- 1Department of Otolaryngology, Jiaxing University Master Degree Cultivation Base, Zhejiang Chinese Medical University, Zhejiang, China
- 2Department of Otolaryngology, The First Hospital of Jiaxing, Jiaxing, China
The prevalence rate of allergic rhinitis (AR) is high worldwide. The inhalation of allergens induces AR, which is an immunoglobulin E-mediated and type 2 inflammation-driven disease. Recently, the role of neuroimmune communication in AR pathogenesis has piqued the interest of the scientific community. Various neuropeptides, such as substance P (SP), vasoactive intestinal peptide (VIP), calcitonin gene-related peptide (CGRP), nerve growth factor (NGF), and neuromedin U (NMU), released via “axon reflexes” or “central sensitization” exert regulatory effects on immune cells to elicit “neurogenic inflammation,” which contributes to nasal hyperresponsiveness (NHR) in AR. Additionally, neuropeptides can be produced in immune cells. The frequent colocalization of immune and neuronal cells at certain anatomical regions promotes the establishment of neuroimmune cell units, such as nerve-mast cells, nerve-type 2 innate lymphoid cells (ILC2s), nerve-eosinophils and nerve-basophils units. Receptors expressed both on immune cells and neurons, such as TRPV1, TRPA1, and Mas-related G protein-coupled receptor X2 (MRGPRX2) mediate AR pathogenesis. This review focused on elucidating the mechanisms underlying neuroimmune communication in AR.
1 Introduction
Allergic rhinitis (AR), a highly prevalent atopic disorder, is associated with immunoglobulin E (IgE) antibody-mediated immune responses (1). Globally, AR affects up to 40% of the human population. Recent studies have focused on the diagnosis, pathophysiological mechanisms, epidemiology, treatment, and comorbidities of AR. Previous studies have reported the correlation between AR and asthma. AR and asthma, which are characterized by similar inflammatory reactions, are associated with enhanced responses to various inhalants (2). Moreover, AR is an independent risk factor for the development of asthma. In particular, 40% of patients with AR have or will develop asthma. As the inflammatory responses in the upper and lower airways are similar and interconnected in AR and asthma, they can be considered “one airway, one disease” (3). The mutual effects of AR and asthma have been previously reported. Additionally, allergic conjunctivitis may also be associated with AR. Nasal symptoms, which are characterized by mucosal inflammation and hyperresponsiveness, may be induced by the hyperresponsiveness of the upper airways after allergen inhalation (2, 4–6).
The submucosa or subepithelial region of the nose comprises abundant immune cells that contribute to allergic inflammation, which is also regulated by neuroimmune-derived factors (7). The exposure to an allergen promotes the cross-linking of mucosal mast cell (MC) and basophil surface-bound IgE with its receptor and rapidly induces nasal symptoms in sensitized individuals through degranulation and the release of various chemical mediators, such as histamine, prostaglandin D2, and cysteinyl leukotrienes (5, 8, 9). Eosinophils in the nasal mucosa are also activated during this process. Nasal epithelial injury exposes nerve fibers and promotes the release of granule contents, which elicit T helper 2 cell (Th2) response, resulting in the recruitment of eosinophils, basophils, and T cells to sustain the inflammatory response (8). Th17 and regulatory T (Treg) cells, rather than the traditional Th1/Th2 balance paradigm, were recently reported to mediate AR pathogenesis (10). Substance P (SP) and calcitonin gene-related peptide (CGRP) regulate Th17/Treg differentiation (11, 12). These reactions result in vasodilation, increased vascular permeability, and mucus production, as well as the stimulation of sensory nerves, inducing typical symptoms, such as itching, sneezing, watery rhinorrhea, and nasal congestion and impairing the quality of life (QOL) (13). As neuronal activity is involved in the induction of these symptoms, AR can be characterized as a neuroimmune disorder.
Neuroimmunology, a biological science field, is an area of active research. Bidirectional interactions between the nervous system and the immune system involve the following four basic components: neuromediators secreted by the neurons; immune factors released by the immune cells; cytokines released by the neuroendocrine cells and neuropeptides released by the immune cells; common receptors expressed in the immune cells and neurons (14, 15). Recent studies have reported the role of neuroimmune interactions in the development of allergic diseases, such as atopic dermatitis, prurigo nodularis, AR, asthma, food allergy, chronic rhinosinusitis with nasal polyps, eosinophilic esophagitis, allergic rhinoconjunctivitis, and urticaria (16–20). Thus, the processes involved in neuroimmune communication must be elucidated to improve our understanding of AR pathogenesis.
This review summarizes neuroimmune communication in AR and highlights the role of the immune system-nervous system interaction in AR pathogenesis. The review of known neuroimmunomodulation mechanisms of AR provides a potential research direction for exploring therapeutic strategies to target neuroimmune interaction. Additionally, this review can serve as a theoretical foundation for the application of these therapeutic strategies and provide useful insights for the development of novel diagnostic and therapeutic strategies for AR.
2 Neurogenic inflammation and AR nasal hyperresponsiveness
2.1 The sensory neuron of nasal mucosa and reflex hyperresponsiveness
Nociceptors are specialized sensory neurons that detect and respond to noxious or potentially noxious stimuli, such as mechanical, chemical, or thermal stimuli. Additionally, nociceptors of afferent nerves are highly susceptible to stimulation during an acute allergic reaction (21). These nerve terminals in the human nasal cavity are the peripheral processes of the trigeminal ganglion-based primary sensory neurons (22). Generally, human nasal nociceptors are C-fibers, which are typically sensitive to chemical and physical stimulation (multimode) (22). These sensory endings express several receptors and ion channels, including transient receptor potential (TRP) channels (such as TRPV1 and TRPA1), G protein-coupled receptors (GPCRs), acid-sensing ion channels, mechano-sensitive channels, voltage-gated ion channels, and purinergic receptors, which convert environmental signals into electrical signals (22, 23).
NHR, which is observed in 60–70% of patients with AR, is associated with alterations in the following two types of innervating nasal nerves: afferent nerves (somatosensory system) and efferent nerves (sympathetic or parasympathetic motor system). Some nasal symptoms, such as sneezing, rhinorrhea, itching, or obstruction may develop in a hyperresponsive nasal cavity in response to various stimuli, such as cold dry air, capsaicin, hyperosmolar saline, and bradykinin with markedly enhanced nasal reflexes (1, 6, 22, 24). The application of afferent C-fiber activators to the nasal mucosa in animal models and in humans increases reflex hypersensitivity, markedly enhancing NHR (25). In addition to the nasal mucosa, reflex hypersensitivity also affects other areas of the airways in humans (26). However, not all NHR symptoms in patients are directly caused by neuronal malfunction, some of them may even be brought on by immune cells (22).
2.2 “Axon reflexes” and “central sensitization” associated with “neurogenic inflammation” of nasal hyperresponsiveness
Previous studies have demonstrated that various proinflammatory neuropeptides, including CGRP, SP, neuromedin U (NMU), nerve growth factor (NGF), and vasoactive intestinal peptide (VIP), are released by different nerve endings, especially sensory nerves in the nasal mucosa, in response to surrounding environmental stimuli. The exposure to allergens stimulates human C-fibers, resulting in a local peripheral reflex in sensitive individuals. The signal in this reflex is not integrated into the central nervous system (CNS) but is transduced via local axons (hence called “axon reflexes”). The action potentials generated in the peripheral sensory nerve endings are concentrated and transmitted until they reach the bifurcation, where they reverse back to other peripheral terminals of the same nerve. The afferent-efferent synapses of nasal mucosa promote neuropeptide release and immune cell recruitment, which can augment allergic inflammation. “Central sensitization” is mediated by integrating the reflex signal with the CNS (21, 27–31). Both these pathways can induce “neurogenic inflammation” and promote NHR by recruiting and activating immune cells (29, 32) (Figure 1). Nerves and type 2 cytokines, including interleukin (IL)-4, IL-5, and IL-13 can contribute to the neurological symptoms of AR, such as itching and sneezing. Type 2 cytokines induce neurological symptoms by activating sensory nerves. In addition, it has recently been discovered that thymic stromal lymphopoietin (TSLP) in mice binds to its receptor, TSLPR, activating TRPA1 (33). IL-31 produced by Th2 cells activates TRPV1+ TRPA1+ sensory nerves in animal models, inducing an MC-independent itching response (34–37). Additionally, IL-33 promotes itching through the receptor ST2 in peripheral TRPV1-expressing sensory neurons in mice. This is a histamine-independent way to activate Ca2+ influx into neurons (38, 39). Histamine upregulates mucin production in AR through the activation of cholinergic nerve terminals and cysteinyl leukotrienes (22).
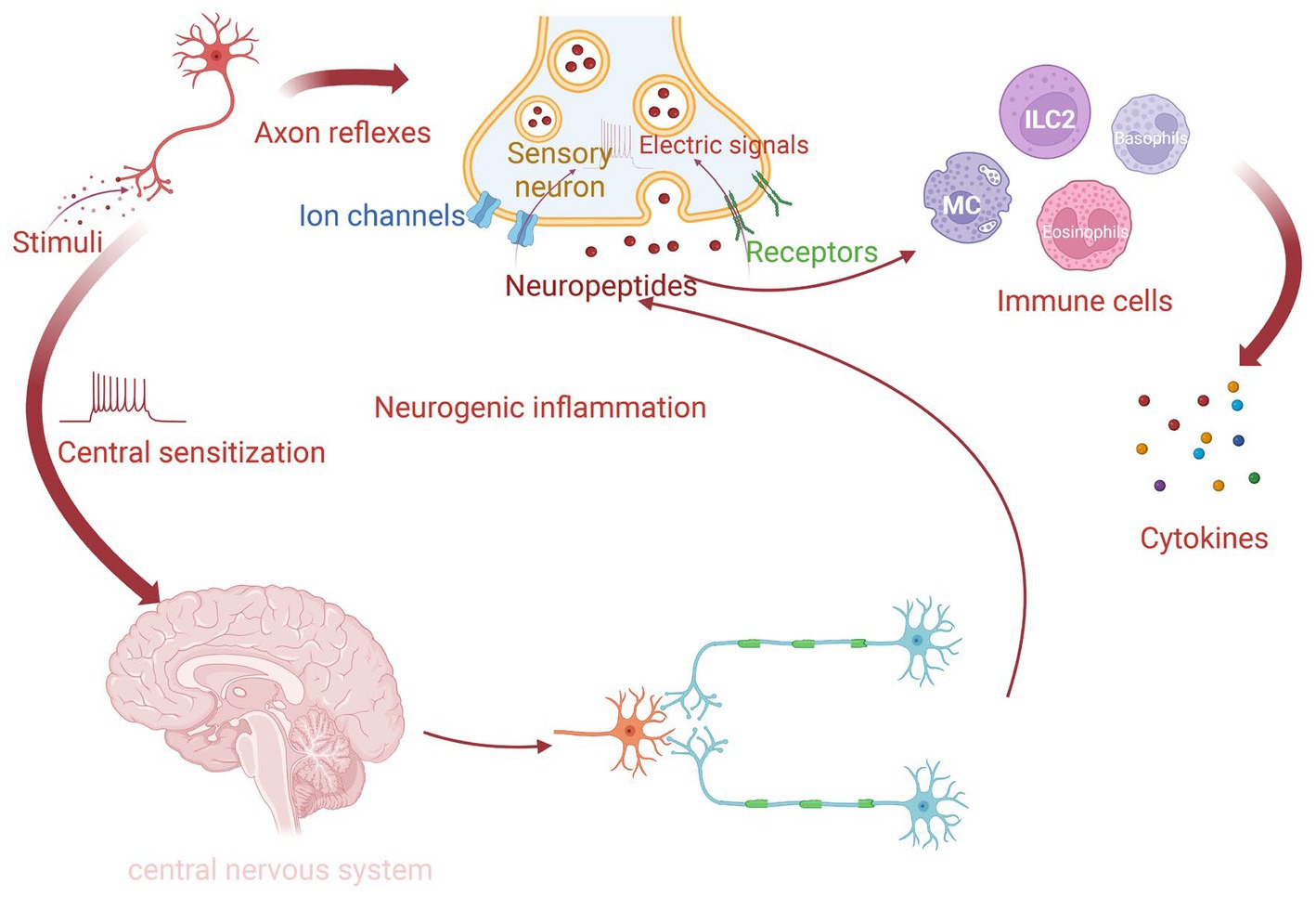
Figure 1. The process of neurogenic inflammation in AR. For sensitive individuals, when nasal nerve endings detect routine stimuli, they can transform environmental signals into electrical signals via various receptors and ion channels. There are two pathways to form neurogenic inflammation. One is called local “axon reflexes,” which releases neuropeptides through local afferent-efferent synapses independently. Another way is “central sensitization,” in which signals integrate in the central nervous system (CNS). The release of neuropeptides is facilitated by both of these mechanisms and then promotes different immune cells to produce cytokines.
2.3 Role of TRP in AR
Members of the ion channels known as the transient receptor potential (TRP) family, which link the nervous system with the immune system, are frequently expressed on afferent C-fibers. TRPV1 and TRPA1 mediate neurogenic and immunogenic inflammation associated with common respiratory illnesses, including AR. Additionally, TRPs are expressed on the surface of immune cells, such as MCs. In combination with activated MCs, activated TRPA1 and TRPV1 in mice mediate neuroimmune communication, inducing airway allergy symptoms through interaction with sensory nerves (35). The opening of the TRP channel promotes the influx of cations, such as sodium and calcium, generating action potentials and promoting the release of neuropeptides, such as SP and CGRP from the nasal mucosa to induce neurogenic inflammation. Additionally, TRP channels increase proinflammatory gene expression, vasodilation, capillary permeability, and plasma extravasation and regulate MC functions (35–37, 40, 41).
