- 1Department of Neurosurgery, Dehua County Hospital, Quanzhou, China
- 2Department of Imaging, Dehua County Hospital, Quanzhou, China
- 3Department of Internal Medicine, Dehua County Hospital, Quanzhou, China
- 4Department of Geriatrics, Changji People’s Hospital, Changji, China
- 5Department of Laboratory Medicine, Dehua County Hospital, Quanzhou, China
- 6Department of Neurology, Dehua County Hospital, Quanzhou, China
Objective: Recent reports have demonstrated that a wider pulse pressure upon admission is correlated with heightened in-hospital mortality following spontaneous supratentorial intracerebral hemorrhage (ssICH). However, the underlying mechanism remains ambiguous. We investigated whether a wider pulse pressure was associated with hematoma expansion (HE).
Methods: Demographic information, clinical features, and functional outcomes of patients diagnosed with ssICH were retrospectively collected and analyzed. Multivariate logistic regression was conducted to identify independent predictors of HE. Weighted logistic regression, restricted cubic spline models, and propensity score matching (PSM) were employed to estimate the association between pulse pressure and HE.
Results: We included 234 eligible adult ssICH patients aged 60 (51–71) years, and 55.56% were male. The mean pulse pressure was 80.94 ± 23.32 mmHg. Twenty-seven patients (11.54%) developed early HE events, and 116 (49.57%) experienced a poor outcome (modified Rankin scale 3–6). A wider mean pulse pressure as a continuous variable was a predictor of HE [odds ratios (OR) 1.026, 95% confidence interval (CI) 1.007–1.046, p = 0.008] in multivariate analysis. We transformed pulse pressure into a dichotomous variable based on its cutoff value. After adjusting for confounding of HE variables, the occurrence of HE in patients with ssICH with wider pulse pressure levels (≥98 mmHg) had 3.78 times (OR 95% CI 1.47–9.68, p = 0.006) compared to those with narrower pulse pressure levels (<98 mmHg). A linear association was observed between pulse pressure and increased HE risk (P for overall = 0.036, P for nonlinear = 0.759). After 1:1 PSM (pulse pressure ≥98 mmHg vs. pulse pressure <98 mmHg), the rates of HE events and poor outcome still had statistically significant in wider-pulse pressure group [HE, 12/51 (23.53%) vs. 4/51 [7.84%], p = 0.029; poor outcome, 34/51 (66.67%) vs. 19/51 (37.25%), p = 0.003].
Conclusion: Widened acute pulse pressure (≥98 mmHg) levels at admission are associated with increased risks of early HE and unfavorable outcomes in patients with ssICH.
Introduction
Spontaneous supratentorial intracerebral hemorrhage (ssICH) is one of the most catastrophic types of strokes with high morbidity, disability, and mortality, and poses a substantial burden on health and social services (1). Most brain injuries result from the mechanical effects of the initial hemorrhage (2). Nonetheless, hematoma expansion (HE) contributes to secondary brain damage and is a major determinant of clinical deterioration and unfavorable prognosis (3–5).
After the onset of symptoms of intracerebral hemorrhage (ICH), there is often a sudden rise in blood pressure (BP), which is associated with an increased risk of hematoma growth and a poor prognosis (6). Numerous studies have linked high BP with HE and adverse clinical outcomes (4, 6, 7). The VISTA-ICH Cohort finding indicates that elevated blood pressure within 24 h after ICH is associated with HE and unfavorable prognosis (8). Several subsequent studies have revealed that acutely elevated systolic blood pressure (SBP) is independently associated with HE following spontaneous intracerebral hemorrhage (sICH) (9, 10). A meta-analysis of individual patient data from randomized clinical trials has demonstrated that intensive SBP lowering is safe and significantly reduces hematoma growth, implicating also that elevated SBP may be associated with early hematoma enlargement (11).
However, current guidelines for defining and treating spontaneous cerebral hemorrhage focus on SBP and diastolic blood pressure (DBP) rather than pulse pressure (12). Pulse pressure is defined as the difference between SBP and DBP, reflecting the stiffness of the large arteries (13). Pulse pressure increases with age and may be a key indicator of blood pressure in the elderly (14). According to the strain vessel hypothesis, small, short, penetrating arteries in the brain arising from large arteries are exposed to high pressures and maintain high vascular tone, thus providing a large pressure gradient from the large arteries to the penetrating arteries (15). As arterial stiffness increases, pulse wave velocity increases (16). A study has revealed that increased arterial stiffness is physiologically associated with the development of cerebral small vessel disease, such as cerebral microhemorrhage (16, 17). Therefore, in stroke patients with cerebral microbleeds or cerebral hemorrhages, increased pulse pressure may lead to increased perforating arterial injury.
Secondary analyses of the Antihypertensive Treatment of Acute Cerebral Hemorrhage II study (ATACH2) trial demonstrated that the time-sensitivity of medical interventions targeting HE, in which rapid intensive blood pressure lowering (within 2 h of the onset of ICH symptoms) reduced HE and improved functional outcomes (6). Indeed, the complex relationships between blood pressure parameters and clinical characteristics in patients with ICH have not been thoroughly investigated. Recent literature demonstrates that wide pulse pressure is an independent risk factor and a predictor of in-hospital mortality (18). Nonetheless, the role of pulse pressure in ICH patients is not fully understood, and its potential to differentiate risks and predict early HE has not been well-explored. Hence, this study investigated the correlation between admission pulse pressure and early HE in patients with ssICH.
Methods and materials
Study design and population
This retrospective observational study was conducted at Dehua County Hospital for Neurology and Neurosurgery between December 2020 and July 2023, focusing on patients aged 20 years and older who diagnosed with ssICH. The ethical clearance was obtained from the institutional ethical committee. Patients who met the following inclusion criteria were considered eligible: (a) they had confirmed initial supratentorial ICH by computed tomography (CT) scan within 6 h of symptom onset; (b) they underwent follow-up CT scans within 25 h after the baseline CT scan (19). Exclusion criteria included: (a) age < 20 years old; (b) having incomplete or missing case data; (c) undergoing emergency surgery before follow-up CT; (d) having a malignant tumor or hemolytic anemia. A flow diagram of the patient selection process is presented in Figure 1.
