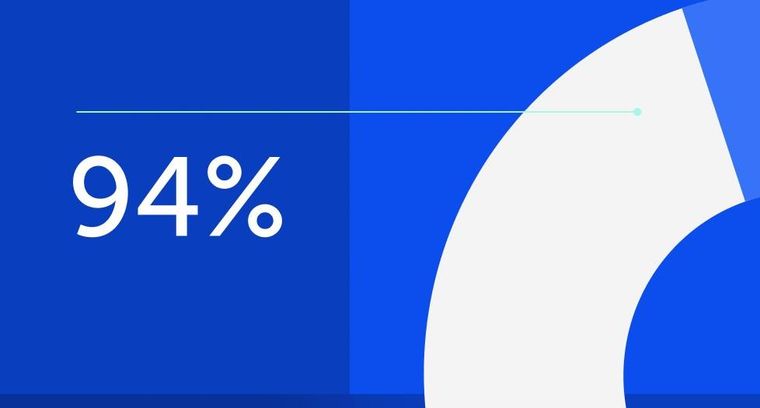
94% of researchers rate our articles as excellent or good
Learn more about the work of our research integrity team to safeguard the quality of each article we publish.
Find out more
REVIEW article
Front. Neurol., 06 May 2024
Sec. Headache and Neurogenic Pain
Volume 15 - 2024 | https://doi.org/10.3389/fneur.2024.1366372
Migraine is a highly prevalent disease worldwide, imposing enormous clinical and economic burdens on individuals and societies. Current treatments exhibit limited efficacy and acceptability, highlighting the need for more effective and safety prophylactic approaches, including the use of nutraceuticals for migraine treatment. Migraine involves interactions within the central and peripheral nervous systems, with significant activation and sensitization of the trigeminovascular system (TVS) in pain generation and transmission. The condition is influenced by genetic predispositions and environmental factors, leading to altered sensory processing. The neuroinflammatory response is increasingly recognized as a key event underpinning the pathophysiology of migraine, involving a complex neuro-glio-vascular interplay. This interplay is partially mediated by neuropeptides such as calcitonin gene receptor peptide (CGRP), pituitary adenylate cyclase activating polypeptide (PACAP) and/or cortical spreading depression (CSD) and involves oxidative stress, mitochondrial dysfunction, nucleotide-binding domain-like receptor family pyrin domain containing-3 (NLRP3) inflammasome formation, activated microglia, and reactive astrocytes. Omega-3 polyunsaturated fatty acids (PUFAs), particularly eicosapentaenoic acid (EPA) and docosahexaenoic acid (DHA), crucial for the nervous system, mediate various physiological functions. Omega-3 PUFAs offer cardiovascular, neurological, and psychiatric benefits due to their potent anti-inflammatory, anti-nociceptive, antioxidant, and neuromodulatory properties, which modulate neuroinflammation, neurogenic inflammation, pain transmission, enhance mitochondrial stability, and mood regulation. Moreover, specialized pro-resolving mediators (SPMs), a class of PUFA-derived lipid mediators, regulate pro-inflammatory and resolution pathways, playing significant anti-inflammatory and neurological roles, which in turn may be beneficial in alleviating the symptomatology of migraine. Omega-3 PUFAs impact various neurobiological pathways and have demonstrated a lack of major adverse events, underscoring their multifaceted approach and safety in migraine management. Although not all omega-3 PUFAs trials have shown beneficial in reducing the symptomatology of migraine, further research is needed to fully establish their clinical efficacy and understand the precise molecular mechanisms underlying the effects of omega-3 PUFAs and PUFA-derived lipid mediators, SPMs on migraine pathophysiology and progression. This review highlights their potential in modulating brain functions, such as neuroimmunological effects, and suggests their promise as candidates for effective migraine prophylaxis.
Migraine, a disorder influenced by genetics and characterized by multiple factors involving neurological, glial, and vascular systems, ranks as the second most debilitating condition worldwide, impacting the health of over one billion individuals globally (1). While there are established pharmacological guidelines for the prevention of migraines (2, 3), a significant number of patients still experience unmet needs, with many not benefiting from or failing to respond to preventative treatments (4, 5).
Migraine manifests as recurrent bouts of moderate to severe headache lasting between 4 to 72 h, typically one-sided, and accompanied by nausea, sensitivity to light (photophobia), sensitivity to sound (phonophobia), and/or vomiting (6, 7). Its underlying mechanisms are multifaceted, encompassing genetic variations, environmental influences, cerebral artery constriction, abnormalities in monoamine neurotransmitters, oxidative stress, mitochondrial disturbances, the involvement of calcitonin gene-related peptide (CGRP), neurogenic inflammation, and broader neuroinflammation (6). The once-prevailing vascular theory, which posited that headaches stem from dilation of dural and extracranial blood vessels, has been refuted, with such vasodilation now regarded as an effect rather than a cause of migraines (8). The prevailing theory suggests that migraine is a neurovascular disorder initiated within the central nervous system (CNS), characterized by an increased sensitivity of the trigeminovascular system (TVS) leading to pain. From a neurobiological standpoint, the development and intensification of migraine into a debilitating condition involve intricate interactions between neurons, glial cells, vascular components, and inflammatory mediators within the TVS (6, 9–12). The interactions within the TVS, immune cells, and ongoing neurogenic or neuroinflammatory responses play significant roles in migraine pathophysiology, potentially leading to the establishment of chronic maladaptive pain.
Omega-3 polyunsaturated fatty acids (PUFAs), such as eicosapentaenoic acid (EPA) and docosahexaenoic acid (DHA), are vital micronutrients for neurological health. These fatty acids play a key role in numerous physiological processes and have shown benefits in managing cardiovascular, neurological, and psychiatric conditions, demonstrating a well-tolerated side effect profile (13–15). Specifically, omega-3 PUFAs and their derivatives exhibit strong anti-inflammatory, anti-nociceptive, and neuromodulatory effects, impacting the pathophysiology of migraines (14, 16, 17). Moreover, various specialized pro-resolving mediators (SPMs) produced from omega-3 PUFAs help in moderating undue neuroinflammatory responses and inflammatory pain, facilitating communication between glial cells and neurons. This could significantly contribute to the mitigation of migraine symptoms (18). This paper aims to outline the neuroimmunological actions of omega-3 PUFAs in migraine prevention and offer an up-to-date review on this subject.
Migraines can be categorized into five distinct phases: the prodrome, a possible aura, the headache attack, the postdrome (recovery stage), and the interictal phase. However, not all individuals experience every stage (19). The prodrome, marking the initial clinical phase of a migraine, manifests through symptoms believed to result from hypothalamic activation, such as difficulty concentrating, fatigue, yawning, neck stiffness, feelings of depression, and irritability (20, 21). In the lead-up to the migraine, alterations in the connectivity between the hypothalamus, brainstem, spinal trigeminal nuclei, and the dorsal rostral pons in the hindbrain have been noted as early as 48 h before the pain onset. Additionally, specific brainstem activations have been recorded 24 h before the headache begins (22, 23). These findings suggest that the genesis of migraine attacks is associated with intrinsic brain dysfunction in more central regions, including the diencephalic nuclei.
The aura phenomenon, representing a temporary dysfunction of the CNS, occurs in 25% of migraines (22, 23). Cortical spreading depression (CSD), characterized by a strong wave of neuronal depolarization accompanied by glial and vascular activation, is widely believed to underlie the aura phase of migraines (24). During CSD, an excitatory wave sweeps across the cerebral cortex, altering the cerebral blood supply, enhancing neuronal excitability, and triggering the release of neuroactive peptides. This results in focal hyperemia with increased tissue metabolism that persists for minutes and is succeeded by a wave of neuronal depolarization and significant oligemia (25). CSD has been demonstrated to activate the trigeminovascular system (TVS) both centrally and peripherally by exposing the dura to inflammatory molecules, indicating that susceptibility to CSD may contribute to the initiation of migraine attacks (26, 27). Furthermore, migraines with aura have been linked to neuroinflammation, as evidenced by preclinical models and imaging studies of the human migraine condition (28).