TRPA1, a nociceptive cationic (mostly Ca2+) thermo-responsive channel, is expressed in the trigeminal sensory neurons, which innervate the respiratory and nasal epithelia. The TRPA1 mRNA level is upregulated in the nasal mucosa of patients with AR. TRPA1 is involved in the perception of cold temperatures (<17°C) (40, 42, 43). Low temperatures are reported to activate sensory nerves and induce neurogenic inflammation. This can explain the reason for cold air-stimulated sneezing in patients with AR. In the MC line RBL2H3, TRPA1 interacts with the granulogenesis-related protein secretogranin III in vesicular structures. Prostaglandin D2 and its metabolites can gate TRPA1 and strongly activate C-fibers (44). Recent studies have demonstrated that the inhibition of TRPA1 with the pharmacological antagonist HC-030031 alleviates nasal hyperresponsiveness and allergic inflammation of the upper airways in the ovalbumin-induced AR mouse model. The underlying mechanism may involve the TRPA1-SP signaling pathway (43). Thus, the inhibition of TRPA1 is a potential therapeutic strategy for patients with AR.
Receptors of TRPV1, which is a receptor of capsaicin that is directly activated by thermal, chemical, and mechanical stimuli, are associated with histamine-dependent arachidonic acid metabolite-induced itching (45). The density of TRPV1 on the surface of sensory fibers was upregulated in patients with hyperresponsiveness. Additionally, TRPV1 is widely distributed in the submucosal glands, vascular endothelial cells, and nasal mucosa. Inflammatory mediators can activate TRPV1 in the airway mucosa to induce inflammation (41, 46). The proximity of MCs to the sensory nerve fibers expressing TRPV1 facilitates nerve-MCs interactions (47). In AR mice, TRPV1 can express on the surface of CD4+ T cells and TRPV1 knockout downregulates the levels of infiltrating eosinophils, Th2/Th17 cells in the nasal mucosa, and specific IgE in the serum. TRPV1 is associated with AR clinical symptoms, especially itching and sneezing (48). Acupuncture treatment alleviates the symptoms of AR mice by modulating TRPV1 expression (49). Treatment with the TRPV1 agonist capsaicin alleviates cold dry air-induced symptoms and hyperreactivity in humans (22, 50, 51). Further clinical trials are needed to verify the therapeutic effectiveness of capsaicin in AR mediated through the TRPV1-SP nociceptive signaling pathway. Additionally, Chinese medicines exert therapeutic effects on AR through TRPV1 (41), although the underlying mechanisms have not been elucidated.
3 Role of neuropeptides in neuroimmune communication in AR
Several immune cells express receptors for the neuropeptides released from nerves, including acetylcholine, NGF, catecholamines, SP, NMU, VIP, and CGRP, which exert regulatory effects on various immune cells (34, 52). Additionally, some neuropeptides are secreted from the immune cells in AR.
3.1 Substance P
SP, a member of the tachykinin family of neuropeptides, is upregulated in the CNS, peripheral nervous system, and the immune system, has been found to have dramatically elevated levels in AR, and primarily operates through the neurokinin 1 receptor (NK1R) (11, 53). The expression of NK1R is upregulated in patients with AR (54). NK2R and NK3R are also SP receptors, however, their affinity is much lower than that of NK1R (53, 55, 56). Recent research has shown that SP, in addition to the classical receptor NK-1R, stimulates the MRGPRX2 receptor (and its murine counterpart, MRGPRB2) to produce allergies (57–59). SP exerts regulatory effects on immune cells, inducing vasodilation, increasing vascular permeability, and stimulating the submucosal glands in patients with AR (60). The SP levels are upregulated in the nasal lavages of individuals with AR. Additionally, the number of SP-positive nerve fibers was upregulated in individuals with NHR, suggesting the regulation of SP during neurogenic inflammation (61). The expression of the capsaicin receptor TRPV1 on the surface of all SP-positive sensory neurons indicated the functional role of TRPV1 in SP synthesis and release (61). Acupuncture treatment markedly downregulated allergen-specific IgE and SP and alleviated symptoms, especially those that are mediated by TRPV1, such as eye itching, nasal itching, and sneezing (49, 62).
The therapeutic strategies for AR patients targeting the nerves include selective vidian neurectomy with amputation of the posterior nasal nerve and pharyngeal branch of the vidian nerve, vidian neurectomy, radiofrequency neurolysis of the posterior nasal nerve area, and acupuncture at the sphenopalatine ganglion (63–65). These therapies may target various nerves. However, all of these nerves are branches of the trigeminal nerve. The trigeminal ganglion’s sensory neuronal cell bodies and afferent nerve terminals are where SP is concentrated. The production of SP in trigeminal ganglion neurons (TGNs) after allergen stimulation was 500-fold higher than that in nasal epithelial cells, indicating the capacity of TGNs to synthesize SP (66).
The balance between Th17 and Treg cells may be crucial for the pathogenesis of AR (10). The differentiation of Th17 is closely associated with both IL-6 and tumor growth factor (TGF)-β. Treg cells are correlated with the IL-10 levels (67). SP regulates the expression of IL-6 and TNF-α in MCs through the nuclear factor (NF)-κB pathway or the MyD88 pathway. The levels of SP are positively correlated with those of IL-6 and TNF-α but negatively correlated with those of IL-10 (11). Future studies must elucidate the underlying mechanism. SP is reported to promote dendritic cell (DC) migration through MRGPRA1. TRPV1+ sensory neurons and SP-driven DC migration are druggable pathways in type-2 immune response (68). NK1R-signaling is critical for efficient Ca2+ flux in T cell receptor-activated T cells. The deficiency or the pharmacological inhibition of NK1R on the surface of T cells downregulated Ca2+ flux (69). Additionally, SP can directly promote Treg dysfunction and induce the release of IL-17 by human memory-phenotype T cells through NK1R. These effects are successfully eliminated when SP-NK1R signaling is blocked in mice (70). These findings indicate that SP-mediated Th17/Treg differentiation induces AR.
In addition to nerve cells, MCs and eosinophils can synthesize and release SP. SP promotes TNF-α secretion from MCs. Additionally, the SP-induced Ca2+ influx affects the degranulation mechanism of MCs. Furthermore, SP induces histamine release from MCs (71, 72) (Figure 2A). McCary reported that SP can downregulate the expression of the high-affinity IgE receptor (FcεRI) mRNA and protein on the surface of human MCs, which in turn limits MC activation (73). This may contradict the ability of SP to activate MCs or indicate the presence of an SP-mediated non-IgE-dependent MC activation mechanism. Recent studies on non-IgE-mediated allergy reactions have revealed the role of SP in MC activation (59, 74–76). SP elicits inflammatory responses through MRGPRX2, challenging our previous understanding of the SP/NK1R axis (74). The intricate mechanism must be further investigated. Additionally, Sch 50971, a potent and highly selective H3 receptor agonist, alleviates nasal allergy symptoms in mice by inhibiting the production of SP through ATP-sensitive K+ channels (77).
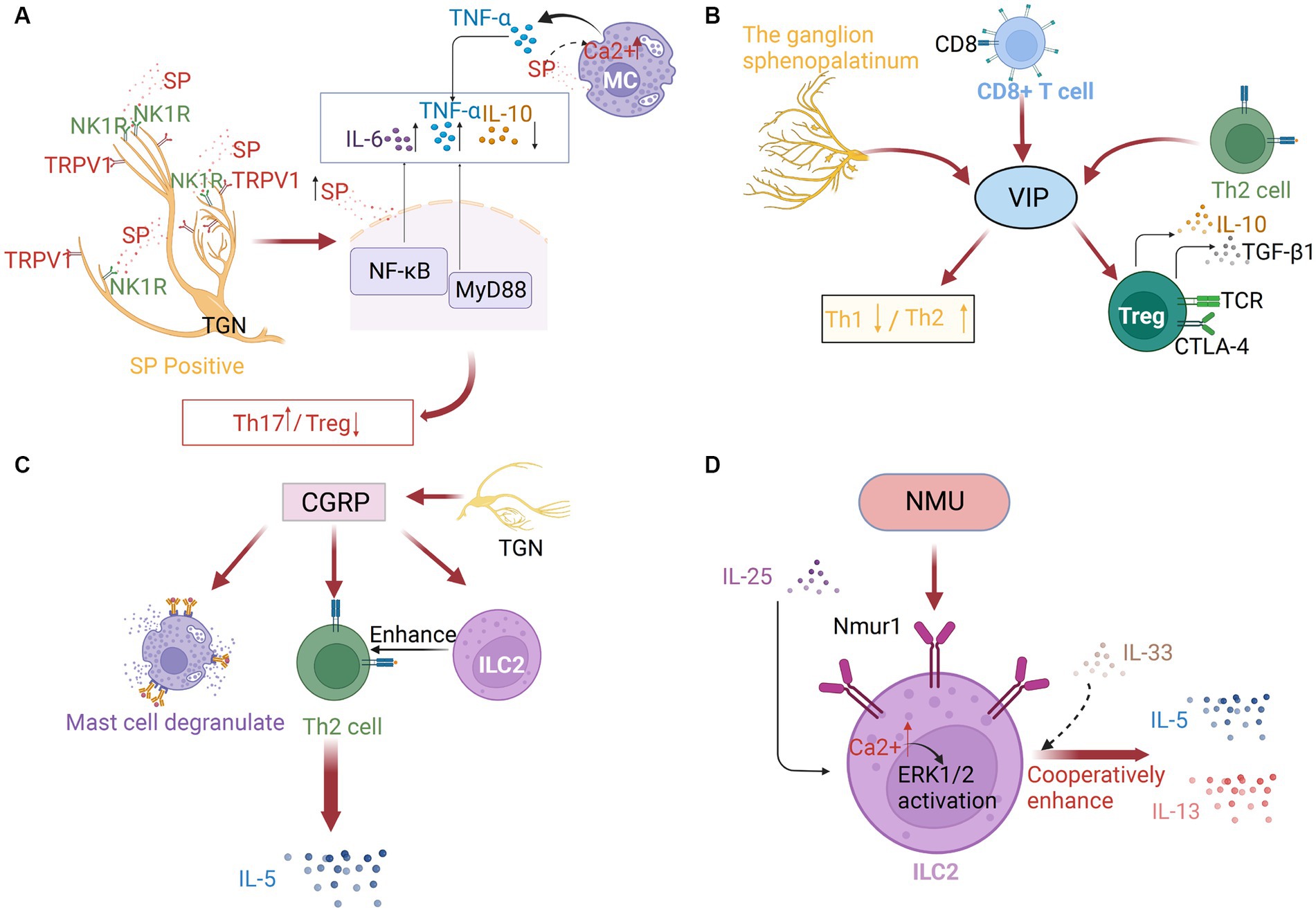
Figure 2. (A) Substance P (SP) in neuroimmune communication in AR. The trigeminal ganglion’s sensory neuronal cell bodies and afferent nerve terminals are where SP is concentrated, which are frequently TRPV1-positive. After binding to the neurokinin 1 receptor (NK1R), SP may control neurogenic inflammation via the NF-κB pathway or the MyD88 pathway. As SP concentrations increased, IL-6 and TNF-α were elevated while IL-10 was downregulated. By increasing the intracellular [Ca2+] of mast cells, SP can also stimulate TNF-α production. (B) Vasoactive intestinal peptide (VIP) in neuroimmune communication of AR. The ganglion sphenopalatinum is the origin of VIP. It can also be secreted by immune cells, especially by type 2 CD8+ T cells and Th2 cells. It can regulate Th1/Th2 balance, and promote Treg cell release of IL10 and TGF-β1. (C) Calcitonin gene-related peptide (CGRP) in neuroimmune communication of allergic rhinitis. Massive amounts of CGRPs are secreted by TGNs. They can trigger mast cell degranulation, ILC2 proliferation, and the following Th2 response, which induces the production of IL-5. (D) Neuromedin U (NMU) in neuroimmune communication of allergic rhinitis. ILC2s preferentially express neuromedin U receptor 1 (Nmur1), especially after IL-25 stimulation, and with the enhanced Ca2+ influx and the subsequent ERK1/2 activation, IL-5 and IL-13 expression, with IL-33 or independently of IL-33, is also increased.
In addition to its effect on MCs, recent studies have demonstrated that a burst of neuronal and epithelial SP serves as an initial defensive response in the nose (66). A recent study on patients with AR demonstrated that the SP-Toll-like receptor axis is impaired with delayed and prolonged upregulation of TLR4, which contributes to the more severe and persistent responses to infection in AR (78). This may aggravate nasal symptoms of AR, especially when encountered with infections.
3.2 Vasoactive intestinal peptide
VIP is mainly produced by parasympathetic nerves, and the sphenopalatine ganglion is the origin of nerve fibers in the upper respiratory tract that contain VIP. Additionally, VIP is additionally secreted by a variety of immune cells, including MCs, eosinophils, lymphocytes, and primarily type 2 CD8+ T cells and Th2 cells, especially under inflammatory conditions or after antigenic stimulation (79–81). VIP can maintain the Th1/Th2 balance by downregulating inflammatory Th1 type immune responses and upregulating Th2 type immune responses. Additionally, VIP-induced CD4+ CD25+ cells exhibit an active Treg cell phenotype and release high quantities of IL10 and TGF-β1 (79, 80) (Figure 2B). Compared with those in the nasal mucosa of healthy subjects, the mRNA and protein levels of VIP receptors were upregulated in the nasal mucosa of patients with AR. Additionally, the nasal tissue and secretion levels of VIP in patients with AR were upregulated when compared with those in healthy individuals. The number of VIP-positive fibers was significantly upregulated in the nasal tissues of patients with AR (81). Acupuncture treatment in AR patients significantly downregulated the salivary VIP levels (49). VIP can stimulate the secretion of human serous cells, expand the nasal blood tube, and regulate the clearance of nasal mucosa cilia (82, 83). The effects of VIP on the AR are mediated through their specialized receptors (CRTH2, PAC1, VPAC-1, and VPAC-2), which are expressed on various immune cells, including MCs, eosinophils, and lymphocytes (79, 84). These receptors mediate the regulatory effects of VIP on immunological response through various protein kinases, including the phospholipase C/PKC and mitogen-activated protein kinase (MAPK) pathways, as well as the adenylate cyclase/PKA pathway. On inflammatory cells like basophils, eosinophils, and Th2 lymphocytes, the CRTH2 receptor has been identified. Blocking this receptor suppresses allergic airway inflammation (79). AR may be significantly influenced by the relationship between mast cells and the VPAC-2 receptor. The proximity of neurons expressing VIP to the immune system components and the expression of VIP receptors on the surface of immune cells indicated the VIP-immune system interaction in AR.
