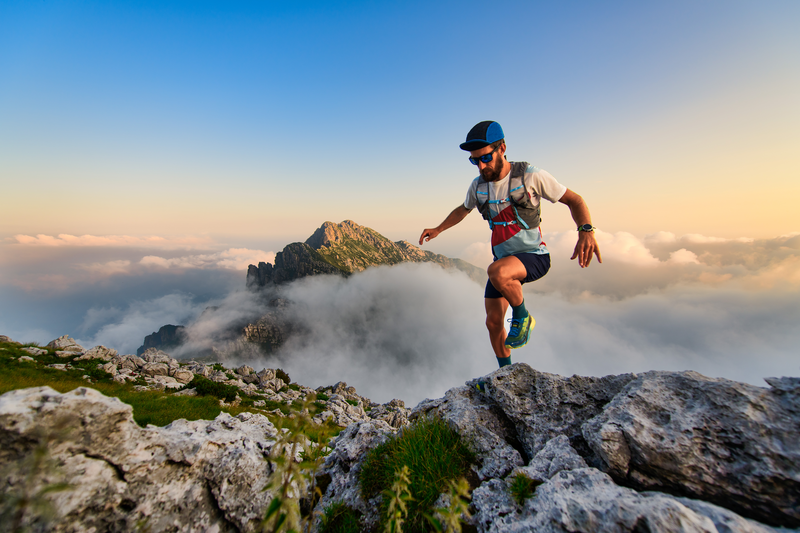
94% of researchers rate our articles as excellent or good
Learn more about the work of our research integrity team to safeguard the quality of each article we publish.
Find out more
MINI REVIEW article
Front. Neurol. , 04 January 2024
Sec. Sleep Disorders
Volume 14 - 2023 | https://doi.org/10.3389/fneur.2023.1323454
This article is part of the Research Topic Reviews in: Sleep Disorders View all 13 articles
Synucleinopathies are a group of neurodegenerative diseases characterized by abnormal accumulations of insoluble alpha-synuclein in neurons or glial cells. These consist of Parkinson's disease (PD), dementia with Lewy bodies (DLB), and multiple system atrophy (MSA). Moreover, idiopathic REM sleep behavior disorder (iRBD) is often the first manifestation of synucleinopathies, demonstrating a pathophysiological continuum. While these disorders vary in prevalence, symptom patterns, and severity, they can all include autonomic nervous system (ANS) dysfunction, which significantly reduces quality of life and worsens prognosis. Consequently, identifying abnormalities of the ANS can provide opportunities for improving quality of life through symptomatic treatments that are tailored to the individual's symptoms. An exciting development is using heart rate variability (HRV) as a non-invasive research tool for analyzing how the ANS regulates physiological processes. HRV during sleep, however, may provide a more accurate and reliable measure of ANS activity than during wakefulness, as during awake time, ANS activity is influenced by a variety of factors, including physical activity, stress, and emotions, which may mask or confound the underlying patterns of ANS activity. This review aims to provide an overview of the current knowledge regarding sleep-related HRV in synucleinopathies and to discuss contributing mechanisms. Evidence suggests that iRBD, PD, and MSA are associated with nocturnal ANS dysfunction. Further, comparative studies indicate that the presence of RBD could exacerbate this abnormality. In contrast, no studies have been conducted in patients with DLB. Overall, this review provides new insight into the complex interplay between the ANS and synucleinopathies and underscores the need for further research in this area to develop effective therapies to improve sleep and overall quality of life in patients with synucleinopathies.
Synucleinopathies are a group of neurodegenerative disorders characterized by abnormal aggregation of alpha-synuclein protein in the brain. These conditions include Parkinson's disease (PD), multiple system atrophy (MSA), and dementia with Lewy bodies (DLB). Rapid eye movement (REM) sleep behavior disorder (RBD) is an early manifestation of synucleinopathies (1–4). Sleep disorders including RBD are common in synucleinopathies, and can significantly impact quality of life (5, 6). In PD, RBD prevalence ranges from 35% to 50%, while in MSA it can be as high as 90% (7–9). Currently, however, there is no evidence that juvenile-onset RBD, such as would be associated with narcolepsy, is associated with synucleinopathies. Due to the lack of non-invasive biomarkers, there are no good treatment options, resulting in worsened quality of life (5).
