinical neuroscience: a comprehensive review of fundamental principles and novel insights. Neurosci Biobehav Rev. (2017) 83:381–404. doi: 10.1016/j.neubiorev.2017.10.006
9. Siebner, H, Peller, M, Bartenstein, P, Willoch, F, Rossmeier, C, Schwaiger, M, et al. Activation of frontal premotor areas during Suprathreshold transcranial magnetic stimulation of the left primary sensorimotor cortex: a glucose metabolic pet study. Hum Brain Mapp. (2001) 12:157–67. doi: 10.1002/1097-0193(200103)12:3<157::aid-hbm1012>3.0.co;2-v
10. Moser, DJ, Jorge, RE, Manes, F, Paradiso, S, Benjamin, ML, and Robinson, RG. Improved executive functioning following repetitive transcranial magnetic stimulation. Neurology. (2002) 58:1288–90. doi: 10.1212/wnl.58.8.1288
11. Zheng, H, Jia, F, Guo, G, Quan, D, Li, G, Wu, H, et al. Abnormal anterior cingulate N-Acetylaspartate and executive functioning in treatment-resistant depression after rTMS therapy. Int J Neuropsychopharmacol. (2015) 18:pyv059. doi: 10.1093/ijnp/pyv059
12. Cristancho, P, Arora, J, Nishino, T, Berger, J, Carter, A, Blumberger, D, et al. A pilot randomized sham controlled trial of bilateral Itbs for depression and executive function in older adults. Int J Geriatr Psychiatry. (2023) 38:e5851. doi: 10.1002/gps.5851
13. Herremans, SC, Vanderhasselt, MA, De Raedt, R, and Baeken, C. Reduced intra-individual reaction time variability during a go-Nogo task in detoxified alcohol-dependent patients after one right-sided dorsolateral prefrontal Hf-rTMS session. Alcohol Alcohol. (2013) 48:552–7. doi: 10.1093/alcalc/agt054
14. Ameis, SH, Blumberger, DM, Croarkin, PE, Mabbott, DJ, Lai, MC, Desarkar, P, et al. Treatment of executive function deficits in autism spectrum disorder with repetitive transcranial magnetic stimulation: a double-blind, sham-controlled, pilot trial. Brain Stimul. (2020) 13:539–47. doi: 10.1016/j.brs.2020.01.007
15. Rektorova, I, Megova, S, Bares, M, and Rektor, I. Cognitive functioning after repetitive transcranial magnetic stimulation in patients with cerebrovascular disease without dementia: a pilot study of seven patients. J Neurol Sci. (2005) 229-230:157–61. doi: 10.1016/j.jns.2004.11.021
16. Dawar, D, Kamalakannan, S, Chawla, N, Mathew, J, Mehmood, E, Bhatnagar, U, et al. A scoping review of recent advancements in intervention and outcome measures for post-stroke cognitive impairments. Ann Indian Acad Neurol. (2023) 26:S32–42. doi: 10.4103/aian.aian_1099_21
17. Liu, M, Bao, G, Bai, L, and Yu, E. The role of repetitive transcranial magnetic stimulation in the treatment of cognitive impairment in stroke patients: a systematic review and meta-analysis. Sci Prog. (2021) 104:368504211004266. doi: 10.1177/00368504211004266
18. Gao, Y, Qiu, Y, Yang, Q, Tang, S, Gong, J, Fan, H, et al. Repetitive transcranial magnetic stimulation combined with cognitive training for cognitive function and activities of daily living in patients with post-stroke cognitive impairment: a systematic review and meta-analysis. Ageing Res Rev. (2023) 87:101919. doi: 10.1016/j.arr.2023.101919
19. Gong, C, Hu, H, Peng, XM, Li, H, Xiao, L, Liu, Z, et al. Therapeutic effects of repetitive transcranial magnetic stimulation on cognitive impairment in stroke patients: a systematic review and meta-analysis. Front Hum Neurosci. (2023) 17:1177594. doi: 10.3389/fnhum.2023.1177594