3.3 Calcitonin gene-related peptide
The neuropeptide CGRP is massively secreted by TGNs. The expression of CGRP has been detected in 50% of human TGNs. CGRP, which is released from both peripheral nerve and central nerve terminals, exerts various biological effects, especially vasodilation (71). In patients with AR, allergen challenge promotes CGRP-mediated nasal congestion and upregulates mucosal edema caused by histamine and lipids. The level of CGRP is significantly upregulated in nasal secretions. The alleviation of nasal symptoms in AR patients is associated with the downregulation of CGRP in the nasal mucosa (85, 86). MCs are frequently detected near CGRP-expressing nerve fibers. CGRP regulates the expression of several genes involved in nerve fiber activation. Additionally, CGRP promotes the recruitment of MCs in mice (87). Animal studies have demonstrated that CGRP induces MCs degranulation and facilitates Th2 differentiation (34, 88). The direct effect of CGRP on innate lymphoid cells (ILCs) stimulates a Th2 response (71), which may promote the development of AR.
CGRP stimulates the expression of nuclear factor of activated T cells c2(NFATC2) through the cAMP/PKA pathway by binding to the activity-modifying protein 1 (RAMP1) and calcitonin receptor-like receptor (CLR), promoting the production of IL-17 in Th17 cells. This mechanism is suppressed in RAMP1-deficient mice (89). Recent studies have demonstrated that the CGRP-ILC2 axis contributes to allergic asthma development, and may be crucial in the initiation of AR type 2 inflammation. CGRP can act directly to stimulate the maturation of ILC2s and promote the generation of cytokines, such as IL-5. ILC2s that produce IL-5 have critical roles in the development of allergy symptoms. Activated ILC2s promote the recruitment of eosinophils and initiate a cascade of Th2 responses. These ILC2s can be suppressed upon treatment with CGRP antagonists (Figure 2C) (90, 91). Targeting ILC2 to inhibit the CGRP signaling is a novel therapeutic strategy for allergy disorders.
3.4 Neuromedin U
Cholinergic neuron-derived NMU, a 33 amino-acid peptide that is widely conserved, functions as a rapid and effective type 2 cytokine regulator (92). NMU and its receptor NMUR1 are expressed in T cells, DCs, eosinophils, and MCs. MCs may regulate the NMU/NMUR axis in humans (93). NMU promotes Ca2+ mobilization and degranulation in MCs, inducing vasodilation and plasma extravasation (94). Previous studies have demonstrated that NMU stimulates ILC2s and consequently induces the production of type 2 cytokines in enteritis and asthma mouse models (95, 96). After allergen exposure, NMU functions as a neuronal amplifier to elicit a strong, rapid, and selective activation of ILC2s (96). ILC2s preferentially express NMUR1, especially after IL-25 stimulation, while mucosal neurons express NMU. The co-administration of NMU and IL-25 markedly exacerbated allergic inflammation in vivo (95, 97). NMU cooperatively enhanced IL-5 and IL-13 expression through IL-33-dependent or IL-33-independent mechanisms (96, 98). A recent study in AR patients demonstrated that NMU activates ILC2s, resulting in the upregulation of the inflammatory factors IL-5 and IL-13 rather than IL-33 in patients with AR, triggering a type 2 inflammatory response, which was inhibited upon ERK pathway blockade (99).
These findings indicate the presence of a unique neuroimmune pathway that can exacerbate allergic inflammation of the mucosa and induce nasal hyperresponsiveness (97). The induction of MC degranulation is critical for AR pathogenesis (100). ILC2s, which express NMUR1, are associated with neurons that express NMU to generate neuroimmune cell units (92). The mechanism may involve NMUR1-mediated NMU stimulation of ILC2s, resulting in enhanced Ca2+ influx, effective ERK1/2 activation, and cytokine generation (95) (Figure 2D).
3.5 Nerve growth factor
NGF was the first member of the neurotrophin family protein to be discovered. The main function of NGF is to promote the growth, differentiation, and survival of peripheral and central nerves (101). NGF, which is expressed in the nasal epithelium, glandular epithelium, and peripheral nerves in the nasal mucosa, plays a critical role in the bidirectional signaling mechanisms between the neurosensory network structures and immune cells that can lead to hyperresponsiveness (1, 102, 103). In patients with AR, the NGF protein level is upregulated in the nasal submucosa, submucosal glands, nasal lavage fluids, and serum. NGF is localized to the MCs and eosinophils of the nasal mucosa (4, 6, 103, 104). The levels of NGF-positive nerve bundles, tropomyosin receptor kinase A (TrkA), and p75 receptors of NGF are significantly upregulated in patients with AR (1), indicating the role of NGF in the allergic inflammatory process of AR.
In addition to neurons, immune cells, including MCs and eosinophils, can release NGF (29, 104). TrkA and p75 receptors are expressed in the nerves, the nasal epithelium, and submucosal glands (1). MCs that express and secrete NGF are close to the tachykinin-containing nerve terminals in the peripheral tissue. NGF, which upregulates the expression of neuropeptides, such as SP, regulates the transit of SP in sensory nerves to promote the antidromic release of SP upon nerve activation (6, 104). In the human nasal mucosa, TrkA and p75 receptors were identified along the sensory nerves (4). These characteristics favor the formation of neuroimmune regulatory units, revealing neuroimmune interaction in AR-associated NHR.
A recent study reported that the prolonged stimulation of isolated sensory neurons by NGF promotes a switch from PKA activation to Epacs activation in the signaling pathway, resulting in prostaglandin E2-induced hypersensitivity (105). Exposure to cold temperatures upregulates the number of eosinophils and the production of NGF in mice, which promotes sympathetic axonal outgrowth. In both humans and mice, sympathetic nerves are located close to IL-33-expressing stromal cells and eosinophils. The signal from sympathetic nerves triggers calcium flux in stromal cells and the subsequent release of IL-33, which upregulates IL-5 in ILC2s (106). Additionally, NGF directly affects eosinophils by promoting IL-4 secretion (1) (Figure 3). These immediate and delayed effects constitute the mechanism through which NGF mediates AR inflammation and hyperresponsiveness (1).
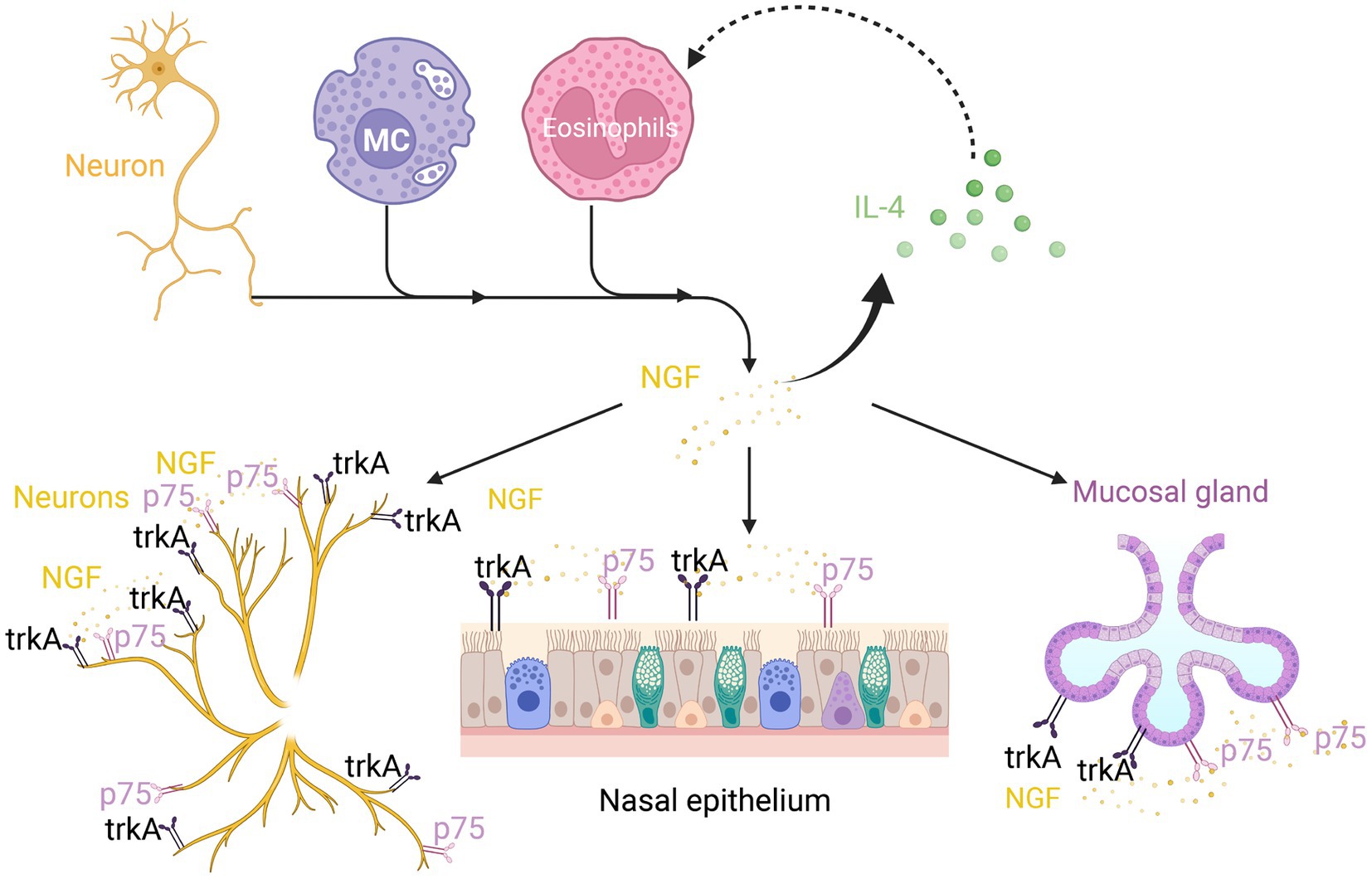
Figure 3. Nerve growth factor (NGF) in neuroimmune communication in AR. In addition to neurons, mast cells and eosinophils can also release NGF. The TrkA and p75 receptors can not only express on neurons but also on the nasal epithelium and submucosal glands. This can boost IL-4 secretion, which in turn promotes NGF secretion by eosinophils.
4 Formation of neuroimmune cells units in AR
Immune and neural cells are frequently colocalized at certain anatomical locations. Eosinophils, lymphocytes, and MCs accumulate in neuroimmune cell units around the airway nerves, where they coordinate their responses for bidirectional neuroimmune interactions (107–109). The number of neuroimmune cell units is upregulated in patients with AR.
4.1 Nerve-MCs unit
4.1.1 Role of MCs in AR
The high-affinity IgE receptor FceR1 is expressed on the surface of MCs (110). Various inflammatory mediators, including eicosanoids, proteases, biogenic amines, chemokines, and cytokines, are released upon allergen-mediated activation of MCs through the cross-linking of cell surface-bound IgE to its high-affinity receptor (FcεRI) in sensitized individuals, exacerbating allergic inflammation (111, 112). Additionally, the binding of IgE to FceR1 stabilizes the expression of the receptor on cell surfaces, upregulating the expression of FceR1 (113). Histamine, leukotrienes, and tryptase are MC-derived mediators that interact with specific receptors on sensory nerve endings, promoting the production of neuropeptides (74). MCs can also respond to stimulation with neuropeptides in an FcεRI-independent manner. These findings indicate the function of MCs in neuroimmune interactions (79).
Tryptases and chymases, which are proteases expressed in MCs, are regulated by IL-10 and IL-4 (111). MCs can be classified based on the contents of their secretory granules as follows: MCTC, granules contain both tryptase and chymase; MCT, granules contain tryptase but little or no chymase; MCC, an infrequent phenotype and the granules contain little chymase or no tryptase (114–116). Consequently, only MCT and MCTC are referenced in some studies. In patients with AR, the tryptase levels are upregulated in the nasal lavage and the phenotype of MCs resembles that of MCT. The serum tryptase levels were associated with the levels of neuropeptides, indicating that neuropeptides are involved in the regulation of MCs (81, 116).
4.1.2 Nerve-MCs colocalization and communication with neuropeptides
MCs and neuropeptide-containing nerves are anatomically connected and are prevalent in patients with AR. The MC-mediated immune reaction is downregulated in the absence of sensory nerves (34, 117, 118). The anatomical distance between MCs and nerves enables bidirectional communication. A small number of MCs form synaptic-like contacts with nerves that are dependent on integrins, especially cell adhesion molecule 1 (CADM1; also known as SynCAM1). CADM1, which is expressed in MCs and sensory neurons, mediates interactions between MCs and sensory neurons. IL-6 production and degranulation are activated in MCs through CADM1-dependent interactions with sensory neurons. CADM1 knockdown in MCs markedly impaired the physical interaction and the functional relationship with sensory neurons in mice (119). Intragranular particles of MCs can be transported into neurons through direct fusion with the plasma membrane of the neuron or through the neuronal capture of insoluble granule remnants (120).