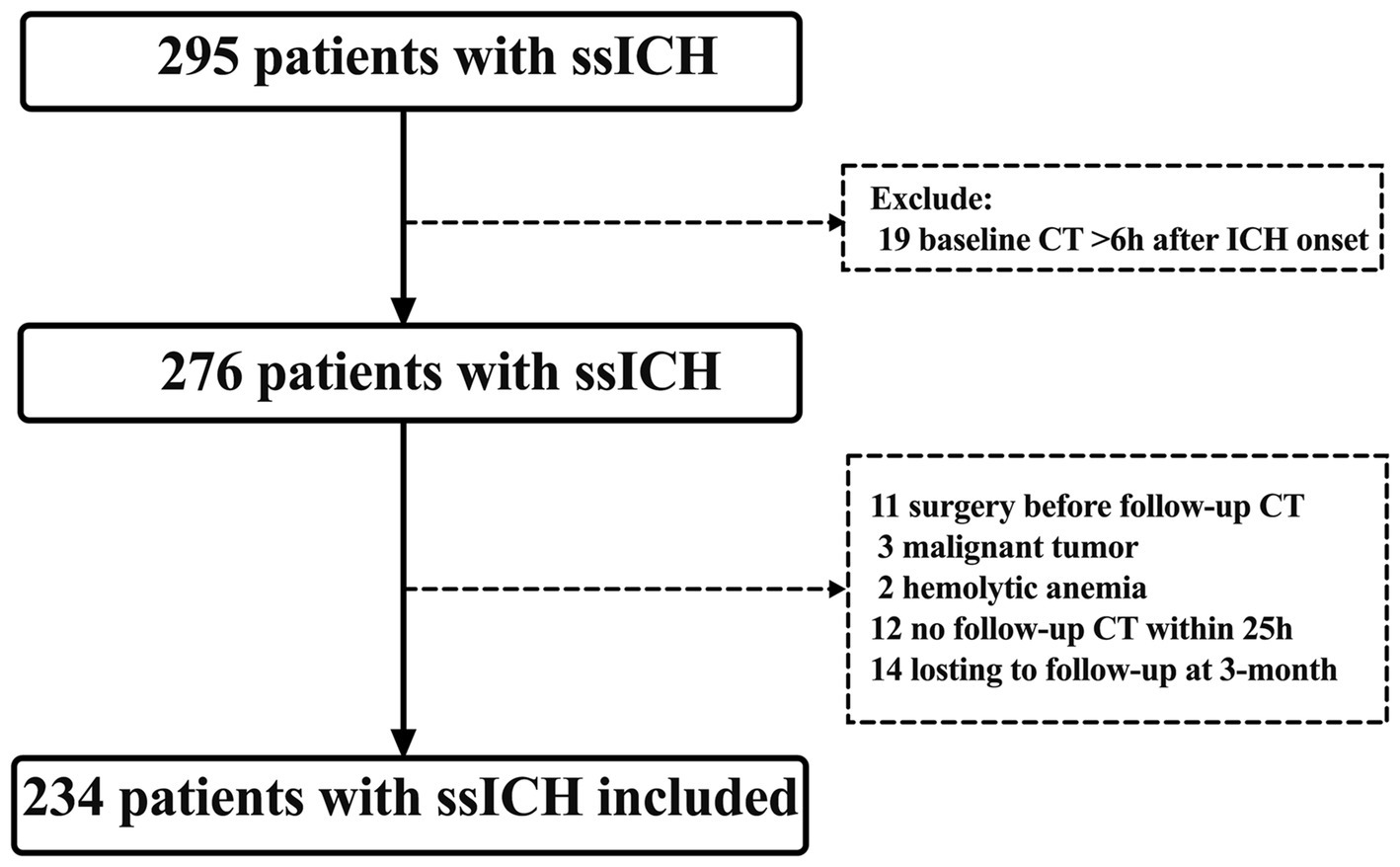
Figure 1. Flowchart screening for eligibility. CT, computed tomography; ICH, intracerebral hemorrhage; ssICH, spontaneous supratentorial intracerebral hemorrhage.
Clinical management followed the American Heart Association and American Stroke Association guidelines (12). Patients with stable neurological status or those who were unsuitable for surgery due to age or comorbidities were given conservative treatment. When the ICH volume exceeded 30 mL, or the patient’s consciousness declined, a craniotomy to remove the hematoma or cranial decompression resection was performed (12). Concomitant external ventricular drainage was performed if acute hydrocephalus or elevated intracranial pressure occurred.
Data collection
Baseline characteristics and clinical information were collected from the electronic database. Baseline characteristics included demographics (age, sex), medical history (hypertension and diabetes mellitus), adverse lifestyle factors (smoking and alcoholism), admission SBP, DBP, admission Glasgow Coma Scale (GCS) score, time from onset of illness to baseline CT, ssICH location, baseline ssICH volume, presence of intraventricular hemorrhage (IVH), treatment, and admission laboratory parameters. Admission laboratory parameters included hemoglobin, platelets, activated partial thromboplastin time (aPTT), thrombin time (PT), and international normalized ratio (INR). Treatment options for surgery included microinvasive removal of hematoma, craniotomy evacuation of hematoma, and decompressive craniectomy.
Blood pressure variables
Upon arrival at the hospital, the on-admission BP was recorded immediately. BP was measured according to the standard in-hospital care protocol, an automated, non-invasive cuff measurement of systolic and diastolic blood pressure at the mid-biceps. Mean arterial pressure (MAP) was calculated by the formula (SBP + 2 × DBP)/3. Pulse pressure was determined by the absolute difference between SBP and DBP (19). An intensive strategy to lower the patient’s SBP to 150–160 mmHg, especially in patients with SBP over 220 mmHg after ssICH (2). Close monitoring of renal function and renal volume status was necessary.
Imaging analysis
The CT scan was conducted upon admission, and a subsequent CT scan was reviewed within 25 h. The 25 h cut-off was selected to encompass all CT scans performed within 24 h following the initial scan, typically done right away or within the first few minutes after admission (19). Assessment of the initial CT included determining the location of the hematoma (lobar or deep) and the presence of IVH. Supratentorial deep ICH is characterized by its selective involvement of the thalamus, basal ganglia, internal capsule, and deep periventricular white matter. At the same time, lobar ICH originates at the cortex and cortical–subcortical junction (20). Using a post-processing workstation (Advantage Workstation 4.6, GE Healthcare, Chicago, Illinois, United States), hematoma volumes on the initial and follow-up CT scans were estimated (21). HE was defined as an increase of hematoma volume >6 mL and relative hematoma volume increase >33% between initial and follow-up CT scans (5, 22).
Outcome assessment
A favorable outcome is indicated by a modified Rankin scale (mRS) score <3 (mRS score 0–2, representing good recovery, no significant disability, or slight disability), while a poor outcome is indicated by an mRS score ≥3 (mRS score 3–6, representing moderate disability, moderately severe disability, severe disability, or death) (23). Outpatient clinic visits, telephone interviews, and WeChat interviews were utilized to evaluate the mRS grades three months post-discharge (24).
Statistical analysis
For the statistical analyses, SPSS Statistics 26 (IBM, Armonk, NY), MedCalc version 18.2.1 (MedCalc Software, Ostend, Belgium), and R 4.2.1(RStudio, Boston, MA, United States) were all employed. A two-sided p < 0.05 was established as statistically significant. The mean ± standard deviation (SD), or median (interquartile range, IQR) was used to present continuous variables, while counts with percentages [n (%)] were used for categorical variables. Normal distribution was assessed by the Shapiro–Wilk normality test. Continuous variables were compared using the Student’s t-test (normal distribution) or the Mann–Whitney U-test (nonnormal distribution). Categorical variables were compared using the χ2 test or Fisher’s exact test. HE associates with an univariable p-value of less than 0.05 were included in the multivariate models. Multivariate logistic regression and linear analysis examined the independent association between pulse pressure and outcome variables.
Then, the area under a receiver-operating characteristic (ROC) curve was determined without covariate adjustment. Pulse pressure was transformed into a binary categorical variable based on the ROC analysis’s threshold to make the model better applied to clinical practice. Weighted multivariate linear regression models were developed. The pulse pressure was dichotomized as “< optimal cutoff value” and “≥ optimal cutoff value. Model I was unadjusted. Model II was adjusted for the variables with a p-value of less than 0.05 in univariable analysis.
The restricted cubic spline (RCS) model is a statistical analysis method used to create a smooth curve that visually describes the nonlinear relationship between pulse pressure and early HE. This study used the RCS model to observe nonlinear trends, with the 5th, 50th, and 95th pulse pressures serving as the three knots. Our construction of the RCS models was based on weighted multivariate linear regression models.
Moreover, the patients with ssICH were categorized into a wider pulse pressure group and a narrower group based on the optimal cutoff value obtained from ROC analysis. After conducting propensity score matching (PSM) with a 1:1 match and a caliper of 0.01 using the nearest neighbor approach to account for all potential confounders, the rates of early HE events, and poor outcomes were compared between the two groups.
Results
Baseline characteristics
After screening according to the inclusion and exclusion criteria, 234 adult patients with ssICH were included in this study (Figure 1). The Shapiro–Wilk normality test for continuous variables is displayed in Supplementary Table S1. Of the continuous variables, all continuous variables were non-normally distributed except for SBP, MAP, and pulse pressure. The median age of the included patients was 60.0 (IQR, 51.0–71.0) years and 55.56% were male. 82.05% of patients had a history of hypertension, indicating a significant prevalence among the study population. The mean SBP was 185.92 ± 30.21 mmHg, while the median DBP was 103 (IQR, 93.75–114) mmHg. The mean pulse pressure was 80.94 ± 23.32 mmHg. The median time from symptom onset to initial CT was 2 h (IQR, 1.0–3.0 h), the median baseline GCS scores being 14 (IQR, 9.0–15), and the median baseline volume was 8.4 mL (IQR, 4.12–14.0 mL). Out of the 234 patients, 27 patients (11.54%) developed early HE events, and 116 (49.57%) experienced a poor outcome (mRS 3–6). The baseline characteristics are summarized in detail in Table 1.
