- 1Graduate School of Beijing University of Chinese Medicine, Beijing, China
- 2Department of Neurology, China-Japan Friendship Hospital, Beijing, China
- 3Department of Neurology, Peking University People's Hospital, Beijing, China
Introduction: This study aimed to study the therapeutic effects of ofatumumab in patients with myasthenia gravis (MG) in addition to the immunomodulatory effects on peripheral follicular T helper (Tfh) cells and T helper type 17 (Th17) cells.
Methods: Thirty-one patients with anti-acetylcholine receptor (AChR) antibody-positive MG were included in this study. At weeks 0, 1, 2, and 4, an initial dose of 20 mg of ofatumumab was injected subcutaneously, with a 2-month follow-up after completing this first cycle. At baseline, 1 month, and 3 months, we assessed the Quantitative MG (QMG), 15-item MG-Quality of Life (MG-QOL15), and MG-Activities of Daily Living (MG-ADL) scales and measured the frequencies of Tfh, Th17, and B cells and the levels of anti-AChR antibody, IL-6, IL-21, and IL-17 in the peripheral blood.
Results: At 1 month and 3 months, the QMG, MG-QOL15, and MG-ADL scores were all significantly reduced. At 3 months, doses of prednisone were reduced by an average of 37%. Decreased frequencies of Tfh and Th17 cells, depletion of B cells, and reduced levels of IL-6, IL-21, and IL-17 were all observed at 1 month or 3 months.
Discussion: Therefore, the therapeutic effect of ofatumumab could be detected after one cycle of treatment, which was maintained for 2 months. The immunomodulatory effect of ofatumumab during the observation period may involve depletion of B cells, reduction of Tfh and Th17 cells frequencies, and reduced levels of IL-6, IL-21, and IL-17. The findings provide novel data for the potential application of ofatumumab in MG.
1 Introduction
Myasthenia gravis (MG) is a chronic organ-specific autoimmune disease caused by autoantibodies directed against components of the neuromuscular junction. Acetylcholine receptor (AChR) autoantibodies are observed in approximately 85% of MG patients (1). MG is clinically characterized by partial or generalized muscle weakness and fatigue, aggravated with activity and relieved with rest (2). MG is a rare disease, with an estimated incidence of 0.3 to 2.8 per 100,000 people and a global prevalence of 700,000 (3). MG can affect daily activities, and if untreated, approximately 20% of patients will suffer exacerbated muscle weakness and respiratory failure.
Although MG is an antibody-mediated autoimmune disease, antibody synthesis requires the intervention of cellular immunity. The peripheral immune response, which is regulated by autoreactive T cells and B cells that have escaped central tolerance, is the primary pathogenesis of MG (4, 5). CD4+ T cells serve as the main driver in the immune pathogenesis of MG; the redistribution of CD4+ T cells leads to an increase in the frequency of follicular T helper (Tfh) cells and T helper type 17 (Th17) cells (6, 7). Circulating Tfh cells move between the peripheral blood and lymphoid tissue and are the most potent regulators of humoral immunity (8). Th17 cells contribute to the induction of tissue inflammation in autoimmune diseases (9). The increased frequency of Tfh cells and Th17 cells was reported to significantly exacerbate the development of MG in humans and experimental models (10–12). Autoreactive B cells, coordinated primarily by CD4+ T cells and cytokines, produce anti-AChR autoantibodies at the neuromuscular junction in MG, leading to the loss of AChR function at the postsynaptic membrane, impaired neuromuscular transmission, and clinical symptoms of muscle weakness (13). Therefore, similar to B cells, Tfh cells and Th17 cells also play an important role in the immune mechanism of MG.
At present, there is no consensus on the ideal therapeutic approach for MG. Targeted immunotherapy appears to be the most promising treatment avenue for MG, effectively overcoming the limitations of the currently applied non-specific immunotherapy and potentially inducing remission (14). Ofatumumab is a fully human second-generation anti-CD20 monoclonal antibody for subcutaneous application. It binds to the CD20 molecule on the B-cell surface and induces B-cell depletion through antibody-dependent and complement-dependent cytotoxicity (15). Ofatumumab has been approved for the treatment of relapsing multiple sclerosis (MS) in adults (16). Rituximab, another monoclonal antibody directed against CD20, provided a meaningful and prolonged benefit in >70% of anti-AChR antibody-positive MG patients (17). However, to the best of our knowledge, there is only one brief report regarding the effectiveness of ofatumumab in treating one patient with refractory MG (18).
To investigate the clinical efficacy as well as the immunomodulatory effects of ofatumumab in MG patients, we evaluated several clinical scales and detected the frequency of Tfh cells, Th17 cells, and B cells, in addition to the levels of anti-AChR antibodies and related cytokines in the peripheral blood. The findings will provide a novel idea for applying ofatumumab in MG.
2 Materials and methods
2.1 Patients
Subjects gave their written informed consent, and the research protocol was approved by the ethics committee of Peking University People's Hospital (2023PHB244-001) before the initiation of this study. The study was conducted in accordance with the World Medical Association Declaration of Helsinki.
We collected data from cases who met the following criteria from January 2023 to April 2023 in the Department of Neurology at Peking University People's Hospital. The study flow diagram is shown in Figure 1. The inclusion criteria were as follows: (1) age ≥ 18 years; (2) diagnosis of MG based on the clinical evidence of muscle weakness and fatigue and at least one positive ancillary diagnostic test, including a positive response to the neostigmine test; (3) anti-AChR antibody-positive; (4) patients with Myasthenia Gravis Foundation of America (MGFA) clinical classification I~V (for patients with thymectomy or patients without thymoma, class I indicates weakness only in ocular muscles, class II for mild generalized disease, class III for moderate generalized disease, class IV for severe generalized disease, and class V for a crisis requiring intubation) (19); (5) class II~V patients, who did not respond to multiple immunosuppressive therapies, have intolerable adverse effects, require repeated intravenous immunoglobulin therapy or plasma exchange, have frequent MG crises (20), or have not received any immunosuppressive therapies; (6) class I patients who did not respond to multiple immunosuppressive therapies or have intolerable adverse effects; and (7) no prior treatment with ofatumumab. The exclusion criteria were as follows: (1) the co-presence of MG and malignant tumors, major organ damage, systemic hematological diseases, and recent infections; (2) pregnancy or lactation; and (3) patients who failed to give or comply with informed consent.