The headache phase follows the prodromal or aura stages of a migraine, marked by the activation and sensitization of the trigeminovascular system (TVS) and brainstem, leading to disrupted sensory processing and various neurological symptoms indicative of altered brain excitability (29). The activation of TVS pain pathways triggers the release of pro-inflammatory neuropeptides and neurotransmitters such as CGRP, substance P, PACAP, VIP, and NO. These molecules interact with receptors on meningeal blood vessels and the dura mater, causing vasodilation, activation of nociceptive fibers, and an inflammatory response (8, 12). CGRP, a potent vasodilator presents in both peripheral and central neurons, plays a crucial role in neurogenic inflammation and sensitization (30). CGRP released from cutaneous trigeminal nerve fibers and acts on surrounding Schwann cells, perpetuating cutaneous allodynia through a cyclic AMP-dependent mechanism that generates NO in endosomes (31). Inflammatory by-products and oxidative stress can stimulate CGRP receptors, indicating their role as detectors of environmental oxidative states (32). Signals from dural perivascular neurons instigate a sequence of events that lead to the release of inflammatory mediators, sterile meningeal inflammation, and sensitization of pain-processing brain regions (33). Afferent fibers from the trigeminal ganglion synapse on neurons in the trigeminal cervical complex (2), and ascending pathways transmit signals to various brain regions, causing pain, cutaneous allodynia, and sensory disturbances like photophobia and phonophobia (6, 34).
The transition from episodic to chronic migraine involves changes in nociceptive thresholds and central sensitization, driven by factors such as high frequency of migraine attacks, overuse of acute medications, obesity, stress, female sex, psychiatric conditions, and low socioeconomic status (34). Significant brain alterations in pain-processing areas, abnormal pain modulation, central sensitization, cortical hyperexcitability, neurogenic inflammation, and ongoing neuroinflammation are central to the development and persistence of chronic migraines (35). Collectively, these findings suggest that in individuals with a genetic predisposition, the pathophysiology of migraine is characterized by repeated activation and sensitization of trigeminal nerves by meningeal inflammation, influenced by significant brain activity and resulting in structural and functional brain changes (36).
Neurogenic inflammation is defined as an acute sterile inflammation triggered by the release of neuropeptides from peripheral nociceptive fibers, leading to plasma protein extravasation and vasodilation (10). The relevance of dural neurogenic inflammation as an initiator and driver of acute migraine attacks has been proposed for decades. Neurogenic inflammatory reactions occur after activation of the TVS in the dura matter due to electrical, chemical stimulation, or the application of an inflammatory soap, rather than by immunological events or pathological microorganisms. Evidence has demonstrated that local sterile meningeal inflammation plays a role in inducing prolonged activation and sensitization of meningeal afferents (5). Neurogenic inflammation in acute migraine attacks is characterized by a TVS activation-induced release of neuropeptides, such as CGRP and substance P, from trigeminal nerve fibers at the dura, which causes increased vascular permeability, plasma protein extravasation, leukocyte infiltration, vasodilation, mast cell degranulation, activation of meningeal nociceptors, and the subsequent release of inflammatory mediators (5). Antidromic axon reflexes may then cause further release of CGRP in the dura (30). Prolonged TVS activation may result in a sustained release of CGRP. The continued activation of C-fibers during recurrent migraine attacks and the ensuing activation of CGRP-related nociception maintain TVS sensitization, thereby promoting migraine chronicity (5, 30).
Neurogenic inflammation refers to an acute, non-infectious inflammation initiated by neuropeptide release from peripheral pain-sensing fibers, causing plasma protein leakage and blood vessel expansion (10). The concept of neurogenic inflammation within the dura mater serving as a catalyst and perpetuator of acute migraine episodes has been considered for many years. This type of inflammation is triggered in the dura mater by activation of the trigeminovascular system (TVS) through electrical or chemical stimuli, or the application of an inflammatory substance, rather than immune or microbial factors. Research indicates that localized sterile inflammation in the meninges contributes to the prolonged activation and sensitization of meningeal sensory nerves (10). In the context of acute migraine, neurogenic inflammation involves the release of neuropeptides such as CGRP and substance P by trigeminal nerve endings within the dura mater. This release leads to increased vascular permeability, plasma protein extravasation, white blood cell infiltration, vasodilation, mast cell breakdown, stimulation of meningeal pain receptors, and further release of inflammatory substances (10). Additionally, antidromic axon reflexes could trigger additional CGRP release in the dura (33). Continuous TVS activation might cause an ongoing discharge of CGRP. The repetitive triggering of C-fibers during migraine attacks and the resultant stimulation of CGRP-mediated pain pathways keep the TVS in a state of heightened sensitivity, thus contributing to the progression of migraine into a chronic condition (10, 33).
Neuroinflammation refers to an inflammatory reaction within the CNS, regulated by cytokines, chemokines, reactive oxygen species (ROS), and secondary messengers such as nitric oxide (NO) and prostaglandins (37). These compounds are generated by activated microglia — the CNS’s primary immune cells — and astrocytes, as well as by neurovascular units consisting of neurons, pericytes, and endothelial cells (38). Neuroinflammatory mechanisms have been implicated in both episodic and chronic forms of migraine, regardless of the presence of aura (10).
Within the trigeminal ganglion, neurons and surrounding satellite glial cells engage in mutual communication during activation of the TVS. CGRP, when released from the bodies or axonal swellings of stimulated trigeminal neurons, can trigger satellite glial cells within the trigeminal ganglia, as well as microglia and astrocytes in the trigeminal nucleus caudalis. This interaction leads to the glial cells producing inflammatory cytokines and NO, which in turn further stimulates trigeminal neurons (10, 39, 40). The presence of cytokines and NO increases CGRP release, forming a self-reinforcing loop within the ganglion. Additionally, direct neuron–glia communication is facilitated through two-way calcium signaling, primarily via purinergic P2 receptors (notably P2X7) and gap junctions (39). This intricate neuron–glia dialogue intensifies pain signaling and contributes to central sensitization (41, 42).
The consensus is that CSD triggers the trigeminovascular system, leading to a meningeal inflammatory response (43). CSD is characterized by a propagating wave of neuronal and glial depolarization driven by significant ion movements across the cortex, which subsequently results in prolonged suppression of neuronal activity. This mechanism can perpetuate the activation and sensitization of meningeal pain receptors through the release of protons, potassium ions, and glutamate. Such releases promote local neurogenic inflammation and activate the trigeminovascular system (10). The ensuing broad activation of ion pumps, coupled with elevated metabolism and oxygen demand, may cause brain tissue hypoxia.
Cellular oxygen deficiency hampers the mitochondrial electron transport chain, restricting or stopping mitochondrial oxidative phosphorylation. This leads to the accumulation of excess oxygen radicals, contributing to oxidative stress and further triggering CSD (44). Research has linked migraine to mitochondrial dysfunction, evidenced by reduced mitochondrial membrane potential and the dysregulated activity of mitochondrial permeability transition pores. This dysfunction results in neuronal energy depletion, cell death, reduced pain tolerance, and migraine episodes (45). Therefore, a combination of brain energy shortage, mitochondrial dysfunction, and oxidative stress surpassing the body’s antioxidant defenses is recognized as a key element in migraine pathophysiology (45, 46).
At the cellular level, CSD can incite neuroinflammation through a series of interactions involving the pannexin-1 channel, caspase-1, interleukin (IL) 1β, and high-mobility group box 1 in neurons. Additionally, there is nuclear factor-κB-dependent upregulation of inflammatory genes, such as cyclooxygenase and inducible nitric oxide synthase, in astrocytes. These mechanisms lead to the prolonged activation of trigeminal neurons, astrocytes, and microglia around meningeal blood vessels, inflammatory cascades within brain parenchyma reaching the glia limitans, and the dispersal of arachidonic acid (AA) and potassium ions into the perivascular area (9, 10, 39). Simultaneously, the assembly and activation of the NLRP3 (nucleotide-binding domain-like receptor family pyrin domain containing-3) inflammasome complex, regulated by NF-kB, are enhanced in neurons, microglia, and astrocytes within the trigeminal ganglia, trigeminal nucleus caudalis, and cortical areas (9, 10, 39, 47). The NLRP3 inflammasome, a cytosolic protein complex, upon activation, leads to the activation of proinflammatory caspase-1 (9). Activated caspase-1 fosters inflammation through several pathways: (1) it facilitates the maturation and release of proinflammatory cytokines (IL-1β and IL-18); (2) it activates caspase-3, an enzyme inducing apoptosis; and (3) it triggers pyroptosis through activating the protein gasdermin D, hence initiating neuro-inflammatory signals within the brain parenchyma (10, 48). These processes are believed to play a role in central sensitization, contributing to the onset, progression, and intensification of migraine symptoms (10). Collectively, the neuro-inflammatory response is emerging as a pivotal element in the pathophysiology of migraine, characterized by intricate interactions within the neuro-glio-vascular network. This response is partially mediated by CGRP and/or CSD, and involves oxidative stress, mitochondrial dysfunction, the assembly of NLRP3 inflammasomes, activation of microglia, and the reactivity of astrocytes.
