- 1Department of Radiology, Affiliated Hospital 6 of Nantong University, Yancheng Third People’s Hospital, Yancheng, China
- 2Department of Orthopedics, Affiliated Hospital 6 of Nantong University, Yancheng Third People’s Hospital, Yancheng, China
- 3Department of Central Laboratory, Affiliated Hospital 6 of Nantong University, Yancheng Third People’s Hospital, Yancheng, China
Background: Neuroimaging studies have suggested a pivotal role for the amygdala involvement in chronic low back pain (CLBP). However, the relationship between the amygdala subregions and CLBP has not yet been delineated. This study aimed to analyze whether the amygdala subregions were linked to the development of CLBP.
Methods: A total of 45 patients with CLBP and 45 healthy controls (HCs) were included in this study. All subjects were asked to complete a three-dimensional T1-weighted magnetic resonance imaging (3D-T1 MRI) scan. FreeSurfer 7.3.2 was applied to preprocess the structural MRI images and segment the amygdala into nine subregions. Afterwards, comparisons were made between the two groups in terms of the volumes of the amygdala subregions. Correlation analysis is utilized to examine the relationship between the amygdala subregion and the scale scores, as well as the pain duration in patients with CLBP. Additionally, logistic regression was used to explore the risk of the amygdala and its subregions for CLBP.
Results: In comparison to HCs, patients with CLBP exhibited a significant enlargement of the left central nucleus (Ce) and left cortical nucleus (Co). Furthermore, the increased volume of the left Ce was associated with a higher risk of CLBP.
Conclusion: Our study suggests that the left Ce and left Co may be involved in the pathophysiological processes of CLBP. Moreover, the volume of the left Ce may be a biomarker for detecting the risk of CLBP.
1 Introduction
Chronic low back pain (CLBP) is the leading cause of disability globally (1), affecting approximately 20% of the global population (2). Despite such high prevalence and social burden, the pathophysiology of CLBP remains obscure (3). Studies in neuroimaging have revealed that individuals suffering from CLBP have structural changes in their brains that are not present in healthy people (4–10). For example, alterations in gray matter volume were found in several brain regions such as the posterior parietal cortex, anterolateral prefrontal cortex, left precuneus, bilateral putamen, and temporal lobe, etc. (4–7). Meanwhile, several brain regions showed changes in cortical thickness, like the paracentral lobule, right rostral middle frontal gyrus, and left medial temporal lobe, etc. (8–10). These findings indicate that the pathogenesis of CLBP is related to structural alterations in the brain.
The amygdala, a limbic brain region, is essential in managing pain and affects the emotional and cognitive responses to it (11, 12). Structural neuroimaging studies have demonstrated alterations in the volume of the amygdala in patients with CLBP, and these changes may be linked to their impaired cognitive-affective and emotional processing abilities, as well as to a more persistent chronic pain condition (6, 13, 14). However, these investigations have focused only on the total amygdala, not reflecting the subtle changes in the subregions of the amygdala.
In recent years, an atlas of the amygdala at the sub-regional level, which was created by combining ultra-high resolution ex vivo MRI with in vivo data, is available in FreeSurfer 6 and later versions1 (15). This statistical atlas divides the amygdala into nine subregions, including the anterior amygdaloid area (AAA), cortico-amygdaloid transition area (CAT), basal nucleus (Ba), lateral nucleus (La), paralaminar nucleus (PL), accessory basal nucleus (AB), medial nucleus (Me), central nucleus (Ce), and cortical nucleus (Co). AAA is populated by magnocellular cholinergic neurons that secrete acetylcholine, and it plays a role in preserving attention and memory (16, 17). CAT is likely to play an important role in social communication and may be linked to the assessment of negative emotions (18–20). PL is thought to be part of the Ba (21, 22), which is linked to emotion, cognition, and has the ability to regulate voluntary motor movements (23). La is the primary input structure to the amygdala, and it is believed to be a major factor in emotional processing and responses, as well as in stress-inducing stimuli (24, 25). AB is linked internally within the basal nucleus and receives input from the cortex and other subcortical regions (26). Me is considered to be the core of the neuroendocrine system that processes social data and is strongly associated with the control of defensive behavior (27, 28). Ce, the nociceptive center in the brain, is critical for pain regulation and processing and is the main output nucleus of the amygdala that drives pain-related functions (29, 30). Co is mainly responsible for olfactory processing, and its more posterior section is linked to memory processes (31–33). By utilizing FreeSurfer, we can accurately assess minor alterations in the amygdala subregions.
To date, no studies have been conducted to determine if there are any volumetric changes in the subregions of the amygdala in those with CLBP. Therefore, in this study, we hypothesized that the volumes of the amygdala subregions changed in patients with CLBP and that these alterations may be involved in the development of CLBP.
2 Methods
2.1 Participants
From the period of January 2021 to August 2022, a total of 45 patients with CLBP were recruited from the Sixth Affiliated Hospital of Nantong University (Yancheng Third People’s Hospital). Healthy controls (HCs) were enrolled from the Yancheng area through advertisements. The Yancheng Third People’s Hospital Ethics Committee approved this study, and all participants gave written informed consent.
The inclusion criteria for patients with CLBP were pain persisting or fluctuating for more than 3 months (34), Visual Analogue Scale (VAS) scores of three points or higher, no history of neuropsychiatric disorders, major systemic diseases, head injuries or comas, other chronic pain disorders, and right-handedness. All of the patients took analgesic medication on a regular basis, and Table 1 listed the types of analgesics they used. Meanwhile, HCs with no current experience of pain or past history of chronic pain, nervous system diseases, diabetes, hypertension, hyperlipidemia, mental illnesses, long-term smoking, or alcoholism were included. If patients with CLBP or HCs could not complete MRI scans, had organic brain lesions, or had contraindications to MRI, they would be excluded.