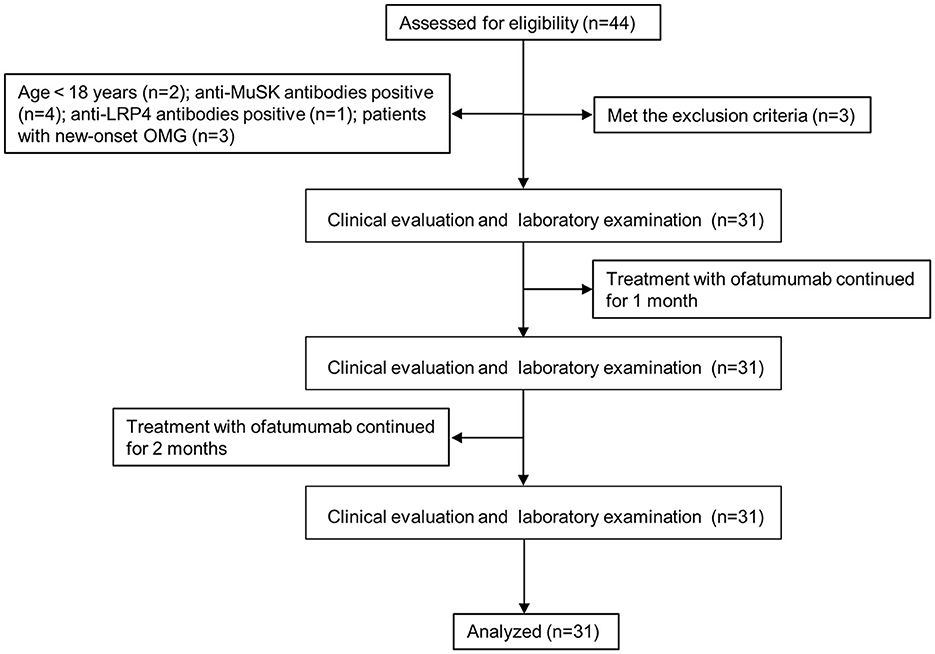
Figure 1. Flow diagram. OMG, ocular myasthenia gravis; MuSK, muscle-specific tyrosine kinase; LRP4, low-density lipoprotein receptor-related protein 4.
2.2 Ofatumumab and other therapies
Before starting ofatumumab treatment, patients were required to discontinue immunosuppressive agents in case of unexpected compromise to the immune system. Patients were allowed to continue taking oral prednisone, and the dose was adjusted according to clinical response during the treatment period. At weeks 0, 1, 2, and 4, an initial dose of 20 mg was injected subcutaneously. Periodic clinical and laboratory assessments were performed after the completion of the first cycle. Patients were permitted to receive a repeat cycle of 20 mg once a month according to assessment results and patient's preference.
2.3 Clinical evaluation
Disease activity was assessed using the Quantitative MG (QMG) scale of the MGFA (19), 15-item MG-Quality of Life (MG-QOL15) scale (21), and MG-Activities of Daily Living (MG-ADL) scale (22) before treatment and at 1 and 3 months after the initiation of ofatumumab treatment.
2.4 Flow cytometry
At 0, 1, and 3 months, heparinized peripheral blood from patients with MG was collected and processed within 24 h. Peripheral blood mononuclear cells (PBMCs) were isolated by Ficoll-Hypaque density gradient centrifugation. PBMCs were stimulated and incubated for 5 h with 50 ng/mL phorbol 12-myristate 13-acetate (PMA; Sigma-Aldrich, Steinheim, Germany) and 500 ng/mL ionomycin (Sigma-Aldrich, Steinheim, Germany) in the presence of 10 μg/mL Brefeldin-A (BFA; BD Biosciences, San Diego, California, USA). The PBMCs were then incubated with the following fluorochrome-conjugated anti-human murine monoclonal antibodies: FITC-CD4, Alexa Fluor 647-CXCR5, Brilliant Violet 510-CD45RA, PE-CF594-CD19, PE-CD16, and PE-CD56 for 30 min at 4°C in the dark to stain the cell surface markers. Next, the cells were fixed and permeabilized for 30 min at room temperature in the dark with the Foxp3 Fixation/Permeabilization Concentrate and Diluent Buffer Set (eBioscience, San Diego, California, USA). PBMCs were then incubated for 30 min in the dark at 4°C with PE-labeled IL-17A antibody for intracellular cytokine staining. All antibodies were purchased from BD Biosciences (Franklin Lakes, New Jersey, USA) and BioLegend (San Diego, California, USA). Tfh cells were defined as CD4+CXCR5+CD45RA-, Th17 cells were defined as CD4+ T cells secreting IL-17A, and B cells were defined as CD19+ and not expressing CD16 and 56. After proper instrument set up, measurements were performed using a FACS Aria II flow cytometer (BD Biosciences, San Jose, California, USA), and FlowJov10 (Tree Star, Woodburn, OR) was applied for data analysis.
2.5 Enzyme-linked immunosorbent assay
The serum was separated from the peripheral blood by centrifugation (3,000 rpm, 10 min). The serum levels of interleukin (IL)-6, IL-21, IL-17, and anti-AChR antibodies in patients with MG were measured using the corresponding ELISA kits (Cusabio, Wuhan, China). The diluted serum and standard were added to each well to bind with the specific antibody for incubation, followed by horseradish peroxidase-labeled streptavidin and chromogenic substrate, and when the sample turned blue, the stop solution was added. The optical density at the absorbance of 450 nm was measured.