Human MCs express a CGRP receptor (CGRPR), SP receptor (NK1R), VIP receptors (VPAC-1 and 2), and Mas-related gene receptor X2 (MRGPRX2), a cation-sensing receptor for various ligands such as SP, VIP and somatostatin (SST) (121). MRGPRX2 mediates non-IgE-dependent activation in patients with AR, inducing hypersensitivity, neurogenic inflammation, and itching (122). Additionally, MCs promote the release of neuropeptides, such as SP and CGRP from nerves (117). Trypsin and MC tryptase activate the protease-activated receptor 2 (PAR2), which is expressed on the surface of nerve fibers, including trigeminal neurons in the human nasal mucosa, eliciting a generalized inflammatory response through unidentified pathways. Additionally, trypsin and tryptase promote the release of SP and related peptides from sensory neurons, mediating neurogenic inflammation (123, 124). The CRTH2 receptor is a G protein-coupled receptor for the Prostaglandin D2 receptor. It was discovered that VIP activates the synthesis of the CRTH2 receptor, which is expressed by 34% of mast cells in human nasal polyps. Recruitment of inflammatory cells is induced by this interaction. Furthermore, blocking this receptor decreases allergy manifestation (79) (Figure 4A). In addition, it has been demonstrated that nerve–MC interactions allow corticotropin releasing hormone (CRF) and associated peptides to regulate ion and water secretion. C nerve fibers express H1 and H4 receptors for histamine, and those activated nerves release neuropeptides which in turn induce histamine and prostaglandin D2 release from MCs (125). These findings revealed that MCs serve as a functional homeostatic regulatory unit to facilitate neuroimmune interaction.
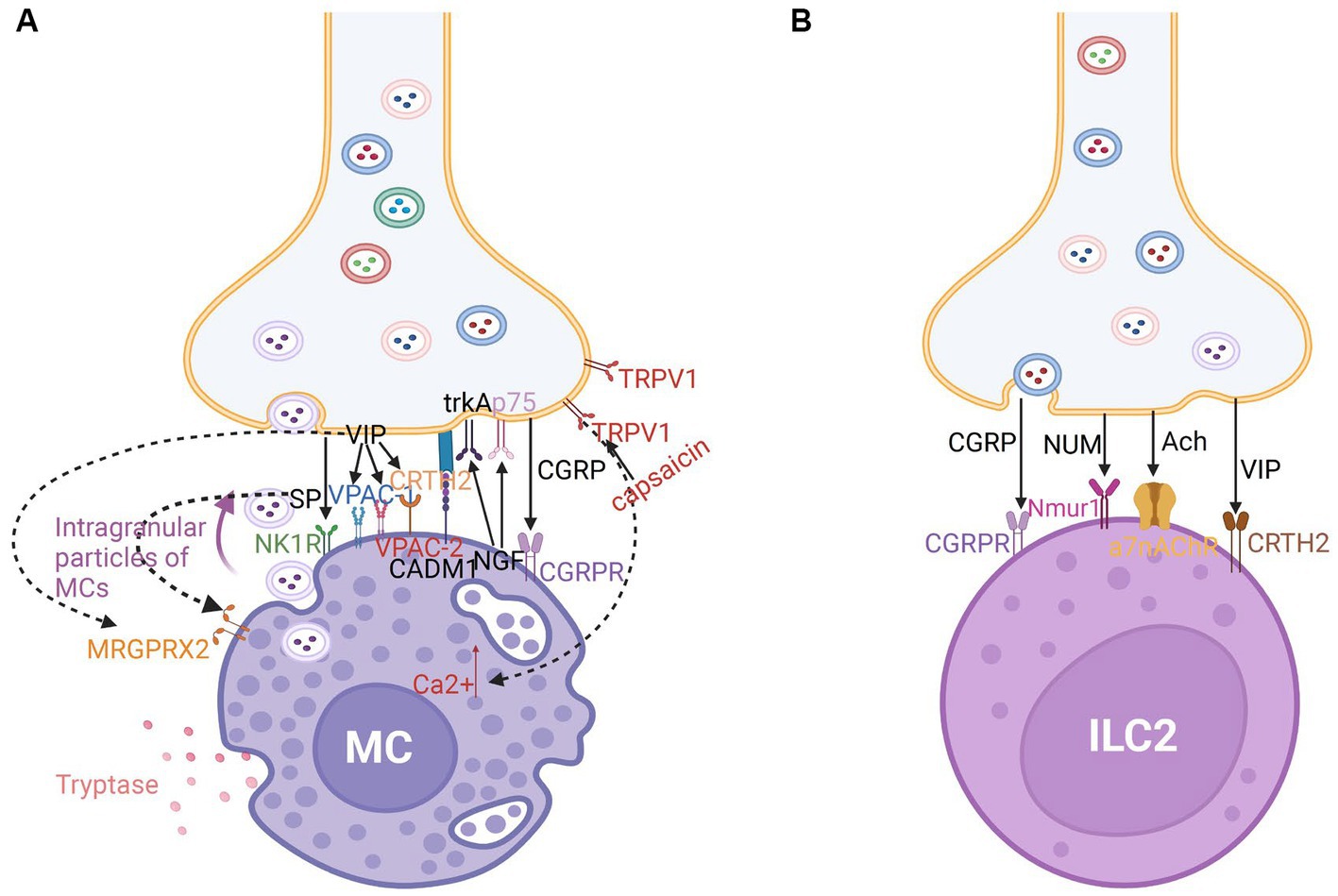
Figure 4. (A) Nerve-MC unit in neuroimmune communication in allergic rhinitis. Tryptase-secreting MCs play a significant role in allergic rhinitis. The development of nerve-MC regulating units is mediated by CADM1. Intragranular particles of MCs can be transported into neurons. There are a large number of receptors on MCs such as calcitonin-receptor-like receptor (CGRPR) for CGRP; NK1 and MRGPRX2 receptors for SP; VPAC-1, VPAC-1, MRGPRX2, and CRTH2 receptors for VIP; and TrkA and p75 receptors for NGF. Capsaicin stimulates TRPV1, which encourages mast cell Ca2+ increase and the ensuing immunological response. (B) Nerve-ILC2 unit in neuroimmune communication in allergic rhinitis. ILC2s express CGRPR for CGRP, Nmur1 for NUM, a7nAChR for Ach, and CRTH2 receptors for VIP.
4.2 Nerve-ILC2s unit
Innate lymphoid cells (ILCs) without receptors to recognize antigens like T and B cells are novel immune cells with a lymphoid appearance and are abundantly distributed in the mucosal tissues. Additionally, ILCs have been divided into three different subpopulations. ILC2s share phenotypic characteristics with Th2 cells (126). Various cytokines, especially IL-25 and IL-33, can induce ILC2s to release type 2 cytokines, such as IL-13 and IL-5 to trigger allergic tissue inflammation at the mucosal surfaces in patients with AR (97, 127). Recent studies on the communication between nerves and ILC2s revealed that type 2 inflammation in allergy disorders is significantly influenced by neuroimmune interactions (34). Neurons, especially cholinergic neurons in mice, form close intercellular contacts with ILC2s, generating a novel neuron-ILC2 unit (95, 96, 98).
Cholinergic neurons release the neuropeptide NMU, which promotes type 2 cytokine responses through ILC2 activation. Compared with that in other ILC subtypes, the expression of NMUR1 is upregulated in ILC2s. Additionally, CGRP modulates the activation of ILC2s, which regulate the intensity of type 2 inflammation in mice (95, 96, 128). The a7-nicotinic acetylcholine receptor (a7nAChR) is expressed by ILC2s in mice (129) (Figure 4B). The agonists act on a7nAchR and may suppress phosphorylation of upstream kinase IKKa/b and NF-kB expression to reduce the release of cytokines like IL-5 and IL-13 and suppress airway hyperreactivity (AHR). Acetylcholine may inhibit ILC2-mediated inflammation through a7nAchR (127, 129).
Most neuropeptides bind to GPCRs, which comprise alpha, beta, and gamma subunits. G alpha proteins are categorized into Gas, Gai, and Gaq subtypes. Gas activates adenyl cyclase (AC), which upregulates intracellular cAMP levels and activates PKA, enhancing the cellular Ca2+ concentration. Gai inhibits AC activation and decreases intracellular cAMP levels. Intracellular Ca2+ induces ILC2s to trigger a cascade of Th2 responses. In contrast, cAMP inhibits ILC2 activation. Therefore, the type of Ga protein, which can exert different effects on intracellular Ca2+ and cAMP levels, can be used to elucidate the regulatory mechanisms of neuropeptides in ILC2s. Catecholamines suppress ILC2-mediated inflammation by upregulating cAMP levels through Gas protein (127).
4.3 Nerve-eosinophils unit
Eosinophils are recruited to nasal nerves after an allergen challenge through the reduction of neuron activation threshold. The recruitment of eosinophils promotes nerve growth and modulates neuropeptide production, resulting in neuronal hyperreactivity in AR (109, 130). Inhibitory M2 muscarinic receptors (M2Rs) expressed on the surface of postganglionic nerves in experimental animals can be blocked by basic protein (MBP) expressed in activated eosinophils, promoting acetylcholine release (131). Eosinophils tend to colocalize with the choline acetyltransferase (ChAT) immunoreactive nerves. In patients with AR, eosinophils exhibit CRTH2 expression (109). Previous investigations in AR patients showed that VIP peptide and CRTH2 molecules co-localized and had a significant interaction (83). The co-localization may be mediated by eosinophil chemoattractant CCL-26 and VCAM-1 expressed by nasal nerve fibers in animal models (109). In combination with other mechanisms, including SP immunoreactive nerves, this leads to hyperresponsiveness of the nasal nerves, contributing to NHR development (109) (Figure 5A).
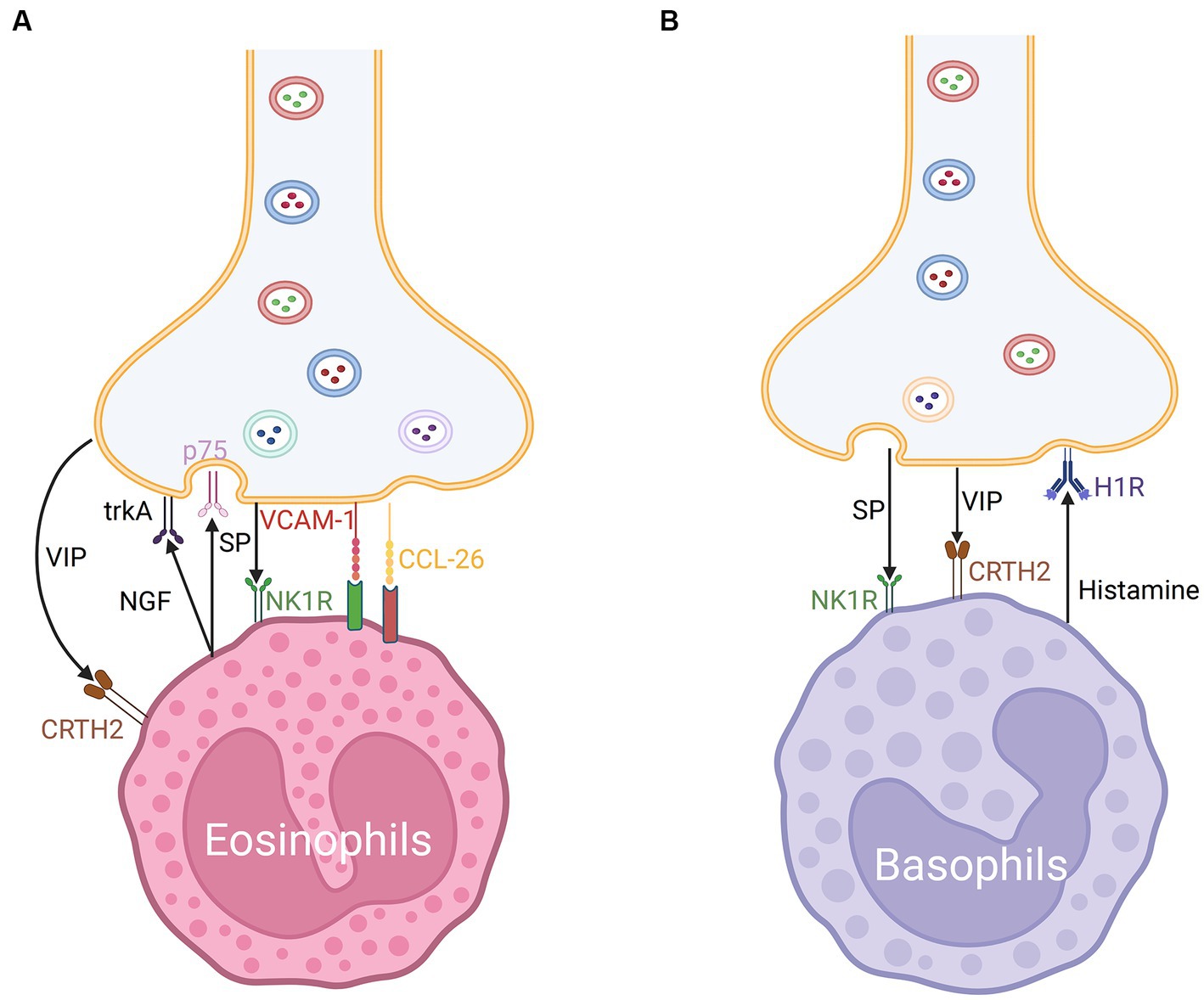
Figure 5. (A) Nerve-eosinophils unit in neuroimmune communication in allergic rhinitis. The co-localization of nerve fibers and eosinophils may be mediated by CCL-26 and VCAM-1. Eosinophils express CRTH2 receptors for VIP and NK1 receptors for SP. Eosinophils can secrete NGF which acts on trkA and p75 of nerves via synaptic-like contacts. (B) Nerve-basophils unit in neuroimmune communication in allergic rhinitis. Basophils produce histamine which acts on the H1R of nerve fibers and expresses NK1 receptors for SP and CRTH2 receptors for VIP.