2.2 Clinical assessment
VAS and the Oswestry Disability Index (ODI) were employed to measure the severity of pain and dysfunction experienced by patients with CLBP before MRI scans. Utilizing VAS, patients were asked to make a mark on a 10 cm line based on their perception of the pain intensity (35). The further along the line they marked, the more intense the pain. Meanwhile, ODI, comprising pain intensity, personal care, lifting, walking, sitting, standing, sleeping, sex life, social life, and traveling, was used to measure the extent of dysfunction in patients, with scores that increased as dysfunction became more severe (36).
2.3 MRI data acquisition
All participants underwent three-dimensional T1-weighted (3D-T1) structural imaging at a 3.0 Tesla scanner (Discovery 750w, GE, United States) at the Sixth Affiliated Hospital of Nantong University. Parameters of the sequence were as follows: Repetition time (TR) = 7.5 ms, Echo time (TE) = 2.8 ms, Field of View (FOV) = 24 cm × 24 cm, Slice thickness = 1.0 mm, Number of slices = 152, Flip Angle = 15°, Voxel size = 0.5 × 0.5 × 1 mm.
2.4 Amygdala segmentation
A standard FreeSurfer recon-all pipeline was employed for pre-processing T1-weighted MRI (version 7.3.2) (see text footnote 1). Then, automated amygdala segmentation and volume calculation was achieved through the use of the FreeSurfer 7.3.2 automated pipeline for subcortical structure segmentation (15). After segmentation was done, one researcher visually checked the segmentation results to ensure they were accurate.
2.5 Statistical analysis
Statistical analysis was carried out using Statistical Package for the Social Sciences version 27 (SPSS 27). Initially, we utilized the Shapiro–Wilk test to assess the normal distribution of age, clinical scale scores, pain duration, and intracranial volume (ICV) in patients with CLBP and HCs. If the normal distribution was met, we then used the Student’s t-test to evaluate the difference between the two groups; otherwise, the Mann–Whitney U test was applied. Meanwhile, the Chi-square test was used to compare the gender differences between the two groups. The significance level of the results was set at p < 0.05.