2.6 Statistical analysis
The statistical analysis was conducted using SPSS 26.0 software (SPSS Inc., Chicago, IL, USA) and GraphPad Prism 5 software (GraphPad Software Inc., La Jolla, CA, USA). Continuous variables were presented as mean ± standard deviation (SD) and categorical data were presented as percentages. To determine whether the data used for comparison between groups followed the normal distribution, the Shapiro–Wilk test was performed. The Student's t-test was used to analyze normally distributed data and the non-parametric Wilcoxon test for non-normally distributed data. A P-value of < 0.05 (two-tailed) was considered to be statistically significant.
3 Results
3.1 Clinical characteristics
In total, 31 subjects were included in the study (17 females and 14 males). The mean subject age was 53.32 ± 14.56 years and the disease duration was 18.42 ± 15.09 months. Ten patients with thymoma had undergone thymectomy. Of the 31 patients included, 24 were patients with refractory MG and seven were patients with new-onset MG who had not received prior treatment for MG. These 24 patients discontinued all other immunosuppressive agents, except prednisone, before the use of ofatumumab. During the study period, all 31 patients received the first cycle of ofatumumab, followed by a 2-month follow-up. Doses of prednisone were reduced by an average of 37% at 3 months in 24 patients, although none were able to free from prednisone entirely. No patients developed MG crises during the study period (Table 1).
3.2 Clinical efficacy of ofatumumab
A significant decrease in the QMG, MG-QOL15, and MG-ADL scores was observed at 1 month of ofatumumab treatment (P < 0.0001), and the scores were maintained at 3 months (P < 0.0001) (Figure 2). In terms of safety, three patients (9.68%) reported mild adverse events during the study period, including one patient with mild fever and two patients with headaches, nasal congestion, and sore throat, all of which resolved on their own. The other patients tolerated the treatment well.
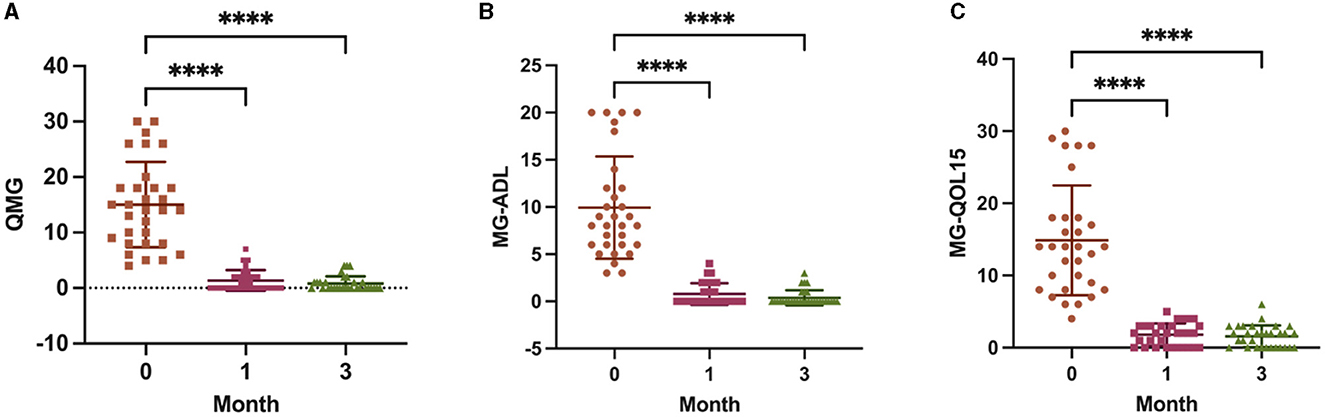
Figure 2. Scores from the Quantitative Myasthenia Gravis (QMG) (A), 15-item MG-Quality of Life (MG-QOL15) (B), and MG-Activities of Daily Living (MG-ADL) (C) assessments at baseline, 1 month, and 3 months. ****P < 0.0001 vs. baseline.
3.3 Ofatumumab reduced the Tfh and Th17 cells frequencies and depleted B cells
The proportion of Tfh cells in CD4+ T cells was unchanged at 1 month of ofatumumab treatment (P = 0.8051) and significantly decreased at 3 months (P = 0.0024) (Figure 3A). However, the proportion of Th17 cells in CD4+ T cells was consecutively and significantly decreased at 1 (P = 0.0002) and 3 months (P < 0.0001) (Figure 3B). Furthermore, B-cell depletion was achieved at both 1 and 3 months (P < 0.0001) (Figure 3C). The anti-AChR antibody titer did not change significantly at 1 (P = 0.7476) and 3 months (P = 0.1350) (Figure 3D).