During sleep, heart rate variability (HRV) serves as an objective, non-invasive assessment of autonomic nervous system (ANS) function in people with neurodegenerative disorders (10). HRV reflects beat-to-beat heart rate variation and is regulated by the ANS. Sleep stages and circadian rhythms influence HRV. For example, the parasympathetic nervous system (PNS) dominates during non-REM (NREM) sleep, particularly during deep sleep (slow-wave sleep), resulting in increased HRV (11). Conversely, during REM sleep, the sympathetic nervous system dominates, reducing HRV (12). Furthermore, circadian factors influence HRV, with HRV being highest during the nighttime and lowest during the daytime (13). Synucleinopathies reduce HRV during sleep, possibly related to the degeneration of brain regions controlling the ANS (14, 15). Thus, HRV analysis during sleep may provide insights into potential biomarkers, therapeutic interventions, underlying mechanisms of autonomic dysfunction, and early warning signs for disease progression and complications.
Sleep-related heart rate variability (HRV) can be analyzed in several ways, including time-domain, frequency-domain, and non-linear analyses (14). Time-domain analysis measures the variation in intervals between successive heartbeats. Commonly used time-domain measures of HRV during sleep, which provide general information about ANS activity, are the standard deviation of normal-to-normal (NN) intervals (SDNN), root mean square of successive differences (RMSSD), and percent of NN intervals that differ by more than 50 ms (pNN50). Frequency-domain analysis uses Fourier transform to convert time-domain signals into frequency components. The three standard frequency-domain measures of HRV during sleep are low-frequency power (LF; 0.04–0.15 Hz, proposed to represent combined sympathetic and parasympathetic influences and baroreflex modulation), high-frequency power (HF; 0.15–0.4 Hz, proposed to represent parasympathetic influences), and the ratio of LF to HF, which is thought to represent sympathovagal balance (16). Table 1 describes these and additional HRV measures. Non-linear analysis measures the complexity of heart rate signals using complex mathematical models. Non-linear measures of HRV during sleep include approximate entropy (ApEn), sample entropy (SampEn), and detrended fluctuation analysis (DFA). Despite non-linear analyses providing new insights into HRV, applications to clinical outcomes are unclear and therefore this analysis will not be discussed further in this review. Rather, this review will summarize available literature exploring HRV during sleep in patients with synucleinopathies including RBD (Table 2).
As previous meta-analysis (36) has synthesized differences in HRV between patients with PD, mostly during awake studies, this narrative review aims to provide an overview of the current knowledge concerning sleep-related HRV in synucleinopathies and discuss potential contributing mechanisms. The PubMed database was searched by using the keywords “Heart rate variability” AND “sleep,” AND “alpha synucleinopathies,” OR “Parkinson's disease,” OR “Alzheimer's disease,” OR “Rapid eye movement sleep behavior disorder,” OR “multiple system atrophy” OR “dementia with Lewy Bodies.” We selected only those articles that measured HRV during sleep in synucleinopathies based on the title and abstract.
RBD is characterized by REM sleep without atonia (RSWA) and dream-enactment behavior and is an early manifestation of synucleinopathies (37). RBD in individuals without a diagnosis of PD, DLB, or MSA is termed idiopathic RBD (iRBD), and termed symptomatic RBD in individuals with these diagnoses. Longitudinal cohort studies show that >80% of patients with iRBD diagnosed with a neurodegenerative disease over 12 years of follow up (3, 37, 38). Several case-control studies evaluated HRV comparing iRBD or RWSA to controls.
One case-control study showed that patients with iRBD had decreased cardiac autonomic function (35). The authors evaluated the first 5-min each of stages N2, REM, and wake recorded during video polysomnography (PSG). Compared to controls, the iRBD group showed reductions in SDNN, RMSSD, and HF values. Furthermore, quantified tonic EMG activity was inversely correlated with normalized LF values (LFnu) and LF/HF ratios and positively correlated with normalized HF values (HFnu). These results suggest that parasympathetic activity is disrupted in iRBD.
Another study (22) evaluated the ratio of R-R intervals preceding and following body movements during NREM and REM sleep in 14 patients with RBD compared to 14 matched controls. Notably, four of the RBD patients in this study had other neurodegenerative diseases (Alzheimer's disease, PD, or MSA). There was a decrease in the R-R interval ratio (reduced tachycardic response) related to body movements during REM sleep and NREM in RBD compared to controls. There were no differences between idiopathic RBD and symptomatic RBD.
One retrospective study evaluated 10 patients with iRBD compared to 10 matched controls (26). The expected increase in LF and LF/HF and the expected decrease in HF in the transition from NREM to REM sleep occurred only in controls but was absent in iRBD. Thus, the typical sympathetic predominance during REM sleep appears to be lost or decreased in iRBD.
One case-control study investigated individuals with iRWSA (21), finding no significant differences in HRV time or frequency domains during wakefulness or the first 5 min of the first N2 and last REM stages compared between 33 individuals with iRSWA and 28 controls (21). In addition, this study reported a non-significant reduction in NN during wake and NREM in individuals with iRSWA compared to controls. Taken together, case-control studies in iRBD suggest that there may be both sympathetic and parasympathetic dysfunction measurable during sleep through HRV. However, more research in larger cohorts is needed to fully understand sleep-related HRV changes in RBD.