Two vital types of polyunsaturated fatty acids (PUFAs) are omega-3 and omega-6 PUFAs, named for the position of the first double bond in relation to the end methyl group. Both omega-3 and omega-6 PUFAs play significant roles in cardiovascular, inflammatory, metabolic, neurological, and psychiatric health (14, 49, 50). Omega-3 PUFAs originate from alpha-linolenic acid (ALA), while omega-6 PUFAs come from linoleic acid (LA). These are deemed essential fatty acids because they must be acquired through the diet, as the human body cannot synthesize them independently.
Alpha-linolenic acid (ALA) and linoleic acid (LA) undergo competitive metabolism through two distinct enzymatic pathways. In the liver, a sequence of desaturation, elongation, and β-oxidation reactions converts ALA into EPA and docosahexaenoic acid (DHA), while LA is transformed into AA (51). The absence of desaturase enzymes necessary for the interconversion between omega-6 and omega-3 PUFAs renders these two families of PUFAs metabolically distinct and potentially opposed in their physiological functions (52). Omega-3 PUFAs are crucial for brain development and function. DHA represents the most significant omega-3 PUFA in the brain, comprising over 40% of the brain’s total omega-3 content, especially concentrated within the gray matter (13). It plays vital roles in neurotransmission, neuroplasticity, and signal transduction (53). Specifically, DHA significantly influences the biophysical characteristics of neuronal and glial cell membranes, facilitating immunomodulatory effects (13, 54). Both DHA and EPA act as natural ligands for various nuclear receptors, including peroxisome proliferator-activated receptors, which are abundantly present in microglia and pivotal for the transcriptional regulation of many cellular activities, such as lipid balance and inflammation control (54).
Omega-3 and omega-6 polyunsaturated fatty acids (PUFAs) play distinct roles in inflammation, with omega-3 PUFAs generally exhibiting anti-inflammatory effects and omega-6 PUFAs tending towards pro-inflammatory actions, through competitive metabolic processes. They act as precursors for various bioactive lipid mediators, known collectively as oxylipins (55). The production of oxylipins from tissue PUFAs depends on both the dietary intake of these fatty acids and their local availability for incorporation into cell membrane phospholipids during metabolic processing (55). Despite the liver and brain’s capacity to convert ALA into DHA, the endogenous synthesis of EPA and DHA in the brain is relatively low, necessitating their active uptake from the bloodstream (54, 56). The modern diet, which typically contains up to 15 times more omega-6 PUFAs than omega-3 PUFAs, along with a common deficiency of DHA in the brain, results in the production of large amounts of lipid mediators derived from LA and AA, contributing to chronic inflammation and oxidative stress implicated in cardiovascular, metabolic, inflammatory, and neuropsychiatric disorders (18, 50, 57–59).
PUFAs are released from cell membranes by the action of phospholipase enzymes, such as phospholipase A2 and phospholipase C, and are then converted into various bioactive compounds by cyclooxygenase, lipoxygenase, and cytochrome P450 enzymes. The predominant oxylipins include AA derivatives like prostaglandins, thromboxanes, and leukotrienes (60). EPA can be transformed into three-series prostaglandins, thromboxanes, five-series leukotrienes, endocannabinoids, eicosapentanoyl glycerol, and eicosapentaenoyl ethanolamide, while DHA gives rise to docosahexaenoyl ethanolamide and docosahexaenoyl glycerol (49). Despite structural similarities to AA-derived eicosanoids, some EPA-derived eicosanoids are potent anti-inflammatory and anti-nociceptive agents. Oxylipins regulate a broad range of physiological processes, including cell death, tissue repair, coagulation, cell proliferation, vascular permeability, pain, inflammation, immune functions, and blood pressure (55).
Specialized pro-resolving mediators (SPMs) are a class of PUFA-derived lipid mediators (49). Different SPMs are derived from differing PUFA substrates: E-series resolvins (RvE1, RvE2, and RvE3) are derived from EPA; D-series resolvins (RvD1, RvD2, RvD3, RvD4), protectins (protectin D1, a.k.a. neuroprotectin D1 when formed in the nervous system), and maresins are derived from DHA; and lipoxins are derived from AA (49, 61). SPMs are critical for resolution of inflammation, pain reduction, and tissue regeneration via specific cellular and molecular mechanisms (62). During the resolution of inflammation, SPMs produced by polymorphonuclear cells and macrophages act to limit local tissue damage caused by inflammatory responses, including signaling cessation of polymorphonuclear cell infiltration, macrophage switching to an anti-inflammatory phenotype, apoptotic cell clearance, and reducing pain caused by nociceptor sensitization (49, 61). SPMs can decrease levels of pro-inflammatory cytokines, such as IL-1β, IL-6, and tumor necrosis factor (TNF)-α, through activating their G protein-coupled receptors, thus causing activation of anti-inflammatory transcription factors, such as peroxisome proliferator-activated receptor gamma, and down-regulating intracellular NF-kB signaling pathway (14, 63). SPMs have both anti-inflammatory and pro-resolving properties without immune suppression. Although these activities are distinct, they act in tandem to promote defense from injury, inflammation, and eventually restoration of tissue homeostasis.
Astrocytes, which hold about 10–12% of the brain’s DHA, alongside oligodendrocytes and microglia, which account for 5% and 2%, respectively (64), express specific receptors for SPMs. These receptors, such as ALX/FPR2 and ChemR23/ERV1 on astrocytes, play crucial roles in controlling astrogliosis, inhibiting inflammation, and supporting neuroprotection. Microglia express a wide array of SPM receptors, whose activation encourages anti-inflammatory responses and homeostatic restoration. Thus, omega-3 PUFA-derived SPMs effectively balance the neuroinflammatory and pain processes in the interaction between glial cells and neurons (18).
Although the effects of omega-3 PUFAs on ROS production within mitochondria have yielded mixed results, omega-3 PUFAs are known to influence mitochondrial function in several ways, including impacts on membrane potential, respiration rates, the activity of mitochondrial complexes, and ROS production. These effects are linked to alterations in mitochondrial structure, such as changes in membrane phospholipid composition, viscosity, and the structure and function of lipid microdomains, which in turn affect membrane-bound signaling proteins (65, 66). Moreover, omega-3 PUFAs can enhance a cell’s endogenous antioxidant capabilities through multiple mechanisms: they can increase the activity of enzymes involved in antioxidant defense, such as glutathione peroxidase and superoxide dismutase, thus enhancing resistance to ROS-induced damage and reducing lipid peroxidation; inhibit the activity of cyclooxygenase-2 enzyme, reducing the production of pro-inflammatory and pro-oxidant prostaglandins along with lipid peroxidation; and elevate the expression of nuclear factor-erythroid 2-related factor 2 (Nrf2), a key transcription factor that governs the expression of a wide array of antioxidant and anti-inflammatory genes (65, 67–69). Therefore, omega-3 PUFAs play a critical role in supporting mitochondrial dynamics, biogenesis, structural organization, and the kinetics of mitochondrial respiration.
Elevated levels of systemic inflammation, neuroinflammation, and hyperactivity of the hypothalamic–pituitary–adrenal (HPA) axis have been identified as key factors contributing to the pathogenesis of neuropsychiatric disorders, including depression and anxiety (70–74). These disorders, alongside post-traumatic stress disorder, are prevalent psychiatric conditions co-occurring with migraine, significantly influencing the process of migraine becoming chronic (75, 76). Omega-3 polyunsaturated fatty acids (PUFAs) potentially modulate neurobiological pathways implicated in the coexistence of migraine with depression and/or anxiety through several mechanisms. Firstly, by dampening inflammatory responses integral to the pathophysiology of depression and anxiety, omega-3 PUFAs and their metabolites can inhibit leukocyte chemotaxis, reduce the expression of adhesion molecules, decrease the production of pro-inflammatory mediators, and encourage a shift in microglia polarization towards a reparative, non-inflammatory state (75, 76). Furthermore, they may modulate inflammatory cytokine signaling that affects glucocorticoid receptor functionality and the negative feedback control of the HPA axis; the down-regulation of inflammatory markers by omega-3 PUFAs enhances the responsiveness of the HPA-axis to negative feedback, thereby curbing HPA axis overactivity (70).