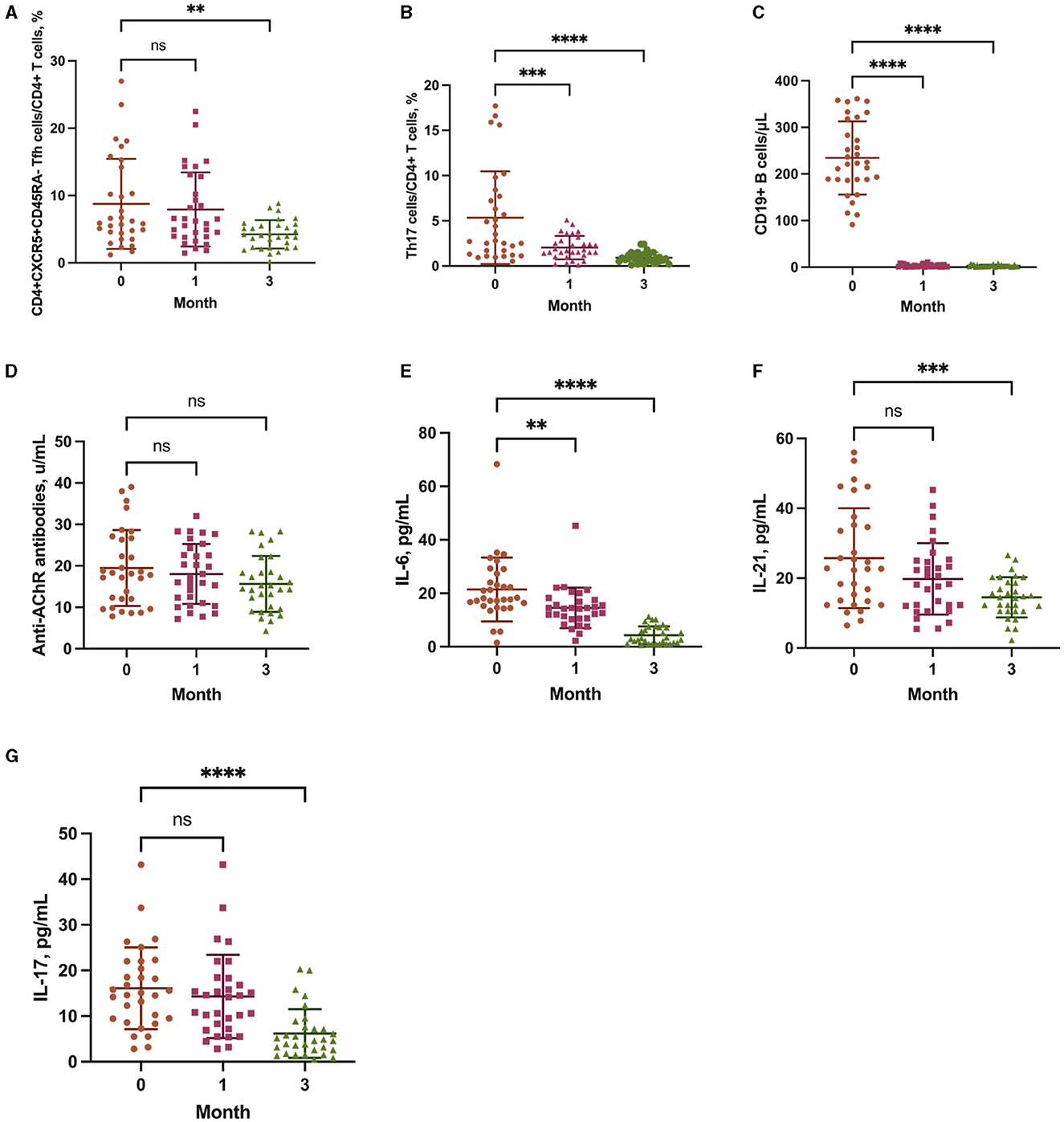
Figure 3. The frequency of CD4+CXCR5+CD45RA follicular T helper (Tfh) cells (A), T helper type 17 (Th17) cells (B), and CD19+ B cells (C), and the levels of anti-AChR antibodies (D), interleukin (IL)-6 (E), IL-21 (F), and IL-17 (G) at baseline, 1 month, and 3 months. **P < 0.01, ***P < 0.001, and ****P < 0.0001 vs. baseline.
3.4 Ofatumumab decreased the levels of IL-6, IL-21, and IL-17
The levels of IL-6 (P = 0.0049), IL-21 (P = 0.0787), and IL-17 (P = 0.6512) were significantly decreased at 1 month of ofatumumab treatment and were consistently decreased at 3 months (IL-6, P < 0.0001; IL-21, P = 0.0002; IL-17, P < 0.0001) (Figures 3E–G).
4 Discussion
The effects of ofatumumab in patients with MG have yet to be explored. In this study, we found that ofatumumab has a therapeutic effect in patients with anti-AChR antibody-positive MG and that the immunomodulatory effect may involve the regulation of Tfh cells and Th17 cells. This study is the first to report the role of ofatumumab in MG, providing evidence for the therapeutic application of ofatumumab in the treatment of MG and a future direction to explore the therapeutic mechanism of ofatumumab in MG.
Only one case report has reported the effectiveness of ofatumumab in a patient with refractory MG after receiving two infusions of ofatumumab (700 mg) 2 weeks apart (18). This dose (700 mg) is relatively large; in the present study, we used a standard dose of 20 mg and followed up with the patients after completing the first cycle (20 mg at weeks 0, 1, 2, and 4). This dosage regimen was consistent with that reported in the recent review on the treatment of relapsing MS with ofatumumab (23). Similarly, the standard protocol of rituximab is 375 mg/m2 weekly for 4 weeks, after which investigators have used varying numbers of cycles with retreatments (17, 24, 25). In addition, many studies have suggested that repeated rituximab infusion, if clinically indicated, should be considered at 6 months after a cycle (17, 26). In the present study, clinical efficacy and B-cell depletion were maintained for 3 months after completing one cycle; however, the reason for this may be that our observation period was only 3 months.
The QMG, MG-QOL15, and MG-ADL are validated outcome measures that can be used to assess the therapeutic effect of interventions in MG clinical trials. QMG is a standardized and reliable scale to quantitatively assess a patient's clinical status and is the primary outcome indicator (27). MG-QOL15 is used to assess patients' health-related quality of life (28). MG-ADL correlates well with the QMG and can be used as a secondary measurement of clinical efficacy (22). In this study, the QMG, MG-QOL15, and MG-ADL scores of patients with MG were all significantly reduced after treatment with ofatumumab. Therefore, these results indicate that ofatumumab has a therapeutic effect in patients with MG; ofatumumab can reduce the severity of the disease and improve the quality of life. Rituximab has been used to treat MG for more than a decade and was thought to be effective in treating refractory MG patients; however, the improvement in the QMG, MG-ADL, or MG-QOL15 scores was often reported after 6 months of treatment (29, 30). Rituximab appeared to be a potentially effective treatment option for new-onset MG, which significantly improved the QMG, MG-ADL, and MG-QOL15 scores; a significant change in the QMG score was even detected after 1 month of treatment (31, 32). In contrast to rituximab, patients enrolled in this study included those with both refractory MG and new-onset MG, with significant reductions in the QMG, MG-ADL, and MG-QOL-15 scores at 1 month of treatment with ofatumumab, although this needs to be validated in a large-scale randomized controlled trial.
