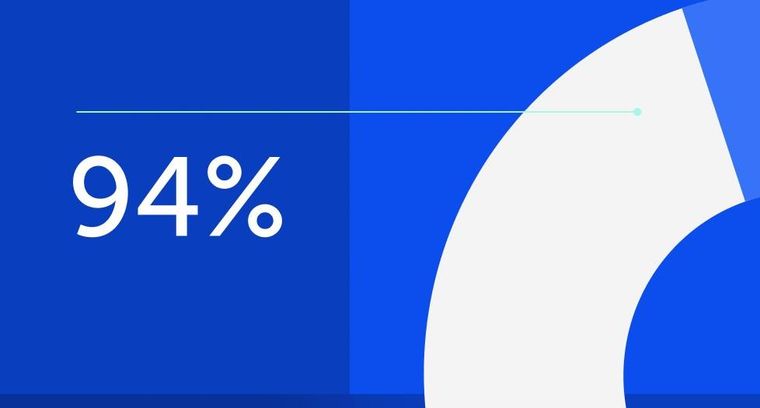
94% of researchers rate our articles as excellent or good
Learn more about the work of our research integrity team to safeguard the quality of each article we publish.
Find out more
ORIGINAL RESEARCH article
Front. Neurol., 03 May 2024
Sec. Neurocritical and Neurohospitalist Care
Volume 15 - 2024 | https://doi.org/10.3389/fneur.2024.1340749
Aim: We aimed to describe the neurosonological findings related to cerebral hemodynamics acquired using transcranial Doppler and to determine the frequency of elevated ICP by optic nerve sheath diameter (ONSD) measurement in patients with severe coronavirus disease (COVID-19) hospitalized in the intensive care unit of a national referral hospital in Peru.
Methods: We included a retrospective cohort of adult patients hospitalized with severe COVID-19 and acute respiratory failure within the first 7 days of mechanical ventilation under deep sedoanalgesia, with or without neuromuscular blockade who underwent ocular ultrasound and transcranial Doppler. We determine the frequency of elevated ICP by measuring the diameter of the optic nerve sheath, choosing as best cut-off value a diameter equal to or >5.8 mm. We also determine the frequency of sonographic patterns obtained by transcranial Doppler. Through insonation of the middle cerebral artery. Likewise, we evaluated the associations of clinical, mechanical ventilator, and arterial blood gas variables with ONSD ≥5.8 mm and pulsatility index (PI) ≥1.1. We also evaluated the associations of hemodynamic findings and ONSD with mortality the effect size was estimated using Poisson regression models with robust variance.
Results: This study included 142 patients. The mean age was 51.39 ± 13.3 years, and 78.9% of patients were male. Vasopressors were used in 45.1% of patients, and mean arterial pressure was 81.87 ± 10.64 mmHg. The mean partial pressure of carbon dioxide (PaCO2) was elevated (54.08 ± 16.01 mmHg). Elevated intracranial pressure was seen in 83.1% of patients, as estimated based on ONSD ≥5.8 mm. A mortality rate of 16.2% was reported. In the multivariate analysis, age was associated with elevated ONSD (risk ratio [RR] = 1.07). PaCO2 was a protective factor (RR = 0.64) in the cases of PI ≥ 1.1. In the mortality analysis, the mean velocity was a risk factor for mortality (RR = 1.15).
Conclusions: A high rate of intracranial hypertension was reported, with ONSD measurement being the most reliable method for estimation. The increase in ICP measured by ONSD in patients with severe COVID-19 on mechanical ventilation is not associated to hypercapnia or elevated intrathoracic pressures derived from protective mechanical ventilation.
The coronavirus disease (COVID-19) pandemic was declared on March 11, 2020 (1), and it was not until May 5, 2023, that the World Health Organization (WHO) announced that the global health emergency caused by this disease had ended (2). In recent years, it has been shown that severe acute respiratory syndrome coronavirus 2 (SARS-CoV-2) infection not only involves the respiratory system but also other organs and systems, such as the nervous system (3).
Early studies on the neurological manifestations of COVID-19 reported on the involvement of the central and peripheral nervous system in up to 36% of patients. The reported symptoms included dizziness, headache, delirium, stroke, altered taste, and altered smell, among other manifestations (3).
Neurological manifestations have also been reported in patients with acute respiratory failure on mechanical ventilation owing to severe COVID-19, and delirium was the main neurological manifestation that was described (4). Critically ill patients with severe SARS-CoV-2 infection require elevated oxygen and ventilatory and hemodynamic support; therefore, deep sedation and neuromuscular blockade are necessary (5–7).
This critical condition limits the neurological evaluation of these patients to pupillary examination and imaging modalities, such as computed tomography (CT) or magnetic resonance imaging (MRI); these examinations require transfer to imaging rooms and the results are complicated hindered by patients being placed in respiratory isolation and their hemodynamic status (8–10).
Neurosonology techniques have proven to be useful, practical, and noninvasive techniques that can be used in critically ill patients. The main advantage of these techniques is the possibility of performing them without moving the patient (11–14).