The spraying of glucocorticoids targeting eosinophilic inflammation is the main drug for treating AR and is currently considered relatively safe (132). Additionally, in antigen-induced individuals, neutralizing MBP with a specific antibody or with polyanionic substances such as heparin or poly-l-glutamic acid and reducing eosinophils with an anti-IL-5 antibody may prevent M2R dysfunction and nasal hyperresponsiveness. A CCR3 antagonist suppressed antigen-induced eosinophil recruitment along the nerves in animals, which may provide novel strategies for the treatment of AR (131).
4.4 Nerve-basophils unit
Basophils promote Th2 cytokine-mediated inflammation. The cross-linking of the high-affinity IgE receptor FcεRI and IgE on basophil surfaces after allergen challenge promotes the release of various mediators, which contribute to allergic inflammation in AR (133). Additionally, basophils were discovered in the nasal lavage fluid of AR individuals, which are considered to be the dominant source of histamine in anaphylactic late phase response (LPR). Therapeutics for AR may exert their effects on MCs and partially regulate basophil-derived histamine and leukotrienes (134).
Additionally, various immune inflammatory factors and neuropeptides modulate the function of basophils. Allergen-activated basophil-derived factors can directly activate and interact with sensory neurons. The basophil-neuronal circuit is involved in the pathogenesis of various neuroimmune processes associated with allergic reactions in AR, such as the formation of the basophil-leukotriene (LT) axis (135). The upregulation of MRGPRX2 was related to degranulation and CD63 expression in basophils in mice (122). CRTH2, a receptor of VIP, is also expressed on the surface of basophils and may mediate chemotaxis (79, 83) (Figure 5B). ILC2s from basophil-depleted mice express less neuromedin B (NMB) receptor, a member of the neuromedin family of neuropeptides that also includes NMU, NMK, NMC, NMS, NMN, and NML, demonstrating that basophils act as the transition switch necessary for ILC2s to react to NMB-mediated inhibition, which may work by modulating the expression of P2rx7 (128).
5 Potential roles of MRGPRX2 in neuroimmunomodulation in AR
MRGPRX2, originally discovered in neurons, is found not only in neurons but also in MCs and is expressed on comparable levels on peripheral blood basophils and eosinophils (122, 136, 137). The MRGPRX2-mediated activation of MCs is associated with neurogenic inflammation (138). Recent studies on MCs have focused on MRGPRX2, especially on its role in IgE-independent degranulation (116). Previous studies have reported that MRGPRX2 is mainly expressed in MCTC but not in MCT, while MCT is found on mucosal surfaces such as the lung (74, 116, 139). Although it was previously considered that mucosal MCs are of the MCT type and do not express MRGPRX2, it now appears that 20% of normal human lung mast cells express MRGPRX2 (74). Manorak et al. reported that the MRGPRX2 level was downregulated in non-asthmatic lung MCs but markedly upregulated in asthma lung MCs in humans (140). An et al. confirmed that the mean serum MRGPRX2 levels in patients with asthma were higher than those in patients without asthma (136). The serum MRGPRX2 levels in patients with chronic spontaneous urticaria were positively correlated with specific IgE against Dermatophagoides farinae, which is a major etiological factor for AR (141).
Ai J et al. discovered that endoscopic vidian neurectomy of AR improved QOL related to asthma in roughly half of the patients with AR (142). Imperatorin (IMP), which was recently demonstrated to be an inhibitor of MRGPRX2 and exert therapeutic effects on allergic asthma, exerts anti-inflammatory effects in the AR model (143). AR and asthma share pathogenesis and histological characteristics and are considered different manifestations of the same underlying atopic state. MRGPRX2 activation is hypothesized to significantly increase the inflammatory response in AR.
MRGPRX2 is suggested to contribute to the pathogenesis of AR by promoting IgE-independent activation of human MCs through neuropeptides (SP, NGF, CGRP, and VIP), cysteine proteases, antimicrobial peptides, and eosinophilic cationic proteins (137, 144). Treatment with MRGPRX2 ligands upregulates the calcium influx in human MCs (115, 145). SP causes itchiness by activating MRGPRX2 in addition to NK1R (59). In addition to SP, HK-1 stimulates human MCs via MRGPRX2 rather than NK1R, especially during airway inflammation (140). Selective MRGPRX2 antagonists are potential therapeutics for AR although further studies are needed to clarify their underlying mechanisms and enable their clinical application.
6 Psychological condition-mediated neuroimmune regulation in AR
Previous studies have reported a correlation between AR and psychological disorders (146). The neurons in the prefrontal cortex and olfactory bulbs of AR rats produce Th2 cytokines (147). The mechanism associated with IgE production includes cortisol-induced alterations in serotonin secretion mediated by the activated hypothalamic–pituitary–adrenal axis (HPA) due to hypersensitive response (146).
Several mediators released in AR can alter neuronal activity (especially in areas of the brain that process emotion and affective behaviors), activate the HPA axis, and induce stress response (148, 149). IL-1β can be generated by almost all nucleated cells, including B lymphocytes, natural killer cells, MCs, and epithelial cells (150, 151). Additionally, IL-1β directly acts on MCs and promotes the secretion of cytokines (152). Allergen exposure promotes IL-1β secretion in human nasal epithelial cells of patients with AR (153). IL-1β can also stimulate the HPA axis, promoting the production of cortisol, which triggers the release of serotonin and contributes to mood changes (146). The serum NGF levels are upregulated in caregivers who experience prolonged stress (154). Several studies have reported the correlation between allergic symptoms in children and the psychological state of caregivers (155). Warm and responsive caregiving can reduce the occurrence of allergic diseases in children (156).
7 Conclusion
Neuroimmune communication is reported to be involved in the pathogenesis of AR, which is now classified as a neuroimmune illness. Pharmacological agents that target neuroimmune communication can be potentially effective. Clinical evidence suggests that acupuncture can alleviate the symptoms of AR by downregulating SP, a proinflammatory neuropeptide (49). Vidian neurectomy (VN) or posterior nasal neurectomy (PNN) via endoscopy have been introduced to treat refractory AR and can significantly alleviate the symptoms of sneezing and rhinorrhea (157). Office-based cryotherapy of the PNN region can significantly decrease symptom scores (158). The effectiveness of nerve desensitization with repetitive application of capsaicin or resection of the vidian nerve indicated that the nasal mucosa of patients with AR exhibited nerve-immune mediated hyperresponsiveness (6). These treatments may affect neuroimmune interaction in AR. The development of nasal hyperresponsiveness is linked to immune cell modulation and reflexes. The formation of “axon reflexes” and “central sensitization” promotes the development of “neurogenic inflammation,” which stimulates the release of various neuropeptides from nerve endings and the recruitment and activation of immune cells, resulting in the development of symptoms. Several immune cells have receptors for neuropeptides. Immune cells also release neuropeptides, which exert regulatory effects. The SP levels in nasal lavages and the number of SP-positive nerve fibers in patients with AR were higher than those in healthy individuals. TRPV1 and TRPA1 are involved in SP-induced allergic immune responses. The concentrations of VIP and the number of VIP-positive fibers are upregulated in the nasal tissues of patients with AR. VIP maintains the Th1/Th2 balance through various receptors. TGN can secrete a large amount of CGRP, which induces the activation of both MCs and ILCs to elicit a Th2 response. NMU, NGF, and their receptors are crucial factors regulating neuroimmune communication in AR.
Immune cells are colocalized with nerves in specific anatomical locations, forming neuroimmune cell units. CADM1 mediates the formation of synaptic-like contacts in the nerve-MC unit, promoting bidirectional transport between MCs and neurons. TRPA1 and TRPV1 regulate the function of the nerve-MC unit. Nerve-ILC2 units are in the vicinity of cholinergic neurons and can regulate the severity of type 2 inflammation in AR. The nerve-basophils and nerve-eosinophils units are also involved in the pathogenesis of AR. Additionally, the inflammatory response in AR is exacerbated upon the activation of MRGPRX2, which is expressed in some immune cells and nerve cells. The silencing of MRGPRX2 downregulates MC degranulation. Analysis of the function of the HPA axis revealed that AR is correlated with psychological disorders.
The major therapeutic strategies for AR are drug therapy and immunotherapy. However, these therapies are associated with side effects, such as fatigue. Additionally, the adherence rate of immunotherapy is poor. The therapeutics for AR exhibit broad anti-inflammatory properties (typically glucocorticoids), neutralize secreted products (antihistamines), or inhibit IgE (omalizumab). However, these therapies are not effective in alleviating symptoms in some individuals. Thus, there is an urgent need to develop novel and efficient therapeutic interventions. The clinical application and potential mechanisms of surgical treatment and acupuncture targeting neuroimmune interaction must be examined in future studies. The TRPA1 antagonist HC-030031 and the TRPV1 agonist capsaicin were demonstrated to exert effective therapeutic effects on AR. However, the underlying therapeutic mechanisms of HC-030031 and capsaicin have not been elucidated. Additionally, although neuropeptides can be detected in nasal secretions, their clinical value has not been established. The neuropeptide levels in preformed nasal secretions can predict the treatment response of patients. Pharmacological products that counteract the effects of neuropeptides are promising therapeutic agents for AR. To suppress SP-induced itching, nociception, and inflammation in mouse models, various pharmacological NK1R antagonists have been developed. However, human trials with NK1R antagonists have yielded inconsistent results. Hence, novel SP receptor antagonists must be developed and their safety must be evaluated before clinical application. Neuroimmune communication may offer novel insights for developing diagnostic and therapeutic strategies. Hence, the ability of currently used therapeutics for AR to regulate neuroimmune communication must be investigated in the future.
Author contributions
YZ: Writing – original draft, Writing – review & editing. RC: Writing – original draft. LK: Resources, Writing – original draft. YS: Resources, Writing – original draft. JD: Supervision, Writing – review & editing.
Funding
The author(s) declare financial support was received for the research, authorship, and/or publication of this article. This work was supported by a grant from the Zhejiang Traditional Chinese Medicine Science and Technology Plan Project (no. 2022ZB358).
Conflict of interest
The authors declare that the research was conducted in the absence of any commercial or financial relationships that could be construed as a potential conflict of interest.
Publisher’s note
All claims expressed in this article are solely those of the authors and do not necessarily represent those of their affiliated organizations, or those of the publisher, the editors and the reviewers. Any product that may be evaluated in this article, or claim that may be made by its manufacturer, is not guaranteed or endorsed by the publisher.
