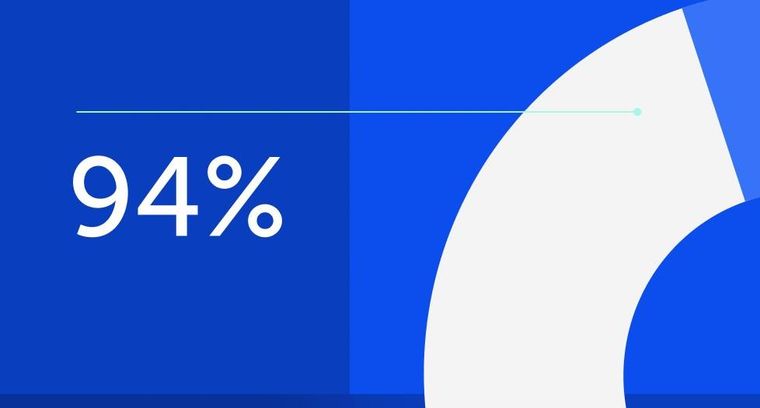
94% of researchers rate our articles as excellent or good
Learn more about the work of our research integrity team to safeguard the quality of each article we publish.
Find out more
MINI REVIEW article
Front. Neurol., 02 November 2023
Sec. Stroke
Volume 14 - 2023 | https://doi.org/10.3389/fneur.2023.1274709
This article is part of the Research TopicAdvances and controversies in ischemic stroke management: from prevention to diagnosis and acute treatmentView all 95 articles
Stroke is one of the most common cerebrovascular diseases, which is the cause of long-term mental illness and physical disability, Post-stroke depression (PSD) is the most common neuropsychiatric complication after stroke, and its mechanisms are characterized by complexity, plurality, and diversity, which seriously affects the quality of survival and prognosis of patients. Studies have focused on and recognized neurotransmitter-based mechanisms and selective serotonin-reuptake inhibitors (SSRIs) can be used to treat PSD. Neuroinflammation, neuroendocrinology, neurotrophic factors, and the site of the stroke lesion may affect neurotransmitters. Thus the mechanisms of PSD have been increasingly studied. Pharmacological treatment mainly includes SSRIs, noradrenergic and specific serotonergic antidepressant (NaSSA), anti-inflammatory drugs, vitamin D, ect, which have been confirmed to have better efficacy by clinical studies. Currently, there is an increasing number of studies related to the mechanisms of PSD. However, the mechanisms and pharmacologic treatment of PSD is still unclear. In the future, in-depth research on the mechanisms and treatment of PSD is needed to provide a reference for the prevention and treatment of clinical PSD.
A significant proportion of older adults will suffer from one or more age-related diseases, including two significant conditions that can cause high morbidity and disability, with high economic burden consequences: stroke (1) and depression (2). Stroke is divided into ischemic stroke and hemorrhagic stroke. The latter includes intracerebral hemorrhage (ICH) and subarachnoid hemorrhage (SAH). Depression is one of the most common symptoms of mental disorders after stroke, with a predicted prevalence of 18 to 33% (3–5). Depression will become the leading cause of global burden by 2030, so attention should be paid to treating depression (6, 7). PSD is mainly manifested as a lack of energy, apathy, sleep disorders, reduced interest, passivity, pessimism, and even suicide, and PSD quickly leads to stroke recurrence. Because many stroke patients have cognitive and language disorders, PSD is not easy to find but seriously hinders the rehabilitation of patients. PSD is a crucial risk factor for long-term adverse physical and mental health outcomes after stroke (8), and PSD can lead to lower quality of life and higher mortality (9). Several stroke-related psycho-social factors worsen, at best, the PSD symptoms by negatively impacting the patient’s daily life (10–12). Much literature has focused on the mechanism of post-ischemic depression, and its mechanisms has become a research hotspot in recent years. Given this, it is necessary to understand the mechanisms and drug treatment of PSD, which can provide a reference for the prevention and treatment of clinical PSD.