Transcranial Doppler (TCD) is a non-invasive technique that provides real-time measurement of blood flow velocity in the cerebral arteries, which correlates with cerebral blood flow (CBF), allowing for the assessment of cerebral hemodynamics in critically ill patients. With TCD, the use of invasive CBF measurement techniques can be avoided, while similar prognostic information can be acquired (15, 16).
Optic nerve sheath diameter (ONSD) measurement is an established technique for the assessment of intracranial pressure (ICP) and detection of intracranial hypertension in critically ill patients. It is widely available, cost-effective, time-effective, and non-invasive and does not require additional resources. Therefore, an extended ONSD is a robust predictor of elevated ICP (17–19).
The aim of the present study was to determine the frequency of elevated ICP by optic nerve sheath diameter (ONSD) measurement and describe the neurosonological findings related to cerebral hemodynamics acquired via TCD in patients with severe COVID-19 hospitalized in the intensive care unit of a national referral hospital in Peru.
This retrospective cohort study was conducted in the critical respiratory unit of a national referral hospital in Peru, which is intended exclusively for the care of critically ill patients diagnosed with COVID-19.
The study population included patients older than 18 years, who were hospitalized in the intensive care unit of the emergency department during the COVID-19 pandemic in Peru from May 23 to December 31, 2020; these patients were diagnosed with severe COVID-19 and acute respiratory failure, and they were included within the first 7 days of mechanical ventilation under deep sedoanalgesia, with or without neuromuscular blockade.
Patients with a clinical manifestation compatible with that of COVID-19 and positive rapid serological test (immunoglobulin M or G) or nasopharyngeal swab reverse transcriptase polymerase chain reaction (RT-PCR) test were considered as COVID-19 patients.
The severity criteria of the Infectious Diseases Society of America/American Thoracic Society were used to determine the severity of COVID-19 pneumonia (20): Patients with COVID-19 and Acute Respiratory Failure on Mechanical Ventilation with Hypoxemia PaO2/FiO2 ≤ 300 + PEEP ≥ 5 cmH2O and Chest CT bilateral with peripheral, and sub-pleural or peribroncho-vascular areas of patchy or segmental pure ground-glass opacities with the involvement of lower lobes, rare consolidations, reverse halo sign and vascular dilatation.
Patients with hemodynamic instability, those with hypotension (mean arterial pressure [MAP] < 60 mmHg) despite the use of vasopressors, and those with refractory hypoxemia (arterial oxygen partial pressure [PaO2] < 40 mmHg with fractional inspired oxygen [FiO2] = 100%) were excluded.
Non-probabilistic consecutive selections were performed, which included the entire universe of patients in the study during the established period, resulting in a total of 142 selected participants. Information was collected from the enrolled patients' clinical history (physical or electronic) recorded from admission to discharge or death.
The study was approved by the ethics committee of our institution, formed exclusively to evaluate research on COVID 19.
Demographic data (age and sex) and vital function at admission were recorded. The systolic blood pressure (SBP), diastolic blood pressure (DBP) and mean arterial pressure (MAP) values were obtained and recorded from a multiparameter monitor using an invasive arterial catheter. Similarly, the end tidal carbon dioxide (etCO2) levels and temperature values were recorded at the same time as sonographic studies. During the hospital follow-up, mortality outcomes (discharge or death) were recorded.
Mechanical ventilator parameters such as positive end-expiratory pressure (PEEP), peak airway pressure (Ppeak), plateau pressure (Pplateau), mean airway pressure (Pmean), and pulmonary compliance (Cst) were measured and recorded just before starting the sonographic studies.
Blood samples were obtained from arterial lines for arterial gas analysis. The hemoglobin concentration was obtained and recorded from first blood test performed in ICU. Arterial blood gases were obtained simultaneously with ocular ultrasound and transcranial Doppler. Arterial oxygen partial pressure (PaO2), arterial carbon dioxide partial pressure (PaCO2), arterial oxygen saturation (SatO2), and pH were measured and recorded, these samples were analyzed immediately.
To obtain information about neurological compromise, neurosonology was performed as a routine practice in all intubated patients with severe COVID-19 hospitalized in the intensive care unit. Sonological measurement in our patient cohort was done when patients consistently achieved SpO2 > 92% and/or MAP > 60 mmHg on the multiparametric monitor, regardless of FiO2 levels and required vasopressor doses, all of this to avoid the influence of hypoxemia and hypotension in the results obtained from neurosonology studies. No daily evaluations of ONSD and TCD were done, only a single measurement was performed, first the transcranial Doppler was performed and immediately after the ONSD. Normally this measurement was made between 24 to 48 h after intubation and mechanical ventilation. No patient received anti-cerebral edema measures before the neurosonology studies. At the time of performing the ultrasound evaluations, all our patients were in a supine position to avoid the influence of the prone position on ONSD and TCD.