References
1. Gelincik, A, Aydin, F, Ozerman, B, Erguven, M, Aydin, S, Bilir, A, et al. Enhanced nerve growth factor expression by mast cells does not differ significantly between idiopathic and allergic rhinitis. Ann Allergy Asthma Immunol. (2012) 108:396–401. doi: 10.1016/j.anai.2012.04.006
2. Wang, XD, Zheng, M, Lou, HF, Wang, CS, Zhang, Y, Bo, MY, et al. An increased prevalence of self-reported allergic rhinitis in major Chinese cities from 2005 to 2011. Allergy. (2016) 71:1170–80. doi: 10.1111/all.12874
3. Cheng, L, Chen, J, Fu, Q, He, S, Li, H, Liu, Z, et al. Chinese Society of Allergy Guidelines for diagnosis and treatment of allergic rhinitis. Allergy, Asthma Immunol Res. (2018) 10:300–53. doi: 10.4168/aair.2018.10.4.300
4. Wu, X, Myers, AC, Goldstone, AC, Togias, A, and Sanico, AM. Localization of nerve growth factor and its receptors in the human nasal mucosa. J Allergy Clin Immunol. (2006) 118:428–33. doi: 10.1016/j.jaci.2006.04.037
5. Wheatley, LM, and Togias, A. Clinical practice. Allergic Rhinitis N Engl J Med. (2015) 372:456–63. doi: 10.1056/NEJMcp1412282
6. Sanico, AM, Stanisz, AM, Gleeson, TD, Bora, S, Proud, D, Bienenstock, J, et al. Nerve growth factor expression and release in allergic inflammatory disease of the upper airways. Am J Respir Crit Care Med. (2000) 161:1631–5. doi: 10.1164/ajrccm.161.5.9908028
7. Klimov, V, Cherevko, N, Klimov, A, and Novikov, P. Neuronal-immune cell units in allergic inflammation in the nose. Int J Mol Sci. (2022) 23:6938. doi: 10.3390/ijms23136938
8. Li, Q, Zhang, X, Feng, Q, Zhou, H, Ma, C, Lin, C, et al. Common allergens and immune responses associated with allergic rhinitis in China. J Asthma Allergy. (2023) 16:851–61. doi: 10.2147/JAA.S420328
9. Okubo, K, Kurono, Y, Ichimura, K, Enomoto, T, Okamoto, Y, Kawauchi, H, et al. Japanese guidelines for allergic rhinitis 2020. Allergol Int. (2020) 69:331–45. doi: 10.1016/j.alit.2020.04.001
10. Gu, ZWWY, and Cao, ZW. Neutralization of interleukin-17 suppresses allergic rhinitis symptoms by downregulating Th2 and Th17 responses and upregulating the Treg response. Oncotarget. (2017) 8:22361–9. doi: 10.18632/oncotarget.15652
11. Ma, Y, Liu, C, Xi, G, Guan, Y, Tang, Y, Zhang, J, et al. Bioinformatic analysis and cellular assays identify substance P influencing Th17/Treg differentiation via the MyD88 pathway as a potential contributor to the progression of asthma and allergic rhinitis. Dis Markers. (2022) 2022:1–11. doi: 10.1155/2022/3843954
12. Ding, WSL, Xu, L, Zhou, XK, Manni, M, Wagner, JA, and Granstein, RD. Calcitonin gene-related peptide-exposed endothelial cells Bias antigen presentation to CD4+ T cells toward a Th17 response. J Immunol. (2016) 196:2181–94. doi: 10.4049/jimmunol.1500303
13. Galli, SJ, Tsai, M, and Piliponsky, AM. The development of allergic inflammation. Nature. (2008) 454:445–54. doi: 10.1038/nature07204
14. Schiller, M, Ben-Shaanan, TL, and Rolls, A. Neuronal regulation of immunity: why, how and where? Nat Rev Immunol. (2021) 21:20–36. doi: 10.1038/s41577-020-0387-1
15. Delgado, M, Pozo, D, and Ganea, D. The significance of vasoactive intestinal peptide in immunomodulation. Pharmacol Rev. (2004) 56:249–90. doi: 10.1124/pr.56.2.7
16. Weihrauch, T, Limberg, MM, Gray, N, Schmelz, M, and Raap, U. Neurotrophins: Neuroimmune interactions in human atopic diseases. Int J Mol Sci. (2023) 24:6105. doi: 10.3390/ijms24076105
17. Kadowaki, M, Yamamoto, T, and Hayashi, S. Neuro-immune crosstalk and food allergy: focus on enteric neurons and mucosal mast cells. Allergol Int. (2022) 71:278–87. doi: 10.1016/j.alit.2022.03.004
18. Steinhoff, M, Ahmad, F, Pandey, A, Datsi, A, AlHammadi, A, Al-Khawaga, S, et al. Neuroimmune communication regulating pruritus in atopic dermatitis. J Allergy Clin Immunol. (2022) 149:1875–98. doi: 10.1016/j.jaci.2022.03.010
19. Kim, B, Rothenberg, ME, Sun, X, Bachert, C, Artis, D, Zaheer, R, et al. Neuroimmune interplay during type 2 inflammation: symptoms, mechanisms, and therapeutic targets in atopic diseases. J Allergy Clin Immunol. (2023). doi: 10.1016/j.jaci.2023.08.017
20. Konstantinou, GN, Konstantinou, GN, Koulias, C, Petalas, K, and Makris, M. Further understanding of neuro-immune interactions in allergy: implications in pathophysiology and role in disease progression. J Asthma Allergy. (2022) 15:1273–91. doi: 10.2147/JAA.S282039
21. Undem, BJ, and Taylor-Clark, T. Mechanisms underlying the neuronal-based symptoms of allergy. J Allergy Clin Immunol. (2014) 133:1521–34. doi: 10.1016/j.jaci.2013.11.027
22. Velasco, E, Delicado-Miralles, M, Hellings, PW, Gallar, J, Van Gerven, L, and Talavera, K. Epithelial and sensory mechanisms of nasal hyperreactivity. Allergy. (2022) 77:1450–63. doi: 10.1111/all.15259
23. Brierley, SM, and Linden, DR. Neuroplasticity and dysfunction after gastrointestinal inflammation. Nat Rev Gastroenterol Hepatol. (2014) 11:611–27. doi: 10.1038/nrgastro.2014.103
24. Van Gerven, L, Steelant, B, and Hellings, PW. Nasal hyperreactivity in rhinitis: a diagnostic and therapeutic challenge. Allergy. (2018) 73:1784–91. doi: 10.1111/all.13453
25. Hennel, M, Brozmanova, M, and Kollarik, M. Cough reflex sensitization from esophagus and nose. Pulm Pharmacol Ther. (2015) 35:117–21. doi: 10.1016/j.pupt.2015.10.007
26. Braunstahl, GJ, Fokkens, WJ, Overbeek, SE, KleinJan, A, and Hoogsteden, HC. Mucosal and systemic inflammatory changes in allergic rhinitis and asthma: a comparison between upper and lower airways. Clin Exp Allergy. (2003) 33:579–87. doi: 10.1046/j.1365-2222.2003.01652.x
27. Nordin, S . Mechanisms underlying nontoxic indoor air health problems: a review. Int J Hyg Environ Health. (2020) 226:113489. doi: 10.1016/j.ijheh.2020.113489
28. McKay, DMBJ . The interaction between mast cells and nerves in the gastrointestinal tract. Immunol Today. (1994) 15:533–8. doi: 10.1016/0167-5699(94)90210-0
29. Skaper, SD . Nerve growth factor: a neuroimmune crosstalk mediator for all seasons. Immunology. (2017) 151:1–15. doi: 10.1111/imm.12717
30. Baluk, P . Neurogenic inflammation in skin and airways. J Investig Dermatol Symp Proc. (1997) 2:76–81. doi: 10.1038/jidsymp.1997.15
31. Woolf, CJ . Central sensitization: implications for the diagnosis and treatment of pain. Pain. (2011) 152:S2–S15. doi: 10.1016/j.pain.2010.09.030
32. Meng, Y, Lu, H, Wang, C, Wang, Y, Meng, N, Yang, K, et al. Naso-ocular neuropeptide interactions in allergic rhinoconjunctivitis, rhinitis, and conjunctivitis. World Allergy Organ J. (2021) 14:100540. doi: 10.1016/j.waojou.2021.100540
33. Wilson, SR, The, L, Batia, LM, Beattie, K, Katibah, GE, McClain, SP, et al. The epithelial cell-derived atopic dermatitis cytokine TSLP activates neurons to induce itch. Cells. (2013) 155:285–95. doi: 10.1016/j.cell.2013.08.057
34. Kabata, H, and Artis, D. Neuro-immune crosstalk and allergic inflammation. J Clin Invest. (2019) 129:1475–82. doi: 10.1172/JCI124609
35. Devos, FC, Boonen, B, Alpizar, YA, Maes, T, Hox, V, Seys, S, et al. Neuro-immune interactions in chemical-induced airway hyperreactivity. Eur Respir J. (2016) 48:380–92. doi: 10.1183/13993003.01778-2015
36. Li, X, Yang, H, Han, Y, Yin, S, Shen, B, Wu, Y, et al. Tick peptides evoke itch by activating MrgprC11/MRGPRX1 to sensitize TRPV1 in pruriceptors. J Allergy Clin Immunol. (2021) 147:2236–2248.e16. doi: 10.1016/j.jaci.2020.12.626
37. Wilson, SR, Gerhold, KA, Bifolck-Fisher, A, Liu, Q, Patel, KN, Dong, X, et al. TRPA1 is required for histamine-independent, mas-related G protein-coupled receptor-mediated itch. Nat Neurosci. (2011) 14:595–602. doi: 10.1038/nn.2789
38. Liu, B, Tai, Y, Achanta, S, Kaelberer, MM, Caceres, AI, Shao, X, et al. IL-33/ST2 signaling excites sensory neurons and mediates itch response in a mouse model of poison ivy contact allergy. Proc Natl Acad Sci U S A. (2016) 113:E7572–9. doi: 10.1073/pnas.1606608113
39. Okano, M, Hirahara, K, Kiuchi, M, Onoue, M, Iwamura, C, Kokubo, K, et al. Interleukin-33-activated neuropeptide CGRP-producing memory Th2 cells cooperate with somatosensory neurons to induce conjunctival itch. Immunity. (2022) 55:2352–2368.e7. doi: 10.1016/j.immuni.2022.09.016
40. Gouin, O, L'Herondelle, K, Lebonvallet, N, Le Gall-Ianotto, C, Sakka, M, Buhe, V, et al. TRPV1 and TRPA1 in cutaneous neurogenic and chronic inflammation: pro-inflammatory response induced by their activation and their sensitization. Protein Cell. (2017) 8:644–61. doi: 10.1007/s13238-017-0395-5
41. Liu, M, Jia, X, Liu, H, He, R, Zhang, X, and Shao, Y. Role of TRPV1 in respiratory disease and association with traditional Chinese medicine: a literature review. Biomed Pharmacother. (2022) 155:113676. doi: 10.1016/j.biopha.2022.113676
42. Zhang, L, Kunkler, PE, Knopp, KL, Oxford, GS, and Hurley, JH. Role of intraganglionic transmission in the trigeminovascular pathway. Mol Pain. (2019) 15:174480691983657. doi: 10.1177/1744806919836570
43. Fang, Z, Yi, F, Peng, Y, Zhang, JJ, Zhang, L, Deng, Z, et al. Inhibition of TRPA1 reduces airway inflammation and hyperresponsiveness in mice with allergic rhinitis. FASEB J. (2021) 35:e21428. doi: 10.1096/fj.201902627R
44. Froghi, S, Grant, CR, Tandon, R, Quaglia, A, Davidson, B, and Fuller, B. New insights on the role of TRP channels in calcium Signalling and immunomodulation: review of pathways and implications for clinical practice. Clin Rev Allergy Immunol. (2021) 60:271–92. doi: 10.1007/s12016-020-08824-3
45. Shim, WS, Tak, MH, Lee, MH, Kim, M, Kim, M, Koo, JY, et al. TRPV1 mediates histamine-induced itching via the activation of phospholipase A2 and 12-lipoxygenase. J Neurosci. (2007) 27:2331–7. doi: 10.1523/JNEUROSCI.4643-06.2007
46. Lee, JH, Lee, YS, Lee, EJ, Lee, JH, and Kim, TY. Capsiate inhibits DNFB-induced atopic dermatitis in NC/Nga mice through mast cell and CD4+ T-cell inactivation. J Invest Dermatol. (2015) 135:1977–85. doi: 10.1038/jid.2015.117
47. Wouters, MM, Vicario, M, and Santos, J. The role of mast cells in functional GI disorders. Gut. (2016) 65:155–68. doi: 10.1136/gutjnl-2015-309151
48. Li, F, Jiang, H, Shen, X, Yang, W, Guo, C, Wang, Z, et al. Sneezing reflex is mediated by a peptidergic pathway from nose to brainstem. Cells. (2021) 184:3762–3773.e10. doi: 10.1016/j.cell.2021.05.017
49. McDonald, JL, Smith, PK, Smith, CA, Changli Xue, C, Golianu, B, Cripps, AW, et al. Effect of acupuncture on house dust mite specific IgE, substance P, and symptoms in persistent allergic rhinitis. Ann Allergy Asthma Immunol. (2016) 116:497–505. doi: 10.1016/j.anai.2016.04.002
50. Van Gerven, L, Alpizar, YA, Wouters, MM, Hox, V, Hauben, E, Jorissen, M, et al. Capsaicin treatment reduces nasal hyperreactivity and transient receptor potential cation channel subfamily V, receptor 1 (TRPV1) overexpression in patients with idiopathic rhinitis. J Allergy Clin Immunol. (2014) 133:1332–9. doi: 10.1016/j.jaci.2013.08.026
51. Van Gerven, L, Alpizar, YA, Steelant, B, Callebaut, I, Kortekaas Krohn, I, Wouters, M, et al. Enhanced chemosensory sensitivity in patients with idiopathic rhinitis and its reversal by nasal capsaicin treatment. J Allergy Clin Immunol. (2017) 140:437–446.e2. doi: 10.1016/j.jaci.2017.03.014
53. Douglas, SD, and Leeman, SE. Neurokinin-1 receptor: functional significance in the immune system in reference to selected infections and inflammation. Ann N Y Acad Sci. (2011) 1217:83–95. doi: 10.1111/j.1749-6632.2010.05826.x
54. Han, PCL, Chen, D, Yang, R, Wang, W, Liu, J, He, S, et al. Upregulated expression of substance P and NK1R in blood monocytes and B cells of patients with allergic rhinitis and asthma. Clin Exp Immunol. (2022) 210:39–52. doi: 10.1093/cei/uxac074
55. Nizam, E, and Erin, N. Differential consequences of neurokinin receptor 1 and 2 antagonists in metastatic breast carcinoma cells; effects independent of substance P. Biomed Pharmacother. (2018) 108:263–70. doi: 10.1016/j.biopha.2018.09.013