Association of pulse pressure with early HE and poor outcome
Table 2 presents demographic characteristics, clinical features, and laboratory data that may have significance for HE. Univariate analysis revealed a statistically significant association between the occurrence of HE and SBP (p = 0.005), pulse pressure (p < 0.001), time from onset to baseline CT (p = 0.021), and admission GCS score (p = 0.005).
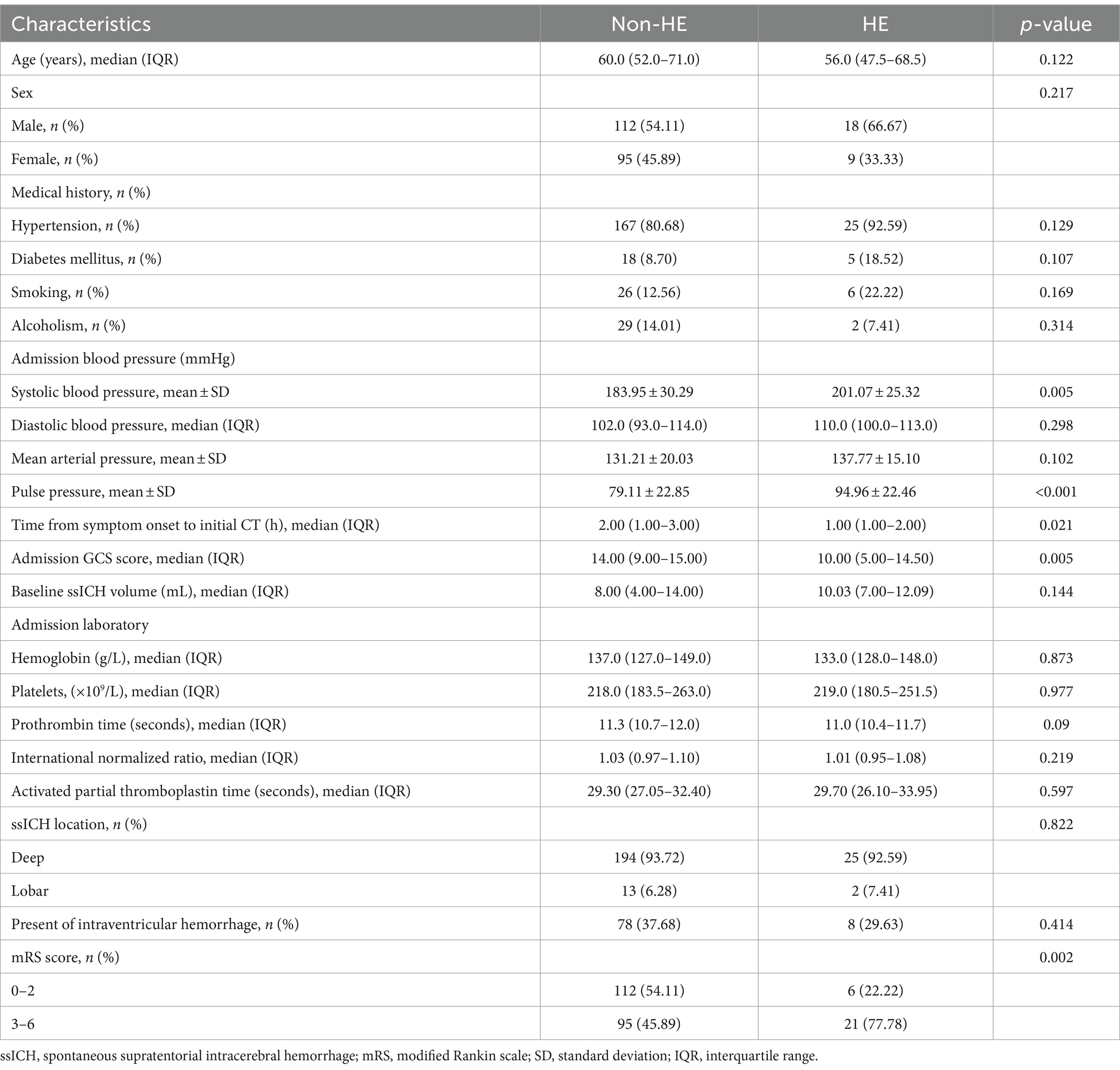
Table 2. Univariate analysis of patient characteristics associated with early hematoma expansion following ssICH.
A multivariate logistic regression analysis was conducted to avoid the confounding effects of each factor. Variables with significant effects in univariate analyses (p < 0.05), including admission GCS score, pulse pressure, and time from symptom onset to baseline CT, were assessed in multivariate analyses. The area under the curve (AUC) of pulse pressure and the AUC of SBP were comparable by Z-test, and the AUC of pulse pressure (AUC = 0.685) was slightly greater than that of SBP (AUC = 0.667). Still, no statistically significant difference existed (Z = 0.562, p = 0.574). In addition, there may be multicollinearity between pulse pressure and SBP. Finally, we referred to previous literature and included pulse pressure in the multivariable analysis instead of SBP (18, 19). Multivariable analysis revealed wider pulse pressure levels as a continuous variable were statistically associated with HE [pulse pressure, odds ratios (OR) 1.026, 95% confidence interval (CI) 1.007–1.046, p = 0.008, Table 2], while the time from illness onset to baseline CT (OR 0.629, 95%CI 0.394–1.004, p = 0.052), and admission GCS score (OR 0.91, 95%CI 0.82–1.011, p = 0.079) were not associated with HE. In Figure 2A and Table 3, the ROC analysis demonstrated an AUC of 0.685 (95% CI 0.622–0.744, p < 0.0001, Figure 2A), with a sensitivity of 48.15% and specificity of 80.19% for predicting HE in ssICH patients, and the optimal cutoff threshold of pulse pressure level was identified at 98 mmHg (Table 3). Moreover, we found that HE was associated with an increased risk of 3 month poor outcome (mRS 3–6, HE vs. Non-HE: 77.78% vs. 45.89%, p = 0.002, Table 2). ROC analysis of pulse pressure to predict poor outcome determined the best cut-off value of 78 mmHg with an AUC of 0.663 (95% CI, 0.598–0.723, p < 0.001, Figure 2B and Table 3).