Sonographic examinations were conducted using an ATYS MEDICAL LOOKI Transcranial Doppler equipped with a 2 MHz transducer and a General Electric Vivid T8 Ultrasound Machine equipped with a 6.0–13.0 MHz linear probe used for ocular ultrasound. The Sonographic examinations were done by one experienced sonographer trained in ocular sonography and transcranial Doppler.
The Transcranial Doppler (TCD) exams were performed in the middle cerebral arteries and the peak systolic velocity (PSV), end-diastolic velocity (EDV), mean velocity (MFV), and pulsatility index (PI) were measured and recorded. The middle cerebral artery was identified through the temporal window with an insonation depth at 40–65 mm from the skull surface. Immediately after TCD measurements, optic nerve studies were performed. The ONSD was measured 3 mm posterior to the papilla. The ONSD recorded was the mean value obtained from at least 3 repeated measurements in both eyes.
The information was recorded in a Microsoft Excel (2016) database and was exported to STATA v17. In brief, categorical variables were expressed as frequency and percentage, while normally distributed quantitative variables were described as mean and standard deviation; partial pressure of carbon dioxide (PaCO2), PaO2, ONSD, and lung compliance were not normally distributed and were, therefore, presented as median and interquartile range.
As the proportion of missing data was null for most variables, no statistical artifacts were used to deal with missing data.
We determined the frequency of elevated ICP by measuring the optic nerve sheath, choosing as the best cut-off value a diameter equal to or >5.8 mm. We also determined the frequency of sonographic patterns obtained by transcranial Doppler through insonation of the middle cerebral artery, to establish the cerebral ultrasound patterns we use the diagnostic correlation diagram proposed by Muñoz Sanchez et al. (21).
To evaluate the associations of clinical, mechanical ventilator, and arterial blood gas variables with ONSD ≥ 5.8 mm and PI ≥ 1.1, hypothesis tests were performed according to the nature of the independent variables. The chi-square test or Fisher's exact test was used for categorical variables, whereas Student's t-test or the Mann-Whitney U test was used for quantitative variables. Likewise, the associations of hemodynamic findings acquired by TCD and ONSD with mortality outcomes were evaluated.
Finally, we estimated the effect size (relative risk [RR] and 95% confidence intervals [95% CI]) by creating Poisson regression models with robust variance. Crude models were adjusted for age, sex, and PaCO2. Statistical significance was set at p < 0.05.
The information used in this study was collected from clinical histories; therefore, informed consent was not required. The authors undertook to maintain the confidentiality of the patients, whose data were collected without identifiers, and to respect the veracity of the information. All collected information was stored in a safe place, and access was granted only to the principal investigator, who was solely responsible for safeguarding this information.
The study was conducted in accordance with the Declaration of Helsinki and Belmont Report, and patient anonymity was maintained. The research protocol was approved by the COVID-19 Research Ethics Committee of the Social Health Insurance program.
A total of 142 patients were enrolled in the study. The mean age was 51.39 ± 13.3 years, and 78.9% were men. In addition, vasopressors were used in 45.1% of the patients, and the mean MAP was 81.87 ± 10.64 mmHg. Additionally, the mean PaCO2 was elevated reaching 54.08 ± 16.01 mmHg.
We found that ONSD was elevated (≥5.8 mm) in 83.1% of the patients and that PI was elevated (≥1.1) in 40.1%. Moreover, the pulsatility pattern was normal in 48.6% of the patients (PI = 0.7–1.0) and was low in the rest (11.3%) (PI < 0.7). The reported mortality rate was 16.2%. Further details regarding the general characteristics of the patients are presented in Table 1.
Regarding the cerebral sonographic patterns found through TCD, 24% of the patients exhibited a low velocity pattern (MFV < 40 cm/s), 22% exhibited a high velocity pattern (MFV > 79 cm/s), and the majority (54%) exhibited a normal velocity pattern (MFV = 40–79 cm/s).
The following hemodynamic patterns were found: cerebral hypoperfusion (24%), cerebral hyperperfusion (22%), normal blood flow (30%), and normalized (abnormal) blood flow with intracranial hypertension (24%).
On comparing demographic, clinical, sonographic, and ventilatory characteristics according to ONSD and PI findings, significant differences were found in oxygen saturation (SpO2) (difference in SpO2 between patients with ONSD < 5.8 mm and those ONSD ≥ 5.8 mm = 0.3%; p = 0.02). Similarly, on comparing gasometric (PaCO2 and SpO2), TCD (PSV, EDV, and MFV), and ventilatory (positive end-expiratory pressure [PEEP], peak airway pressure [Ppeak], plateau pressure [Pplateau], mean airway pressure [Pmean], and pulmonary compliance [Cst]) variables according to MAP, significant differences were found between the groups with and without elevated PI. Notably, on comparing the distribution of both ONSD and PI patterns, we found that there no significant differences between the proportions of patients who survived and those who did not (Table 1).