The prevalence of ANS dysfunction in PD patients, which includes constipation, urinary dysfunction, erectile dysfunction, and orthostatic hypotension, is estimated to be between 50% and 70% (15). Because dysautonomia can occur during prodromal stages of PD, monitoring HRV parameters may aid in earlier diagnosis (15). Several case-control studies assessed sleep-related HRV parameters in both time and frequency domains in PD. The studies found that PD patients have altered cardiac autonomic function during the night or during sleep compared to controls.
Three studies evaluated 24-h ECG for HRV in PD and matched controls. One study (27) compared 13 PD and 13 controls and found significantly reduced SDNN, LF, and HF in PD compared to controls during nighttime hours. PD patients also had reduced SDNN and LF during the full 24-h period and during the day compared to controls. A larger study (23) evaluated 54 dopaminergic-naïve PD patients and 47 age-matched controls. Over 24-h, SDNN, VLF, LF, and HF were significantly lower in PD than controls. The night-time HRV was not analyzed separately, but was reported in another paper (30), showing lower night time LF and HF in PD compared to controls. This group also showed negative correlations between total and motor UPDRS and 24-h LF and VLF, but no relationship between HRV and disease duration (23). Additionally, patients with less bradykinesia had significantly higher 24-h HF. Another study evaluated 24-h HRV and included actigraphy to distinguish between time in and out of bed (28), finding that PD patients had lower HF when in bed, but higher HF when out of bed. The LF/HF ratio was lower (parasympathetic predominance) in patients than controls both in and out of bed. A limitation of these studies was the absence of EEG identification of sleep or wake.
PD patients also had altered HRV in response to body movements during REM sleep in a study that compared 26 untreated PD patients to 15 controls (22). This study found reduced tachycardia response to body movements during REM sleep in PD compared to controls.
In another study, 35 PD patients demonstrated lower sympathetic influence on HRV during both REM and NREM compared to 35 matched controls. No differences were found in time domain HRV parameters (RR interval and pN50) for REM or NREM. However, in the frequency domain, LFnu was lower and HFnu was higher in PD compared to controls, representing reduced sympathetic influence. Similarly, LF/HF was lower (parasympathetic predominance) in PD patients than controls for REM and NREM. Dopaminergic medications did not influence the HRV parameters (31). The authors attributed the findings to partial post-ganglionic noradrenergic cardiac denervation corresponding to observed blunted sympathetic responses during dream enactment behavior.
Palma et al. (16) evaluated HRV during REM and NREM (N1-N2 combined, and separately N3) sleep in 33 PD participants compared to 29 matched controls. Time and frequency domain HRV parameters were analyzed by averaging 10-min epochs across REM, N1-N2, N3, and wake. RR intervals were lower in PD compared to controls in wake, N3, and REM, with no differences in SDNN in any sleep stage. ULF was lower in N3, VLF and LF were lower during REM sleep, and HF was lower in N1-N2 in PD compared to controls. Furthermore, Unified Parkinson's Disease Rating Scale (UDPRS) part III ON and OFF scores were inversely related to VLF and LF during REM sleep. Similarly, Covassin et al. (20) found that lower HF (parasympathetic) HRV during REM sleep and higher LF/HF (sympathetic) during REM sleep were significantly correlated with disease severity in PD. These studies highlight the significance of cardiac autonomic dysfunction in PD and suggest utility in measuring disease severity.
Another study compared HRV and diffusion tensor imaging (DTI) in the medulla between 52 PD patients and 24 controls (29). PD patients did not demonstrate the expected sympathetic predominance during REM sleep, with significantly lower LF and higher HF compared to controls. In contrast to controls, there was no change from N3 to REM in either LF or HF. DTI measures in the medulla were negatively correlated with LF, HF, and LF/HF ratio in REM but not in N3, suggesting that neurodegeneration in the medulla influences loss of sympathovagal balance during REM sleep.
In addition to these case-control studies, Liu et al. (39) evaluated effects of subthalamic nucleus (STN) deep brain stimulation (DBS) on nighttime HRV in PD by comparing nights with DBS on vs. off. They found increased LF/HF ratio during the DBS-on night, suggesting that DBS may restore sympathetic regulation. However, a significant limitation of this study is that sleep and sleep stage were not confirmed during the recordings.
Thus, sleep-related HRV measures suggest alterations in sympathovagal balance and reduced sympathetic predominance in REM in people with PD. Additionally, these measures appear to be related to motor disease severity.
Multiple system atrophy (MSA) is a neurodegenerative disorder with glial cytoplasmic synuclein inclusions that results in autonomic dysfunction and Parkinsonism or cerebellar ataxia (19). Only two small case-control studies have evaluated HRV during sleep in MSA and another study compared HRV in MSA, PD, and controls.