56. Sun, W, Yang, F, Zhang, H, Yuan, Q, Ling, S, Wang, Y, et al. Structural insights into neurokinin 3 receptor activation by endogenous and analogue peptide agonists. Cell Discov. (2023) 9:66. doi: 10.1038/s41421-023-00564-w
57. Vander Does, A, Ju, T, Mohsin, N, Chopra, D, and Yosipovitch, G. How to get rid of itching. Pharmacol Ther. (2023) 108355:243. doi: 10.1016/j.pharmthera.2023.108355
58. Kuhn, H, Kolkhir, P, Babina, M, Dull, M, Frischbutter, S, Fok, JS, et al. Mas-related G protein-coupled receptor X2 and its activators in dermatologic allergies. J Allergy Clin Immunol. (2021) 147:456–69. doi: 10.1016/j.jaci.2020.08.027
59. Varricchi, G, Pecoraro, A, Loffredo, S, Poto, R, Rivellese, F, Genovese, A, et al. Heterogeneity of human mast cells with respect to MRGPRX2 receptor expression and function. Front Cell Neurosci. (2019) 13:299. doi: 10.3389/fncel.2019.00299
60. Douglas, SD, and Leeman, SE. Is substance P a nasal epithelial neuropeptide? J Allergy Clin Immunol. (2018) 142:1677. doi: 10.1016/j.jaci.2018.05.034
61. Dinh, QT, Groneberg, DA, Mingomataj, E, Peiser, C, Heppt, W, Dinh, S, et al. Expression of substance P and vanilloid receptor (VR1) in trigeminal sensory neurons projecting to the mouse nasal mucosa. Neuropeptides. (2003) 37:245–50. doi: 10.1016/S0143-4179(03)00065-9
62. Gellrich, D, Pfab, F, Ortiz, M, Binting, S, Brinkhaus, B, and Groger, M. Acupuncture and its effect on cytokine and chemokine profiles in seasonal allergic rhinitis: a preliminary three-armed, randomized, controlled trial. Eur Arch Otorhinolaryngol. (2022) 279:4985–95. doi: 10.1007/s00405-022-07335-5
63. He, M, Qin, W, Qin, Z, and Zhao, C. Acupuncture for allergic rhinitis: a systematic review and meta-analysis. Eur J Med Res. (2022) 27:58. doi: 10.1186/s40001-022-00682-3
64. Ehmer, D, McDuffie, CM, Scurry, WC Jr, McIntyre, JB, Mehendale, NH, Willis, JH, et al. Temperature-controlled radiofrequency Neurolysis for the treatment of rhinitis. Am J Rhinol Allergy. (2022) 36:149–56. doi: 10.1177/19458924211033400
65. Qi, Y, Liu, J, Peng, S, Hou, S, Zhang, M, and Wang, Z. Efficacy of selective Vidian Neurectomy for allergic rhinitis combined with chronic rhinosinusitis. ORL J Otorhinolaryngol Spec. (2021) 83:327–34. doi: 10.1159/000512083
66. Larsson, O, Tengroth, L, Xu, Y, Uddman, R, Kumlien Georen, S, and Cardell, LO. Substance P represents a novel first-line defense mechanism in the nose. J Allergy Clin Immunol. (2018) 141:128–136.e3. doi: 10.1016/j.jaci.2017.01.021
67. Huang, F, Yin, JN, Wang, HB, Liu, SY, and Li, YN. Association of imbalance of effector T cells and regulatory cells with the severity of asthma and allergic rhinitis in children. Allergy Asthma Proc. (2017) 38:70–7. doi: 10.2500/aap.2017.38.4076
68. Perner, C, Flayer, CH, Zhu, X, Aderhold, PA, Dewan, ZNA, Voisin, T, et al. Substance P release by sensory neurons triggers dendritic cell migration and initiates the Type-2 immune response to allergens. Immunity. (2020) 53:1063–77.e7. doi: 10.1016/j.immuni.2020.10.001
69. Morelli, AE, Sumpter, TL, Rojas-Canales, DM, Bandyopadhyay, M, Chen, Z, Tkacheva, O, et al. Neurokinin-1 receptor signaling is required for efficient Ca2+ flux in T-cell-receptor-activated T cells. Cell Rep. (2020) 30:3448–65.e8. doi: 10.1016/j.celrep.2020.02.054
70. Taketani, Y, Marmalidou, A, Dohlman, TH, Singh, RB, Amouzegar, A, Chauhan, SK, et al. Restoration of regulatory T-cell function in dry eye disease by antagonizing substance P/Neurokinin-1 receptor. Am J Pathol. (2020) 190:1859–66. doi: 10.1016/j.ajpath.2020.05.011
71. Moattari, CR, and Granstein, RD. Neuropeptides and neurohormones in immune, inflammatory and cellular responses to ultraviolet radiation. Acta Physiol. (2021) 232:e13644. doi: 10.1111/apha.13644
72. Mehta, D, and Granstein, RD. Immunoregulatory effects of neuropeptides on endothelial cells: relevance to dermatological disorders. Dermatology. (2019) 235:175–86. doi: 10.1159/000496538
73. McCary, C, Tancowny, BP, Catalli, A, Grammer, LC, Harris, KE, Schleimer, RP, et al. Substance P downregulates expression of the high affinity IgE receptor (FcepsilonRI) by human mast cells. J Neuroimmunol. (2010) 220:17–24. doi: 10.1016/j.jneuroim.2009.12.006
74. Thapaliya, M, Chompunud Na Ayudhya, C, Amponnawarat, A, Roy, S, and Ali, H. Mast cell-specific MRGPRX2: a key modulator of neuro-immune interaction in allergic diseases. Curr Allergy Asthma Rep. (2021) 21:5. doi: 10.1007/s11882-020-00979-5
75. Azimi, E, Reddy, VB, Pereira, PJS, Talbot, S, Woolf, CJ, and Lerner, EA. Substance P activates mas-related G protein-coupled receptors to induce itch. J Allergy Clin Immunol. (2017) 140:447–453.e3. doi: 10.1016/j.jaci.2016.12.980
76. Cop, N, Ebo, DG, Bridts, CH, Elst, J, Hagendorens, MM, Mertens, C, et al. Influence of IL-6, IL-33, and TNF-alpha on human mast cell activation: lessons from single cell analysis by flow cytometry. Cytometry B Clin Cytom. (2018) 94:405–11. doi: 10.1002/cyto.b.21547
77. Yokota, E, Kuyama, S, Ogawa, M, and Kamei, C. Substance P is involved in the effect of histamine H3 receptor agonist, Sch 50971 on nasal allergic symptoms in mice. Int Immunopharmacol. (2008) 8:1083–8. doi: 10.1016/j.intimp.2008.03.018
78. Larsson, O, Sunnergren, O, Bachert, C, Kumlien Georen, S, and Cardell, LO. The SP-TLR axis, which locally primes the nasal mucosa, is impeded in patients with allergic rhinitis. Clin Transl Allergy. (2021) 11:e12009. doi: 10.1002/clt2.12009
79. Verma, AK, Manohar, M, Upparahalli Venkateshaiah, S, and Mishra, A. Neuroendocrine cells derived chemokine vasoactive intestinal polypeptide (VIP) in allergic diseases. Cytokine Growth Factor Rev. (2017) 38:37–48. doi: 10.1016/j.cytogfr.2017.09.002
80. Gonzalez-Rey, E, Anderson, P, and Delgado, M. Emerging roles of vasoactive intestinal peptide: a new approach for autoimmune therapy. Ann Rheum Dis. (2007) 66:iii70. doi: 10.1136/ard.2007.078519
81. Fischer, A, Wussow, A, Cryer, A, Schmeck, B, Noga, O, Zweng, M, et al. Neuronal plasticity in persistent perennial allergic rhinitis. J Occup Environ Med. (2005) 47:20–5. doi: 10.1097/01.jom.0000150238.77663.49
82. Kim, DH, Park, IH, Cho, JS, Lee, YM, Choi, H, and Lee, HM. Alterations of vasoactive intestinal polypeptide receptors in allergic rhinitis. Am J Rhinol Allergy. (2011) 25:e44–7. doi: 10.2500/ajra.2011.25.3568
83. El-Shazly, AE, Begon, DY, Kustermans, G, Arafa, M, Dortu, E, Henket, M, et al. Novel association between vasoactive intestinal peptide and CRTH2 receptor in recruiting eosinophils: a possible biochemical mechanism for allergic eosinophilic inflammation of the airways. J Biol Chem. (2013) 288:1374–84. doi: 10.1074/jbc.M112.422675
84. Laburthe, M, Couvineau, A, and Tan, V. Class II G protein-coupled receptors for VIP and PACAP: structure, models of activation and pharmacology. Peptides. (2007) 28:1631–9. doi: 10.1016/j.peptides.2007.04.026
85. Walker, KBSM, Valone, FH, Harkonen, WS, Frick, OL, Scriven, KH, Ratnoff, WD, et al. Distinctive patterns of release of neuroendocrine peptides after nasal challenge of allergic subjects with ryegrass antigen. J Clin Immunol. (1988) 8:108–13.
86. Mosimann, BLWM, Hohman, RJ, Goldrich, MS, Kaulbach, HC, and Kaliner, MA. Substance P, calcitonin gene-related peptide, and vasoactive intestinal peptide increase in nasal secretions after allergen challenge in atopic patients.Pdf. Allergy. Clin Immunol. (1993) 92:95–104.
87. Sun, HMY, An, S, and Wang, Z. Altered gene expression signatures by calcitonin gene-related peptide promoted mast cell activity in the colon of stress-induced visceral hyperalgesia mice.Pdf. Neurogastroenterol Motil. (2021) 33:e14073. doi: 10.1111/nmo.14073
88. Iyengar, S, Johnson, KW, Ossipov, MH, and Aurora, SK. CGRP and the trigeminal system in migraine. Headache. (2019) 59:659–81. doi: 10.1111/head.13529
89. Mikami, N, Watanabe, K, Hashimoto, N, Miyagi, Y, Sueda, K, Fukada, S-i, et al. Calcitonin gene-related peptide enhances experimental autoimmune encephalomyelitis by promoting Th17-cell functions. Int Immunol. (2012) 24:681–91. doi: 10.1093/intimm/dxs075
90. Ike, EKT, Takahashi, K, Miyasaka, T, and Takahashi, T. Calcitonin gene-related peptide receptor antagonist suppresses allergic asthma responses via downregulation of group 2 innate lymphoid cells in mice. Int Immunopharmacol. (2023) 122:110608. doi: 10.1016/j.intimp.2023.110608
91. PWD, S, Xu, J, Zhang, Y, Lee, J, Van Dyken, S, Lashua, A, et al. Pulmonary neuroendocrine cells amplify allergic asthma responses.Pdf. Science. (2018) 360:6393. doi: 10.1126/science.aan8546
92. Jowett, GM, and Neves, JF. Commentary: neuronal regulation of type 2 innate lymphoid cells via neuromedin U. Front Pharmacol. (2018) 9:230. doi: 10.3389/fphar.2018.00230
93. Tsukada, A, Takata, K, Takano, S, Ohashi, Y, Mukai, M, Aikawa, J, et al. Increased NMUR1 expression in mast cells in the synovial membrane of obese osteoarthritis patients. Int J Mol Sci. (2022) 23:1237. doi: 10.3390/ijms231911237
94. Moriyama, M, Sato, T, Inoue, H, Fukuyama, S, Teranishi, H, Kangawa, K, et al. The neuropeptide neuromedin U promotes inflammation by direct activation of mast cells. J Exp Med. (2005) 202:217–24. doi: 10.1084/jem.20050248
95. Cardoso, V, Chesne, J, Ribeiro, H, Garcia-Cassani, B, Carvalho, T, Bouchery, T, et al. Neuronal regulation of type 2 innate lymphoid cells via neuromedin U. Nature. (2017) 549:277–81. doi: 10.1038/nature23469
96. Klose, CSN, Mahlakoiv, T, Moeller, JB, Rankin, LC, Flamar, AL, Kabata, H, et al. The neuropeptide neuromedin U stimulates innate lymphoid cells and type 2 inflammation. Nature. (2017) 549:282–6. doi: 10.1038/nature23676
97. Wallrapp, A, Riesenfeld, SJ, Burkett, PR, Abdulnour, RE, Nyman, J, Dionne, D, et al. The neuropeptide NMU amplifies ILC2-driven allergic lung inflammation. Nature. (2017) 549:351–6. doi: 10.1038/nature24029
98. Nagashima, H, Mahlakoiv, T, Shih, HY, Davis, FP, Meylan, F, Huang, Y, et al. Neuropeptide CGRP limits group 2 innate lymphoid cell responses and constrains type 2 inflammation. Immunity. (2019) 51:682–695.e6. doi: 10.1016/j.immuni.2019.06.009
99. Qi, X, Zhuo, H, Wang, Y, Ren, F, Xue, J, An, Y, et al. Neuromedin U induces activation of peripheral group 2 innate lymphoid cells through the ERK pathway in allergic rhinitis patients. Int Arch Allergy Immunol. (2023) 184:1–11. doi: 10.1159/000526271
100. Matsuo, Y, Yanase, Y, Irifuku, R, Takahagi, S, Mihara, S, Ishii, K, et al. Neuromedin U directly induces degranulation of skin mast cells, presumably via MRGPRX2. Allergy. (2018) 73:2256–60. doi: 10.1111/all.13555
101. Nockher, WA, and Renz, H. Neurotrophins in inflammatory lung diseases: modulators of cell differentiation and neuroimmune interactions. Cytokine Growth Factor Rev. (2003) 14:559–78. doi: 10.1016/S1359-6101(03)00071-6
102. Raap, U, and Braunstahl, GJ. The role of neurotrophins in the pathophysiology of allergic rhinitis. Curr Opin Allergy Clin Immunol. (2010) 10:8–13. doi: 10.1097/ACI.0b013e328334f5de
103. Manti, S, Brown, P, Perez, MK, and Piedimonte, G. The role of Neurotrophins in inflammation and allergy. Vitam Horm. (2017) 104:313–41. doi: 10.1016/bs.vh.2016.10.010
104. Bresciani, M, Lalibertè, F, Lalibertè, MF, Gramiccioni, C, and Bonini, S. Nerve growth factor localization in the nasal mucosa of patients with persistent allergic rhinitis. Allergy. (2009) 64:112–7. doi: 10.1111/j.1398-9995.2008.01831.x
105. Vasko, MR, Habashy Malty, R, Guo, C, Duarte, DB, Zhang, Y, and Nicol, GD. Nerve growth factor mediates a switch in intracellular signaling for PGE2-induced sensitization of sensory neurons from protein kinase a to Epac. PLoS One. (2014) 9:e104529. doi: 10.1371/journal.pone.0104529
106. Meng, X, Qian, X, Ding, X, Wang, W, Yin, X, Zhuang, G, et al. Eosinophils regulate intra-adipose axonal plasticity. Proc Natl Acad Sci U S A. (2022) 119:1119. doi: 10.1073/pnas.2112281119
107. Huh, JR, and Veiga-Fernandes, H. Neuroimmune circuits in inter-organ communication. Nat Rev Immunol. (2020) 20:217–28. doi: 10.1038/s41577-019-0247-z