Cook’s Distance was utilized to assess possible outliers within the volume of the amygdala and its subregions. After that, the Shapiro–Wilk test was employed to determine if the volume of the amygdala and its subregions in patients with CLBP and HCs followed a normal distribution. The Student’s t-test or Mann–Whitney U test was then utilized to determine any differences between the two groups, and p < 0.05 was considered significant. Subsequently, Pearson or Spearman correlation analyses were used to evaluate the association of the volumes of the amygdala and its subregions with the clinical scale scores, as well as the pain duration in patients with CLBP.
Finally, logistic regression was employed to investigate the correlations between the amygdala and its subregions and the risk of CLBP. Odds ratios (ORs) and 95% confidence intervals (CIs) were evaluated using univariate and multivariate models. The logistic regression analysis involved the implementation of four different schemes: (1) univariate model; (2) model 1, adjusted for age, gender, ICV, and the left Ce; (3) model 2, adjusted for age, gender, ICV, and the left Co; (4) model 3, adjusted for age, gender, ICV, the left Ce and left Co. There was no relevant multicollinearity among these six predictors (all VIF < 5.000).
3 Results
3.1 Demographics and clinical characteristics
In the current cross-sectional study, a total of 45 patients with CLBP and 45 HCs were included. Demographics and clinical characteristics were presented in Table 2. There was no noteworthy difference between gender, age, and ICV between the two groups.
3.2 Amygdala subregion analysis
The amygdala subregions were shown in Figure 1, and the volume comparison between patients with CLBP and HCs was outlined in Table 3. In comparison to HCs, patients with CLBP displayed a significant increase in the volume of the left Ce and left Co. However, there was no significant difference in the volumes of the whole amygdala, bilateral amygdala, or other amygdala subregions.
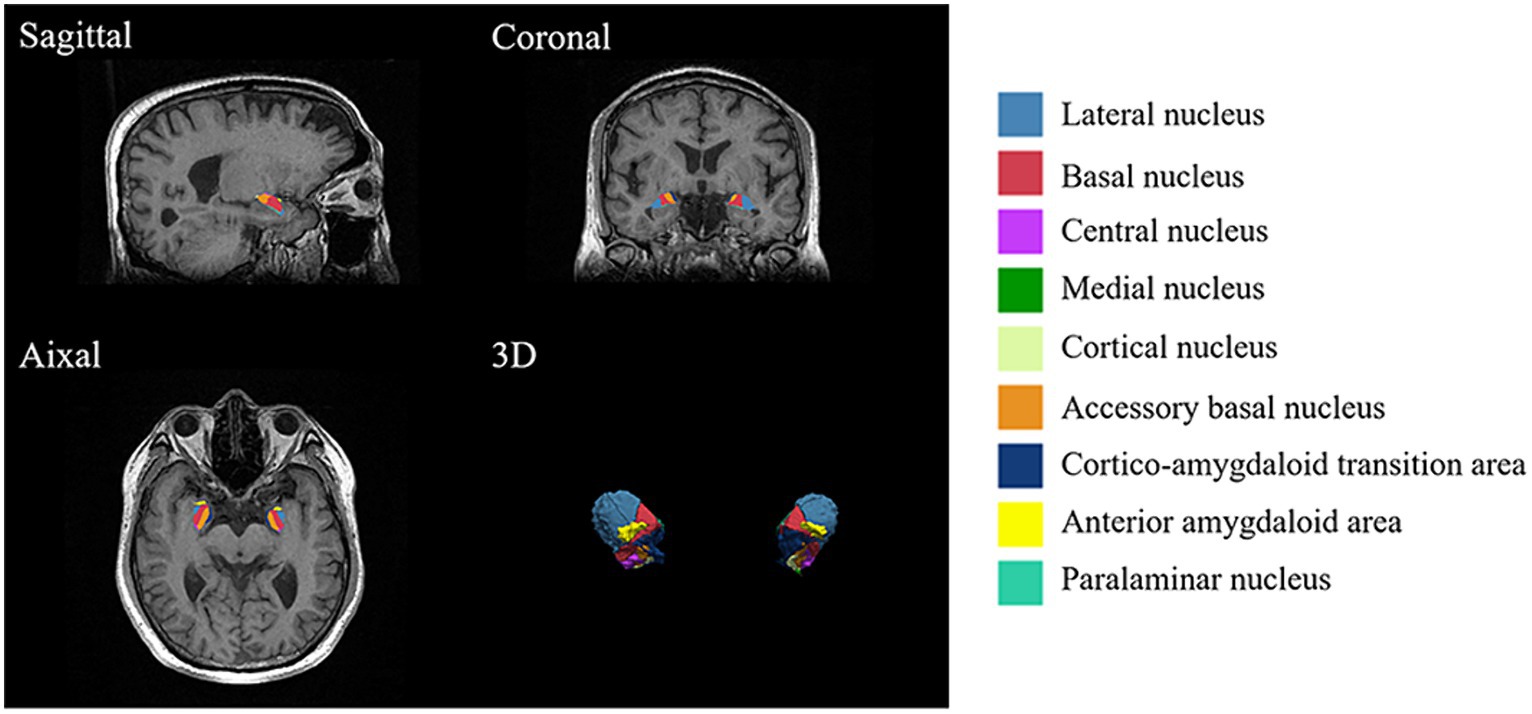
Figure 1. Amygdala subregions. Utilizing FreeSurfer 7.3.2, the amygdala was divided into nine subregions, including the anterior amygdaloid area (AAA), cortico-amygdaloid transition area (CAT), basal nucleus (Ba), lateral nucleus (La), paralaminar nucleus (PL), accessory basal nucleus (AB), medial nucleus (Me), central nucleus (Ce), and cortical nucleus (Co).
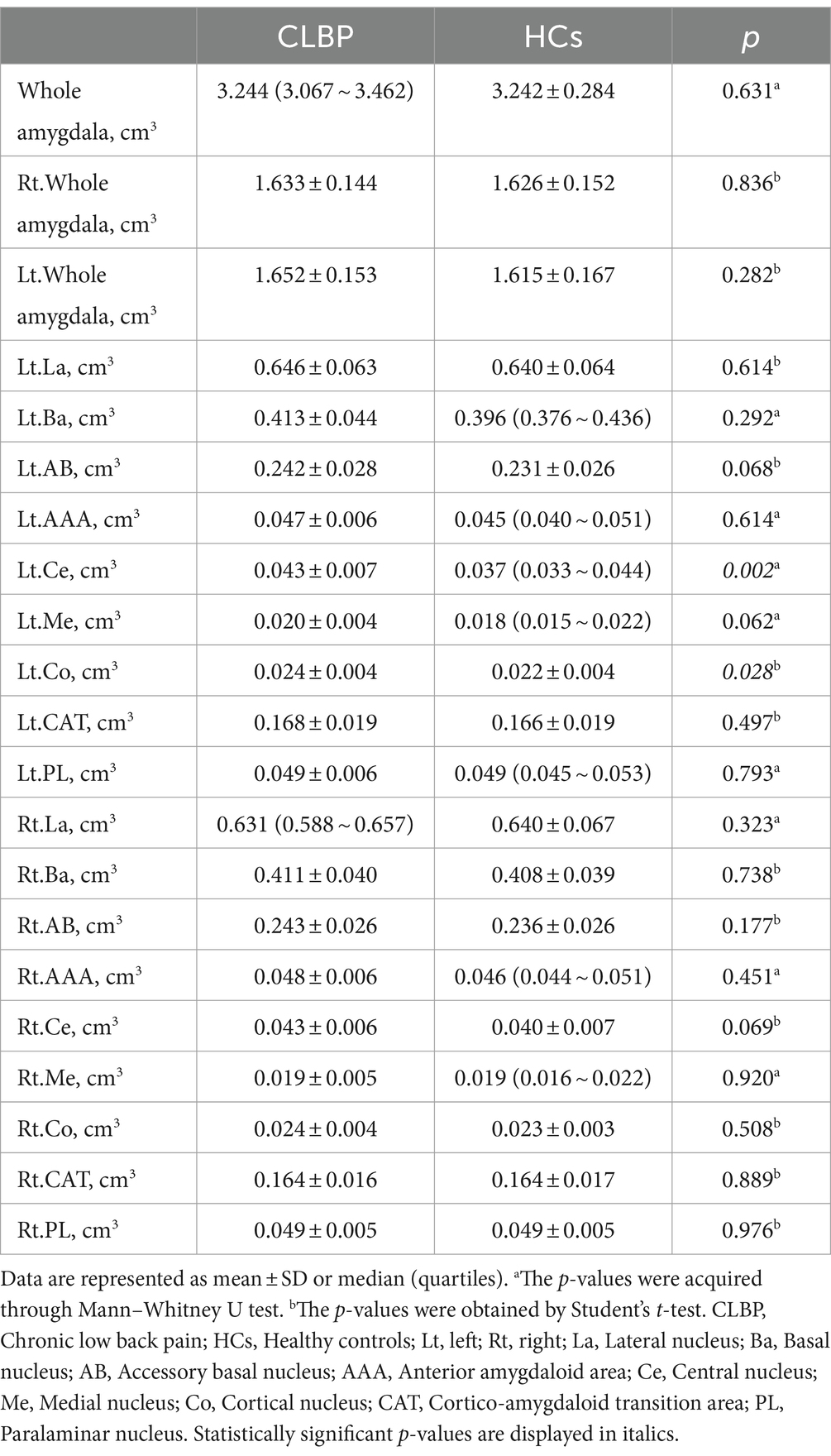
Table 3. Differences between the whole and individual amygdala volumes of the patients with CLBP and HCs.