The endocannabinoid system, which is intrinsically linked to dietary lipids, neuroplasticity, and mood regulation, plays roles in synaptic plasticity, learning, memory, neuroinflammation, pain management, stress responses, and immune function (77). SPMs derived from omega-3 PUFAs have been shown to influence depressive and anxiety-related behaviors through modulation of several molecular signaling pathways, including mTOR, MAP/ERK, NF-kB, PI3K/Akt, and AMPA (14, 59). The endocannabinoids produced from arachidonic acid (AA) include anandamide and 2-arachidonoylglycerol, whereas those from omega-3 PUFAs include docosahexaenoyl ethanolamide (from DHA) and oleylethanolamide and palmitoylethanolamide (from EPA) (78). These endocannabinoids primarily target CB1 and CB2 receptors found in microglia, glial cells, and neurons (54). Endocannabinoids derived from omega-3 PUFAs serve as precursors to more potent bioactive compounds that operate via both cannabinoid-dependent and independent pathways, exerting anti-inflammatory, anti-proliferative, and mood-regulating effects (59, 79). Therefore, omega-3 PUFAs act as immunomodulatory agents that play a significant role in the regulation of inflammation and mood.
Overall, omega-3 PUFAs and their metabolites play an essential role in neuropsychoimmunology, influencing a range of brain processes including neuroinflammation, pain signaling, mitochondrial function, oxidative stress, and mood regulation. These aspects are deeply interconnected with the underlying mechanisms of migraine pathophysiology as depicted in Figure 1.
Figure 1. Neuropsychoimmunological impact of omega-3 PUFAs on brain functions and migraine pathophysiology.
Omega-3 PUFAs play a pivotal role in supporting neurological health and mitigating neuroinflammation through a series of biochemical processes. These processes include influencing membrane fluidity, generating anti-inflammatory mediators, affecting intracellular signaling, and modulating gene expression (17, 80, 81). The extensive physiological actions of omega-3 PUFAs involve neurons, microglia, and astrocytes, facilitating the modulation of neuroinflammation, pain signal propagation, mitochondrial dysfunction, oxidative stress, and mood dysregulation, all of which are integral to the complex pathology of migraines (80, 81). Specifically, omega-3 PUFAs contribute to reducing CNS levels of critical inflammatory mediators like TNF-α, cyclooxygenase-2/NO synthase, and IL-1β. These reductions are believed to alleviate neuroinflammation and neurogenic pain, crucial elements in migraine pathophysiology. Moreover, supplementation with EPA and DHA has been shown to modulate nociceptive responses, potentially through activating the opioid system, offering benefits in neuropathic pain scenarios. Additionally, these fatty acids help rebalance vital neurotransmitters, such as serotonin and dopamine, essential for the functioning of the trigeminovascular nociceptive pathway, and can inhibit TNF-α expression, potentially reducing cerebral vasodilation implicated in migraine episodes. The noted high efficacy of EPA/DHA supplementation, combined with its favorable acceptability and patient compliance, sets it apart from traditional pharmacological treatments, which often exhibit higher adverse event rates and lower adherence (81). This blend of effectiveness, safety, and tolerability highlights the potential of omega-3 PUFAs in offering a more comprehensive and patient-centric approach to migraine management, thus enhancing the quality of life for those affected by this incapacitating ailment.
Omega-3 PUFAs and their metabolites, such as oxylipins and SPMs, potentially offer relief from migraines and aid in mood regulation. They achieve this by modifying the biophysical properties of neuronal and glial cell membranes, suppressing pro-inflammatory mediators, fostering the resolution of inflammation, enhancing mitochondrial stability, and reducing reactive oxygen species (ROS) production (55). Discrepancies in clinical outcomes concerning the impact of omega-3 PUFA supplementation on the frequency, severity, and duration of migraines may be attributed to methodological variations. These include differences in dosages, PUFA ratios, treatment durations, study sample sizes, and the heterogeneity of study populations, especially concerning medical and psychiatric comorbidities (17, 80, 81).
Regarding the potential short-term and long-term side effects associated with high-dose omega-3 supplementation, concerns about bleeding risks have been meticulously evaluated. However, current research suggests that daily doses of up to 4 grams are generally safe, even in conjunction with antiplatelet or anticoagulant therapies (82). Although mild gastrointestinal symptoms might occur, severe adverse effects are rare. It’s critical to recognize that PUFAs are vulnerable to lipid oxidative degradation caused by free radicals, potentially presenting health risks over extended periods (83). Presently, there’s a lack of extensive data on the long-term safety and tolerability of high-dose, prolonged omega-3 supplementation, underscoring the need for further investigation (84). Despite these considerations, the broad neuroimmunological regulatory effects of omega-3 PUFAs make them compelling candidates as therapeutic agents or adjuncts in the management of migraines.
Omega-3 PUFAs, specifically EPA and DHA, present significant potential benefits in the prophylaxis of migraines. Emerging evidence, particularly from a comprehensive network meta-analysis (NMA), highlights that high-dosage EPA/DHA supplementation can markedly reduce the frequency and severity of migraines (85). This finding positions these omega-3 PUFAs as a promising treatment option, offering an effective alternative to the currently available pharmacological strategies which typically show limited efficacy and patient acceptability.
Djalali et al. (86) conducted a randomized, double-blind, placebo-controlled trial over 8 weeks with 40 patients prone to episodic migraines, finding that omega-3 PUFAs supplementation increased anti-inflammatory cytokine IL-4 and decreased pro-inflammatory cytokine IFN-γ levels, suggesting a potential beneficial effect on inflammatory responses in migraine patients. Rist et al. (87) utilized a two-by-two factorial design over 4.6 years with 25,871 middle-aged or older adults, including 1,032 with a history of probable migraine, and found that neither vitamin D nor marine omega-3 PUFAs supplementation affected migraine frequency or severity compared to a placebo. Honarvar et al. (88) in an 8-week randomized double-blind, placebo-controlled trial with 80 individuals experiencing episodic migraines, discovered that the combination of omega-3 PUFAs and nano-curcumin significantly reduced migraine attack frequency and serum levels of IL-1β in a synergistic manner, though the initial significant reduction in IL-1β gene expression did not remain significant after Bonferroni corrections. Soares et al. (89), through a 60-day prospective, experimental, controlled, double-blind study with 60 patients diagnosed with chronic migraine, predominantly women, observed that omega-3 PUFAs intake led to a significant reduction in headache days, indicating the benefit of omega-3 PUFAs in migraine prophylaxis. Abdolahi et al. (90) evaluated the effects of omega-3 PUFAs and nano-curcumin supplementation over 8 weeks in 80 episodic migraine patients, noting a decrease in IL-6 and hs-CRP levels, with a potential synergistic effect in the combination group. Harel et al. (91), in a 16-week randomized, double-blind, cross-over study with 27 adolescents suffering from migraines, suggested that dietary supplementation with fish oil rich in very long-chain omega-3 PUFAs could reduce the frequency, duration, and severity of migraines, a benefit also observed with olive oil. Pradalier et al. (92) conducted a 16-week randomized, double-blind, controlled trial with 196 patients, finding that omega-3 PUFAs significantly reduced the total number of migraine attacks compared to placebo, though a strong placebo effect was observed, and the study did not confirm earlier findings from smaller studies. The outcomes of these interventions have demonstrated inconsistency, with previous research predominantly focusing on mixed types of PUFAs (EPA combined with DHA) rather than examining EPA or DHA individually. Recently, Wang et al. (93) carried out a 12-week randomized, double-blind, placebo-controlled trial involving seventy individuals with episodic migraine, splitting them into two groups: 35 received 2 g of fish oil containing 1.8 g of EPA daily, while the other 35 were given 2 g of soybean oil daily as a placebo. The intervention showed significant effects, with the EPA group experiencing fewer monthly migraine days, reduced usage of headache medication, and improvements in headache severity (VAS score), disability (MIDAS score), anxiety and depression (HADS score), and quality of life (MSQ score). The main findings highlight that high-dose EPA substantially reduced migraine frequency and severity, improved psychological symptoms, and enhanced the quality of life for episodic migraine patients, suggesting its potential as an effective prophylactic, particularly for female patients.