One study compared seven MSA patients and seven controls, showing lower RR50, LF, and HF during sleep in MSA compared to controls (24). In controls, the LF/HF ratio was lower (parasympathetic predominance) during sleep than wake; however, this variation was absent in MSA. Another case-control sleep study showed increased HFnu in 7 MSA patients compared with 5 controls during REM and NREM 3/4 sleep (17). These findings suggest relative abnormal predominance of parasympathetic activity in MSA during REM sleep.
Brisinda et al. (19) evaluated HRV during 24-h ECG in PD, MSA, and controls showing lower LF and HF power in PD and MSA during sleep and awake activity, although PD participants had more impairment in LF/HF (parasympathetic predominance) during sleep and activity, while MSA patients had impaired LF/HF (parasympathetic predominance) only during daytime activity. However, the study is difficult to interpret because it was unclear how sleep was defined and no EEG was performed. Larger studies of sleep-related HRV are needed to explore autonomic dysfunction during sleep in MSA.
DLB is a synucleinopathy that manifests as progressive cognitive decline, cognitive and attentional fluctuations, visual hallucinations, RBD, and Parkinsonism. The neuronal synuclein deposits associated with DLB can affect various areas of the brain, including those that regulate the ANS. To the best of our knowledge, there have been no studies evaluating HRV during sleep in patients with DLB to date. In a study that evaluated 5-min EEG recordings of RR intervals during the day comparing DLB to Alzheimer's disease, lower values were found in DLB for most HRV parameters, including SDNN, pNN50, RMSSD, VLF, LF, HF, and total power (25). These findings suggest autonomic dysfunction in DLB and the authors proposed that HRV evaluation may be one way to distinguish between these two causes of dementia.
Cardiac autonomic dysfunction is associated with both iRBD and PD. However, whether ANS dysfunction is specific to RBD or if PD patients without RBD also demonstrate this dysfunction remains unclear.
One study (32) found attenuated heart rate responses (HRR) to both leg movements and arousals during both REM and NREM in patients with iRBD, PD-RBD, and PD-noRBD compared to controls, but no difference between PD patients with and without RBD. Sub-analysis showed that the attenuated HRR was not caused by dopaminergic medications. The most pronounced attenuation was found in PD patients, with iRBD having findings intermediate between PD and controls. These changes may be related to degeneration of cortical and subcortical regions, which are thought to be involved in modulating sleep EEG, HRR, and motor activity (40). As iRBD often precedes PD, the ANS changes may be early manifestations of brainstem neurodegeneration. HRV parameters during wakefulness and during consolidated sleep (without arousals) were also evaluated in this cohort, showing no differences in LF, HF, or LF/HF during N2 or REM between controls, iRBD, PD with RBD, and PD without RBD (33).
Another study (34) conducted 24-h ambulatory ECG recordings to elucidate whether RBD might influence circadian cardiac autonomic activity. They found increased nocturnal (10 pm to 6 am) LF and HF in PD-RBD compared to patients with PD without RBD. Further, there was no difference between daytime and nighttime LF and HF in PD without RBD, but these HRV parameters were higher at night compared to daytime in PD-RBD. These findings are unexpected based on other studies finding suppression of these measures during sleep in RBD. Because EEG was not recorded in this study, it is possible that differences between the groups in terms of percentages of sleep stages and time spent awake during the night may have been responsible for these unexpected results.
These studies demonstrate the importance of identifying sleep stages when evaluating nocturnal autonomic fluctuations, although such methods have shown mixed results when evaluating the influence of RBD on ANS in PD patients. One study (33) found no frequency domain HRV differences during sleep between PD with and without RBD. However, Bugalho et al. (18) found significant attenuation of parasympathetic HRV values (HF) in all stages of sleep in RBD (iRBD and PD-RBD) compared to PD without RBD. Further, there was a blunting of the expected increase of HF in NREM. Divergent results in these studies may be related to demographics in that participants had a higher mean age in the Bugalho study. Additionally, the Bugalho study included both iRBD and PD-RBD in the RBD group and compared to PD without RBD, while the other study compared the groups to a control group. Further study is needed to definitely determine the influence of RBD on HRV in PD.
This review summarizes available literature investigating sleep-related HRV in synucleinopathies, demonstrating nocturnal ANS dysfunction in these individuals. Studying HRV during sleep may provide clues as to potential biomarkers or therapeutic targets for these disorders. Figure 1 summarizes the key findings of the paper.