20. Han, K, Liu, J, Tang, Z, Su, W, Liu, Y, Lu, H, et al. Effects of excitatory transcranial magnetic stimulation over the different cerebral hemispheres dorsolateral prefrontal cortex for post-stroke cognitive impairment: a systematic review and meta-analysis. Front Neurosci. (2023) 17:1102311. doi: 10.3389/fnins.2023.1102311
21. Sterne, JAC, Savović, J, Page, MJ, Elbers, RG, Blencowe, NS, Boutron, I, et al. ROB 2: a revised tool for assessing risk of bias in randomised trials. BMJ (Clin Res Ed). (2019) 366:l4898. doi: 10.1136/bmj.l4898
22. Murad, MH, Wang, Z, Chu, H, and Lin, L. When continuous outcomes are measured using different scales: guide for meta-analysis and interpretation. BMJ (Clin Res Ed). (2019) 364:k4817. doi: 10.1136/bmj.k4817
23. Li, W, Wen, Q, Xie, YH, Hu, AL, Wu, Q, and Wang, YX. 3.1 improvement of Poststroke cognitive impairment by intermittent Theta bursts: a double-blind randomized controlled trial. Brain Behav. (2022) 12:e2569. doi: 10.1002/brb3.2569
24. Pan, L, Li, X, Lu, X, Yang, Z, Meng, Y, Qie, H, et al. Beneficial effects of repetitive transcranial magnetic stimulation on cognitive function and self-care ability in patients with non-dementia vascular cognitive impairment. Int J Clin Exp Med. (2020) 13:3197–204.
25. Yin, M, Liu, Y, Zhang, L, Zheng, H, Peng, L, Ai, Y, et al. Effects of rTMS treatment on cognitive impairment and resting-state brain activity in stroke patients: a randomized clinical trial. Front Neural Circuits. (2020) 14:563777. doi: 10.3389/fncir.2020.563777
26. Yugong, H, and Qing, Z. Effect of repetitive transcranial magnetic stimulation on non-dementia-type vascular cognitive dysfunction. China J Phys Med Rehabil. (2017) 39:464–6. doi: 10.3760/CMA.j.issn.0254-1424.2017.06.018
27. Xujia, L, Lu, Z, Yang, X, and Xueyong, L. Effect of repetitive transcranial magnetic stimulation combined with scalp acupuncture in patients with post-stroke cognitive impairment. J China Med Univ. (2023) 52:115–9. doi: 10.12007/j.issn.0258-4646.2023.02.004
28. Wei, W, Xiaohui, Z, Huaying, T, and Rong, X. The clinical research of the repetitive transcranial magnetic stimulation (rTMS) treatment on VCIND cerebral infarction patient. Chinese J. Gen. Pract. (2015) 13:356–357, 514.
29. Guangyu, Z, Guo, L, and Shan, Y. Transcranial magnetic stimulation combined with oxiracetam improves cognitive impairment in CIS patients. China J Geriatr Heart Brain Vessel Dis. (2021) 23:735–7. doi: 10.3969/j.issn.1009-0126.2021.07.017
30. Luoman, H, Qixiu, Z, Yunxia, L, Yanmin, L, Naisu, T, Wenhua, S, et al. The therapeutic effects of supplementing rehabilitation with repetitive transcranial magnetic stimulation in treating vascular cognitive impairment but no dementia. China J Phys Med Rehabil. (2016) 38:278–82. doi: 10.3760/cma.j.issn.0254-1424.2016.04.009
31. Zhengyi, C, Jianqiu, G, Yuefeng, W, Renxiang, P, Yiting, S, Wenwen, N, et al. Effect of repeated transcranial magnetic stimulation combined with cognitive rehabilitation training on cognitive impairment after stroke. China J Phys Med Rehabil. (2019) 41:199–201. doi: 10.3760/cma.j.issn.0254-1424.2019.03.008
32. Xiaolin, L, Yanjun, D, Danjuan, Z, and Qixuan, S. Electroacupuncture combined with repetitive transcranial magnetic stimulation in the treatment of post stroke cognitive impairment. Acta Chinese Med. (2021) 36:2455–8. doi: 10.16368/j.issn.1674-8999.2021.11.508