On evaluating associations using Poisson regression models, it was found that none of the variables were significantly associated with a greater risk of presenting with ONSD ≥ 5.8 mm. For every 10 mmHg increase in PaCO2, the risk of presenting with PI ≥ 1.1 decreased by 39% (95% CI: 0.50–0.74). The results related to all the mechanical ventilation variables were significant in the crude PI analysis (Table 2).
Table 2. Simple regression of hemodynamic parameters in patients with COVID-19 according to ONSD and PI.
In the multivariate analysis of hemodynamic variables according to ONSD and PI, age was associated with increased ONSD such that for every 10-year increase in age, the risk of ONSD being ≥5.8 increased by 7%. In the multiple regression analysis of PI ≥ 1.1, only PaCO2 maintained its statistical association as a protective factor at 36% for every 10-mmHg increase (Table 3).
Table 3. Multiple regression of hemodynamic parameters in patients with COVID-19 according to ONSD and PI.
On comparing demographic, clinical, sonographic, and ventilatory characteristics according to mortality, significant differences were found in the use of vasopressors and hemoglobin (Hb) levels (difference in Hb between surviving and deceased patients = 1.7; p < 0.001). Similarly, significant differences were found in the TCD variables (PSV and MFV), as well as in the ventilator variables (PEEP, Ppeak, Pmean, and Cst except for in P Plateau; Table 4).
On evaluating associations using Poisson regression models, SPV and MFV were identified as risk factors for mortality in the crude analysis. In the multivariate analysis of hemodynamic variables according to mortality, MFV had an adjusted RR of 1.15, implying that for every 1-unit increase in MFV, the risk of mortality increased by 15% (95% CI: 1.03–1.28; Table 5).
Table 5. Simple and multiple regression of the associations of hemodynamic parameters and ONSD with mortality in patients with COVID-19.
The present study was conducted with the objective of describing neurosonological and ultrasound findings related to cerebral hemodynamics acquired using TCD and ONSD measurement in patients with severe COVID-19 hospitalized in the intensive care unit of a national referral hospital in Peru; additionally, factors associated with neurosonological and ultrasound findings related to intracranial hypertension and the association of cerebral hemodynamic variables and ONSD with mortality were also explored.
The most relevant finding of this study was that a very large proportion (83%) of patients who were admitted with acute respiratory failure due to severe COVID-19 and were on mechanical ventilation presented with ONSD ≥ 5.8 mm. In addition, it is important to highlight the following findings: of the 142 patients included in this study, 23 patients (16%) died, and of these 23 deceased patients, four died due to intracranial hypertension resulting in brain death.
ONSD has been widely studied as a useful, reliable, and non-invasive measure for estimating ICP. The accuracy of ONSD for the detection of elevated ICP was evaluated through a meta-analysis, where the pooled area under the receiver operating characteristic (ROC) curve for ONSD was reported to be 0.94 (22), 0.93, (17) and 0.94 (23) by different studies. A significant linear correlation was found between ONSD and ICP; therefore, the diagnostic accuracy of ONSD acquired via ultrasound was found to be good when used to detect intracranial hypertension (24, 25).
However, there is no consensus regarding the optimal ONSD threshold for detecting elevated ICP. Wang et al. found that extended ONSD was a strong predictor of elevated ICP; an ONSD cutoff of 5.83 mm indicated an estimated ICP of > 22 mmHg with a sensitivity of 94.4% and specificity of 81.0% (26). Geeraerts reported that 5.86 mm is the ideal ONSD cutoff value (sensitivity, 95%; specificity, 79%) for detecting elevated ICP, (25) and Robba found that the ideal cutoff value for predicting ICP > 20 mmHg was 5.85 mm (27). Therefore, we chose the cutoff value of 5.8 mm in order to estimate the frequency of intracranial hypertension in our study.
An important finding of this study was the lack of an association between elevated carbon dioxide (CO2) levels and ONSD. Patients who underwent ocular ultrasound had acute respiratory distress syndrome caused by SARS-CoV-2 and were on mechanical ventilation with low tidal volumes (protective mechanical ventilation) (28–31), which resulted in increased arterial CO2 pressure (PaCO2).
The mean PaCO2 in the study population was 54 mmHg. We first considered that this permissive hypercapnia could be a physiological cause of the increase in ONSD in these patients, as the arteriolar vasodilator effect would lead to an increase in CBF, which in turn would increase ICP. However, on evaluating the RR between PaCO2 and ONSD, we found no risk, with a non-significant RR of 1.02 (95% CI: 0.98–1.06; p = 0.312); therefore, we established that the increase in ONSD was not associated with the hypercapnia due to which these patients underwent mechanical ventilation.
On the other hand, the patients were subjected to high levels of PEEP, with an average PEEP level of 14 ± 3 mmHg. These elevated PEEP levels lead to an increase in mean airway pressure [Pmean], and intrathoracic positive pressure levels, resulting in a decrease in venous return to the heart, with a consequent decrease in cerebral venous drainage and an increase in ICP.