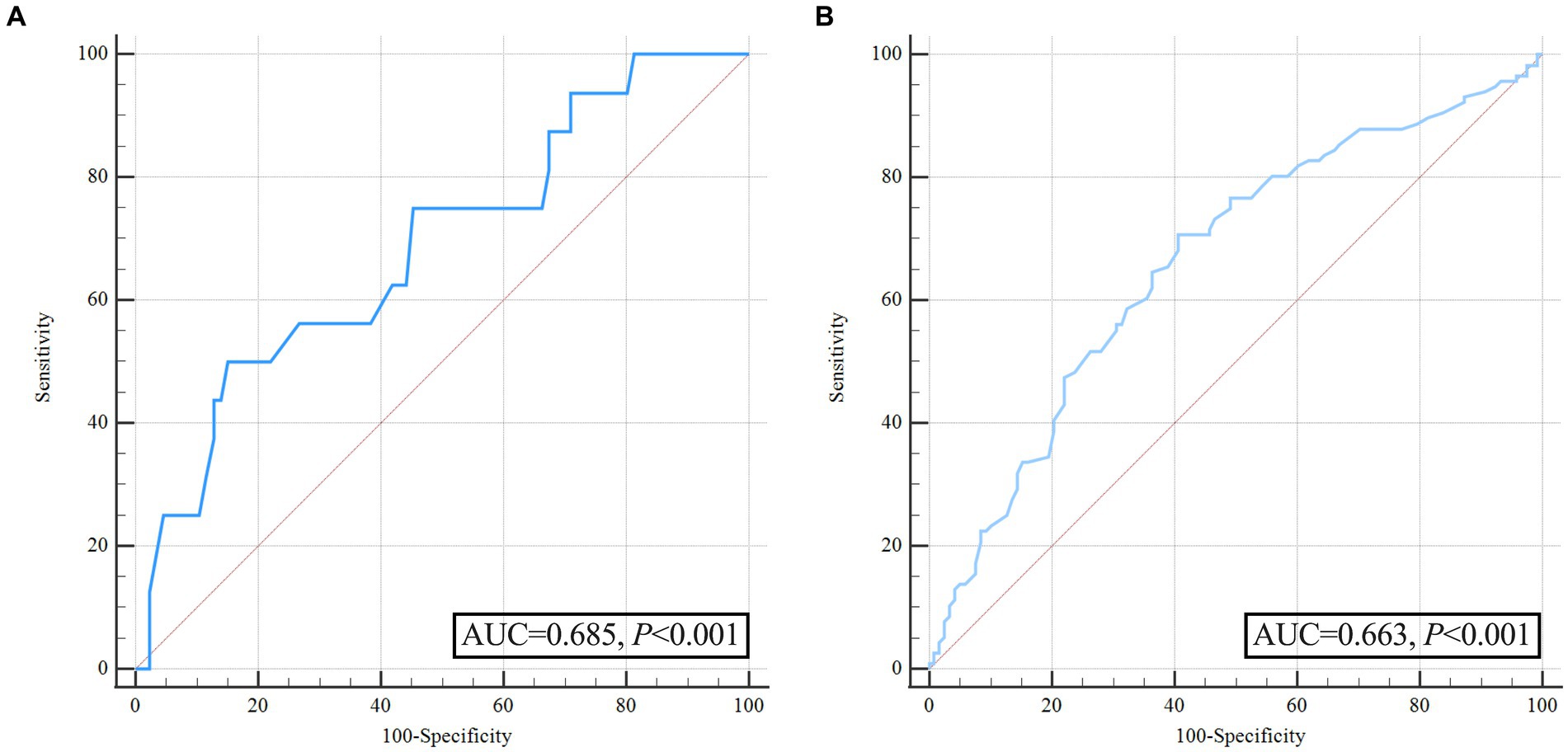
Figure 2. Receiver operating characteristic curve analysis of pulse pressure for predicting hematoma expansion and unfavorable outcome before propensity score matching. (A) ROC for pulse pressure to predict hematoma expansion; (B) ROC for pulse pressure to predict poor outcome.
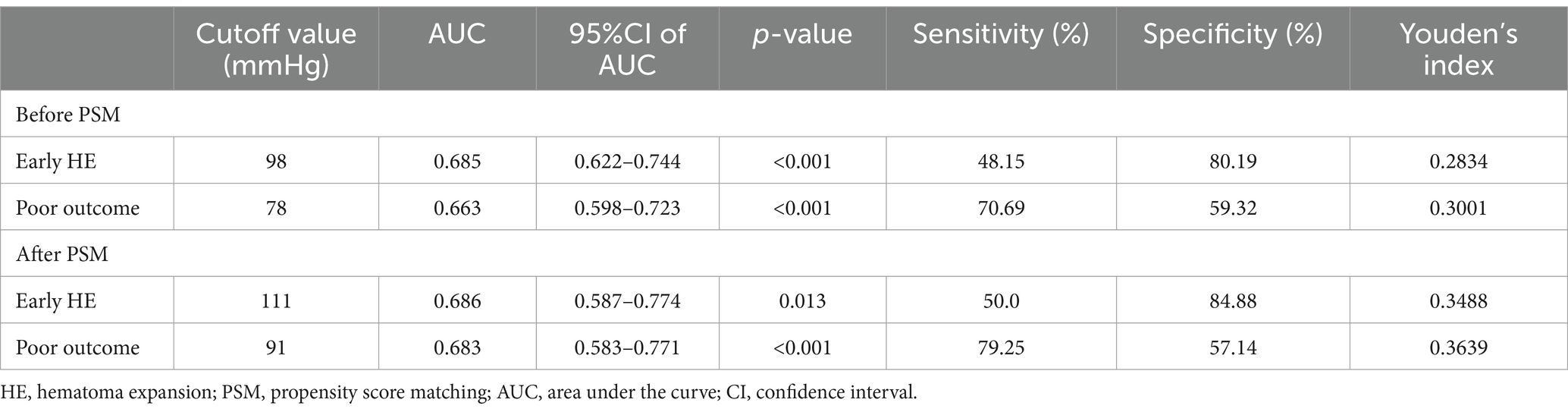
Table 3. Area under the pulse pressure curve associated with early hematoma expansion and poor outcomes at 3 months.
We converted pulse pressure into a binary variable and developed several multivariate models to investigate the effect of different pulse pressure ranges on early HE. The pulse pressure was dichotomized as “<98 mmHg” and “≥98 mmHg.” Two weighted logistic regression models were used to analyze the relationship between pulse pressure and early HE in patients with ssICH, as presented in Table 4. We constructed a weighted logistic regression model, adjusting for variables with p < 0.05 in the univariate analysis (including admission GCS score and time from illness onset to initial CT), which revealed that HE occurred 3.78 times (95% CI 1.47–9.68, p = 0.006) more frequently in patients with pulse pressure ≥98 mmHg than in those with pulse pressure <98 mmHg. Therefore, the result of the regression model supports the conclusion that wider pulse pressure levels (≥98 mmHg) are associated with an increased risk of early HE occurrence in patients with ssICH.
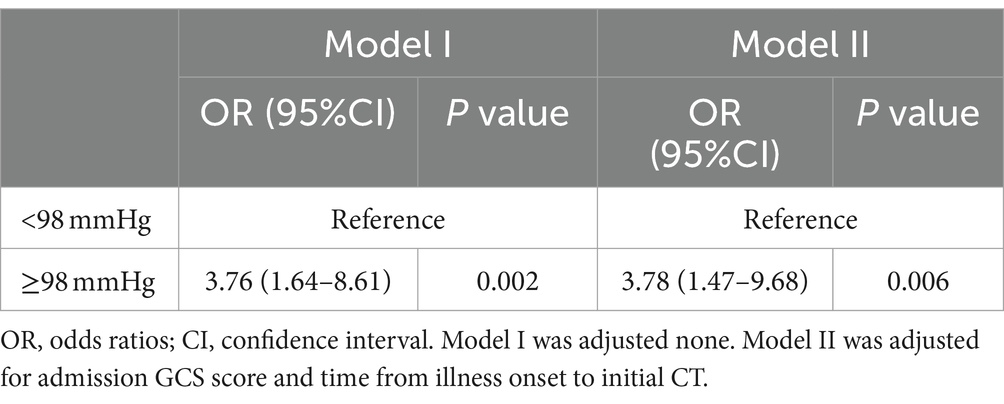
Table 4. ORs (95% CIs) of the association between pulse pressure and early hematoma expansion in multivariate adjustment models.
Dose–response relationship between pulse pressure level and the risk of early HE
Our study utilized RCS plots to illustrate the relationships between pulse pressure levels and early HE. Two models were developed to adjust for different confounding factors. Results from two linear RCS models demonstrated a significant association between pulse pressure level and an increased risk of HE (model I, p for overall = 0.007, p for nonlinear = 0.876; model II, p for overall = 0.036, p for nonlinear = 0.759; Figures 3A,B). The RCS curve indicated that the risk of HE increased as pulse pressure increased (Figure 3).