Many questions remain regarding the pathophysiological mechanisms of ANS dysfunction in iRBD and PD. However, neuropathological and imaging studies conducted in iRBD patients demonstrate abnormalities in several brainstem areas (41) that are important to autonomic network function of the central nervous system (CNS) (26). In PD, deposition of alpha-synuclein in both the PNS and CNS and post-ganglionic neuronal degeneration may lead to autonomic dysfunction (15). According to the Braak hypothesis (42), since iRBD often precedes PD clinical manifestations (8), the change in autonomic function may be an early manifestation of neurodegeneration in the brainstem.
There are also studies assessing whether ANS dysfunction is predominantly related to RBD or also influenced by PD pathology. There was a marked reduction in cardiac 123I-labeled meta-iodobenzylguanidine scintigraphy (a physiological norepinephrine analog used to assess sympathetic autonomic function) in patients with iRBD in the same range as PD (43, 44). However, another study found that the degree of MIBG dysfunction in iRBD was intermediately reduced between controls and patients with PD (33, 45). Using HRV as a marker, one study (34) found an increase in nocturnal sympathetic and parasympathetic activity in PD-RBD patients compared to PD-noRBD patients. Because the study lacked EEG sleep staging, it is difficult to speculate whether or how RBD may increase nocturnal ANS drive. ANS alterations could result from dream enactment behavior or microawakenings, or from cardiac nonadrenergic fiber involvement. Another proposed mechanism is spread of alpha-synuclein to pontomedullary structures, particularly the sublaterodorsal nucleus and subcoerulus complex (46). Additionally, the dorsal motor nucleus of the vagus, the nucleus solitarius, and the rostral ventrolateral medulla may be affected during the early disease stages, causing parasympathetic nervous system dysfunction (46). Future prospective studies are needed to clarify these conflicting results.
A few small studies suggest that ANS dysfunction occurs during sleep in MSA. Thus far, no studies have evaluated sleep-related HRV in DLB. More studies are needed to determine whether HRV may serve as an effective biomarker for earlier detection of MSA or DLB and provide further insight into the autonomic imbalance present in these disorders.
Although several case-control and/or retrospective studies of nocturnal HRV are available, much remains to be investigated. For example, longitudinal studies should examine how changes in sleep-related HRV impact health outcomes such as cognitive and motor decline in synuclein disorders. Additional areas of study should include wearable technology and sophisticated analytical methods. Moreover, HRV data from diverse populations should be collected to allow better understanding of normative values during sleep as well as the effects of age, sex, race/ethnicity, and comorbidities on HRV outcomes. Future research may identify clinical applications of HRV during sleep, such as its utility as a biomarker for various sleep or ANS disorders in synucleinopathies. In addition, interventional studies examining effects of sleep therapies such as cognitive-behavioral therapy, exercise, or pharmacological treatments on HRV during sleep could broaden treatment options for ANS dysfunction in neurodegenerative disorders. This review explored the nuances of HRV during sleep in synucleinopathies. Because this review is narrative, we did not follow PRISMA guidelines, and synthesizing evidence involves inherent subjectivity and potential for bias.
HRV during sleep is a non-invasive measure that can inform our understanding of ANS dysfunction in synuclein disorders. Available research in iRBD, PD, and MSA demonstrate alterations in parasympathetic and sympathetic function as well as sympathovagal balance. Additional work is needed to determine if sleep-related HRV can be used as a biomarker of early disease identification, prognosis, or progression and if guided therapies targeting ANS dysfunction in sleep could improve patient outcomes and quality of life.
AM: Conceptualization, Methodology, Writing – original draft, Writing – review & editing. EG: Data curation, Methodology, Writing – review & editing. TN: Data curation, Methodology, Writing – review & editing. YS: Data curation, Methodology, Writing – review & editing. CC: Visualization, Writing – review & editing. AA: Conceptualization, Methodology, Supervision, Writing – review & editing.
The author(s) declare that no financial support was received for the research, authorship, and/or publication of this article.
The authors declare that the research was conducted in the absence of any commercial or financial relationships that could be construed as a potential conflict of interest.
All claims expressed in this article are solely those of the authors and do not necessarily represent those of their affiliated organizations, or those of the publisher, the editors and the reviewers. Any product that may be evaluated in this article, or claim that may be made by its manufacturer, is not guaranteed or endorsed by the publisher.