3.3 Correlation analysis of amygdala subregions with clinical characteristics
Our research did not reveal any significant correlation between the volumes of the left Ce and left Co in patients with CLBP and their clinical scale scores and pain duration (Table 4).
3.4 OR risk of amygdala subregions for patients with CLBP
The multivariate logistic regression was employed to assess whether alterations in the volumes of the amygdala subregions were correlated with a higher risk of CLBP. We adjusted for age, gender, ICV, left Ce volume, and left Co volume in the final model (Tables 5, 6). The subjects with an increased volume of the left Ce (OR = 1.095; 95%CI, 1.015–1.181; p = 0.02) had a higher risk of being CLBP compared to those with a normal volume of the left Ce.
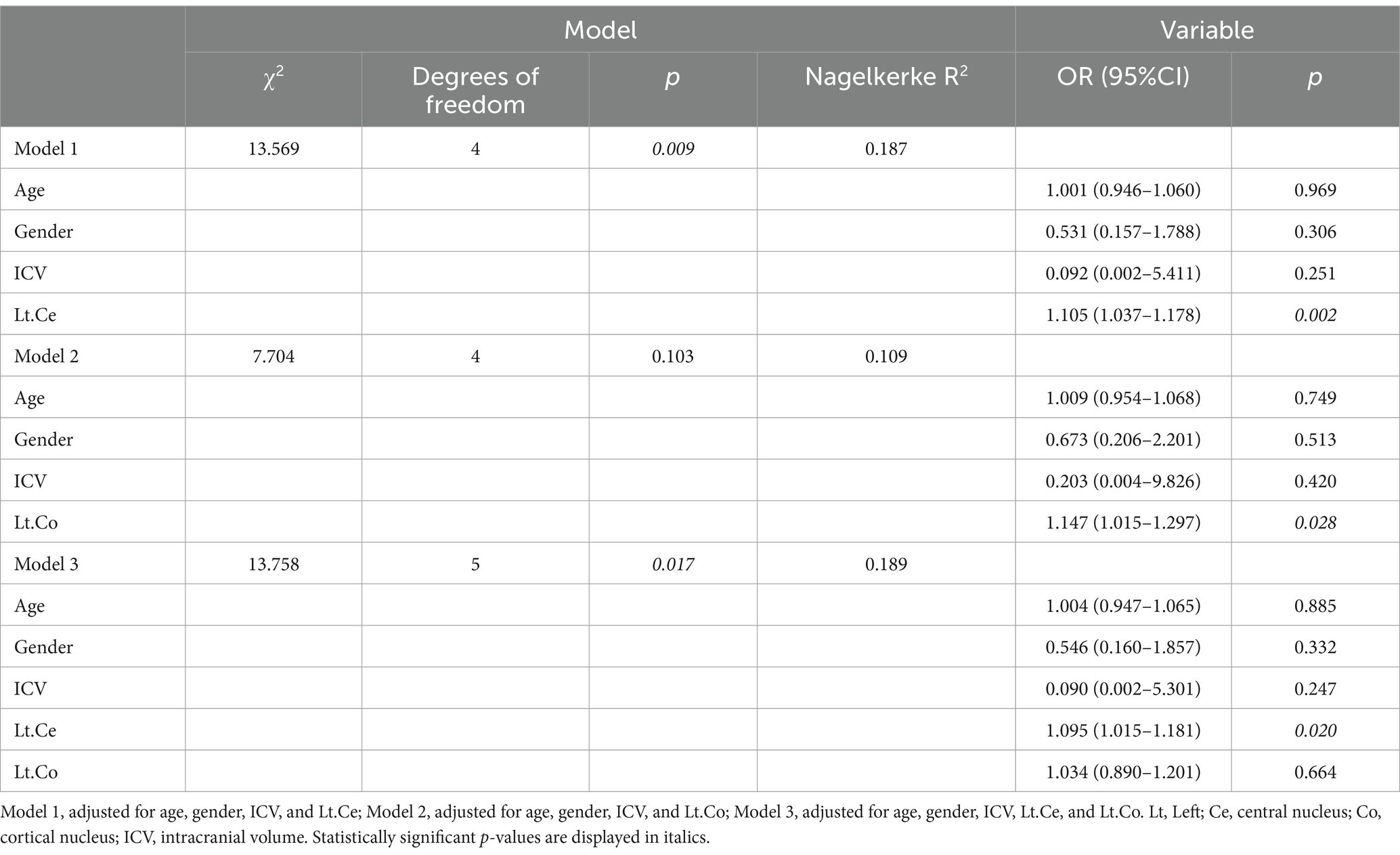
Table 5. Multivariate logistic regression analysis of the association between amygdala subregions and CLBP.
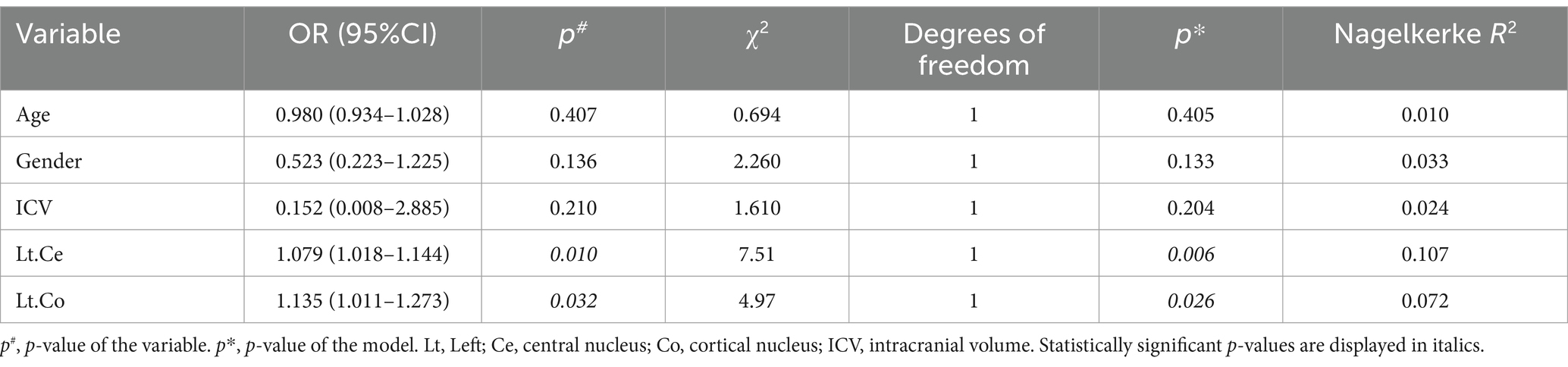
Table 6. Univariate logistic regression analysis of the association between amygdala subregions and CLBP.