Tfh cells are a distinct subset of CD4+ T cells. In the immune response, Tfh cells are specialized helpers of B cells, including promoting the maturation of B cells and inducing the differentiation of B cells into antibody-producing plasma cells (PCs) and long-lived memory B cells. Tfh cells were hypothesized to be a target for preventing B cells from producing autoantibodies against their own antigens (33, 34). IL-21 is mainly secreted by Tfh cells and plays a crucial role in B cells proliferation, PCs differentiation, and antibody secretion (35, 36). A study reported that inhibition of Tfh cells transcription decreased the frequency of Tfh cells and IL-21 production (37). The Tfh cells frequency and IL-21 level were increased in patients with anti-AChR antibody-positive MG and were significantly correlated with antibody titer and disease severity (38, 39). In this study, treatment with ofatumumab significantly reduced the Tfh cells frequency and IL-21 level in patients with anti-AChR antibody-positive MG. These results indicate that the immunoregulatory effect of ofatumumab in MG might involve a reduction in the Tfh cells frequency and IL-21 level.
Th17 cells are highly pro-inflammatory and result in increased chronic inflammation at the MG neuromuscular junction through the release of cytokines, such as IL-17 (7). IL-17 has been shown to be involved in the immune response in various autoimmune diseases, such as MS, rheumatoid arthritis, systemic lupus erythematosus, and MG. The pro-inflammatory factor IL-17 is emerging as an important contributor to exacerbated autoimmunity in MG patients and experimental autoimmune MG animals. Th17 cells and IL-17, as B-cell helpers, can not only directly affect the survival, proliferation, and differentiation of human B cells but also trigger antibody production (40). In anti-AChR antibody-positive MG patients, the Th17 cells frequency and IL-17 level were increased and positively correlated with antibody titer and disease severity (41–43). In this study, ofatumumab significantly decreased the Th17 cells frequency and IL-17 level. Furthermore, ofatumumab also affected the frequency and reactivity of Th17 cells in patients with MS (44, 45).
IL-6 is a pleiotropic inflammatory cytokine. As a regulator of B cells and T cells function, IL-6 promotes T cells activation and B cells proliferation and differentiation (7). Moreover, IL-6 can promote Tfh cells and Th17 cells differentiation (46). The serum level of IL-6 was increased in patients with anti-AChR antibody-positive MG and was correlated with the severity of MG (47). In this study, ofatumumab decreased the IL-6 level in MG patients, which suggests that the immunoregulatory effect of ofatumumab in MG might also involve reducing the IL-6 level.
MG is an autoimmune disease mediated by B cells. Autoantibodies produced by B cells or PCs are thought to be closely related to the pathogenesis of MG (14). In anti-AChR antibody-positive MG patients, the increases in B cells frequency and antibody titers were associated with the exacerbation of MG (48). In this study, B-cell depletion was observed after treatment with ofatumumab; however, no significant change in antibody levels was observed. Ofatumumab has been reported to successfully deplete B cells in the treatment of MS (49, 50). The majority of circulating immunoglobulin was secreted by long-lived PCs lacking CD20 on their surface, including anti-AChR autoantibodies. Specific B cells (CD20+) producing anti-AChR autoantibodies could also be found in the circulation, and their levels were often positively associated with serum autoantibody titers (51). Due to the contribution from AChR-specific B cells sensitive to B-cell depletion and long-lived PCs differing in each patient (52), the change in anti-AChR antibody titers was also variable after B-cell depletion by ofatumumab treatment in patients. The anti-AChR antibody titer showed no significant decrease after statistical analysis, which suggested that the autoreactive PC pool might be more entrenched. In addition, when targeting CD20, the anti-AChR antibody levels did not change notably after treatment with rituximab, while the clinical symptoms were still improved (29). After rituximab treatment, most patients with MG did not show a significant reduction in the anti-AChR antibody level, which was independent of clinical improvement (53); this data supports our results. Accumulated evidence suggests that B-cell depletion therapy has a regulatory effect on T cell subsets. The immunomodulatory effect of rituximab on T cells in patients with MG has been repeatedly reported (30, 54). Furthermore, rituximab or ofatumumab also plays an immunomodulatory role on T cells in other autoimmune diseases, such as MS and rheumatoid arthritis (44, 55, 56). However, the mechanism by which B-cell depletion exerts a regulatory effect on T cells is unknown. The study found that B cells are necessary for the survival of Tfh cells (57). In response to self-antigens, B cells can stimulate the proliferation of Th17 cells and the production of IL-17 by Th17 cells (58). Therefore, the diminished effect of B cells on T cells after B-cell depletion may contribute to the change in T-cell subsets.
Although our study achieved exciting results, only 31 patients were included, and the observation period of 3 months was an impressive shortcoming. Furthermore, non-blinding or other immunosuppressive agents may have affected our results to some extent. For the first time, we have identified the clinical efficacy and partial immunomodulatory mechanism of ofatumumab in patients with MG. This motivates us to observe the long-term efficacy of ofatumumab on MG in a larger cohort of subjects, which may reveal additional effects and reduce the risk of type I statistical errors. This study provides support for conducting randomized controlled prospective studies and animal experiments to further determine the effect of ofatumumab in MG.
5 Conclusion
A significant reduction in the QMG, MG-QOL15, and MG-ADL scores was observed in anti-AChR antibody-positive MG patients after one cycle of treatment with ofatumumab; these decreased scores were maintained for a further 2 months. The immunomodulatory effects of ofatumumab may involve the depletion of B cells, reduction in Tfh and Th17 cells frequencies, and reduced IL-6, IL-21, and IL-17 levels during the observation period of 3 months.
Data availability statement
The raw data supporting the conclusions of this article will be made available by the authors, without undue reservation.
Ethics statement
The studies involving humans were approved by Ethics Committees of Peking University People's Hospital. The studies were conducted in accordance with the local legislation and institutional requirements. The participants provided their written informed consent to participate in this study. Written informed consent was obtained from the individual(s) for the publication of any potentially identifiable images or data included in this article.