1. Iranzo A, Santamaria J, Tolosa E. The clinical and pathophysiological relevance of REM sleep behavior disorder in neurodegenerative diseases. Sleep Med Rev. (2009) 13:385–401. doi: 10.1016/j.smrv.2008.11.003
2. Postuma RB, Iranzo A, Hogl B, Arnulf I, Ferini-Strambi L, Manni R, et al. Risk factors for neurodegeneration in idiopathic rapid eye movement sleep behavior disorder: a multicenter study. Ann Neurol. (2015) 77:830–9. doi: 10.1002/ana.24385
3. Schenck CH, Montplaisir JY, Frauscher B, Hogl B, Gagnon JF, Postuma R, et al. Rapid eye movement sleep behavior disorder: devising controlled active treatment studies for symptomatic and neuroprotective therapy–a consensus statement from the International rapid eye movement sleep behavior disorder study group. Sleep Med. (2013) 14:795–806. doi: 10.1016/j.sleep.2013.02.016
4. Claassen DO, Josephs KA, Ahlskog JE, Silber MH, Tippmann-Peikert M, Boeve BF, et al. REM sleep behavior disorder preceding other aspects of synucleinopathies by up to half a century. Neurology. (2010) 75:494–9. doi: 10.1212/WNL.0b013e3181ec7fac
5. Bailey GA, Hubbard EK, Fasano A, Tijssen MA, Lynch T, Anderson KN, et al. Sleep disturbance in movement disorders: insights, treatments and challenges. J Neurol Neurosurg Psychiatr. (2021) 92:723–36. doi: 10.1136/jnnp-2020-325546
6. Iranzo A, Molinuevo JL, Santamaría J, Serradell M, Martí MJ, Valldeoriola F, et al. Rapid-eye-movement sleep behaviour disorder as an early marker for a neurodegenerative disorder: a descriptive study. Lancet Neurol. (2006) 5:572–7. doi: 10.1016/S1474-4422(06)70476-8
7. Baumann-Vogel H, Hor H, Poryazova R, Valko P, Werth E, Baumann CR, et al. sleep behavior in Parkinson disease: frequent, particularly with higher age. PLoS One. (2020) 15:e0243454. doi: 10.1371/journal.pone.0243454
8. Postuma RB, Gagnon JF, Vendette M, Fantini ML, Massicotte-Marquez J, Montplaisir J. Quantifying the risk of neurodegenerative disease in idiopathic REM sleep behavior disorder. Neurology. (2009) 72:1296–300. doi: 10.1212/01.wnl.0000340980.19702.6e
9. Palma J-A, Fernandez-Cordon C, Coon EA, Low PA, Miglis MG, Jaradeh S, et al. Prevalence of REM sleep behavior disorder in multiple system atrophy: a multicenter study and meta-analysis. Clin Auton Res. (2015) 25:69–75. doi: 10.1007/s10286-015-0279-9
10. Kerkering EM, Greenlund IM, Bigalke JA, Migliaccio GCL, Smoot CA, Carter JR. Reliability of heart rate variability during stable and disrupted polysomnographic sleep. Am J Physiol Heart Circ Physiol. (2022) 323:H16–23. doi: 10.1152/ajpheart.00143.2022
11. de Zambotti M, Trinder J, Silvani A, Colrain IM, Baker FC. Dynamic coupling between the central and autonomic nervous systems during sleep: a review. Neurosci Biobehav Rev. (2018) 90:84–103. doi: 10.1016/j.neubiorev.2018.03.027
12. Somers VK, Dyken ME, Mark AL, Abboud FM. Sympathetic-nerve activity during sleep in normal subjects. N Engl J Med. (1993) 328:303–7. doi: 10.1056/NEJM199302043280502
13. Boudreau P, Yeh W-H, Dumont GA, Boivin DB. Circadian variation of heart rate variability across sleep stages. Sleep. (2013) 36:1919–28. doi: 10.5665/sleep.3230
14. Tobaldini E, Nobili L, Strada S, Casali KR, Braghiroli A, Montano N. Heart rate variability in normal and pathological sleep. Front Physiol. (2013) 4:294. doi: 10.3389/fphys.2013.00294
15. Mendoza-Velásquez JJ, Flores-Vázquez JF, Barrón-Velázquez E, Sosa-Ortiz AL, Illigens B-MW, Siepmann T. Autonomic dysfunction in α-synucleinopathies. Front Neurol. (2019) 10:363. doi: 10.3389/fneur.2019.00363
16. Palma J-A, Urrestarazu E, Alegre M, Pastor MA, Valencia M, Artieda J, et al. Cardiac autonomic impairment during sleep is linked with disease severity in Parkinson's disease. Clin Neurophysiol. (2013) 124:1163–8. doi: 10.1016/j.clinph.2012.12.042
17. Abele M, Klockgether T, Wüllner U. Spectral analysis of heart rate variability in multiple system atrophy and unexplained sporadic ataxia. J Neurol. (2004) 251:7. doi: 10.1007/s00415-004-0460-x