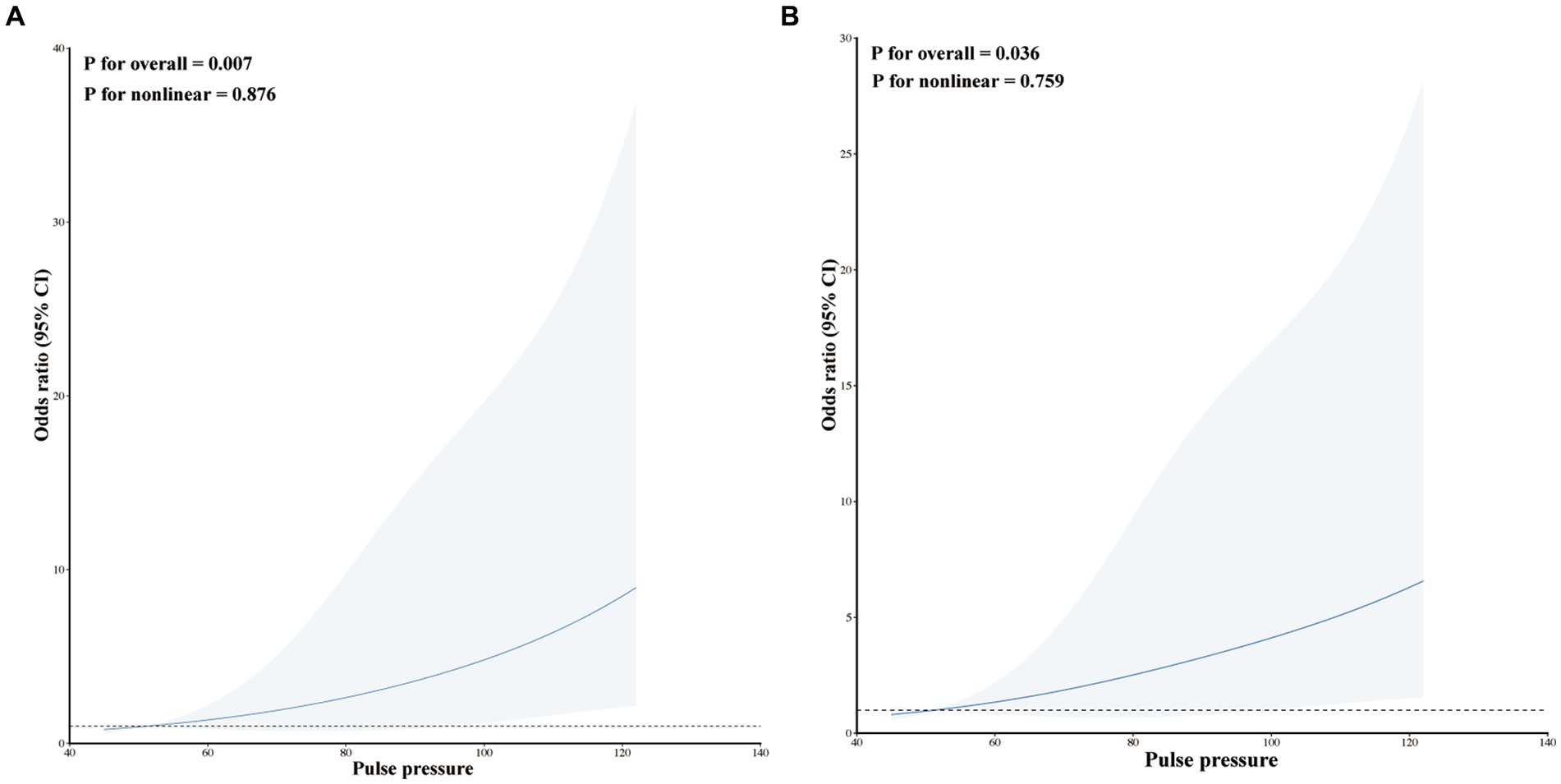
Figure 3. Restricted cubic spline modeling the relationship between pulse pressure and HE in patients with ssICH. (A) Model I for HE; (B) Model II for HE. Model I was adjusted none. Model II was adjusted for time from illness onset to initial CT and admission GCS score.
Propensity score matching
The patients with ssICH were categorized into a wider pulse pressure group (pulse pressure ≥ 98 mmHg, n = 180) and a narrower pulse pressure group (pulse pressure < 98 mmHg, n = 54) based on the optimal threshold. Univariate analysis revealed significant differences in history of hypertension, SBP, DBP, MAP, pulse pressure, admission GCS score, and early HE and mRS score between the two groups (Table 5).
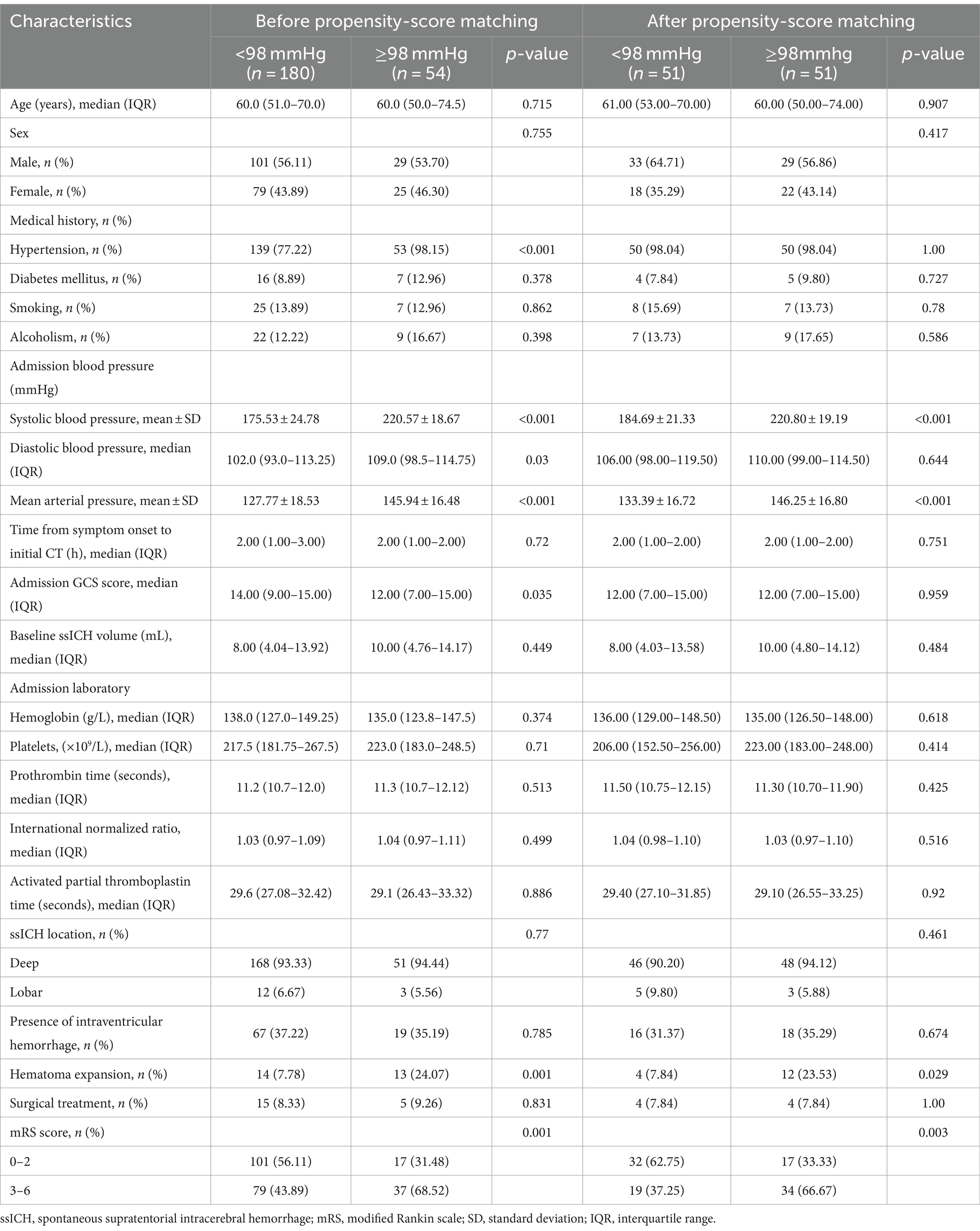
Table 5. Characteristics of patients dichotomized to the identified pulse pressure threshold (98 mmHg) before and after propensity-score matching.