33. Kim, BR, Kim, DY, Chun, MH, Yi, JH, and Kwon, JS. Effect of repetitive transcranial magnetic stimulation on cognition and mood in stroke patients: a double-blind, sham-controlled trial. Am J Phys Med Rehabil. (2010) 89:362–8. doi: 10.1097/PHM.0b013e3181d8a5b1
34. Tereshin, AE, Kiryanova, VV, Reshetnik, DA, Karyagina, MV, Konstantinov, KV, Lapin, SV, et al. The effect of non-invasive brain stimulation on neuroplasticity in the early recovery period after ischemic stroke. Vopr Kurortol Fizioter Lech Fiz Kult. (2022) 99:5–12. doi: 10.17116/kurort2022990515
35. Song, P, Jian, W, and Jiayi, X. Effect of repetitive transcranial magnetic intermittent θ burst stimulation on post-stroke cognitive impairment. Chongqing Med. (2022) 51:3120–5. doi: 10.3969/j.issn.1671-8348.2022.18.012
36. Defu, Z, Jun, J, Qi, F, Qingqing, Z, Shengjia, N, and Min, S. Repetitive transcranial magnetic stimulation combined with upper limb robot virtual scenario training for stroke patients with cognitive impairment. Chinese J Rehabil. (2020) 35:295–8. doi: 10.3870/zgkf.2020.06.004
37. Liu, Y, Yin, M, Luo, J, Huang, L, Zhang, S, Pan, C, et al. Effects of transcranial magnetic stimulation on the performance of the activities of daily living and attention function after stroke: a randomized controlled trial. Clin Rehabil. (2020) 34:1465–73. doi: 10.1177/0269215520946386
38. Yuanwen, L, Jie, F, Rongrong, J, Nan, H, Cuihuan, P, Zhengmao, Y, et al. Effects of high frequency repetitive transcranial magnetic stimulation on executive function in patients after stroke. Chin J Neurol. (2017) 50:745–50. doi: 10.3760/cma.j.issn.1006-7876.2017.10.006
39. Jingjing, Z, Lingli, W, Dandan, C, Jieyin, R, Qian, L, and Yan, L. The effect of high-frequency repetitive transcranial magnetic stimulation on cognitive function after stroke based on electroencephalogram nonlinear analysis. J Chongqing Med Univ. (2022) 47:762–7. doi: 10.13406/j.cnki.cyxb.003059
40. Yu, H, Liu, S, Dai, P, Wang, Z, Liu, C, and Zhang, H. Effects of repetitive transcranial magnetic stimulation on gait and postural control ability of patients with executive dysfunction after stroke. Brain Sci. (2022) 12:1185. doi: 10.3390/brainsci12091185
41. Yali, L, Jinsong, C, Jie, B, Shasha, C, and Shuang, L. Effect of transcranial direct current stimulation combined with repeated transcranial magnetic stimulation on post-stroke cognitive impairment. Chinese J Pract Nerv Dis. (2022) 25:1203–9. doi: 10.12083/SYSJ.220974
42. Mingyu, Y, Jing, L, Xiquan, H, Qinglin, X, Li, H, Shuxian, Z, et al. Effects of high frequency repetitive transcranial magnetic stimulation on post-stroke cognitive impairment. Chinese J Rehabil Med. (2018) 33:763–9. doi: 10.3969/j.issn.1001-1242.2018.07.003
43. Hsu, FC, Raffield, LM, Hugenschmidt, CE, Cox, A, Xu, J, Carr, JJ, et al. Relationships between cognitive performance, neuroimaging and vascular disease: the DHS-mind study. Neuroepidemiology. (2015) 45:1–11. doi: 10.1159/000435775
44. O'Brien, JT, and Thomas, A. Vascular dementia. Lancet. (2015) 386:1698–706. doi: 10.1016/s0140-6736(15)00463-8
45. Yuanwen, L, Cuihuan, P, Nan, H, Yan, C, and Jie, F. Advance in repetitive transcranial magnetic stimulation for post-stroke executive impairment (review). Chinese J Rehabil Theory Pract. (2016) 22:1132–6. doi: 10.3969/j.issn.1006-9771.2016.10.004
46. Zhu, H, Ding, C, Li, Y, and Xu, G. Repetitive transcranial magnetic stimulation significantly improves cognitive impairment and neuronal excitability during aging in mice. Sheng Wu Yi Xue Gong Cheng Xue Za Zhi. (2020) 37:380–8. doi: 10.7507/1001-5515.201905072