Table 1 summarized that omega-3 fatty acids have the potential to modulate inflammatory responses and reduce migraine frequency or severity in certain populations, particularly when used in combination with other supplements like nano-curcumin. However, the effectiveness appears to be influenced by various factors including age, baseline health status, and the presence of other nutritional interventions, necessitating further research to clarify the conditions under which omega-3 supplementation might be most beneficial for migraine sufferers.
Omega-3 PUFAs could provide advantages for enhancing migraine management; however, prior randomized controlled trials, reviews, and meta-analyses have yielded inconsistent results regarding symptom improvement (17). Before omega-3 PUFAs can be endorsed for use either as a primary or supplementary option for migraine prevention, key challenges must be resolved. These include fine-tuning dosages, formulations, and the ratios of PUFAs, along with identifying the optimal durations for treatment.
Historically, omega-3 supplements were derived from fatty fish species such as salmon, mullet, and mackerel. However, increased fish demand due to the expansion of aquaculture, pressure on dwindling marine species, and marine pollution have prompted the search for alternative omega-3 PUFA sources (94). In light of these concerns, sources such as seeds, herbs, genetically engineered plants, microalgae, macroalgae, and thraustochytrids are now being investigated for the development of vegan omega-3 PUFA commercial products (83). Lipids are prone to oxidation, leading to the formation of unsaturated carbonyls and harmful by-products during extraction, storage, and processing (95). With a shift in consumer preferences towards natural products over synthetic ones, there has been an increased emphasis on incorporating natural antioxidants (94). Micro- and nano-encapsulation presents an effective strategy for shielding core PUFAs from oxygen, light, and transition metals, thereby improving their oxidative stability and bioactivities (96).
As there are no definitive markers to determine which patient groups would most benefit from this treatment method, additional research is necessary to clearly define the demographic and clinical factors linked to treatment efficacy (85). These factors include age, sex, existing comorbidities, targeted symptoms, blood biomarkers, and specific treatment protocols, before fully endorsing the use of omega-3 PUFAs either as an independent treatment or in conjunction with other medications for migraine management (80). Furthermore, conducting risk–benefit evaluations of omega-3 PUFA administration is crucial, aimed at identifying patient groups for whom omega-3 PUFA therapy would present an optimal risk–benefit balance (97).
The favorable impacts of omega-3 PUFAs on the neurovascular microenvironment indicate their potential to influence neural and immune cells in the brain through various physical and biochemical pathways, leading to outcomes such as anti-inflammatory, anti-nociceptive, antioxidative, and mood regulation. Omega-3 PUFAs might play a crucial role in sustaining both physical and mental health, as well as managing neuropsychiatric symptoms during the acute and chronic phases of migraines. Despite the lack of definitive evidence from well-conducted randomized trials on the effectiveness of regular daily omega-3 PUFA supplementation in mitigating migraine symptoms for those with episodic or chronic migraines, the overall positive effects in different areas (e.g., immune, neurological, psychiatric, and cardiovascular) suggest that omega-3 PUFAs could be considered as potential health supplements or adjuncts in migraine prevention and treatment. To fully explore and leverage the neuroimmunological effects of omega-3 PUFA bioactivities for migraine prevention and treatment, extensive epidemiological studies, experimental research, and randomized controlled trials are essential for thorough testing, validation, and effective implementation.
T-BC: Conceptualization, Data curation, Formal analysis, Investigation, Methodology, Resources, Writing – original draft. C-CY: Conceptualization, Formal analysis, Investigation, Resources, Writing – original draft. I-JT: Conceptualization, Data curation, Formal analysis, Investigation, Methodology, Writing – original draft. H-WY: Data curation, Investigation, Writing – original draft. Y-CH: Investigation, Supervision, Writing – review & editing. C-MC: Investigation, Methodology, Project administration, Supervision, Validation, Writing – review & editing. C-PY: Conceptualization, Project administration, Supervision, Validation, Writing – review & editing.
The author(s) declare that no financial support was received for the research, authorship, and/or publication of this article.
The authors declare that the research was conducted in the absence of any commercial or financial relationships that could be construed as a potential conflict of interest.
All claims expressed in this article are solely those of the authors and do not necessarily represent those of their affiliated organizations, or those of the publisher, the editors and the reviewers. Any product that may be evaluated in this article, or claim that may be made by its manufacturer, is not guaranteed or endorsed by the publisher.
1. Leonardi, M, and Raggi, A. A narrative review on the burden of migraine: when the burden is the impact on people's life. J Headache Pain. (2019) 20:41. doi: 10.1186/s10194-019-0993-0
2. Wu, JW, and Yang, CP. Treatment guideline Subcommittee of the Taiwan Headache S. 2022 Taiwan guidelines for preventive treatment of migraine. Acta Neurol Taiwanica. (2022) 31:164–202.
3. American, HS . The American headache society position statement on integrating new migraine treatments into clinical practice. Headache. (2019) 59:1–18. doi: 10.1111/head.13456
4. Delussi, M, Vecchio, E, Libro, G, Quitadamo, S, and de Tommaso, M. Failure of preventive treatments in migraine: an observational retrospective study in a tertiary headache center. BMC Neurol. (2020) 20:256. doi: 10.1186/s12883-020-01839-5
5. Park, S, Han, S, and Suh, HS. The disease burden of migraine patients receiving prophylactic treatments in Korea: a population-based claims database analysis. BMC Health Serv Res. (2022) 22:902. doi: 10.1186/s12913-022-08191-z
6. Khan, J, Asoom, LIA, Sunni, AA, Rafique, N, Latif, R, Saif, SA, et al. Genetics, pathophysiology, diagnosis, treatment, management, and prevention of migraine. Biomed Pharmacother. (2021) 139:111557. doi: 10.1016/j.biopha.2021.111557
8. Charles, A . Vasodilation out of the picture as a cause of migraine headache. Lancet Neurol. (2013) 12:419–20. doi: 10.1016/S1474-4422(13)70051-6
9. Kursun, O, Yemisci, M, van den Maagdenberg, A, and Karatas, H. Migraine and neuroinflammation: the inflammasome perspective. J Headache Pain. (2021) 22:55. doi: 10.1186/s10194-021-01271-1
10. Biscetti, L, Cresta, E, Cupini, LM, Calabresi, P, and Sarchielli, P. The putative role of neuroinflammation in the complex pathophysiology of migraine: from bench to bedside. Neurobiol Dis. (2023) 180:106072. doi: 10.1016/j.nbd.2023.106072
11. Yamanaka, G, Suzuki, S, Morishita, N, Takeshita, M, Kanou, K, Takamatsu, T, et al. Role of Neuroinflammation and blood-brain barrier Permutability on migraine. Int J Mol Sci. (2021) 22:8929. doi: 10.3390/ijms22168929
12. Spekker, E, Tanaka, M, Szabo, A, and Vecsei, L. Neurogenic inflammation: the participant in migraine and recent advancements in translational research. Biomedicines. (2021) 10:76. doi: 10.3390/biomedicines10010076
13. Dighriri, IM, Alsubaie, AM, Hakami, FM, Hamithi, DM, Alshekh, MM, Khobrani, FA, et al. Effects of Omega-3 polyunsaturated fatty acids on brain functions: a systematic review. Cureus. (2022) 14:e30091. doi: 10.7759/cureus.30091
14. Giacobbe, J, Benoiton, B, Zunszain, P, Pariante, CM, and Borsini, A. The anti-inflammatory role of Omega-3 polyunsaturated fatty acids metabolites in pre-clinical models of psychiatric, neurodegenerative, and neurological disorders. Front Psych. (2020) 11:122. doi: 10.3389/fpsyt.2020.00122
15. Mason, RP, Libby, P, and Bhatt, DL. Emerging mechanisms of cardiovascular protection for the Omega-3 fatty acid Eicosapentaenoic acid. Arterioscler Thromb Vasc Biol. (2020) 40:1135–47. doi: 10.1161/ATVBAHA.119.313286
16. Soveyd, N, Abdolahi, M, Bitarafan, S, Tafakhori, A, Sarraf, P, Togha, M, et al. Molecular mechanisms of omega-3 fatty acids in the migraine headache. Iran J Neurol. (2017) 16:210–7.