18. Bugalho P, Mendonça M, Lampreia T, Miguel R, Barbosa R, Salavisa M. Heart rate variability in Parkinson disease and idiopathic REM sleep behavior disorder. Clin Auton Res. (2018) 28:557–64. doi: 10.1007/s10286-018-0557-4
19. Brisinda D, Sorbo AR, Di Giacopo R, Venuti A, Bentivoglio AR, Fenici R. Cardiovascular autonomic nervous system evaluation in Parkinson disease and multiple system atrophy. J Neurol Sci. (2014) 336:197–202. doi: 10.1016/j.jns.2013.10.039
20. Covassin N, Neikrug AB, Liu L, Maglione J, Natarajan L, Corey-Bloom J, et al. Relationships between clinical characteristics and nocturnal cardiac autonomic activity in Parkinson's disease. Auton Neurosci. (2012) 171:85–8. doi: 10.1016/j.autneu.2012.10.003
21. Dijkstra F, Viaene M, De Volder I, Fransen E, Cras P, Crosiers D. Polysomnographic phenotype of isolated REM sleep without atonia. Clin Neurophysiol. (2020) 131:2508–15. doi: 10.1016/j.clinph.2020.07.005
22. Ferini-Strambi L, Smirne S. Cardiac autonomic function during sleep in several neuropsychiatric disorders. J Neurol. (1997) 244:S29–36. doi: 10.1007/BF03160569
23. Haapaniemi TH, Pursiainen V, Korpelainen JT, Huikuri HV, Sotaniemi KA, Myllylä VV. Ambulatory ECG and analysis of heart rate variability in Parkinson's disease. J Neurol Neurosurg Psychiatry. (2001) 70:305–10. doi: 10.1136/jnnp.70.3.305
24. Kitae S, Murata Y, Tachiki N, Okazaki M, Harada T, Nakamura S. Assessment of cardiovascular autonomic dysfunction in multiple system atrophy. Clin Auton Res. (2001) 11:39–44. doi: 10.1007/BF02317801
25. Kasanuki K, Iseki E, Fujishiro H, Ando S, Sugiyama H, Kitazawa M, et al. Impaired heart rate variability in patients with dementia with Lewy bodies: Efficacy of electrocardiogram as a supporting diagnostic marker. Parkinsonism Relat Disord. (2015) 21:749–54. doi: 10.1016/j.parkreldis.2015.04.024
26. Lanfranchi PA, Fradette L, Gagnon J-F, Colombo R, Montplaisir J. Cardiac autonomic regulation during sleep in idiopathic REM sleep behavior disorder. Sleep. (2007) 30:1019–25. doi: 10.1093/sleep/30.8.1019
27. Mastrocola C, Vanacore N, Giovani A, Locuratolo N, Vella C, Alessandri A, et al. Twenty-four-hour heart rate variability to assess autonomic function in Parkinson's disease. Acta Neurol Scand. (1999) 99:245–7. doi: 10.1111/j.1600-0404.1999.tb07355.x
28. Niwa F, Kuriyama N, Nakagawa M, Imanishi J. Circadian rhythm of rest activity and autonomic nervous system activity at different stages in Parkinson's disease. Auton Neurosci. (2011) 165:195–200. doi: 10.1016/j.autneu.2011.07.010
29. Pyatigorskaya N, Mongin M, Valabregue R, Yahia-Cherif L, Ewenczyk C, Poupon C, et al. Medulla oblongata damage and cardiac autonomic dysfunction in Parkinson disease. Neurology. (2016) 87:2540–5. doi: 10.1212/WNL.0000000000003426
30. Pursiainen V, Haapaniemi TH, Korpelainen JT, Huikuri HV, Sotaniemi KA, Myllylä VV. Circadian heart rate variability in Parkinson's disease. J Neurol. (2002) 249:1535–40. doi: 10.1007/s00415-002-0884-0
31. Sauvageot N, Vaillant M, Diederich NJ. Reduced sympathetically driven heart rate variability during sleep in Parkinson's disease: a case-control polysomnography-based study. Mov Disord. (2011) 26:234–40. doi: 10.1002/mds.23479
32. Sorensen GL, Kempfner J, Zoetmulder M, Sorensen HBD, Jennum P. Attenuated heart rate response in REM sleep behavior disorder and Parkinson's disease. Mov Disord. (2012) 27:888–94. doi: 10.1002/mds.25012
33. Sorensen GL, Mehlsen J, Jennum P. Reduced sympathetic activity in idiopathic rapid-eye-movement sleep behavior disorder and Parkinson's disease. Auton Neurosci. (2013) 179:138–41. doi: 10.1016/j.autneu.2013.08.067
34. Salsone M, Vescio B, Fratto A, Sturniolo M, Arabia G, Gambardella A, et al. Cardiac sympathetic index identifies patients with Parkinson's disease and REM behavior disorder. Parkinsonism Relat Disord. (2016) 26:62–6. doi: 10.1016/j.parkreldis.2016.03.004
35. Yang JH, Choi SH, Lee MH, Oh SM, Choi J-W, Park JE, et al. Association of heart rate variability with REM sleep without atonia in idiopathic REM sleep behavior disorder. J Clin Sleep Med. (2021) 17:461–9. doi: 10.5664/jcsm.8934