47. Xiaoqiao, Z, Li, L, and Guoping, M. Effects of repetitive transcranial magnetic stimulation on learning, memory and dendrite morphology in the hippocampus. Chinese J Phys Med Rehabil. (2014) 36:2–6. doi: 10.3760/cma.j.issn.0254-1424.2014.01.002
48. Yang, HY, Liu, Y, Xie, JC, Liu, NN, and Tian, X. Effects of repetitive transcranial magnetic stimulation on synaptic plasticity and apoptosis in vascular dementia rats. Behav Brain Res. (2015) 281:149–55. doi: 10.1016/j.bbr.2014.12.037
49. Hu, W, Yang, W, Han, WH, and Zhu, H. Effects of Electroacupuncture combined with transcranial magnetic stimulation on learning-memory ability of rats with vascular dementia. Chinese J Cerebrovasc Dis. (2021) 18:458–64. doi: 10.3969/j.issn.1672-5921.2021.07.005
50. Zhang, XQ, Li, L, Huo, JT, Cheng, M, and Li, LH. Effects of repetitive transcranial magnetic stimulation on cognitive function and cholinergic activity in the rat Hippocampus after vascular dementia. Neural Regen Res. (2018) 13:1384–9. doi: 10.4103/1673-5374.235251
51. Wang, F, Zhang, C, Hou, S, and Geng, X. Synergistic effects of mesenchymal stem cell transplantation and repetitive transcranial magnetic stimulation on promoting autophagy and synaptic plasticity in vascular dementia. J Gerontol A Biol Sci Med Sci. (2019) 74:1341–50. doi: 10.1093/gerona/gly221
52. Wang, F, Geng, X, Tao, HY, and Cheng, Y. The restoration after repetitive transcranial magnetic stimulation treatment on cognitive ability of vascular dementia rats and its impacts on synaptic plasticity in hippocampal Ca1 area. J Mol Neurosci. (2010) 41:145–55. doi: 10.1007/s12031-009-9311-7
53. Wang, F, Chang, GM, Yu, Q, and Geng, X. The neuroprotection of repetitive transcranial magnetic stimulation pre-treatment in vascular dementia rats. J Mol Neurosci. (2015) 56:198–204. doi: 10.1007/s12031-014-0480-7
54. Hong, J, Chen, J, Zeng, Y, Zhang, X, Xie, M, Li, C, et al. Different combinations of high-frequency rTMS and cognitive training improve the cognitive function of cerebral ischemic rats. Brain Res Bull. (2021) 175:16–25. doi: 10.1016/j.brainresbull.2021.07.012
55. Hong, J, Chen, J, Li, C, An, D, Tang, Z, and Wen, H. High-frequency rTMS improves cognitive function by regulating synaptic plasticity in cerebral ischemic rats. Neurochem Res. (2021) 46:276–86. Epub 2020/11/03. doi: 10.1007/s11064-020-03161-5
56. Zhang, N, Xing, M, Wang, Y, Tao, H, and Cheng, Y. Repetitive transcranial magnetic stimulation enhances spatial learning and synaptic plasticity via the VEGF and BDNF-NMDAR pathways in a rat model of vascular dementia. Neuroscience. (2015) 311:284–91. doi: 10.1016/j.neuroscience.2015.10.038
57. Liu, S, Wang, X, Ma, J, Wang, K, Wang, Z, Li, J, et al. Effect of low-frequency repetitive transcranial magnetic stimulation on executive function and its neural mechanism: An event-related potential study. Front Neurosci. (2021) 15:701560. doi: 10.3389/fnins.2021.701560
58. Koch, G, Esposito, Z, Kusayanagi, H, Monteleone, F, Codecá, C, Di Lorenzo, F, et al. Csf tau levels influence cortical plasticity in Alzheimer's disease patients. J Alzheimers Dis. (2011) 26:181–6. doi: 10.3233/JAD-2011-110116
59. Starosta, M, Cichoń, N, Saluk-Bijak, J, and Miller, E. Benefits from repetitive transcranial magnetic stimulation in post-stroke rehabilitation. J Clin Med. (2022) 11:2149. doi: 10.3390/jcm11082149