To further account for significant differences in baseline characteristics, we conducted a one-to-one PSM. Age, sex, hypertension, smoking, diabetes mellitus, alcoholism, baseline ssICH volume, admission GCS score, time from onset to initial CT, hemoglobin, platelets, PT, INR, aPTT, ssICH location, and presence of IVH were included in PSM. After adjusting for potential confounding with PSM (caliper 0.01, ratio 1:1, nearest neighbor approach), early HE events (p = 0.029) and poor outcome (p = 0.003) still had statistically significant differences between the two groups (Table 5). The incidence of HE events and unfavorable outcomes remained significantly higher in the group with wider pulse pressure, as evidenced by the statistical analysis (HE, 12/51[23.53%] vs. 4/51[7.84%], p = 0.029; poor outcome, 34/51[66.67%] vs. 19/51 (37.25%), p = 0.003; Table 5). According to the ROC curve, the AUC of pulse pressure level for predicting early HE and poor prognosis was 0.686 and 0.683, respectively (Figures 4A,B; Table 3).
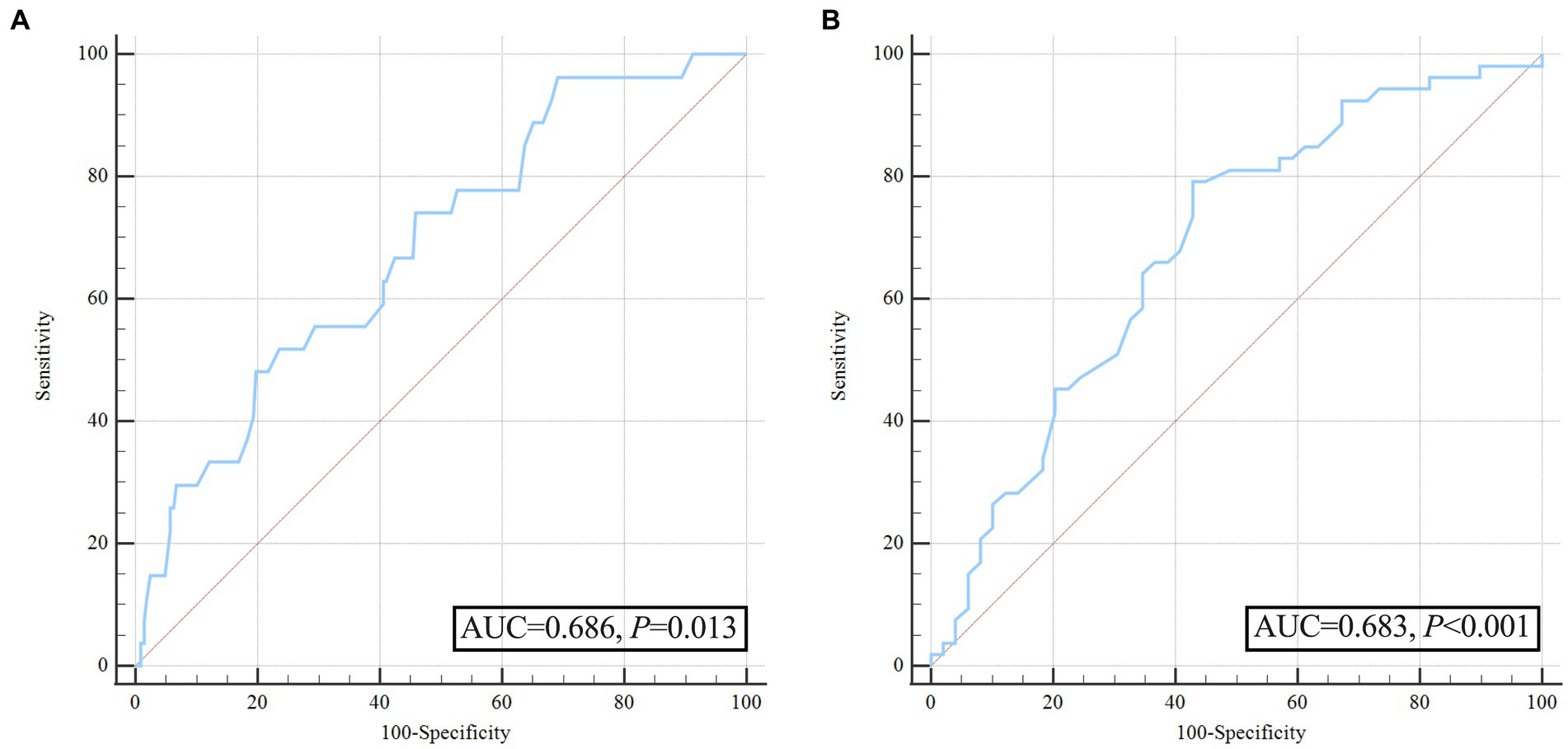
Figure 4. Receiver operating characteristic curve analysis of pulse pressure for predicting hematoma expansion and unfavorable outcome after propensity score matching. (A) ROC for pulse pressure to predict hematoma expansion after propensity score matching; (B) ROC for pulse pressure to predict poor outcome after propensity score matching.
Discussion
The present study investigated the association between pulse pressure and early HE in patients with ssICH. Our results demonstrated that widened acute pulse pressure (≥98 mmHg) levels upon admission are associated with increased risks of early hematoma expansion in patients with ssICH. Moreover, a significant link exists between admission pulse pressure levels and an increased risk of early HE in the multivariate logistic regression analysis, weighted generalized additive model, and RCS models. Lastly, using a 1:1 PSM to balance the variations in baseline characteristics, we found that admission pulse pressure remained an independent predictor for early hematoma enlargement and unfavorable prognosis. To our knowledge, this was the first study to investigate the association of admission pulse pressure with early HE using nonlinear models.
sICH is commonly attributed to acute hypertension and prolonged high BP can worsen the secondary injury. It can lead to early hematoma enlargement by fueling the active hemorrhagic process in the acute phase and exacerbating tissue and microvascular damage in the subsequent hours. Despite the lack of detriment to rapid BP control shown in the ATACH2 study (22), it failed to demonstrate any benefits for preventing HE or improving functional outcomes. A prior study by Larse and colleagues illustrated that elevated prehospital DBP and MAP correlated with HE in sICH patients (19). Still, there was no link between prehospital pulse pressure and HE in their study (19). However, our study is inconsistent with this study. We found that wider pulse pressure levels upon admission are associated with increased risks of early HE in ssICH patients. First, the background of the patients studied by Larse et al. differed from ours. The monitoring of BP was performed in a prehospital ambulance in his research, and BP measurements may be inaccurate (as mentioned by the authors in Limitations) (19). Our BP monitoring was monitored at a steady state upon admission. Furthermore, the previous study encompassed all hematoma locations, including lobar, deep, infratentorial, and unclassifiable ICH, while our research specifically focused on patients with ssICH.
Wide pulse pressure can be visualized in the classic Cushing’s response to elevated intracranial pressure (25). The Cushing triad typically includes bradycardia, respiratory depression, and hypertension, which may be associated with brainstem distortion or ischemia. Hence, the link between wide pulse pressure and more severe findings on admission CT scans (such as larger hematoma volume and significant midline shift) may be attributed to a pathophysiological reflex activated by elevated intracranial pressure (18). The present study highlighted an association between elevated pulse pressure at admission and an increased likelihood of early HE during hospitalization. Even though we observed the correlation, the underlying mechanisms driving the connection between increased pulse pressure and HE in ssICH patients remain elusive. The potential underlying mechanism might be as follows. First, wider pulse pressure imposes increased mechanical stress on the vascular walls, particularly in the microvasculature surrounding the site of intracranial hemorrhage. This heightened stress may compromise the integrity of endothelial cells and the basal membrane, potentially contributing to the initiation of early HE (26). Second, increased pulse pressure may disrupt the blood–brain barrier. This disruption allows components from the bloodstream to permeate into the brain tissue, initiating inflammatory responses and exacerbating neuronal damage, thereby promoting hematoma growth (27, 28). Third, high pulse pressure levels could lead to autonomic nervous system dysregulation, impairing blood flow autoregulation. This imbalance might contribute to hematoma enlargement, especially within the microvascular network near the site of intracranial hemorrhage (29). Fourth, elevated pulse pressure may subject the vascular walls to mechanical stress, inducing structural alterations and the formation of micro-fissures. This pathway could provide a route for further hematoma expansion by compromising the structural integrity of blood vessels (30). Fifth, increased pulse pressure might trigger inflammatory responses, accelerating HE by migrating inflammatory cells and releasing inflammatory mediators. The complex network of inflammatory reactions could contribute to the observed association (31). Sixth, elevated pulse pressure levels may increase the propensity for hemorrhagic transformation, creating an environment conducive to early HE in ssICH patients (32). The association between increased pulse pressure and heightened risk of early HE in ssICH patients involves a multifaceted interplay of vascular, inflammatory, and autoregulatory mechanisms. A deeper understanding of these mechanisms provides a foundation for developing targeted interventions to mitigate early HE and improve patients’ prognosis. Further research into these intricate pathways holds promise for optimizing treatment strategies and advancing personalized approaches to the acute management of ssICH.