17. Cardia, L, Calapai, F, Mondello, C, Quattrone, D, Elisa Sorbara, E, Mannucci, C, et al. Clinical use of omega-3 fatty acids in migraine: a narrative review. Medicine (Baltimore). (2020) 99:e22253. doi: 10.1097/MD.0000000000022253
18. Valente, M, Dentoni, M, Bellizzi, F, Kuris, F, and Gigli, GL. Specialized pro-resolving mediators in Neuroinflammation: overview of studies and perspectives of clinical applications. Molecules. (2022) 27:4836. doi: 10.3390/molecules27154836
19. Tzankova, V, Becker, WJ, and Chan, TLH. Diagnosis and acute management of migraine. CMAJ. (2023) 195:E153–8. doi: 10.1503/cmaj.211969
20. Schulte, LH, and May, A. The migraine generator revisited: continuous scanning of the migraine cycle over 30 days and three spontaneous attacks. Brain. (2016) 139:1987–93. doi: 10.1093/brain/aww097
21. Denuelle, M, Fabre, N, Payoux, P, Chollet, F, and Geraud, G. Hypothalamic activation in spontaneous migraine attacks. Headache. (2007) 47:1418–26. doi: 10.1111/j.1526-4610.2007.00776.x
22. Puledda, F, Silva, EM, Suwanlaong, K, and Goadsby, PJ. Migraine: from pathophysiology to treatment. J Neurol. (2023) 270:3654–66. doi: 10.1007/s00415-023-11706-1
23. Schulte, LH, Mehnert, J, and May, A. Longitudinal neuroimaging over 30 days: temporal characteristics of migraine. Ann Neurol. (2020) 87:646–51. doi: 10.1002/ana.25697
24. Somjen, GG . Mechanisms of spreading depression and hypoxic spreading depression-like depolarization. Physiol Rev. (2001) 81:1065–96. doi: 10.1152/physrev.2001.81.3.1065
25. Karsan, N, Gosalia, H, and Goadsby, PJ. Molecular mechanisms of migraine: nitric oxide synthase and neuropeptides. Int J Mol Sci. (2023) 24:11993. doi: 10.3390/ijms241511993
26. Close, LN, Eftekhari, S, Wang, M, Charles, AC, and Russo, AF. Cortical spreading depression as a site of origin for migraine: role of CGRP. Cephalalgia. (2019) 39:428–34. doi: 10.1177/0333102418774299
27. Karatas, H, Erdener, SE, Gursoy-Ozdemir, Y, Lule, S, Eren-Koçak, E, Sen, ZD, et al. Spreading depression triggers headache by activating neuronal Panx1 channels. Science. (2013) 339:1092–5. doi: 10.1126/science.1231897
28. Albrecht, DS, Mainero, C, Ichijo, E, Ward, N, Granziera, C, Zürcher, NR, et al. Imaging of neuroinflammation in migraine with aura: a [(11)C]PBR28 PET/MRI study. Neurology. (2019) 92:e2038–50. doi: 10.1212/WNL.0000000000007371
29. Goadsby, PJ, Holland, PR, Martins-Oliveira, M, Hoffmann, J, Schankin, C, and Akerman, S. Pathophysiology of migraine: a disorder of sensory processing. Physiol Rev. (2017) 97:553–622. doi: 10.1152/physrev.00034.2015
30. Iyengar, S, Ossipov, MH, and Johnson, KW. The role of calcitonin gene-related peptide in peripheral and central pain mechanisms including migraine. Pain. (2017) 158:543–59. doi: 10.1097/j.pain.0000000000000831
31. de Logu, F, Nassini, R, Hegron, A, Landini, L, Jensen, DD, Latorre, R, et al. Schwann cell endosome CGRP signals elicit periorbital mechanical allodynia in mice. Nat Commun. (2022) 13:646. doi: 10.1038/s41467-022-28204-z
32. Iannone, LF, De Logu, F, Geppetti, P, and De Cesaris, F. The role of TRP ion channels in migraine and headache. Neurosci Lett. (2022) 768:136380. doi: 10.1016/j.neulet.2021.136380
33. Levy, D . Endogenous mechanisms underlying the activation and sensitization of meningeal nociceptors: the role of immuno-vascular interactions and cortical spreading depression. Curr Pain Headache Rep. (2012) 16:270–7. doi: 10.1007/s11916-012-0255-1
34. Mungoven, TJ, Henderson, LA, and Meylakh, N. Chronic migraine pathophysiology and treatment: a review of current perspectives. Front Pain Res (Lausanne). (2021) 2:705276. doi: 10.3389/fpain.2021.705276
35. Mungoven, TJ, Marciszewski, KK, Macefield, VG, Macey, PM, Henderson, LA, and Meylakh, N. Alterations in pain processing circuitries in episodic migraine. J Headache Pain. (2022) 23:9. doi: 10.1186/s10194-021-01381-w
36. Ashina, M, Hansen, JM, Do, TP, Melo-Carrillo, A, Burstein, R, and Moskowitz, MA. Migraine and the trigeminovascular system-40 years and counting. Lancet Neurol. (2019) 18:795–804. doi: 10.1016/S1474-4422(19)30185-1
37. Xanthos, DN, and Sandkuhler, J. Neurogenic neuroinflammation: inflammatory CNS reactions in response to neuronal activity. Nat Rev Neurosci. (2014) 15:43–53. doi: 10.1038/nrn3617
38. DiSabato, DJ, Quan, N, and Godbout, JP. Neuroinflammation: the devil is in the details. J Neurochem. (2016) 139:136–53. doi: 10.1111/jnc.13607
39. Zhang, S, Azubuine, J, and Schmeer, C. A systematic literature review on the role of glial cells in the pathomechanisms of migraine. Front Mol Neurosci. (2023) 16:1219574. doi: 10.3389/fnmol.2023.1219574
40. He, W, Wang, Y, Zhang, Y, Zhang, Y, and Zhou, J. The status of knowledge on migraines: the role of microglia. J Neuroimmunol. (2023) 381:578118. doi: 10.1016/j.jneuroim.2023.578118
41. Vila-Pueyo, M, Gliga, O, Gallardo, VJ, and Pozo-Rosich, P. The role of glial cells in different phases of migraine: lessons from preclinical studies. Int J Mol Sci. (2023) 24:12553. doi: 10.3390/ijms241612553
42. Amani, H, Soltani Khaboushan, A, Terwindt, GM, and Tafakhori, A. Glia signaling and brain microenvironment in migraine. Mol Neurobiol. (2023) 60:3911–34. doi: 10.1007/s12035-023-03300-3
43. Carneiro-Nascimento, S, and Levy, D. Cortical spreading depression and meningeal nociception. Neurobiol Pain. (2022) 11:100091. doi: 10.1016/j.ynpai.2022.100091
44. van Hameren, G, Muradov, J, Minarik, A, Aboghazleh, R, Orr, S, Cort, S, et al. Mitochondrial dysfunction underlies impaired neurovascular coupling following traumatic brain injury. Neurobiol Dis. (2023) 186:106269. doi: 10.1016/j.nbd.2023.106269
45. Wang, Y, Wang, Y, Yue, G, and Zhao, Y. Energy metabolism disturbance in migraine: from a mitochondrial point of view. Front Physiol. (2023) 14:1133528. doi: 10.3389/fphys.2023.1133528
46. Borkum, JM . Brain energy deficit as a source of oxidative stress in migraine: a molecular basis for migraine susceptibility. Neurochem Res. (2021) 46:1913–32. doi: 10.1007/s11064-021-03335-9
47. Sun, M, Rong, J, Zhou, M, Liu, Y, Sun, S, Liu, L, et al. Astrocyte-microglia crosstalk: a novel target for the treatment of migraine. Aging Dis. (2023) 15:12. doi: 10.14336/AD.2023.0623
48. Yamanaka, G, Hayashi, K, Morishita, N, Takeshita, M, Ishii, C, Suzuki, S, et al. Experimental and clinical investigation of cytokines in migraine: a narrative review. Int J Mol Sci. (2023) 24:8343. doi: 10.3390/ijms24098343
49. Simonetto, M, Infante, M, Sacco, RL, Rundek, T, and Della-Morte, D. A novel anti-inflammatory role of Omega-3 PUFAs in prevention and treatment of atherosclerosis and vascular cognitive impairment and dementia. Nutrients. (2019) 11:2279. doi: 10.3390/nu11102279
50. Drenjancevic, I, and Pitha, J. Omega-3 polyunsaturated fatty acids-vascular and cardiac effects on the cellular and molecular level (narrative review). Int J Mol Sci. (2022) 23:2104. doi: 10.3390/ijms23042104