60. Li, X, Qi, G, Yu, C, Lian, G, Zheng, H, Wu, S, et al. Cortical plasticity is correlated with cognitive improvement in Alzheimer's disease patients after rTMS treatment. Brain Stimul. (2021) 14:503–10. doi: 10.1016/j.brs.2021.01.012
61. Sheng, R, Chen, C, Chen, H, and Yu, P. Repetitive transcranial magnetic stimulation for stroke rehabilitation: insights into the molecular and cellular mechanisms of neuroinflammation. Front Immunol. (2023) 14:1197422. doi: 10.3389/fimmu.2023.1197422
62. Shang, Y, Wang, X, Li, F, Yin, T, Zhang, J, and Zhang, T. rTMS ameliorates prenatal stress–induced cognitive deficits in male-offspring rats associated with BDNF/TrkB signaling pathway. Neurorehabil Neural Repair. (2019) 33:271–83. doi: 10.1177/1545968319834898
63. Bonni, S, Ponzo, V, Di Lorenzo, F, Caltagirone, C, and Koch, G. Real-time activation of central cholinergic circuits during recognition memory. Eur J Neurosci. (2017) 45:1485–9. doi: 10.1111/ejn.13588
64. Martorana, A, Di Lorenzo, F, Manenti, G, Semprini, R, and Koch, G. Homotaurine induces measurable changes of short latency afferent inhibition in a group of mild cognitive impairment individuals. Front Aging Neurosci. (2014) 6:254. doi: 10.3389/fnagi.2014.00254
65. Guo, F, Lou, J, Han, X, Deng, Y, and Huang, X. Repetitive transcranial magnetic stimulation ameliorates cognitive impairment by enhancing neurogenesis and suppressing apoptosis in the Hippocampus in rats with ischemic stroke. Front Physiol. (2017) 8:559. doi: 10.3389/fphys.2017.00559
66. Hong, Y, Liu, Q, Peng, M, Bai, M, Li, J, Sun, R, et al. High-frequency repetitive transcranial magnetic stimulation improves functional recovery by inhibiting neurotoxic polarization of astrocytes in ischemic rats. J Neuroinflammation. (2020) 17:150. doi: 10.1186/s12974-020-01747-y
67. Sedlackova, S, Rektorova, I, Fanfrdlova, Z, and Rektor, I. Neurocognitive effects of repetitive transcranial magnetic stimulation in patients with cerebrovascular disease without dementia. J Psychophysiol. (2008) 22:14–9. doi: 10.1027/0269-8803.22.1.14
68. Aref, H . Clinical response to high frquency rTMS among patients with cerebral small vessel disease. Ain Shams Med J. (2019) 70:699–704. doi: 10.21608/asmj.2019.101280
69. Liu, Y, Luo, J, Fang, J, Yin, M, Cao, J, Zhang, S, et al. Screening diagnosis of executive dysfunction after ischemic stroke and the effects of transcranial magnetic stimulation: a prospective functional near-infrared spectroscopy study. CNS Neurosci Ther. (2023) 29:1561–70. doi: 10.1111/cns.14118
70. Lee, J, Choi, BH, Oh, E, Sohn, EH, and Lee, AY. Treatment of Alzheimer's disease with repetitive transcranial magnetic stimulation combined with cognitive training: a prospective, randomized, double-blind, placebo-controlled study. J Clin Neurol. (2016) 12:57–64. doi: 10.3988/jcn.2016.12.1.57
71. Youmei, Z, Jianer, C, Weihai, C, and Zhongcai, P. Advances in non-invasive brain stimulation techniques to improve cognitive impairment. Chinese J Phys Med Rehabil. (2021) 43:1048–52. doi: 10.3760/cma.j.issn.0254-1424.2021.11.022
72. Dongshaui, Z, Rui, F, Guizhi, X, and Chong, D. Advances in the clinical application of repetitive transcranial magnetic stimulation for the treatment of cognitive dysfunction. Chinese J Phys Med Rehabil. (2019) 41:780–3. doi: 10.3760/cma.j.issn.0254-1424.2019.10.015
73. Jian, X, and Guohua, Z. Advances in the study of executive function in a stroke patient. Chinese J Rehabil Theory Pract. (2020) 26:797–801. doi: 10.3969/j.issn.1006?97712020.07.012