108. Veiga-Fernandes, H, and Pachnis, V. Neuroimmune regulation during intestinal development and homeostasis. Nat Immunol. (2017) 18:116–22. doi: 10.1038/ni.3634
109. Thornton, MA, Akasheh, N, Walsh, MT, Moloney, M, Sheahan, PO, Smyth, CM, et al. Eosinophil recruitment to nasal nerves after allergen challenge in allergic rhinitis. Clin Immunol. (2013) 147:50–7. doi: 10.1016/j.clim.2013.02.008
110. Vliagoftis, H, and Befus, AD. Rapidly changing perspectives about mast cells at mucosal surfaces. Immunol Rev. (2005) 206:190–203. doi: 10.1111/j.0105-2896.2005.00279.x
111. Abraham, SN, and St John, AL. Mast cell-orchestrated immunity to pathogens. Nat Rev Immunol. (2010) 10:440–52. doi: 10.1038/nri2782
112. Molfetta, R, Lecce, M, Quatrini, L, Caracciolo, G, Digiacomo, L, Masuelli, L, et al. Immune complexes exposed on mast cell-derived nanovesicles amplify allergic inflammation. Allergy. (2020) 75:1260–3. doi: 10.1111/all.14103
113. Galli, SJ, and Tsai, M. IgE and mast cells in allergic disease. Nat Med. (2012) 18:693–704. doi: 10.1038/nm.2755
114. Reber, LL, Sibilano, R, Mukai, K, and Galli, SJ. Potential effector and immunoregulatory functions of mast cells in mucosal immunity. Mucosal Immunol. (2015) 8:444–63. doi: 10.1038/mi.2014.131
115. Subramanian, H, Gupta, K, Lee, D, Bayir, AK, Ahn, H, and Ali, H. beta-Defensins activate human mast cells via mas-related gene X2. J Immunol. (2013) 191:345–52. doi: 10.4049/jimmunol.1300023
116. Kumar, M, Duraisamy, K, and Chow, BK. Unlocking the non-IgE-mediated Pseudo-allergic reaction puzzle with mas-related G-protein coupled receptor member X2 (MRGPRX2). Cells. (2021) 10:1033. doi: 10.3390/cells10051033
117. Maintz, L, Wardelmann, E, Walgenbach, K, Fimmers, R, Bieber, T, Raap, U, et al. Neuropeptide blood levels correlate with mast cell load in patients with mastocytosis. Allergy. (2011) 66:862–9. doi: 10.1111/j.1398-9995.2011.02550.x
118. Forsythe, P . Mast cells in Neuroimmune interactions. Trends Neurosci. (2019) 42:43–55. doi: 10.1016/j.tins.2018.09.006
119. Magadmi, R, Meszaros, J, Damanhouri, ZA, and Seward, EP. Secretion of mast cell inflammatory mediators is enhanced by CADM1-dependent adhesion to sensory neurons. Front Cell Neurosci. (2019) 13:262. doi: 10.3389/fncel.2019.00262
120. Wilhelm, M, Silver, R, and Silverman, AJ. Central nervous system neurons acquire mast cell products via transgranulation. Eur J Neurosci. (2005) 22:2238–48. doi: 10.1111/j.1460-9568.2005.04429.x
121. Tatemoto, K, Nozaki, Y, Tsuda, R, Konno, S, Tomura, K, Furuno, M, et al. Immunoglobulin E-independent activation of mast cell is mediated by Mrg receptors. Biochem Biophys Res Commun. (2006) 349:1322–8. doi: 10.1016/j.bbrc.2006.08.177
122. Wedi, B, Gehring, M, and Kapp, A. The pseudoallergen receptor MRGPRX2 on peripheral blood basophils and eosinophils: expression and function. Allergy. (2020) 75:2229–42. doi: 10.1111/all.14213
123. Dinh, QT, Cryer, A, Dinh, S, Trevisani, M, Georgiewa, P, Chung, F, et al. Protease-activated receptor 2 expression in trigeminal neurons innervating the rat nasal mucosa. Neuropeptides. (2005) 39:461–6. doi: 10.1016/j.npep.2005.07.003
124. Le, DD, Schmit, D, Heck, S, Omlor, AJ, Sester, M, Herr, C, et al. Increase of mast cell-nerve association and neuropeptide receptor expression on mast cells in perennial allergic rhinitis. Neuroimmunomodulation. (2016) 23:261–70. doi: 10.1159/000453068
125. Toyoshima, S, and Okayama, Y. Neuro-allergology: mast cell-nerve cross-talk. Allergol Int. (2022) 71:288–93. doi: 10.1016/j.alit.2022.04.002
126. Morita, H, Moro, K, and Koyasu, S. Innate lymphoid cells in allergic and nonallergic inflammation. J Allergy Clin Immunol. (2016) 138:1253–64. doi: 10.1016/j.jaci.2016.09.011
127. Irie, M, Sasahara, K, Artis, D, and Kabata, H. Current overview of the role of neuropeptides in ILC2s and future directions. Allergol Int. (2022) 71:294–300. doi: 10.1016/j.alit.2022.03.002
128. Inclan-Rico, JM, Ponessa, JJ, Valero-Pacheco, N, Hernandez, CM, Sy, CB, Lemenze, AD, et al. Basophils prime group 2 innate lymphoid cells for neuropeptide-mediated inhibition. Nat Immunol. (2020) 21:1181–93. doi: 10.1038/s41590-020-0753-y
129. Galle-Treger, L, Suzuki, Y, Patel, N, Sankaranarayanan, I, Aron, JL, Maazi, H, et al. Nicotinic acetylcholine receptor agonist attenuates ILC2-dependent airway hyperreactivity. Nat Commun. (2016) 7:13202. doi: 10.1038/ncomms13202
130. Drake, MG, Lebold, KM, Roth-Carter, QR, Pincus, AB, Blum, ED, Proskocil, BJ, et al. Eosinophil and airway nerve interactions in asthma. J Leukoc Biol. (2018) 104:61–7. doi: 10.1002/JLB.3MR1117-426R
131. Fryer, AD . Neuronal eotaxin and the effects of ccr3 antagonist on airway hyperreactivity and M2 receptor dysfunction. J Clin Investig. (2005) 116:228–36. doi: 10.1172/JCI25423
132. Li, P, Wang, N, Kai, L, Si, J, and Wang, Z. Chronic intranasal corticosteroid treatment induces degeneration of olfactory sensory neurons in normal and allergic rhinitis mice. Int Forum Allergy Rhinol. (2023) 13:1889–905. doi: 10.1002/alr.23142
133. Miyake, K, and Karasuyama, H. Emerging roles of basophils in allergic inflammation. Allergol Int. (2017) 66:382–91. doi: 10.1016/j.alit.2017.04.007
134. Siracusa, MC, Kim, BS, Spergel, JM, and Artis, D. Basophils and allergic inflammation. J Allergy Clin Immunol. (2013) 132:789–801. doi: 10.1016/j.jaci.2013.07.046
135. Wang, F, Trier, AM, Li, F, Kim, S, Chen, Z, Chai, JN, et al. A basophil-neuronal axis promotes itch. Cells. (2021) 184:422–440.e17. doi: 10.1016/j.cell.2020.12.033
136. An, J, Lee, JH, Won, HK, Kang, Y, Song, WJ, Kwon, HS, et al. Clinical significance of serum MRGPRX2 as a new biomarker in allergic asthma. Allergy. (2020) 75:959–62. doi: 10.1111/all.14084
137. Quan, PL, Sabate-Bresco, M, Guo, Y, Martin, M, and Gastaminza, G. The multifaceted mas-related G protein-coupled receptor member X2 in allergic diseases and beyond. Int J Mol Sci. (2021) 22:4421. doi: 10.3390/ijms22094421
138. Plum, T, Wang, X, Rettel, M, Krijgsveld, J, Feyerabend, TB, and Rodewald, HR. Human mast cell proteome reveals unique lineage, putative functions, and structural basis for cell ablation. Immunity. (2020) 52:404–416.e5. doi: 10.1016/j.immuni.2020.01.012
139. Babina, M, Guhl, S, Artuc, M, and Zuberbier, T. Allergic FcepsilonRI- and pseudo-allergic MRGPRX2-triggered mast cell activation routes are independent and inversely regulated by SCF. Allergy. (2018) 73:256–60. doi: 10.1111/all.13301
140. Manorak, W, Idahosa, C, Gupta, K, Roy, S, Panettieri, R Jr, and Ali, H. Upregulation of mas-related G protein coupled receptor X2 in asthmatic lung mast cells and its activation by the novel neuropeptide hemokinin-1. Respir Res. (2018) 19:1. doi: 10.1186/s12931-017-0698-3
141. Cao, TBT, Cha, HY, Yang, EM, and Ye, YM. Elevated MRGPRX2 levels related to disease severity in patients with chronic spontaneous Urticaria. Allergy, Asthma Immunol Res. (2021) 13:498–506. doi: 10.4168/aair.2021.13.3.498
142. Ai, J, Xie, Z, Qing, X, Li, W, Liu, H, Wang, T, et al. Clinical effect of endoscopic Vidian Neurectomy on bronchial asthma outcomes in patients with coexisting refractory allergic rhinitis and asthma. Am J Rhinol Allergy. (2018) 32:139–46. doi: 10.1177/1945892418764964
143. Wang, N, Wang, J, Zhang, Y, Zeng, Y, Hu, S, Bai, H, et al. Imperatorin ameliorates mast cell-mediated allergic airway inflammation by inhibiting MRGPRX2 and CamKII/ERK signaling pathway. Biochem Pharmacol. (2021) 184:114401. doi: 10.1016/j.bcp.2020.114401
144. Aguilera-Lizarraga, J, Florens, MV, Van Brussel, T, Clevers, E, Van Oudenhove, L, Lambrechts, D, et al. Expression of immune-related genes in rectum and colon descendens of irritable bowel syndrome patients is unrelated to clinical symptoms. Neurogastroenterol Motil. (2019) 31:e13579. doi: 10.1111/nmo.13579
145. Subramanian, H, Gupta, K, Guo, Q, Price, R, and Ali, H. Mas-related gene X2 (MrgX2) is a novel G protein-coupled receptor for the antimicrobial peptide LL-37 in human mast cells: resistance to receptor phosphorylation, desensitization, and internalization. J Biol Chem. (2011) 286:44739–49. doi: 10.1074/jbc.M111.277152
146. Nanda, MK, LeMasters, GK, Levin, L, Rothenberg, ME, Assa'ad, AH, Newman, N, et al. Allergic diseases and internalizing behaviors in early childhood. Pediatrics. (2016) 137:1922. doi: 10.1542/peds.2015-1922
147. Tonelli, LH, Katz, M, Kovacsics, CE, Gould, TD, Joppy, B, Hoshino, A, et al. Allergic rhinitis induces anxiety-like behavior and altered social interaction in rodents. Brain Behav Immun. (2009) 23:784–93. doi: 10.1016/j.bbi.2009.02.017
148. Costa-Pinto, FA, Basso, AS, De Sa-Rocha, LC, Britto, LR, Russo, M, and Palermo-Neto, J. Neural correlates of IgE-mediated allergy. Ann N Y Acad Sci. (2006) 1088:116–31. doi: 10.1196/annals.1366.028
149. Yang, S, Wu, J, Zhang, Q, Li, X, Liu, D, Zeng, B, et al. Allergic rhinitis in rats is associated with an inflammatory response of the Hippocampus. Behav Neurol. (2018) 2018:1–7. doi: 10.1155/2018/8750464
150. Dinarello, CA . Immunological and inflammatory functions of the interleukin-1 family. Annu Rev Immunol. (2009) 27:519–50. doi: 10.1146/annurev.immunol.021908.132612
151. Kuida, KLJ, Ku, G, Harding, MW, Livingston, DJ, Su, MS, and Flavell, RA. Altered cytokine export and apoptosis in mice deficient in Interleukin-1, converting enzyme. Science. (1995) 267:2000–3.
152. Kim, HY, Jee, H, Yeom, JH, Jeong, HJ, and Kim, HM. The ameliorative effect of AST2017-01 in an ovalbumin-induced allergic rhinitis animal model. Inflamm Res. (2019) 68:387–95. doi: 10.1007/s00011-019-01226-y
153. Bergougnan, C, Dittlein, DC, Hummer, E, Riepl, R, Eisenbart, S, Bock, D, et al. Physical and immunological barrier of human primary nasal epithelial cells from non-allergic and allergic donors. World Allergy Organ J. (2020) 13:100109. doi: 10.1016/j.waojou.2020.100109
154. Lang, UE, Anders, D, Danker-Hopfe, H, and Hellweg, R. Measurement of nerve growth factor serum concentration in a psychologically stressful situation in men. Stress. (2004) 7:39–42. doi: 10.1080/10253890310001638145
155. Cortes, A, Castillo, A, and Sciaraffia, A. Food allergy: Children's symptom levels are associated with mothers' psycho-socio-economic variables. J Psychosom Res. (2018) 104:48–54. doi: 10.1016/j.jpsychores.2017.11.009
156. Engel, ML, Pike, MR, Cohen, MF, Dunlop, AL, Corwin, EJ, Pearce, BD, et al. Intergenerational impacts of maternal stress on early childhood atopy in black Americans. J Pediatr Psychol. (2021) 46:891–901. doi: 10.1093/jpepsy/jsab038
157. Li, S, Cheng, J, Yang, J, Zhao, Y, Zhu, Z, Zhao, C, et al. Efficacy of posterior nasal neurectomy for allergic rhinitis combined with chronic rhinosinusitis with nasal polyps. Acta Otolaryngol. (2019) 139:890–4. doi: 10.1080/00016489.2019.1654132
Keywords: allergic rhinitis (AR), neuroimmune communication, neuropeptides, neurogenic inflammation, nasal hyperresponsiveness (NHR), neuroimmune cell units
Citation: Zhou Y, Chen R, Kong L, Sun Y and Deng J (2023) Neuroimmune communication in allergic rhinitis. Front. Neurol. 14:1282130. doi: 10.3389/fneur.2023.1282130
Edited by:
Wassim Elyaman, Columbia University, United StatesReviewed by:
George N. Konstantinou, 424 General Military Hospital, GreeceTina Sumpter, University of Pittsburgh, United States
Copyright © 2023 Zhou, Chen, Kong, Sun and Deng. This is an open-access article distributed under the terms of the Creative Commons Attribution License (CC BY). The use, distribution or reproduction in other forums is permitted, provided the original author(s) and the copyright owner(s) are credited and that the original publication in this journal is cited, in accordance with accepted academic practice. No use, distribution or reproduction is permitted which does not comply with these terms.
*Correspondence: Jing Deng, dj30590471@163.com