However, the risk analysis did not reveal any relationship between positive intrathoracic pressures (PEEP, Pm, Pplateau, and Ppeak) and ONSD ≥ 5.8 mm; therefore, the finding of increased ONSD was not associated with the levels of PEEP, Pm, Pplateau, Ppeak, Cst, and PaCO2.
Based on the above findings, we can conclude that 83% of our patients with severe COVID-19 on mechanical ventilation exhibited an increase in ICP, as indicated by the ONSD measurement, and that this increase was due to COVID-19 and was not associated with an external factor resulting from the treatment administered. Whether this increase in ICP in patients with severe COVID-19 was due to direct invasion of the virus into the central nervous system or whether it was a secondary development to a systemic inflammatory response could not be determined in this study, and further studies are needed to clarify this.
With regard to the TCD findings, we used a correlation diagram between the ultrasound patterns and cerebral hemodynamic status in our patients, as proposed by Muñoz Sánchez et al. (21) The most striking finding was that 24% of the patients exhibited low velocity echographic patterns, which translates into hemodynamic patterns indicative of low CBF or cerebral hypoperfusion; notably, decreased CBF was found in the absence of arterial hypotension or shock, and a systemic cause for this decrease was not found.
To add to the finding that 83% of patients had increased ICP, as estimated through ONSD, we could suggest that the cause of the decrease in CBF was elevated ICP. Helms et al. found that 100% of patients with severe COVID-19 on mechanical ventilation had cerebral hypoperfusion at the temporal and frontal levels (4).
Another important TCD finding was that 48% of our patients had a high pulsatility pattern; however, this percentage was underestimated because PI values are intimately influenced by PaCO2 levels, as the higher the PaCO2, the lower the PI (32). Therefore, if these patients were not managed with permissive hypercapnia and were instead in normocapnia, the proportion of patients with elevated PI would have been larger.
There are various causes of elevated PI, including intracranial hypertension (33), hypocapnia (21, 32), microangiopathy (34, 35), intracranial vascular stenosis (36), and brain death (37). Thus, an increase in PI does not represent an increase in ICP in every patient. Hence, TCD is not a reliable method to estimate increased ICP, and its usefulness is inferior to that of ONSD (22, 38). However, the frequency of patients with severe COVID-19 on mechanical ventilation having elevated PI was high, which supports the ONSD-based findings.
Twenty-two percent of our patients exhibited an increased mean velocity (VFM ≥ 80 cm/s). Furthermore, we found that increased MFV and systolic velocity were associated with an increased risk of mortality in the simple regression analysis; one explanation for this is that the hyperemia could be reactive to acute endothelial dysfunction, cerebral vascular vasodilatation, and/or sepsis-induced impairment of cerebral autoregulation (39). Therefore, the magnitude of increased MFV would reflect a greater level of systemic inflammation secondary to SARS-CoV-2 sepsis.
In the multiple regression analysis, MFV was found to be a risk factor for mortality (RR: 1.15), which can be explained by a potential relationship between the systemic inflammatory response caused by COVID-19 and increased velocities in cerebral arteries; this deterioration of CBF may be due to the release of inflammatory mediators, as evidenced in other critical conditions.
However, regarding the relationship between PaCO2 levels and cerebral artery velocities, our study demonstrated a close inversely proportional relationship between PaCO2 levels and PI (p < 0.001): the higher the PaCO2 level, the lower the PI. This causes a fall in cerebral vascular resistance and, therefore, an increase in MFV of the cerebral arteries.
Previously published studies have addressed the issue of neurosonological findings in critically ill patients with COVID-19 and their clinical outcomes (40–42); our study, in addition to describing the neurosonological findings, studied the association of hemodynamic and ventilatory parameters with neurosonological findings and mortality. Recording ventilatory mechanics to interpret the results is essential because intrathoracic pressures are very high and the lungs are very damaged, this could cause a decrease in cerebral venous return and increase ICP, therefore, reliable conclusions cannot be obtained without analyzing the relationship between these variables.
The main limitation of this study was that the estimation of ICP was performed non-invasively, and the methods used were operator-dependent. In addition, most enrolled patients had hypercapnia, which could limit the generalizability of the conclusions of our study. Subsequent studies on a population of mechanically ventilated patients with normocapnia, which involve invasive ICP measurements, are needed to corroborate our findings. The present study has some strengths. The study population comprised a well-selected group of critical patients on mechanical ventilation in the acute phase of COVID-19, and ventilatory and arterial gas parameters were recorded and simultaneously analyzed at the time of the neurosonology examinations; this increases internal validity and reduces risk of bias.
A very high rate of intracranial hypertension was found in patients with severe COVID-19, as estimated using noninvasive ultrasound and neurosonology techniques, with ONSD measurement being the most reliable method. This study allows us to establish that the increase in ICP measured by ONSD in patients with severe COVID-19 on mechanical ventilation is not associated to hypercapnia or elevated intrathoracic pressures derived from protective mechanical ventilation.
The raw data supporting the conclusions of this article will be made available by the authors, without undue reservation.