74. Zhang, BBB, Kan, RLD, Giron, CG, Lin, TTZ, Yau, SY, and Kranz, GS. Dose-response relationship between Itbs and prefrontal activation during executive functioning: a Fnirs study. Front Psych. (2022) 13:1049130. doi: 10.3389/fpsyt.2022.1049130
75. Yaning, Z, Jinyun, G, and Yujing, W. A study of factors influencing executive dysfunction in ischemic stroke patients. Chongqing Med. (2012) 41:2058–61. doi: 10.3969/j.issn.1671-8348.2012.20.021
76. Málly, J, Geisz, N, and Dinya, E. Follow up study: the influence of rTMS with high and low frequency stimulation on motor and executive function in Parkinson's disease. Brain Res Bull. (2017) 135:98–104. doi: 10.1016/j.brainresbull.2017.10.002
77. Hu, DM, Cheng, XR, Zhou, WX, Zhang, YX, and Zhou, LC. Treatment of post stroke cognitive impairment by rTMS. Sheng Li Ke Xue Jin Zhan. (2012) 43:411–6.
78. Cantone, M, Fisicaro, F, Ferri, R, Bella, R, Pennisi, G, Lanza, G, et al. Sex differences in mild vascular cognitive impairment: a multimodal transcranial magnetic stimulation study. PLoS One. (2023) 18:e0282751. doi: 10.1371/journal.pone.0282751
79. Surya, JR, Habelhah, B, Haroon, J, Mahdavi, K, Jordan, K, Becerra, S, et al. Functional MRI lateralization [M1] of dlPFC and implications for transcranial magnetic stimulation (TMS) targeting. Diagnostics (Basel). (2023) 13:2690. doi: 10.3390/diagnostics13162690
80. Saczynski, JS, Sigurdsson, S, Jonsdottir, MK, Eiriksdottir, G, Jonsson, PV, Garcia, ME, et al. Cerebral infarcts and cognitive performance: importance of location and number of infarcts. Stroke. (2009) 40:677–82. doi: 10.1161/strokeaha.108.530212
81. Sharbafshaaer, M, Gigi, I, Lavorgna, L, Esposito, S, Bonavita, S, Tedeschi, G, et al. Repetitive transcranial magnetic stimulation (rTMS) in mild cognitive impairment: effects on cognitive functions-a systematic review. J Clin Med. (2023) 12:6190. doi: 10.3390/jcm12196190
82. Di Lorenzo, F, Bonni, S, Picazio, S, Motta, C, Caltagirone, C, Martorana, A, et al. Effects of cerebellar Theta burst stimulation on contralateral motor cortex excitability in patients with Alzheimer's disease. Brain Topogr. (2020) 33:613–7. doi: 10.1007/s10548-020-00781-6
Keywords: repetitive transcranial magnetic stimulation, vascular cognitive impairment, executive function, cognition, vascular dementia, meta-analysis
Citation: Wang X, Ding Q, Li Y, Li T, Li Y, Yin J and Zhuang W (2024) Repetitive transcranial magnetic stimulation impacts the executive function of patients with vascular cognitive impairment: a systematic review and meta-analysis. Front. Neurol. 15:1374395. doi: 10.3389/fneur.2024.1374395
Edited by:
Mariagiovanna Cantone, Gaspare Rodolico Hospital, ItalyReviewed by:
Francesco Di Lorenzo, Santa Lucia Foundation (IRCCS), ItalyAkira Monji, Saga University, Japan
Copyright © 2024 Wang, Ding, Li, Li, Li, Yin and Zhuang. This is an open-access article distributed under the terms of the Creative Commons Attribution License (CC BY). The use, distribution or reproduction in other forums is permitted, provided the original author(s) and the copyright owner(s) are credited and that the original publication in this journal is cited, in accordance with accepted academic practice. No use, distribution or reproduction is permitted which does not comply with these terms.
*Correspondence: Weisheng Zhuang, zhuang20062634@163.com