51. Sokoła-Wysoczańska, E, Wysoczański, T, Wagner, J, Czyż, K, Bodkowski, R, Lochyński, S, et al. Polyunsaturated fatty acids and their potential therapeutic role in cardiovascular system disorders-a review. Nutrients. (2018) 10:1561. doi: 10.3390/nu10101561
52. Ruiz-Lopez, N, Sayanova, O, Napier, JA, and Haslam, RP. Metabolic engineering of the omega-3 long chain polyunsaturated fatty acid biosynthetic pathway into transgenic plants. J Exp Bot. (2012) 63:2397–410. doi: 10.1093/jxb/err454
53. Djuricic, I, and Calder, PC. Beneficial outcomes of Omega-6 and Omega-3 polyunsaturated fatty acids on human health: an update for 2021. Nutrients. (2021) 13:2421. doi: 10.3390/nu13072421
54. Laye, S, Nadjar, A, Joffre, C, and Bazinet, RP. Anti-inflammatory effects of Omega-3 fatty acids in the brain: physiological mechanisms and relevance to pharmacology. Pharmacol Rev. (2018) 70:12–38. doi: 10.1124/pr.117.014092
55. Gabbs, M, Leng, S, Devassy, JG, Monirujjaman, M, and Aukema, HM. Advances in our understanding of Oxylipins derived from dietary PUFAs. Adv Nutr. (2015) 6:513–40. doi: 10.3945/an.114.007732
56. Dyall, SC . Long-chain omega-3 fatty acids and the brain: a review of the independent and shared effects of EPA, DPA and DHA. Front Aging Neurosci. (2015) 7:52. doi: 10.3389/fnagi.2015.00052
57. Mariamenatu, AH, and Abdu, EM. Overconsumption of Omega-6 polyunsaturated fatty acids (PUFAs) versus deficiency of Omega-3 PUFAs in modern-day diets: the disturbing factor for their "balanced antagonistic metabolic functions" in the human body. J Lipids. (2021) 2021:8848161. doi: 10.1155/2021/8848161
58. DiNicolantonio, JJ, and O'Keefe, JH. The importance of marine omega-3s for brain development and the prevention and treatment of behavior, mood, and other brain disorders. Nutrients. (2020) 12:2333. doi: 10.3390/nu12082333
59. Larrieu, T, and Laye, S. Food for mood: relevance of nutritional Omega-3 fatty acids for depression and anxiety. Front Physiol. (2018) 9:1047. doi: 10.3389/fphys.2018.01047
60. Dyall, SC, Balas, L, Bazan, NG, Brenna, JT, Chiang, N, da Costa Souza, F, et al. Polyunsaturated fatty acids and fatty acid-derived lipid mediators: recent advances in the understanding of their biosynthesis, structures, and functions. Prog Lipid Res. (2022) 86:101165. doi: 10.1016/j.plipres.2022.101165
61. Chávez-Castillo, M, Ortega, A, Cudris-Torres, L, Duran, P, Rojas, M, Manzano, A, et al. Specialized pro-resolving lipid mediators: the future of chronic pain therapy? Int J Mol Sci. (2021) 22:10370. doi: 10.3390/ijms221910370
62. Chiang, N, and Serhan, CN. Specialized pro-resolving mediator network: an update on production and actions. Essays Biochem. (2020) 64:443–62. doi: 10.1042/EBC20200018
63. Calder, PC . Mechanisms of action of (n-3) fatty acids. J Nutr. (2012) 142:592S–9S. doi: 10.3945/jn.111.155259
64. Rey, C, Nadjar, A, Joffre, F, Amadieu, C, Aubert, A, Vaysse, C, et al. Maternal n-3 polyunsaturated fatty acid dietary supply modulates microglia lipid content in the offspring. Prostaglandins Leukot Essent Fatty Acids. (2018) 133:1–7. doi: 10.1016/j.plefa.2018.04.003
65. Sullivan, EM, Pennington, ER, Green, WD, Beck, MA, Brown, DA, and Shaikh, SR. Mechanisms by which dietary fatty acids regulate mitochondrial structure-function in health and disease. Adv Nutr. (2018) 9:247–62. doi: 10.1093/advances/nmy007
66. de Oliveira, MR, Nabavi, SF, Nabavi, SM, and Jardim, FR. Omega-3 polyunsaturated fatty acids and mitochondria, back to the future. Trends Food Sci Technol. (2017) 67:76–92. doi: 10.1016/j.tifs.2017.06.019
67. Li, G, Li, Y, Xiao, B, Cui, D, Lin, Y, Zeng, J, et al. Antioxidant activity of docosahexaenoic acid (DHA) and its regulatory roles in mitochondria. J Agric Food Chem. (2021) 69:1647–55. doi: 10.1021/acs.jafc.0c07751
68. Golpour, P, Nourbakhsh, M, Mazaherioun, M, Janani, L, Nourbakhsh, M, and Yaghmaei, P. Improvement of NRF2 gene expression and antioxidant status in patients with type 2 diabetes mellitus after supplementation with omega-3 polyunsaturated fatty acids: a double-blind randomised placebo-controlled clinical trial. Diabetes Res Clin Pract. (2020) 162:108120. doi: 10.1016/j.diabres.2020.108120
69. Heshmati, J, Morvaridzadeh, M, Maroufizadeh, S, Akbari, A, Yavari, M, Amirinejad, A, et al. Omega-3 fatty acids supplementation and oxidative stress parameters: a systematic review and meta-analysis of clinical trials. Pharmacol Res. (2019) 149:104462. doi: 10.1016/j.phrs.2019.104462
70. Zhou, L, Xiong, JY, Chai, YQ, Huang, L, Tang, ZY, Zhang, XF, et al. Possible antidepressant mechanisms of omega-3 polyunsaturated fatty acids acting on the central nervous system. Front Psych. (2022) 13:933704. doi: 10.3389/fpsyt.2022.933704
71. Malhi, GS, and Mann, JJ. Depression. Lancet. (2018) 392:2299–312. doi: 10.1016/S0140-6736(18)31948-2
72. Hassamal, S . Chronic stress, neuroinflammation, and depression: an overview of pathophysiological mechanisms and emerging anti-inflammatories. Front Psych. (2023) 14:1130989. doi: 10.3389/fpsyt.2023.1130989
73. Michopoulos, V, Powers, A, Gillespie, CF, Ressler, KJ, and Jovanovic, T. Inflammation in fear- and anxiety-based disorders: PTSD, GAD, and beyond. Neuropsychopharmacology. (2017) 42:254–70. doi: 10.1038/npp.2016.146
74. Hu, P, Lu, Y, Pan, BX, and Zhang, WH. New insights into the pivotal role of the amygdala in inflammation-related depression and anxiety disorder. Int J Mol Sci. (2022) 23:11076. doi: 10.3390/ijms231911076
75. Minen, MT, Begasse de Dhaem, O, Kroon van Diest, A, Powers, S, Schwedt, TJ, Lipton, R, et al. Migraine and its psychiatric comorbidities. J Neurol Neurosurg Psychiatry. (2016) 87:741–9. doi: 10.1136/jnnp-2015-312233
76. On behalf of School of Advanced Studies of European Headache Federation (EHF-SAS)Torres-Ferrús, M, Ursitti, F, Alpuente, A, Brunello, F, Chiappino, D, et al. From transformation to chronification of migraine: pathophysiological and clinical aspects. J Headache Pain. (2020) 21:42. doi: 10.1186/s10194-020-01111-8
77. Meccariello, R . Molecular advances on cannabinoid and endocannabinoid research. Int J Mol Sci. (2023) 24:12760. doi: 10.3390/ijms241612760
78. Devane, WA, Hanuš, L, Breuer, A, Pertwee, RG, Stevenson, LA, Griffin, G, et al. Isolation and structure of a brain constituent that binds to the cannabinoid receptor. Science. (1992) 258:1946–9. doi: 10.1126/science.1470919
79. Watson, JE, Kim, JS, and Das, A. Emerging class of omega-3 fatty acid endocannabinoids & their derivatives. Prostaglandins Other Lipid Mediat. (2019) 143:106337. doi: 10.1016/j.prostaglandins.2019.106337