Author contributions
SL: Writing—original draft. ZZ: Data curation, Writing—original draft. ZL: Project administration, Writing—review & editing.
Funding
The author(s) declare that financial support was received for the research, authorship, and/or publication of this article. This study was supported by the Peking University People's Hospital Talent Introduction Scientific Research Launch Fund (No. 2022-T-02) and the National Natural Science Foundation of China (No. 81303013).
Conflict of interest
The authors declare that the research was conducted in the absence of any commercial or financial relationships that could be construed as a potential conflict of interest.
Publisher's note
All claims expressed in this article are solely those of the authors and do not necessarily represent those of their affiliated organizations, or those of the publisher, the editors and the reviewers. Any product that may be evaluated in this article, or claim that may be made by its manufacturer, is not guaranteed or endorsed by the publisher.
References
1. Howard JF. Myasthenia gravis: the role of complement at the neuromuscular junction. Ann N Y Acad Sci. (2018) 1412:113–28. doi: 10.1111/nyas.13522
2. Jordan A, Freimer M. Recent advances in understanding and managing myasthenia gravis. F1000Res. (2018) 7:1727. doi: 10.12688/f1000research.15973.1
3. Deenen JC, Horlings CG, Verschuuren JJ, Verbeek AL, van Engelen BG. The epidemiology of neuromuscular disorders: a comprehensive overview of the literature. J Neuromuscul Dis. (2015) 2:73–85. doi: 10.3233/JND-140045
4. Lee S, Ko Y, Kim TJ. Homeostasis and regulation of autoreactive B cells. Cell Mol Immunol. (2020) 17:561–9. doi: 10.1038/s41423-020-0445-4
5. Dominguez-Villar M, Hafler DA. Regulatory T cells in autoimmune disease. Nat Immunol. (2018) 19:665–73. doi: 10.1038/s41590-018-0120-4
6. Yi JS, Guptill JT, Stathopoulos P, Nowak RJ, O'Connor KC. B cells in the pathophysiology of myasthenia gravis. Muscle Nerve. (2018) 57:172–84. doi: 10.1002/mus.25973
7. Uzawa A, Kuwabara S, Suzuki S, Imai T, Murai H, Ozawa Y, et al. Roles of cytokines and T cells in the pathogenesis of myasthenia gravis. Clin Exp Immunol. (2021) 203:366–74. doi: 10.1111/cei.13546
8. Zhang X, Ing S, Fraser A, Chen M, Khan O, Zakem J, et al. Follicular helper T cells: new insights into mechanisms of autoimmune diseases. Ochsner J. (2013) 13:131–9.
9. Korn T, Bettelli E, Oukka M, Kuchroo VK. Il-17 and Th17 cells. Annu Rev Immunol. (2009) 27:485–517. doi: 10.1146/annurev.immunol.021908.132710
10. Conti-Fine BM, Milani M, Wang W. Cd4+ T cells and cytokines in the pathogenesis of acquired myasthenia gravis. Ann N Y Acad Sci. (2008) 1132:193–209. doi: 10.1196/annals.1405.042
11. Cui YZ, Qu SY, Chang LL, Zhao JR, Mu L, Sun B, et al. Enhancement of T follicular helper cell-mediated humoral immunity reponses during development of experimental autoimmune myasthenia gravis. Neurosci Bull. (2019) 35:507–18. doi: 10.1007/s12264-019-00344-1
12. Song J, Yang J, Jing S, Yan C, Huan X, Chen S, et al. Berberine attenuates experimental autoimmune myasthenia gravis via rebalancing the T cell subsets. J Neuroimmunol. (2022) 362:577787. doi: 10.1016/j.jneuroim.2021.577787
13. Yilmaz V, Oflazer P, Aysal F, Parman YG, Direskeneli H, Deymeer F, et al. B cells produce less Il-10, Il-6 and Tnf-α in myasthenia gravis. Autoimmunity. (2015) 48:201–7. doi: 10.3109/08916934.2014.992517
14. Dalakas MC. Immunotherapy in myasthenia gravis in the era of biologics. Nat Rev Neurol. (2019) 15:113–24. doi: 10.1038/s41582-018-0110-z
15. Gasperi C, Stüve O, Hemmer B. B cell-directed therapies in multiple sclerosis. Neurodegener Dis Manag. (2016) 6:37–47. doi: 10.2217/nmt.15.67
16. Rau D, Eichau S, Borriello G, Cerqueira J, Wagner C. Assessment of the treating physicians' first-hand experience with handling and satisfaction of ofatumumab therapy: findings from the peritia survey conducted in Europe. BMC Neurol. (2023) 23:147. doi: 10.1186/s12883-023-03190-x
17. Robeson KR, Kumar A, Keung B, DiCapua DB, Grodinsky E, Patwa HS, et al. Durability of the rituximab response in acetylcholine receptor autoantibody-positive myasthenia gravis. JAMA Neurol. (2017) 74:60–6. doi: 10.1001/jamaneurol.2016.4190
18. Waters MJ, Field D, Ravindran J. Refractory myasthenia gravis successfully treated with ofatumumab. Muscle Nerve. (2019) 60:E45–e7. doi: 10.1002/mus.26707
19. Jaretzki A, Barohn RJ, Ernstoff RM, Kaminski HJ, Keesey JC, Penn AS, et al. Myasthenia gravis: recommendations for clinical research standards task force of the medical scientific advisory board of the myasthenia gravis foundation of America. Ann Thorac Surg. (2000) 70:327–34. doi: 10.1016/s0003-4975(00)01595-2
20. Silvestri NJ, Wolfe GI. Treatment-refractory myasthenia gravis. J Clin Neuromuscul Dis. (2014) 15:167–78. doi: 10.1097/CND.0000000000000034