4 Discussion
As far as we know, this is the first study to analyze structural alterations of the subregions of the amygdala in patients with CLBP. In comparison to the HCs, patients with CLBP had an increase in volume in the left Ce and left Co. Moreover, an increased volume of the left Ce predisposed individuals to a higher risk of CLBP.
In this study, patients with CLBP were found to have an increased volume of the left Ce. Moreover, the increase in the left Ce raises the risk of being CLBP. The Ce of the amygdala (CeA), a nociceptive center in the brain, receives nociceptive information from two main pathways, the first of which originates in the basolateral amygdala (BLA) (30, 37). The central nervous system transmits noxious information resulting from past painful experiences and mood states from the BLA to the CeA (BLA-CeA pathway) (38). By activating the BLA-CeA pathway, reward-related behavior is enabled, thus resulting in pain inhibition (39). Meanwhile, an overstimulation of glutamate (Glu) neurons in the BLA due to noxious stimuli can lead to an excitation of Glu neurons in the ventral hippocampus (vHIP), thus activating GABA neurons in the CeA via the vHIP-CeA pathway (38, 40), which may be a significant factor in Chronic pain. Meanwhile, the second pathway transmits more direct and raw nociceptive information to the CeA via the laterocapsular region of the CeA (CeLC) (41). The CeLC receives pain signals transmitted via the spino-parabrachio-amygdaloid pathway, and may also via direct projections from the spinal cord (42–44). It is noteworthy that the parabrachial input has peptidergic features and is the exclusive source of calcitonin gene-related peptide (CGRP) in the amygdala. Moreover, the CeA is the main output nucleus of the amygdala. GABAergic projection neurons are present in the CeLC, which also contain peptide substances like corticotropin-releasing factor (CRF) (41). Neurons in the CeA, which contain CRF, are innervated by terminals that contain CGRP from the parabrachial region (45–47) and project to distinct regions in the basal forebrain and brainstem (48). It is thought that an abnormal activation of these neurons in the CeA is a main contributor to chronic pain (49). The involvement of the Ce in the pathophysiological mechanism of CLBP is evident from these findings.
Interestingly, our study also observed increased volume of the left Co in patients with CLBP. However, the relationship between Co. and pain is not well understood at present. Co, located in the superficial region of the amygdala, is directly connected to the olfactory system and is involved in the processing of olfactory stimuli (50, 51). Nociception and olfaction are thought to intersect at the sensory, behavioral and emotional levels, as well as the molecular level (52). This is evidenced by the co-expression of pain genes in olfactory brain structures, the interaction of ion channels or G protein-coupled receptors in the transmission and processing of pain and olfaction, and the shared brain regions involved in central processing of nociceptive and olfactory information (52). Meanwhile, previous research has stressed the effect of odors on the qualitative evaluation of pain (53–56). Therefore, the abnormal volume changes in the left Co may impact CLBP by influencing olfaction. Thus, it is essential to examine the changes in olfaction in patients with CLBP and their association with CLBP in future studies.
It should be noted that Ce and Co were both situated in the left amygdala. Unlike the right amygdala, which is thought to be linked to pain promotion, the left amygdala does not usually have a pain-promoting capacity, and may even be associated with pain suppression (57). In the future, it is essential to investigate whether the lateralization of the subregions of the amygdala in this study promotes or inhibits the production of CLBP.
With the exception of the left Ce and left Co, we did not detect any significant variations in the volumes of other amygdala subregions between the groups. Our research also did not demonstrate a relationship between the volume of the left Ce and left Co and clinical scale scores and pain duration. This suggested that these two amygdala subregions could be a phenotypic biomarker of CLBP and may play a role in the pathophysiology of CLBP. However, it also raises the possibility that the abnormal volume changes in these amygdala subregions could be attributed to other factors, such as analgesic medication. The Ce is thought to be involved in tolerance to the antinociceptive effect of NSAIDs (58). Meanwhile, it is believed that Co plays a part in post-stress analgesia (59). Consequently, more research is needed in the future to rule out the influence of analgesics on the volume of the amygdala subregions.
This research has several limitations. To begin with, since this was a cross-sectional study, it is not possible to establish a causal relationship between changes in the amygdala subregions and CLBP. Therefore, further longitudinal research is necessary. Additionally, this did not allow us to examine the effect of medication on the changes of the amygdala and its subregions in CLBP. Finally, this study only concentrated on the structural changes of the amygdala subregions in patients with CLBP, not on the functional changes. Consequently, it is essential to investigate the functional changes of the amygdala subregions in patients with CLBP in the future.
5 Conclusion
In conclusion, our research has, for the first time, demonstrated that altered volumes of the left Ce and left Co in the amygdala subregions may be involved in the pathogenesis of CLBP. Furthermore, the volume of the left Ce may serve as a tool for identifying patients with CLBP and to at risk to develop CLBP.
Data availability statement
The original contributions presented in the study are included in the article/supplementary material, further inquiries can be directed to the corresponding authors.