OH-O: Conceptualization, Data curation, Investigation, Methodology, Project administration, Resources, Supervision, Writing–original draft, Writing–review & editing. MV: Data curation, Methodology, Software, Supervision, Writing–original draft, Writing–review & editing. VF-R: Data curation, Formal analysis, Methodology, Software, Writing–original draft, Writing–review & editing. DF-M: Data curation, Investigation, Methodology, Software, Writing–original draft, Writing–review & editing. RL-C: Project administration, Resources, Supervision, Validation, Writing–original draft, Writing–review & editing. RV: Data curation, Investigation, Project administration, Resources, Supervision, Writing–original draft, Writing–review & editing.
The author(s) declare that no financial support was received for the research, authorship, and/or publication of this article.
The authors thank the health personnel working in Intensive Care Unit 3 of Edgardo Rebagliati Martins Hospital for their collaboration in carrying out this study, with special thanks to the intensivists for their dedication, empathy, and professionalism in their service to the patients. In addition, I (OH-O) would like to thank my family, especially my daughters Gabriela and Fabiana.
The authors declare that the research was conducted in the absence of any commercial or financial relationships that could be construed as a potential conflict of interest.
All claims expressed in this article are solely those of the authors and do not necessarily represent those of their affiliated organizations, or those of the publisher, the editors and the reviewers. Any product that may be evaluated in this article, or claim that may be made by its manufacturer, is not guaranteed or endorsed by the publisher.
1. WHO Director. General's opening remarks at the media briefing on COVID-19. (2020). Available online at: https://www.who.int/es/director-general/speeches/detail/who-director-general-s-opening-remarks-at-the-media-briefing-on-covid-19—11-march-2020 (accessed March 27, 2024).
2. WHO Director. General's opening remarks at the media briefing. (2023). Available online at: https://www.who.int/director-general/speeches/detail/who-director-general-s-opening-remarks-at-the-media-briefing—5-may-2023 (accessed March 27, 2024).
3. Mao L, Jin H, Wang M, Hu Y, Chen S, He Q, et al. Neurologic manifestations of hospitalized patients with coronavirus disease 2019 in Wuhan, China. JAMA Neurol. (2020) 77:683–90. doi: 10.1001/jamaneurol.2020.1127
4. Helms J, Kremer S, Merdji H, Clere-Jehl R, Schenck M, Kummerlen C, et al. Neurologic features in severe SARS-CoV-2 infection. N Engl J Med. (2020) 382:2268–70. doi: 10.1056/NEJMc2008597
5. Meyer NJ, Gattinoni L, Calfee CS. Acute respiratory distress syndrome. Lancet. (2021) 398:622–37. doi: 10.1016/S0140-6736(21)00439-6
6. Gibson PG, Qin L, Puah SH. COVID-19 acute respiratory distress syndrome (ARDS): clinical features and differences from typical pre-COVID-19 ARDS. Med J Aust. (2020) 213:54–56.e1. doi: 10.5694/mja2.50674
7. Parhar KKS, Zuege DJ, Shariff K, Knight G, Bagshaw SM. Prone positioning for ARDS patients—tips for preparation and use during the COVID-19 pandemic. Can J Anaesth. (2021) 68:541–5. doi: 10.1007/s12630-020-01885-0
8. Murata M, Nakagawa N, Kawasaki T, Yasuo S, Yoshida T, Ando K, et al. Adverse events during intrahospital transport of critically ill patients: a systematic review and meta-analysis. Am J Emerg Med. (2022) 52:13–9. doi: 10.1016/j.ajem.2021.11.021
9. Bergman L, Pettersson M, Chaboyer W, Carlström E, Ringdal M. Improving quality and safety during intrahospital transport of critically ill patients: a critical incident study. Aust Crit Care. (2020) 33:12–9. doi: 10.1016/j.aucc.2018.12.003
10. Jia L, Wang H, Gao Y, Liu H, Yu K. High incidence of adverse events during intra-hospital transport of critically ill patients and new related risk factors: a prospective, multicenter study in China. Crit Care. (2016) 20:12. doi: 10.1186/s13054-016-1183-y
11. Hakimi R, Alexandrov AV, Garami Z. Neuro-ultrasonography. Neurol Clin. (2020) 38:215–29. doi: 10.1016/j.ncl.2019.09.006
12. Lau VI, Jaidka A, Wiskar K, Packer N, Tang JE, Koenig S, et al. Better with ultrasound: transcranial Doppler. Chest. (2020) 157:142–50. doi: 10.1016/j.chest.2019.08.2204
13. Robba C, Goffi A, Geeraerts T, Cardim D, Via G, Czosnyka M, et al. Brain ultrasonography: methodology, basic and advanced principles and clinical applications. A narrative review. Intensive Care Med. (2019) 45:913–27. doi: 10.1007/s00134-019-05610-4
14. Lau VI, Arntfield RT. Point-of-care transcranial Doppler by intensivists. Crit Ultrasound J. (2017) 9:21. doi: 10.1186/s13089-017-0077-9