21. Mullins LL, Carpentier MY, Paul RH, Sanders DB. Disease-specific measure of quality of life for myasthenia gravis. Muscle Nerve. (2008) 38:947–56. doi: 10.1002/mus.21016
22. Wolfe GI, Herbelin L, Nations SP, Foster B, Bryan WW, Barohn RJ. Myasthenia gravis activities of daily living profile. Neurology. (1999) 52:1487–9. doi: 10.1212/WNL.52.7.1487
23. Hauser SL, Kappos L, Bar-Or A, Wiendl H, Paling D, Williams M, et al. The development of ofatumumab, a fully human anti-Cd20 monoclonal antibody for practical use in relapsing multiple sclerosis treatment. Neurol Ther. (2023) 12:1491–515. doi: 10.1007/s40120-023-00518-0
24. Anderson D, Phan C, Johnston WS, Siddiqi ZA. Rituximab in refractory myasthenia gravis: a prospective, open-label study with long-term follow-up. Ann Clin Transl Neurol. (2016) 3:552–5. doi: 10.1002/acn3.314
25. Díaz-Manera J, Martínez-Hernández E, Querol L, Klooster R, Rojas-García R, Suárez-Calvet X, et al. Long-lasting treatment effect of rituximab in musk myasthenia. Neurology. (2012) 78:189–93. doi: 10.1212/WNL.0b013e3182407982
26. Tandan R, Hehir MK, Waheed W, Howard DB. Rituximab treatment of myasthenia gravis: a systematic review. Muscle Nerve. (2017) 56:185–96. doi: 10.1002/mus.25597
27. Barohn RJ, McIntire D, Herbelin L, Wolfe GI, Nations S, Bryan WW. Reliability testing of the quantitative myasthenia gravis score. Ann N Y Acad Sci. (1998) 841:769–72. doi: 10.1111/j.1749-6632.1998.tb11015.x
28. Burns TM, Grouse CK, Conaway MR, Sanders DB. Construct and concurrent validation of the Mg-Qol15 in the practice setting. Muscle Nerve. (2010) 41:219–26. doi: 10.1002/mus.21609
29. Jing S, Song Y, Song J, Pang S, Quan C, Zhou L, et al. Responsiveness to low-dose rituximab in refractory generalized myasthenia gravis. J Neuroimmunol. (2017) 311:14–21. doi: 10.1016/j.jneuroim.2017.05.021
30. Jing S, Lu J, Song J, Luo S, Zhou L, Quan C, et al. Effect of low-dose rituximab treatment on T- and B-cell lymphocyte imbalance in refractory myasthenia gravis. J Neuroimmunol. (2019) 332:216–23. doi: 10.1016/j.jneuroim.2019.05.004
31. Du Y, Li C, Hao YF, Zhao C, Yan Q, Yao D, et al. Individualized regimen of low-dose rituximab monotherapy for new-onset achr-positive generalized myasthenia gravis. J Neurol. (2022) 269:4229–40. doi: 10.1007/s00415-022-11048-4
32. Mathew T, Thomas K, K John S, Venkatesh S, Nadig R, Badachi S, et al. Effective early treatment of achr antibody-positive myasthenia gravis with rituximab; the experience from a neuroimmunology clinic in a developing country. J Cent Nerv Syst Dis. (2021) 13:11795735211016080. doi: 10.1177/11795735211016080
33. Fujii Y, Lindstrom J. Regulation of antibody production by helper T cell clones in experimental autoimmune myasthenia gravis. J Immunol. (1988) 141:3361–9. doi: 10.4049/jimmunol.141.10.3361
34. Ma CS, Deenick EK. Human T follicular helper (Tfh) cells and disease. Immunol Cell Biol. (2014) 92:64–71. doi: 10.1038/icb.2013.55
35. Peluso I, Fantini MC, Fina D, Caruso R, Boirivant M, MacDonald TT, et al. Il-21 counteracts the regulatory T cell-mediated suppression of human Cd4+ T lymphocytes. J Immunol. (2007) 178:732–9. doi: 10.4049/jimmunol.178.2.732
36. Kuchen S, Robbins R, Sims GP, Sheng C, Phillips TM, Lipsky PE, et al. Essential role of Il-21 in B cell activation, expansion, and plasma cell generation during Cd4+ T Cell-B cell collaboration. J Immunol. (2007) 179:5886–96. doi: 10.4049/jimmunol.179.9.5886
37. Xin N, Fu L, Shao Z, Guo M, Zhang X, Zhang Y, et al. RNA interference targeting Bcl-6 ameliorates experimental autoimmune myasthenia gravis in mice. Mol Cell Neurosci. (2014) 58:85–94. doi: 10.1016/j.mcn.2013.12.006
38. Zhang CJ, Gong Y, Zhu W, Qi Y, Yang CS, Fu Y, et al. Augmentation of circulating follicular helper T cells and their impact on autoreactive B cells in myasthenia gravis. J Immunol. (2016) 197:2610–7. doi: 10.4049/jimmunol.1500725
39. Zhang X, Liu S, Chang T, Xu J, Zhang C, Tian F, et al. Intrathymic Tfh/B cells interaction leads to ectopic GCS formation and anti-achr antibody production: central role in triggering MG occurrence. Mol Neurobiol. (2016) 53:120–31. doi: 10.1007/s12035-014-8985-1
40. Mitsdoerffer M, Lee Y, Jäger A, Kim HJ, Korn T, Kolls JK, et al. Proinflammatory T helper type 17 cells are effective B-cell helpers. Proc Natl Acad Sci U S A. (2010) 107:14292–7. doi: 10.1073/pnas.1009234107
41. Wang Z, Wang W, Chen Y, Wei D. T Helper type 17 cells expand in patients with myasthenia-associated thymoma. Scand J Immunol. (2012) 76:54–61. doi: 10.1111/j.1365-3083.2012.02703.x
42. Yi JS, Russo MA, Raja S, Massey JM, Juel VC, Shin J, et al. Inhibition of the transcription factor ror-Γ reduces pathogenic Th17 cells in acetylcholine receptor antibody positive myasthenia gravis. Exp Neurol. (2020) 325:113146. doi: 10.1016/j.expneurol.2019.113146
43. Roche JC, Capablo JL, Larrad L, Gervas-Arruga J, Ara JR, Sánchez A, et al. Increased serum interleukin-17 levels in patients with myasthenia gravis. Muscle Nerve. (2011) 44:278–80. doi: 10.1002/mus.22070