Ethics statement
The studies involving humans were approved by the Yancheng Third People’s Hospital Ethics Committee. The studies were conducted in accordance with the local legislation and institutional requirements. The participants provided their written informed consent to participate in this study. Written informed consent was obtained from the individual(s) for the publication of any potentially identifiable images or data included in this article.
Author contributions
S-YG: Data curation, Writing – original draft. F-CS: Data curation, Writing – original draft. SW: Data curation, Writing – original draft. C-YW: Data curation, Writing – review & editing. X-XY: Data curation, Writing – review & editing. Y-FS: Data curation, Writing – review & editing. J-BH: Data curation, Writing – review & editing. FC: Conceptualization, Methodology, Supervision, Writing – review & editing. P-LP: Conceptualization, Methodology, Supervision, Writing – review & editing. W-HL: Conceptualization, Methodology, Supervision, Writing – review & editing.
Funding
The author(s) declare that financial support was received for the research, authorship, and/or publication of this article. This work was supported by the Special Funds for Science Development of the Clinical Teaching Hospitals of Jiangsu Vocational College of Medicine (20219112 and 20219125) and the Yancheng Commission of Health (20219116).
Acknowledgments
We would like to thank all the individuals who took part in the study.
Conflict of interest
The authors declare that the research was conducted in the absence of any commercial or financial relationships that could be construed as a potential conflict of interest.
Publisher’s note
All claims expressed in this article are solely those of the authors and do not necessarily represent those of their affiliated organizations, or those of the publisher, the editors and the reviewers. Any product that may be evaluated in this article, or claim that may be made by its manufacturer, is not guaranteed or endorsed by the publisher.
Footnotes
References
1. Hartvigsen, J, Hancock, MJ, Kongsted, A, Louw, Q, Ferreira, ML, Genevay, S, et al. What low back pain is and why we need to pay attention. Lancet. (2018) 391:2356–67. doi: 10.1016/S0140-6736(18)30480-X
2. Hoy, D, Bain, C, Williams, G, March, L, Brooks, P, Blyth, F, et al. A systematic review of the global prevalence of low back pain. Arthritis Rheum. (2012) 64:2028–37. doi: 10.1002/art.34347
3. Yu, S, Li, W, Shen, W, Edwards, RR, Gollub, RL, Wilson, G, et al. Impaired mesocorticolimbic connectivity underlies increased pain sensitivity in chronic low back pain. Neuroimage. (2020) 218:116969. doi: 10.1016/j.neuroimage.2020.116969
4. Buckalew, N, Haut, MW, Morrow, L, and Weiner, D. Chronic pain is associated with brain volume loss in older adults: preliminary evidence. Pain Med. (2008) 9:240–8. doi: 10.1111/j.1526-4637.2008.00412.x
5. Luchtmann, M, Steinecke, Y, Baecke, S, Lützkendorf, R, Bernarding, J, Kohl, J, et al. Structural brain alterations in patients with lumbar disc herniation: a preliminary study. PLoS One. (2014) 9:e90816. doi: 10.1371/journal.pone.0090816
6. Mao, C, Wei, L, Zhang, Q, Liao, X, Yang, X, and Zhang, M. Differences in brain structure in patients with distinct sites of chronic pain: a voxel-based morphometric analysis. Neural Regen Res. (2013) 8:2981–90. doi: 10.3969/j.issn.1673-5374.2013.32.001
7. Ung, H, Brown, JE, Johnson, KA, Younger, J, Hush, J, and Mackey, S. Multivariate classification of structural MRI data detects chronic low back pain. Cereb Cortex. (2014) 24:1037–44. doi: 10.1093/cercor/bhs378
8. Dolman, AJ, Loggia, ML, Edwards, RR, Gollub, RL, Kong, J, Napadow, V, et al. Phenotype matters: the absence of a positive association between cortical thinning and chronic low back pain when controlling for salient clinical variables. Clin J Pain. (2014) 30:839–45. doi: 10.1097/AJP.0000000000000043
9. Kong, J, Spaeth, RB, Wey, HY, Cheetham, A, Cook, AH, Jensen, K, et al. S1 is associated with chronic low back pain: a functional and structural MRI study. Mol Pain. (2013) 9:43. doi: 10.1186/1744-8069-9-43
10. Seminowicz, DA, Wideman, TH, Naso, L, Hatami-Khoroushahi, Z, Fallatah, S, Ware, MA, et al. Effective treatment of chronic low back pain in humans reverses abnormal brain anatomy and function. J Neurosci. (2011) 31:7540–50. doi: 10.1523/JNEUROSCI.5280-10.2011
11. Hsiao, IH, and Lin, YW. Electroacupuncture reduces fibromyalgia pain by attenuating the HMGB1, S100B, and TRPV1 signalling pathways in the mouse brain. Evid Based Complement Alternat Med. (2022) 2022:2242074. doi: 10.1155/2022/2242074
12. Yang, LN, Chen, K, Yin, XP, Liu, D, and Zhu, LQ. The comprehensive neural mechanism of oxytocin in analgesia. Curr Neuropharmacol. (2022) 20:147–57. doi: 10.2174/1570159X19666210826142107