80. Maghsoumi-Norouzabad, L, Mansoori, A, Abed, R, and Shishehbor, F. Effects of omega-3 fatty acids on the frequency, severity, and duration of migraine attacks: a systematic review and meta-analysis of randomized controlled trials. Nutr Neurosci. (2018) 21:614–23. doi: 10.1080/1028415X.2017.1344371
81. Ramsden, CE, Zamora, D, Faurot, KR, MacIntosh, B, Horowitz, M, Keyes, GS, et al. Dietary alteration of n-3 and n-6 fatty acids for headache reduction in adults with migraine: randomized controlled trial. BMJ. (2021) 374:n1448. doi: 10.1136/bmj.n1448
82. Luo, XD, Feng, JS, Yang, Z, Huang, QT, Lin, JD, Yang, B, et al. High-dose omega-3 polyunsaturated fatty acid supplementation might be more superior than low-dose for major depressive disorder in early therapy period: a network meta-analysis. BMC Psychiatry. (2020) 20:248. doi: 10.1186/s12888-020-02656-3
83. Saini, RK, Prasad, P, Sreedhar, RV, Akhilender Naidu, K, Shang, X, and Keum, YS. Omega-3 polyunsaturated fatty acids (PUFAs): emerging plant and microbial sources, oxidative stability, bioavailability, and health benefits-a review. Antioxidants (Basel). (2021) 10:1627. doi: 10.3390/antiox10101627
84. Chang, JP, Tseng, PT, Zeng, BS, Chang, CH, Su, H, Chou, PH, et al. Safety of supplementation of Omega-3 polyunsaturated fatty acids: a systematic review and Meta-analysis of randomized controlled trials. Adv Nutr. (2023) 14:1326–36. doi: 10.1016/j.advnut.2023.08.003
85. Tseng, PT, Zeng, BY, Chen, JJ, Kuo, CH, Zeng, BS, Kuo, JS, et al. High dosage Omega-3 fatty acids outperform existing pharmacological options for migraine prophylaxis: a network Meta-analysis. Adv Nutr. (2024) 15:100163. doi: 10.1016/j.advnut.2023.100163
86. Djalali, M, Talebi, S, Djalali, E, Abdolahi, M, Travica, N, and Djalali, M. The effect of omega-3 fatty acids supplementation on inflammatory biomarkers in subjects with migraine: a randomized, double-blind, placebo-controlled trial. Immunopharmacol Immunotoxicol. (2023) 45:565–70. doi: 10.1080/08923973.2023.2196600
87. Rist, PM, Buring, JE, Cook, NR, Manson, JE, and Kurth, T. Effect of vitamin D and/or marine n-3 fatty acid supplementation on changes in migraine frequency and severity. Am J Med. (2021) 134:e5:756–762.e5. doi: 10.1016/j.amjmed.2020.11.023
88. Honarvar, NM, Soveid, N, Abdolahi, M, Djalali, M, Hatami, M, and Karzar, NH. Anti-Neuroinflammatory properties of n-3 fatty acids and Nano- curcumin on migraine patients from cellular to clinical insight: a randomized, double-blind and placebo-controlled trial. Endocr Metab Immune Disord Drug Targets. (2021) 21:365–73. doi: 10.2174/1871530320666200729144430
89. Soares, AA, Loucana, PMC, Nasi, EP, Sousa, KMH, Sa, OMS, and Silva-Neto, RP. A double- blind, randomized, and placebo-controlled clinical trial with omega-3 polyunsaturated fatty acids (OPFA ɷ-3) for the prevention of migraine in chronic migraine patients using amitriptyline. Nutr Neurosci. (2018) 21:219–23. doi: 10.1080/1028415X.2016.1266133
90. Abdolahi, M, Sarraf, P, Javanbakht, MH, Honarvar, NM, Hatami, M, Soveyd, N, et al. A novel combination of omega-3 fatty acids and Nano-curcumin modulates Interleukin-6 gene expression and high sensitivity C-reactive protein serum levels in patients with migraine: a randomized clinical trial study. CNS Neurol Disord Drug Targets. (2018) 17:430–8. doi: 10.2174/1871527317666180625101643
91. Harel, Z, Gascon, G, Riggs, S, Vaz, R, Brown, W, and Exil, G. Supplementation with omega-3 polyunsaturated fatty acids in the management of recurrent migraines in adolescents. J Adolesc Health. (2002) 31:154–61. doi: 10.1016/S1054-139X(02)00349-X
92. Pradalier, A, Bakouche, P, Baudesson, G, Delage, A, Cornaille-Lafage, G, Launay, JM, et al. Failure of omega-3 polyunsaturated fatty acids in prevention of migraine: a double-blind study versus placebo. Cephalalgia. (2001) 21:818–22. doi: 10.1046/j.1468-2982.2001.218240.x
93. Wang, HF, Liu, WC, Zailani, H, Yang, CC, Chen, TB, Chang, CM, et al. A 12-week randomized double-blind clinical trial of eicosapentaenoic acid intervention in episodic migraine. Brain Behav Immun. (2024) 118:459–67. doi: 10.1016/j.bbi.2024.03.019
94. Islam, F, Imran, A, Nosheen, F, Fatima, M, Arshad, MU, Afzaal, M, et al. Functional roles and novel tools for improving-oxidative stability of polyunsaturated fatty acids: a comprehensive review. Food Sci Nutr. (2023) 11:2471–82. doi: 10.1002/fsn3.3272
95. Mane, S, Kumari, P, Singh, A, Taneja, NK, and Chopra, R. Amelioration for oxidative stability and bioavailability of N-3 PUFA enriched microalgae oil: an overview. Crit Rev Food Sci Nutr. (2022) 64:2579–600. doi: 10.1080/10408398.2022.2124505
96. Venugopalan, VK, Gopakumar, LR, Kumaran, AK, Chatterjee, NS, Soman, V, Peeralil, S, et al. Encapsulation and protection of Omega-3-rich fish oils using food-grade delivery systems. Food Secur. (2021) 10:1566. doi: 10.3390/foods10071566
97. Zhang, Y, Guo, X, Gao, J, Wei, C, Zhao, S, Liu, Z, et al. The associations of circulating common and uncommon polyunsaturated fatty acids and modification effects on dietary quality with all-cause and disease-specific mortality in NHANES 2003-2004 and 2011-2012. Ann Med. (2021) 53:1744–57. doi: 10.1080/07853890.2021.1937693
Keywords: eicosapentaenoic acid (EPA), docosahexaenoic acid (DHA), omega-3 fatty acids, neuroinflammation, neurogenic inflammation EPA
Citation: Chen T-B, Yang C-C, Tsai I-J, Yang H-W, Hsu Y-C, Chang C-M and Yang C-P (2024) Neuroimmunological effects of omega-3 fatty acids on migraine: a review. Front. Neurol. 15:1366372. doi: 10.3389/fneur.2024.1366372
Received: 08 January 2024; Accepted: 12 April 2024;
Published: 06 May 2024.
Edited by:
Levent Ertugrul Inan, Health Science University Ankara SUAM, TürkiyeReviewed by:
Francesco De Logu, University of Florence, ItalyCopyright © 2024 Chen, Yang, Tsai, Yang, Hsu, Chang and Yang. This is an open-access article distributed under the terms of the Creative Commons Attribution License (CC BY). The use, distribution or reproduction in other forums is permitted, provided the original author(s) and the copyright owner(s) are credited and that the original publication in this journal is cited, in accordance with accepted academic practice. No use, distribution or reproduction is permitted which does not comply with these terms.
*Correspondence: Chun-Pai Yang, bmV1cmFseXVuZ0BnbWFpbC5jb20=; Ching-Mao Chang, bWFnaWNianBAZ21haWwuY29t; Yung-Chu Hsu, MDYwMTVAY3ljaC5vcmcudHc=
Disclaimer: All claims expressed in this article are solely those of the authors and do not necessarily represent those of their affiliated organizations, or those of the publisher, the editors and the reviewers. Any product that may be evaluated in this article or claim that may be made by its manufacturer is not guaranteed or endorsed by the publisher.
Research integrity at Frontiers
Learn more about the work of our research integrity team to safeguard the quality of each article we publish.