15. Bonow RH, Young CC, Bass DI, Moore A, Levitt MR. Transcranial Doppler ultrasonography in neurological surgery and neurocritical care. Neurosurg Focus. (2019) 47:E2. doi: 10.3171/2019.9.FOCUS19611
16. Bertuetti R, Gritti P, Pelosi P, Robba C. How to use cerebral ultrasound in the ICU. Minerva Anestesiol. (2020) 86:327–40. doi: 10.23736/S0375-9393.19.13852-7
17. Robba C, Santori G, Czosnyka M, Corradi F, Bragazzi N, Padayachy L, et al. Optic nerve sheath diameter measured sonographically as non-invasive estimator of intracranial pressure: a systematic review and meta-analysis. Intensive Care Med. (2018) 44:1284–94. doi: 10.1007/s00134-018-5305-7
18. Lochner P, Czosnyka M, Naldi A, Lyros E, Pelosi P, Mathur S, et al. Optic nerve sheath diameter: present and future perspectives for neurologists and critical care physicians. Neurol Sci. (2019) 40:2447–57. doi: 10.1007/s10072-019-04015-x
19. Robba C, Bacigaluppi S, Cardim D, Donnelly J, Bertuccio A, Czosnyka M. Non-invasive assessment of intracranial pressure. Acta Neurol Scand. (2016) 134:4–21. doi: 10.1111/ane.12527
20. Metlay JP, Waterer GW, Long AC, Anzueto A, Brozek J, Crothers K, et al. Diagnosis and treatment of adults with community-acquired pneumonia. An official clinical practice guideline of the American thoracic society and infectious diseases society of America. Am J Respir Crit Care Med. (2019) 200:e45–67. doi: 10.1164/rccm.201908-1581ST
21. Muñoz Sánchez MA, Murillo Cabezas F, Rivera Fernández MV, Rincón Ferrari D, Amaya Villar R, Flores Cordero JM, et al. The effectiveness of a correlation diagram of the echographic and haemodynamic patterns in the brain. Rev Neurol. (2004) 38:411–6.
22. Fernando SM, Tran A, Cheng W, Rochwerg B, Taljaard M, Kyeremanteng K, et al. Diagnosis of elevated intracranial pressure in critically ill adults: systematic review and meta-analysis. BMJ. (2019) 366:l4225. doi: 10.1136/bmj.l4225
23. Dubourg J, Javouhey E, Geeraerts T, Messerer M, Kassai B. Ultrasonography of optic nerve sheath diameter for detection of raised intracranial pressure: a systematic review and meta-analysis. Inten Care Med. (2011) 37:1059–68. doi: 10.1007/s00134-011-2224-2
24. Soldatos T, Karakitsos D, Chatzimichail K, Papathanasiou M, Gouliamos A, Karabinis A. Optic nerve sonography in the diagnostic evaluation of adult brain injury. Crit Care. (2008) 12:R67. doi: 10.1186/cc6897
25. Geeraerts T, Merceron S, Benhamou D, Vigué B, Duranteau J. Non-invasive assessment of intracranial pressure using ocular sonography in neurocritical care patients. Intensive Care Med. (2008) 34:2062–7. doi: 10.1007/s00134-008-1149-x
26. Wang J, Li K, Li H, Ji C, Wu Z, Chen H, et al. Ultrasonographic optic nerve sheath diameter correlation with ICP and accuracy as a tool for noninvasive surrogate ICP measurement in patients with decompressive craniotomy. J Neurosurg. (2019) 133:1–7. doi: 10.3171/2019.4.JNS183297
27. Robba C, Cardim D, Tajsic T, Pietersen J, Bulman M, Donnelly J, et al. Ultrasound non-invasive measurement of intracranial pressure in neurointensive care: a prospective observational study. PLoS Med. (2017) 14:e1002356. doi: 10.1371/journal.pmed.1002356
28. Welker C, Huang J, Gil IJN, Ramakrishna H. 2021 Acute respiratory distress syndrome update, with coronavirus disease 2019 focus. J Cardiothorac Vasc Anesth. (2022) 36:1188–95. doi: 10.1053/j.jvca.2021.02.053
29. Attaway AH, Scheraga RG, Bhimraj A, Biehl M, Hatipoglu U. Severe Covid-19 pneumonia: pathogenesis and clinical management. BMJ. (2021) 372:n436. doi: 10.1136/bmj.n436
30. De Jong A, Wrigge H, Hedenstierna G, Gattinoni L, Chiumello D, Frat JP, et al. How to ventilate obese patients in the ICU. Intensive Care Med. (2020) 46:2423–35. doi: 10.1007/s00134-020-06286-x
31. Acute Respiratory Distress Syndrome Network. Ventilation with lower tidal volumes as compared with traditional tidal volumes for acute lung injury and the acute respiratory distress syndrome. N Engl J Med. (2000) 342:1301–1308. doi: 10.1056/NEJM200005043421801
32. Staboulidou I, Wüstemann M, Schmidt P, Günter HH, Hillemanns P, Scharf A. Transcranial Doppler pulsatility index: what it is and what it isn't. Neurocrit Care. (2012) 17:58–66. doi: 10.1007/s12028-012-9672-6
33. Bellner J, Romner B, Reinstrup P, Kristiansson KA, Ryding E, Brandt L. Transcranial Doppler sonography pulsatility index (PI) reflects intracranial pressure (ICP). Surg Neurol. (2004) 62:45–51. doi: 10.1016/j.surneu.2003.12.007
34. Shen J, Xue Y, Zhang Y, Wang Q. The application of transcranial Doppler in detecting diabetic cerebral macroangiopathy and microangiopathy. Zhonghua Nei Ke Za Zhi. (2002) 41:172–174.