13. Mao, CP, and Yang, HJ. Smaller amygdala volumes in patients with chronic low Back pain compared with healthy control individuals. J Pain. (2015) 16:1366–76. doi: 10.1016/j.jpain.2015.08.012
14. Asada, M, Shibata, M, Hirabayashi, N, Ohara, T, Furuta, Y, Nakazawa, T, et al. Association between chronic low back pain and regional brain atrophy in a Japanese older population: the Hisayama study. Pain. (2022) 163:2185–93. doi: 10.1097/j.pain.0000000000002612
15. Saygin, ZM, Kliemann, D, Iglesias, JE, van der Kouwe, AJW, Boyd, E, Reuter, M, et al. High-resolution magnetic resonance imaging reveals nuclei of the human amygdala: manual segmentation to automatic atlas. Neuroimage. (2017) 155:370–82. doi: 10.1016/j.neuroimage.2017.04.046
16. Zheng, F, Li, C, Zhang, D, Cui, D, Wang, Z, and Qiu, J. Study on the sub-regions volume of hippocampus and amygdala in schizophrenia. Quant Imaging Med Surg. (2019) 9:1025–36. doi: 10.21037/qims.2019.05.21
17. Sims, KS, and Williams, RS. The human amygdaloid complex: a cytologic and histochemical atlas using Nissl, myelin, acetylcholinesterase and nicotinamide adenine dinucleotide phosphate diaphorase staining. Neuroscience. (1990) 36:449–72. doi: 10.1016/0306-4522(90)90440-F
18. Bzdok, D, Laird, AR, Zilles, K, Fox, PT, and Eickhoff, SB. An investigation of the structural, connectional, and functional subspecialization in the human amygdala. Hum Brain Mapp. (2013) 34:3247–66. doi: 10.1002/hbm.22138
19. Moreno, N, and González, A. Evolution of the amygdaloid complex in vertebrates, with special reference to the anamnio-amniotic transition. J Anat. (2007) 211:151–63. doi: 10.1111/j.1469-7580.2007.00780.x
20. Kilts, CD, Egan, G, Gideon, DA, Ely, TD, and Hoffman, JM. Dissociable neural pathways are involved in the recognition of emotion in static and dynamic facial expressions. Neuroimage. (2003) 18:156–68. doi: 10.1006/nimg.2002.1323
21. Barbas, H, and De Olmos, J. Projections from the amygdala to basoventral and mediodorsal prefrontal regions in the rhesus monkey. J Comp Neurol. (1990) 300:549–71. doi: 10.1002/cne.903000409
22. Jimenez-Castellanos, J . The amygdaloid complex in monkey studies by reconstructional methods. J Comp Neurol. (1949) 91:507–26. doi: 10.1002/cne.900910308
23. Stocco, A, Lebiere, C, and Anderson, JR. Conditional routing of information to the cortex: a model of the basal ganglia's role in cognitive coordination. Psychol Rev. (2010) 117:541–74. doi: 10.1037/a0019077
24. Sears, RM, Schiff, HC, and LeDoux, JE. Molecular mechanisms of threat learning in the lateral nucleus of the amygdala. Prog Mol Biol Transl Sci. (2014) 122:263–304. doi: 10.1016/B978-0-12-420170-5.00010-6
25. Šimić, G, Tkalčić, M, Vukić, V, Mulc, D, Španić, E, Šagud, M, et al. Understanding emotions: origins and roles of the amygdala. Biomol Ther. (2021) 11:823. doi: 10.3390/biom11060823
26. Sah, P, Faber, ES, Lopez De Armentia, M, and Power, J. The amygdaloid complex: anatomy and physiology. Physiol Rev. (2003) 83:803–34. doi: 10.1152/physrev.00002.2003
27. Newman, SW . The medial extended amygdala in male reproductive behavior. A node in the mammalian social behavior network. Ann N Y Acad Sci. (1999) 877:242–57. doi: 10.1111/j.1749-6632.1999.tb09271.x
28. Martinez, RC, Carvalho-Netto, EF, Ribeiro-Barbosa, ER, Baldo, MV, and Canteras, NS. Amygdalar roles during exposure to a live predator and to a predator-associated context. Neuroscience. (2011) 172:314–28. doi: 10.1016/j.neuroscience.2010.10.033
29. Janak, PH, and Tye, KM. From circuits to behaviour in the amygdala. Nature. (2015) 517:284–92. doi: 10.1038/nature14188
30. Neugebauer, V, Li, W, Bird, GC, and Han, JS. The amygdala and persistent pain. Neuroscientist. (2004) 10:221–34. doi: 10.1177/1073858403261077
31. Canteras, NS, Simerly, RB, and Swanson, LW. Connections of the posterior nucleus of the amygdala. J Comp Neurol. (1992) 324:143–79. doi: 10.1002/cne.903240203
32. Scalia, F, and Winans, SS. The differential projections of the olfactory bulb and accessory olfactory bulb in mammals. J Comp Neurol. (1975) 161:31–55. doi: 10.1002/cne.901610105
33. Kemppainen, S, Jolkkonen, E, and Pitkänen, A. Projections from the posterior cortical nucleus of the amygdala to the hippocampal formation and parahippocampal region in rat. Hippocampus. (2002) 12:735–55. doi: 10.1002/hipo.10020
34. Last, AR, and Hulbert, K. Chronic low back pain: evaluation and management. Am Fam Physician. (2009) 79:1067–74.
35. Huskisson, EC . Measurement of pain. Lancet. (1974) 2:1127–31. doi: 10.1016/S0140-6736(74)90884-8
36. Fairbank, JC, Couper, J, Davies, JB, and O'Brien, JP. The Oswestry low back pain disability questionnaire. Physiotherapy. (1980) 66:271–3.