36. Heimrich KG, Lehmann T, Schlattmann P, Prell T. Heart rate variability analyses in Parkinson's disease: a systematic review and meta-analysis. Brain Sci. (2021) 11:8. doi: 10.3390/brainsci11080959
37. Postuma RB, Iranzo A, Hu M, Högl B, Boeve BF, Manni R, et al. Risk and predictors of dementia and parkinsonism in idiopathic REM sleep behaviour disorder: a multicentre study. Brain. (2019) 142:744–59. doi: 10.1093/brain/awz030
38. Iranzo A, Fernández-Arcos A, Tolosa E, Serradell M, Molinuevo JL, Valldeoriola F, et al. Neurodegenerative disorder risk in idiopathic REM sleep behavior disorder: study in 174 patients. PLoS ONE. (2014) 9:e89741. doi: 10.1371/journal.pone.0089741
39. Liu K-D, Shan D-E, Kuo TBJ, Yang CCH. The effects of bilateral stimulation of the subthalamic nucleus on heart rate variability in patients with Parkinson's disease. J Neurol. (2013) 260:1714–23. doi: 10.1007/s00415-013-6849-7
40. Ferri R, Zucconi M, Rundo F, Spruyt K, Manconi M, Ferini-Strambi L. Heart rate and spectral EEG changes accompanying periodic and non-periodic leg movements during sleep. Clin Neurophysiol. (2007) 118:438–48. doi: 10.1016/j.clinph.2006.10.007
41. Gagnon J-F, Postuma RB, Mazza S, Doyon J, Montplaisir J. Rapid-eye-movement sleep behaviour disorder and neurodegenerative diseases. Lancet Neurol. (2006) 5:424–32. doi: 10.1016/S1474-4422(06)70441-0
42. Braak H, Del Tredici K, Rüb U, de Vos RAI, Jansen Steur ENH, Braak E. Staging of brain pathology related to sporadic Parkinson's disease. Neurobiol Aging. (2003) 24:197–211. doi: 10.1016/S0197-4580(02)00065-9
43. Miyamoto T, Miyamoto M, Suzuki K, Nishibayashi M, Iwanami M, Hirata K. 123I-MIBG cardiac scintigraphy provides clues to the underlying neurodegenerative disorder in idiopathic REM sleep behavior disorder. Sleep. (2008) 31:717–23. doi: 10.1093/sleep/31.5.717
44. Miyamoto T, Miyamoto M, Inoue Y, Usui Y, Suzuki K, Hirata K. Reduced cardiac 123I-MIBG scintigraphy in idiopathic REM sleep behavior disorder. Neurology. (2006) 67:2236–8. doi: 10.1212/01.wnl.0000249313.25627.2e
45. Postuma RB, Gagnon J-F, Pelletier A, Montplaisir J. Prodromal autonomic symptoms and signs in Parkinson's disease and dementia with Lewy bodies. Mov Disord. (2013) 28:597–604. doi: 10.1002/mds.25445
Keywords: heart rate variability (HRV), synucleinopathies, Parkinson's disease, sleep, Alzheimer's disease, Lewy body dementia, multiple system atrophy, rapid-eye-movement sleep behavior disorder (RBD)
Citation: Memon AA, George EB, Nazir T, Sunkara Y, Catiul C and Amara AW (2024) Heart rate variability during sleep in synucleinopathies: a review. Front. Neurol. 14:1323454. doi: 10.3389/fneur.2023.1323454
Received: 17 October 2023; Accepted: 11 December 2023;
Published: 04 January 2024.
Edited by:
Haitham Jahrami, Arabian Gulf University, BahrainReviewed by:
Keisuke Suzuki, Dokkyo Medical University, JapanCopyright © 2024 Memon, George, Nazir, Sunkara, Catiul and Amara. This is an open-access article distributed under the terms of the Creative Commons Attribution License (CC BY). The use, distribution or reproduction in other forums is permitted, provided the original author(s) and the copyright owner(s) are credited and that the original publication in this journal is cited, in accordance with accepted academic practice. No use, distribution or reproduction is permitted which does not comply with these terms.
*Correspondence: Amy W. Amara, YW15LmFtYXJhQGN1YW5zY2h1dHouZWR1
Disclaimer: All claims expressed in this article are solely those of the authors and do not necessarily represent those of their affiliated organizations, or those of the publisher, the editors and the reviewers. Any product that may be evaluated in this article or claim that may be made by its manufacturer is not guaranteed or endorsed by the publisher.
Research integrity at Frontiers
Learn more about the work of our research integrity team to safeguard the quality of each article we publish.