44. von Essen MR, Hansen RH, Højgaard C, Ammitzbøll C, Wiendl H, Sellebjerg F. Ofatumumab modulates inflammatory T cell responses and migratory potential in patients with multiple sclerosis. Neurol Neuroimmunol Neuroinflamm. (2022) 9:e200004. doi: 10.1212/NXI.0000000000200004
45. Theil D, Smith P, Huck C, Gilbart Y, Kakarieka A, Leppert D, et al. Imaging mass cytometry and single-cell genomics reveal differential depletion and repletion of B-cell populations following ofatumumab treatment in cynomolgus monkeys. Front Immunol. (2019) 10:1340. doi: 10.3389/fimmu.2019.01340
46. Korn T, Mitsdoerffer M, Croxford AL, Awasthi A, Dardalhon VA, Galileos G, et al. Il-6 Controls Th17 immunity in vivo by inhibiting the conversion of conventional T cells into Foxp3+ regulatory T cells. Proc Natl Acad Sci U S A. (2008) 105:18460–5. doi: 10.1073/pnas.0809850105
47. Uzawa A, Kawaguchi N, Himuro K, Kanai T, Kuwabara S. Serum cytokine and chemokine profiles in patients with myasthenia gravis. Clin Exp Immunol. (2014) 176:232–7. doi: 10.1111/cei.12272
48. Bi Z, Zhan J, Zhang Q, Gao H, Yang M, Ge H, et al. Clinical and immune-related factors associated with exacerbation in adults with well-controlled generalized myasthenia gravis. Front Immunol. (2023) 14:1177249. doi: 10.3389/fimmu.2023.1177249
49. Sorensen PS, Lisby S, Grove R, Derosier F, Shackelford S, Havrdova E, et al. Safety and efficacy of ofatumumab in relapsing-remitting multiple sclerosis: a phase 2 study. Neurology. (2014) 82:573–81. doi: 10.1212/WNL.0000000000000125
50. Torres JB, Roodselaar J, Sealey M, Ziehn M, Bigaud M, Kneuer R, et al. Distribution and efficacy of ofatumumab and ocrelizumab in humanized Cd20 mice following subcutaneous or intravenous administration. Front Immunol. (2022) 13:814064. doi: 10.3389/fimmu.2022.814064
51. Newsom-Davis J, Willcox N, Scadding G, Calder L, Vincent A. Anti-acetylcholine receptor antibody synthesis by cultured lymphocytes in myasthenia gravis: thymic and peripheral blood cell interactions. Ann N Y Acad Sci. (1981) 377:393–402. doi: 10.1111/j.1749-6632.1981.tb33747.x
52. Winter O, Dame C, Jundt F, Hiepe F. Pathogenic long-lived plasma cells and their survival niches in autoimmunity, malignancy, and allergy. J Immunol. (2012) 189:5105–11. doi: 10.4049/jimmunol.1202317
53. Blum S, Gillis D, Brown H, Boyle R, Henderson R, Heyworth-Smith D, et al. Use and monitoring of low dose rituximab in myasthenia gravis. J Neurol Neurosurg Psychiatry. (2011) 82:659–63. doi: 10.1136/jnnp.2010.220475
54. Damato V, Spagni G, Monte G, Scandiffio L, Cavalcante P, Zampetti N, et al. Immunological response after SARS-CoV-2 infection and mRNA vaccines in patients with myasthenia gravis treated with rituximab. Neuromuscul Disord. (2023) 33:288–94. doi: 10.1016/j.nmd.2023.02.005
55. Yahyazadeh S, Esmaeil N, Shaygannejad V, Mirmosayyeb O. Comparison of follicular T helper cells, monocytes, and T cells priming between newly diagnosed and rituximab-treated MS patients and healthy controls. Res Pharm Sci. (2022) 17:315–23. doi: 10.4103/1735-5362.343085
56. Bounia CA, Liossis SC. B cell depletion treatment decreases Th17 cells in patients with rheumatoid arthritis. Clin Immunol. (2021) 233:108877. doi: 10.1016/j.clim.2021.108877
57. Baumjohann D, Preite S, Reboldi A, Ronchi F, Ansel KM, Lanzavecchia A, et al. Persistent antigen and germinal center B cells sustain T follicular helper cell responses and phenotype. Immunity. (2013) 38:596–605. doi: 10.1016/j.immuni.2012.11.020
Keywords: myasthenia gravis, ofatumumab, follicular T helper cells, T helper type 17 cells, B cells depletion
Citation: Li S, Zhang Z and Liu Z (2023) Therapeutic effect of ofatumumab in patients with myasthenia gravis: immunoregulation of follicular T helper cells and T helper type 17 cells. Front. Neurol. 14:1278250. doi: 10.3389/fneur.2023.1278250
Received: 16 August 2023; Accepted: 13 November 2023;
Published: 11 December 2023.
Edited by:
Carlo Provenzano, Catholic University of the Sacred Heart, Rome, ItalyReviewed by:
Michelangelo Maestri, University of Pisa, ItalyBin Liu, Shandong Provincial Qianfoshan Hospital, China
Li Xiaoli, Shandong University, China
Copyright © 2023 Li, Zhang and Liu. This is an open-access article distributed under the terms of the Creative Commons Attribution License (CC BY). The use, distribution or reproduction in other forums is permitted, provided the original author(s) and the copyright owner(s) are credited and that the original publication in this journal is cited, in accordance with accepted academic practice. No use, distribution or reproduction is permitted which does not comply with these terms.
*Correspondence: Zunjing Liu, bGl1enVuamluZyYjeDAwMDQwOzE2My5jb20=
†These authors have contributed equally to this work and share first authorship