37. Veinante, P, Yalcin, I, and Barrot, M. The amygdala between sensation and affect: a role in pain. J Mol Psychiatry. (2013) 1:9. doi: 10.1186/2049-9256-1-9
38. Kami, K, Tajima, F, and Senba, E. Brain mechanisms of exercise-induced Hypoalgesia: to find a way out from "fear-avoidance belief". Int J Mol Sci. (2022) 23:2886. doi: 10.3390/ijms23052886
39. Cai, YQ, Wang, W, Paulucci-Holthauzen, A, and Pan, ZZ. Brain circuits mediating opposing effects on emotion and pain. J Neurosci. (2018) 38:6340–9. doi: 10.1523/JNEUROSCI.2780-17.2018
40. Thompson, JM, and Neugebauer, V. Cortico-limbic pain mechanisms. Neurosci Lett. (2019) 702:15–23. doi: 10.1016/j.neulet.2018.11.037
41. Thompson, JM, and Neugebauer, V. Amygdala plasticity and pain. Pain Res Manag. (2017) 2017:8296501. doi: 10.1155/2017/8296501
42. Bernard, JF, and Besson, JM. The spino(trigemino)pontoamygdaloid pathway: electrophysiological evidence for an involvement in pain processes. J Neurophysiol. (1990) 63:473–90. doi: 10.1152/jn.1990.63.3.473
43. Burstein, R, and Potrebic, S. Retrograde labeling of neurons in the spinal cord that project directly to the amygdala or the orbital cortex in the rat. J Comp Neurol. (1993) 335:469–85. doi: 10.1002/cne.903350402
44. Wang, CC, Willis, WD, and Westlund, KN. Ascending projections from the area around the spinal cord central canal: a Phaseolus vulgaris leucoagglutinin study in rats. J Comp Neurol. (1999) 415:341–67. doi: 10.1002/(SICI)1096-9861(19991220)415:3<341::AID-CNE3>3.0.CO;2-7
45. Schwaber, JS, Sternini, C, Brecha, NC, Rogers, WT, and Card, JP. Neurons containing calcitonin gene-related peptide in the parabrachial nucleus project to the central nucleus of the amygdala. J Comp Neurol (1988);270:416–26, 398–9, 426, doi: 10.1002/cne.902700310
46. Dobolyi, A, Irwin, S, Makara, G, Usdin, TB, and Palkovits, M. Calcitonin gene-related peptide-containing pathways in the rat forebrain. J Comp Neurol. (2005) 489:92–119. doi: 10.1002/cne.20618
47. Harrigan, EA, Magnuson, DJ, Thunstedt, GM, and Gray, TS. Corticotropin releasing factor neurons are innervated by calcitonin gene-related peptide terminals in the rat central amygdaloid nucleus. Brain Res Bull. (1994) 33:529–34. doi: 10.1016/0361-9230(94)90079-5
48. Gray, TS . Amygdaloid CRF pathways. role in autonomic, neuroendocrine, and behavioral responses to stress. Ann N Y Acad Sci. (1993) 697:53–60. doi: 10.1111/j.1749-6632.1993.tb49922.x
49. Andreoli, M, Marketkar, T, and Dimitrov, E. Contribution of amygdala CRF neurons to chronic pain. Exp Neurol. (2017) 298:1–12. doi: 10.1016/j.expneurol.2017.08.010
50. Carmichael, ST, Clugnet, MC, and Price, JL. Central olfactory connections in the macaque monkey. J Comp Neurol. (1994) 346:403–34. doi: 10.1002/cne.903460306
51. Keshavarzi, S, Sullivan, RK, Ianno, DJ, and Sah, P. Functional properties and projections of neurons in the medial amygdala. J Neurosci. (2014) 34:8699–715. doi: 10.1523/JNEUROSCI.1176-14.2014
52. Lötsch, J, Hähner, A, Gossrau, G, Hummel, C, Walter, C, Ultsch, A, et al. Smell of pain: intersection of nociception and olfaction. Pain. (2016) 157:2152–7. doi: 10.1097/j.pain.0000000000000599
53. Marchand, S, and Arsenault, P. Odors modulate pain perception: a gender-specific effect. Physiol Behav. (2002) 76:251–6. doi: 10.1016/S0031-9384(02)00703-5
54. Villemure, C, Slotnick, BM, and Bushnell, MC. Effects of odors on pain perception: deciphering the roles of emotion and attention. Pain. (2003) 106:101–8. doi: 10.1016/S0304-3959(03)00297-5
55. Gedney, JJ, Glover, TL, and Fillingim, RB. Sensory and affective pain discrimination after inhalation of essential oils. Psychosom Med. (2004) 66:599–606. doi: 10.1097/01.psy.0000132875.01986.47
56. Martin, GN . The effect of exposure to odor on the perception of pain. Psychosom Med. (2006) 68:613–6. doi: 10.1097/01.psy.0000227753.35200.3e
57. Allen, HN, Bobnar, HJ, and Kolber, BJ. Left and right hemispheric lateralization of the amygdala in pain. Prog Neurobiol. (2021) 196:101891. doi: 10.1016/j.pneurobio.2020.101891
58. Tsagareli, MG, Tsiklauri, N, Gurtskaia, G, Nozadze, I, and Abzianidze, EJH. The central nucleus of amygdala is involved in tolerance to the antinociceptive effect of NSAIDs. J Pain Res. (2010) 2:62–6. doi: 10.4236/health.2010.21010
Keywords: chronic low back pain, amygdala subregions, FreeSurfer, magnetic resonance imaging, structural neuroimaging
Citation: Gu S-Y, Shi F-C, Wang S, Wang C-Y, Yao X-X, Sun Y-F, Hu J-B, Chen F, Pan P-L and Li W-H (2024) Altered volume of the amygdala subregions in patients with chronic low back pain. Front. Neurol. 15:1351335. doi: 10.3389/fneur.2024.1351335
Edited by:
John R. Absher, Prisma Health Neuroscience Associates, United StatesCopyright © 2024 Gu, Shi, Wang, Wang, Yao, Sun, Hu, Chen, Pan and Li. This is an open-access article distributed under the terms of the Creative Commons Attribution License (CC BY). The use, distribution or reproduction in other forums is permitted, provided the original author(s) and the copyright owner(s) are credited and that the original publication in this journal is cited, in accordance with accepted academic practice. No use, distribution or reproduction is permitted which does not comply with these terms.
*Correspondence: Wen-Hui Li, eWNzeWx3aEBnbWFpbC5jb20=; Ping-Lei Pan, cGFucGluZ2xlaUAxNjMuY29t; Fei Chen, c2h1aWJpbjE5ODhAMTYzLmNvbQ==
†These authors have contributed equally to this work