35. Lee KY, Sohn YH, Baik JS, Kim GW, Kim JS. Arterial pulsatility as an index of cerebral microangiopathy in diabetes. Stroke. (2000) 31:1111–5. doi: 10.1161/01.STR.31.5.1111
36. Crespo-Cuevas AM, Canento T, Hernández-Perez M, Cáceres C, González A, Ispierto L, et al. The Barcelona-Asymptomatic Intracranial Atherosclerosis (AsIA) study: subclinical cervico-cerebral stenosis and middle cerebral artery pulsatility index as predictors of long-term incident cognitive impairment. Atherosclerosis. (2020) 312:104–9. doi: 10.1016/j.atherosclerosis.2020.08.025
37. Consensus Group on Transcranial Doppler in Diagnosis of Brain Death. Latin American consensus on the use of transcranial Doppler in the diagnosis of brain death. Rev Bras Ter Intensiva. (2014) 26:240–252. doi: 10.5935/0103-507X.20140035
38. Behrens A, Lenfeldt N, Ambarki K, Malm J, Eklund A, Koskinen LO. Transcranial Doppler pulsatility index: not an accurate method to assess intracranial pressure. Neurosurgery. (2010) 66:1050–7. doi: 10.1227/01.NEU.0000369519.35932.F2
39. de Azevedo DS, Salinet AS, de Lima Oliveira M, Teixeira MJ, Bor-Seng-Shu E, de Carvalho Nogueira R. Cerebral hemodynamics in sepsis assessed by transcranial Doppler: a systematic review and meta-analysis. J Clin Monit Comput. (2017) 31:1123–32. doi: 10.1007/s10877-016-9945-2
40. Battaglini D, Santori G, Chandraptham K, Iannuzzi F, Bastianello M, Tarantino F, et al. Neurological complications and noninvasive multimodal neuromonitoring in critically ill mechanically ventilated COVID-19 patients. Front Neurol. (2020) 11:602114. doi: 10.3389/fneur.2020.602114
41. Battaglini D, Premraj L, Huth S, Fanning J, Whitman G, Arora RC, et al. The use of noninvasive multimodal neuromonitoring in adult critically ill patients with COVID-19 infection. J Neurosurg Anesthesiol. (2023) 35:423. doi: 10.1097/ANA.0000000000000859
Keywords: COVID-19, ultrasonography, Doppler, transcranial, optic nerve, intracranial hypertension, mortality
Citation: Heredia-Orbegoso O, Vences MA, Failoc-Rojas VE, Fernández-Merjildo D, Lainez-Chacon RH and Villamonte R (2024) Cerebral hemodynamics and optic nerve sheath diameter acquired via neurosonology in critical patients with severe coronavirus disease: experience of a national referral hospital in Peru. Front. Neurol. 15:1340749. doi: 10.3389/fneur.2024.1340749
Received: 04 December 2023; Accepted: 15 April 2024;
Published: 03 May 2024.
Edited by:
Anselmo Caricato, Agostino Gemelli University Polyclinic (IRCCS), ItalyReviewed by:
Veena Raghunathan, Amrita Vishwa Vidyapeetham University, IndiaCopyright © 2024 Heredia-Orbegoso, Vences, Failoc-Rojas, Fernández-Merjildo, Lainez-Chacon and Villamonte. This is an open-access article distributed under the terms of the Creative Commons Attribution License (CC BY). The use, distribution or reproduction in other forums is permitted, provided the original author(s) and the copyright owner(s) are credited and that the original publication in this journal is cited, in accordance with accepted academic practice. No use, distribution or reproduction is permitted which does not comply with these terms.
*Correspondence: Miguel A. Vences, bWF2ZW5jZXNAdWN2dmlydHVhbC5lZHUucGU=
†These authors have contributed equally to this work
Disclaimer: All claims expressed in this article are solely those of the authors and do not necessarily represent those of their affiliated organizations, or those of the publisher, the editors and the reviewers. Any product that may be evaluated in this article or claim that may be made by its manufacturer is not guaranteed or endorsed by the publisher.
Research integrity at Frontiers
Learn more about the work of our research integrity team to safeguard the quality of each article we publish.