Our study demonstrated that 27 patients (11.54%) experienced early HE events, indicating a decreased incidence of hematoma enlargement compared to our previous reports (21, 24). Upon analysis, we determined the potential reasons as follows. Our last study encompassed all sICH, including deep, lobar, brainstem, and cerebellum, whereas the current study focused on ssICH. Second, based on the latest literature (19), the present study defined the time point for follow-up CT as 25 h, which is different from the time point of the previous study (24 h) (24). Thirdly, the current study did not exclude patients with an initial hematoma volume of <1 mL. The initial ICH hematoma volume in this study was markedly smaller compared to that in the previous study (8. 4 [4.16–14.0] mL. vs.11.95 [5.0–28.64] mL). Additionally, over the last 3 years, a few sICH patients with more critical conditions were hospitalized in the neurointensive care unit (NICU) at our hospital, yet they were not included in the present study.
The current study revealed that wider pulse pressure (≥78 mmHg) was an independent predictor for poor prognosis based on ROC curve. Interestingly, our study differs from the results of Yu Hua Huang et al. (18), who contended that pulse pressure over 100 mmHg was not correlated with a poor prognosis but solely with mortality during hospitalization. According to the study by Yu Hua Huang et al., a pulse pressure value greater than 100 mmHg was identified as a risk factor for cardiovascular disease prognosis and mortality (25). However, this measurement may not accurately represent the pulse pressure situation of intracranial lesions,especially in patients with ICH induced by hypertension. Additionally, Yu Hua Huang et al. defined mRS 4–6 as a poor diagnosis, whereas we defined mRS 3–6 as a poor diagnosis based on the more common previous literature (23). This difference in mRS definitions may also explain the different conclusions reached.
Several limitations existed in the present study. Firstly, inherent in the design of our single-center, retrospective, observational study with a relatively small sample size is an inability to conclude causation. Additionally, we did not incorporate patients with ssICH in the NICU, who were more critically ill, potentially causing bias. Our strict exclusion criteria may limit the applicability of our findings to other clinical presentations, such as brain stem hemorrhage and infratentorial hematomas, which are often included in general ICH literature. Thirdly, our study failed to account for potential confounders, such as shifts in blood pressure management and treatment strategies during hospitalization, which might have influenced the outcomes. A single admission BP value is not representative of all BP variability. The study’s robustness would have been greatly improved if ambulatory BP monitoring had been utilized. Fourthly, the associations between BP and imaging features of early HE, such as the CT island sign and CT angiographic spot sign, were not analyzed, as previous similar studies had already covered this topic (33). Finally, despite our study finding a notable link between pulse pressure and early HE, the precise mechanism driving this correlation is still not fully understood. Additional research is required to elucidate the mechanisms and explore potential therapeutic interventions that target pulse pressure.
Conclusion
Widened acute pulse pressure (≥98 mmHg) levels upon admission are associated with increased risks of early hematoma expansion in patients with ssICH. This observation underscores the importance of measuring pulse pressure in future trials investigating the association between BP reduction and HE in ssICH patients. Nevertheless, multi-center, larger-sample prospective studies are needed to verify the conclusions further, and basic research is warranted to elucidate the mechanisms.
Data availability statement
The original contributions presented in the study are included in the article/Supplementary material, further inquiries can be directed to the corresponding authors.
Ethics statement
The studies involving humans were approved by Medical Ethics Committee of Dehua County Hospital. The studies were conducted in accordance with the local legislation and institutional requirements. Written informed consent for participation was not required from the participants or the participants’ legal guardians/next of kin because this was a retrospective study and informed consent was not required according to Chinese law.
Author contributions
C-YW: Conceptualization, Data curation, Investigation, Software, Visualization, Writing – original draft, Writing – review & editing. S-ZL: Data curation, Investigation, Methodology, Writing – original draft. B-CK: Data curation, Methodology, Visualization, Writing – original draft. Y-ZL: Data curation, Formal Analysis, Methodology, Writing – original draft. C-JC: Data curation, Investigation, Resources, Visualization, Writing – original draft. X-BH: Conceptualization, Data curation, Methodology, Writing – original draft. J-QW: Formal Analysis, Supervision, Writing – original draft, Writing – review & editing.
Funding
The author(s) declare that no financial support was received for the research, authorship, and/or publication of this article.
Conflict of interest
The authors declare that the research was conducted in the absence of any commercial or financial relationships that could be construed as a potential conflict of interest.
Publisher’s note
All claims expressed in this article are solely those of the authors and do not necessarily represent those of their affiliated organizations, or those of the publisher, the editors and the reviewers. Any product that may be evaluated in this article, or claim that may be made by its manufacturer, is not guaranteed or endorsed by the publisher.
Supplementary material
The Supplementary material for this article can be found online at: https://www.frontiersin.org/articles/10.3389/fneur.2024.1374198/full#supplementary-material
References
1. Gregson, BA, Broderick, JP, Auer, LM, Batjer, H, Chen, XC, Juvela, S, et al. Individual patient data subgroup meta-analysis of surgery for spontaneous supratentorial intracerebral hemorrhage. Stroke. (2012) 43:1496–504. doi: 10.1161/STROKEAHA.111.640284
2. Sheth, KN. Spontaneous intracerebral hemorrhage. N Engl J Med. (2022) 387:1589–96. doi: 10.1056/NEJMra2201449
3. Morotti, A, Boulouis, G, Nawabi, J, Li, Q, Charidimou, A, Pasi, M, et al. Using noncontrast computed tomography to improve prediction of intracerebral hemorrhage expansion. Stroke. (2023) 54:567–74. doi: 10.1161/STROKEAHA.122.041302
4. Hawkes, MA, and Rabinstein, AA. Acute hypertensive response in patients with acute intracerebral hemorrhage: a narrative review. Neurology. (2021) 97:316–29. doi: 10.1212/WNL.0000000000012276
5. Dowlatshahi, D, Demchuk, AM, Flaherty, ML, Ali, M, Lyden, PL, and Smith, EE. VISTA collaboration defining hematoma expansion in intracerebral hemorrhage: relationship with patient outcomes. Neuology. (2011) 76:1238–44. doi: 10.1212/WNL.0b013e3182143317
6. Li, Q, Warren, AD, Qureshi, AI, Morotti, A, Falcone, GJ, Sheth, KN, et al. Ultra-early blood pressure reduction attenuates hematoma growth and improves outcome in intracerebral hemorrhage. Ann Neurol. (2020) 88:388–95. doi: 10.1002/ana.25793
7. Qureshi, AI, Mohammad, YM, Yahia, AM, Suarez, JI, Siddiqui, AM, Kirmani, JF, et al. A prospective multicenter study to evaluate the feasibility and safety of aggressive antihypertensive treatment in patients with acute intracerebral hemorrhage. J Intensive Care Med. (2016) 20:34–42. doi: 10.1177/0885066604271619
8. Francoeur, CL, Mayer, SA, and Collaborators, V-I. Acute blood pressure and outcome after intracerebral hemorrhage: the VISTA-ICH cohort. J Stroke Cerebrovasc Dis. (2021) 30:105456. doi: 10.1016/j.jstrokecerebrovasdis.2020.105456
9. Morotti, A, Boulouis, G, Romero, JM, Brouwers, HB, Jessel, MJ, Vashkevich, A, et al. ATACH-II and NETT investigators blood pressure reduction and noncontrast CT markers of intracerebral hemorrhage expansion. Neuology. (2017) 89:548–54. doi: 10.1212/WNL.0000000000004210
10. Morotti, A, Boulouis, G, Dowlatshahi, D, Li, Q, Shamy, M, Al-Shahi Salman, R, et al. Intracerebral haemorrhage expansion: definitions, predictors, and prevention. Lancet Neurol. (2023) 22:159–71. doi: 10.1016/S1474-4422(22)00338-6
11. Moullaali, TJ, Wang, X, Sandset, EC, Woodhouse, LJ, Law, ZK, Arima, H, et al. Early lowering of blood pressure after acute intracerebral haemorrhage: a systematic review and meta-analysis of individual patient data. J Neurol Neurosurg Psychiatry. (2022) 93:6–13. doi: 10.1136/jnnp-2021-327195
12. Hemphill, JC, Greenberg, SM, Anderson, CS, Becker, K, Bendok, BR, Cushman, M, et al. Guidelines for the Management of Spontaneous Intracerebral Hemorrhage: a guideline for healthcare professionals from the American Heart Association/American Stroke Association. Stroke. (2015) 46:2032–60. doi: 10.1161/STR.0000000000000069
13. James, PA, Oparil, S, Carter, BL, Cushman, WC, Dennison-Himmelfarb, C, Handler, J, et al. 2014 evidence-based guideline for the management of high blood pressure in adults: report from the panel members appointed to the eighth joint National Committee (JNC 8). JAMA. (2014) 311:507–20. doi: 10.1001/jama.2013.284427
14. Glasser, SP, Halberg, DL, Sands, CD, Mosher, A, Muntner, PM, and Howard, G. Is pulse pressure an independent risk factor for incident stroke, REasons for geographic and racial differences in stroke. Am J Hypertens. (2015) 28:987–94. doi: 10.1093/ajh/hpu265
15. Ito, S. Cardiorenal syndrome: an evolutionary point of view. Hypertension. (2012) 60:589–95. doi: 10.1161/HYPERTENSIONAHA.111.188706
16. Park, JH, Lee, J, Kwon, SU, Sung Kwon, H, Hwan Lee, M, and Kang, DW. Elevated pulse pressure and recurrent hemorrhagic stroke risk in stroke with cerebral microbleeds or intracerebral hemorrhage. J Am Heart Assoc. (2022) 11:e022317. doi: 10.1161/JAHA.121.022317
17. Poels, MM, Zaccai, K, Verwoert, GC, Vernooij, MW, Hofman, A, van der Lugt, A, et al. Arterial stiffness and cerebral small vessel disease: the Rotterdam scan study. Stroke. (2012) 43:2637–42. doi: 10.1161/STROKEAHA.111.642264
18. Huang, Y-H, Lee, T-H, and Ting, C-W. Exploring the relationship between admission pulse pressure and clinical features in patients with spontaneous supratentorial intracerebral hemorrhage. Neurosurg Rev. (2023) 47:19. doi: 10.1007/s10143-023-02256-1
19. Larsen, KT, Selseth, MN, Jahr, SH, Hillestad, V, Koubaa, N, Sandset, EC, et al. Prehospital blood pressure and clinical and radiological outcomes in acute spontaneous intracerebral hemorrhage. Stroke. (2022) 53:3633–41. doi: 10.1161/STROKEAHA.121.038524
20. Falcone, GJ, Biffi, A, Brouwers, HB, Anderson, CD, Battey, TW, Ayres, AM, et al. Predictors of hematoma volume in deep and lobar supratentorial intracerebral hemorrhage. JAMA Neurol. (2013) 70:988–94. doi: 10.1001/jamaneurol.2013.98
21. Zhang, YB, Zheng, SF, Yao, PS, Chen, GR, Li, GH, Li, SC, et al. Lower ionized calcium predicts hematoma expansion and poor outcome in patients with hypertensive intracerebral hemorrhage. World Neurosurg. (2018) 118:e500–4. doi: 10.1016/j.wneu.2018.06.223
22. Qureshi, AI, Palesch, YY, Barsan, WG, Hanley, DF, Hsu, CY, Martin, RL, et al. Intensive blood-pressure lowering in patients with acute cerebral hemorrhage. N Engl J Med. (2016) 375:1033–43. doi: 10.1056/NEJMoa1603460
23. Yang, P, Zhang, Y, Zhang, L, Zhang, Y, Treurniet, KM, Chen, W, et al. Endovascular thrombectomy with or without intravenous alteplase in acute stroke. N Engl J Med. (2020) 382:1981–93. doi: 10.1056/NEJMoa2001123
24. Wang, CY, Zhang, YB, Wang, JQ, Zhang, XT, Pan, ZM, and Chen, LX. Association between serum lactate dehydrogenase level and hematoma expansion in patients with primary intracerebral hemorrhage: a propensity-matched analysis. World Neurosurg. (2022) 160:e579–90. doi: 10.1016/j.wneu.2022.01.080
25. Tang, KS, Medeiros, ED, and Shah, AD. Wide pulse pressure: a clinical review. J Clin Hypertens (Greenwich). (2020) 22:1960–7. doi: 10.1111/jch.14051
26. Smith, NL, Chen, MH, Dehghan, A, Strachan, DP, Basu, S, Soranzo, N, et al. Novel associations of multiple genetic loci with plasma levels of factor VII, factor VIII, and von Willebrand factor: the CHARGE (cohorts for heart and aging research in genome epidemiology) consortium. Circulation. (2010) 121:1382–92. doi: 10.1161/CIRCULATIONAHA.109.869156
27. Keep, RF, Xiang, J, Ennis, SR, Andjelkovic, A, Hua, Y, Xi, G, et al. Blood–brain barrier function in intracerebral hemorrhage. Acta Neurochir Suppl. (2008) 105:73–7. doi: 10.1007/978-3-211-09469-3_15
28. Wang, J. Preclinical and clinical research on inflammation after intracerebral hemorrhage. Prog Neurobiol. (2010) 92:463–77. doi: 10.1016/j.pneurobio.2010.08.001
29. Qureshi, AI, Suri, MFK, Ostrow, PT, Kim, SH, Ali, Z, Shatla, AA, et al. Apoptosis as a form of cell death in intracerebral hemorrhage. Neurosurgery. (2003) 52:1041–8.
30. Fisher, CM. Pathological observations in hypertensive cerebral hemorrhage. J Neuropathol Exp Neurol. (1971) 30:536–50. doi: 10.1097/00005072-197107000-00015
31. Wang, J, and Dore, S. Inflammation after intracerebral hemorrhage. J Cereb Blood Flow Metab. (2007) 27:894–908. doi: 10.1038/sj.jcbfm.9600403
32. Yakushiji, Y, Nishiyama, M, Yakushiji, S, Hirotsu, T, Uchino, A, Nakajima, J, et al. Brain microbleeds and global cognitive function in adults without neurological disorder. Stroke. (2008) 39:3323–8. doi: 10.1161/STROKEAHA.108.516112
Keywords: stroke, cerebral hemorrhage, blood pressure, hematoma growth, predictor, dose–response relationship
Citation: Wang C-Y, Lai S-Z, Kang B-C, Lin Y-Z, Cao C-J, Huang X-B and Wang J-Q (2024) Association of pulse pressure with hematoma expansion in patients with spontaneous supratentorial intracerebral hemorrhage. Front. Neurol. 15:1374198. doi: 10.3389/fneur.2024.1374198
Edited by:
Pradeep Kumar, All India Institute of Medical Sciences, IndiaReviewed by:
Ruoran Wang, Sichuan University, ChinaArchana Hinduja, The Ohio State University, United States
Copyright © 2024 Wang, Lai, Kang, Lin, Cao, Huang and Wang. This is an open-access article distributed under the terms of the Creative Commons Attribution License (CC BY). The use, distribution or reproduction in other forums is permitted, provided the original author(s) and the copyright owner(s) are credited and that the original publication in this journal is cited, in accordance with accepted academic practice. No use, distribution or reproduction is permitted which does not comply with these terms.
*Correspondence: Chao-Ying Wang, Wcy_19870520@163.com; Bao-Cai Kang, kbc036@163.com
†These authors have contributed equally to this work