Soares proposed a neuroanatomical model of mood disorders in 1997, i.e., the pathway of emotion regulation includes the frontal lobe, the basal ganglia, the amygdala-hippocampus complex, the thalamus, and the connecting fibers between them (13). The frontal-subcortical neural pathway regulates sleep, mood, neuroendocrine, motor and cognitive behaviors, etc. The basal ganglia is an essential pathway for the axons of 5-HT and DA neurons, and the above ways and lesions block the axonal transmission of neurotransmitters to the cortex. Hence, lesions of the above areas are prone to depression. The central monoaminergic nuclei are located in the brainstem, and they fire ascending projections distributed throughout the brain, including the cerebral cortex and limbic system. It is thought that ischemic damage to these nuclei or their projections may result in decreased monoamine levels in a) the left frontal cortex, leading to depressed mood and cognitive deficits; b) the reward system, leading to a lack of pleasure; and c) the basal ganglia, which directly and indirectly regulate mood, cognition, reward, and fatigue (14, 15). The occurrence of stroke in specific regions such as the prefrontal cortex, limbic area, and basal ganglia can disrupt key pathways of mood-related neurotransmitters, leading to depressive disorders (16, 17). Studies have shown that PSD is strongly associated with the site of stroke lesions, but different studies have different results. Terroni et al. (18) further affirmed that the limbic-cortical-striatal-pallidal-thalamic neural pathway is closely associated with PSD based on previous imaging studies. In the 1980s, Robinson et al. suggested that injury to the anterior part of the left hemisphere is more likely than other body parts to lead to depression and PSD (19, 20). Injury to the left cerebral hemisphere is associated with depression, especially damage to the left frontal cortex and the left basal ganglia has a higher incidence of depression, and 5-HT and NE in the left cerebral hemisphere are more likely to be depleted than those in the right cerebral hemisphere, and damage to NE and 5-HTergic neuronal pathways in the above regions, which decreases the content of NE and 5-HT and thus leads to PSD (21–23). However, one study did not support the hypothesis that left hemisphere lesions are associated with an increased risk of PSD, and there was a significant correlation between right hemisphere stroke and the risk of depression after subacute stroke (1–6 months) (24). A significant association between damage to the subcortical circuit in the frontal lobe and PSD has been found (25). In addition, Hong et al. (26) collected 23 patients with PSD diagnosed with frontal subcortical onset. The gray matter volume of the left middle frontal gyrus was significantly reduced in the PSD patients compared with the non-PSD group. The lesion site was located in the left inferior frontal gyrus in about 14 PSD patients, and the lesion site was found in the dorsolateral prefrontal cortex in about 9 PSD patients. Leukoaraiosis also called white matter lesions (WMLs) and white matter hyperintensities (WMHs). Multiple studies have shown that deep leukorariosis with PSD (27). Determining the extent of pre-existing white matter abnormalities can properly guide decision making in acute stroke settings, as a greater degree of such lesioning is usually coupled with neuropsychiatric aftermaths, such as PSD (28). Although there is no uniform conclusion on whether lesion site is associated with PSD, most researchers still believe there is a relationship between lesion site and the occurrence of PSD.
Monoamine neurotransmitters mainly include norepinephrine (NE), 5-hydroxytryptamine (5-HT), and dopamine (DA), and most of their receptors belong to the G-protein-coupled receptor. NE, 5-HT, and DA transmit messages between nerve cells or neurons and effector cells, integrating the overall coordination of body functions. If these neurotransmitters are defective, the normal functioning of the nervous system is compromised, leading to depression (29, 30). Ischemic injury interferes with upward projections from the midbrain and brainstem, reducing the bioavailability of 5-HT, DA, and NE (31). Many neurophysiological studies found an early involvement of the central serotonergic tone since the very acute phase of stroke and in all stroke patients as a group, regardless the degree of disability and the site of the lesion (32–34). In the presence of the SLC6A4 linked promoter region (5-HTTLPR) s/s genotype promoter methylation status was independently associated with PSD both at 2 weeks and more prominently at 1 year after stroke, and was significantly associated with the worsening of depressive symptoms over 1 year (35). Previous studies have demonstrated that 5-hydroxytryptamine transporter length polymorphism (5-HTTLPR) predicts stress and depression (36). Wang et al. (37) successfully prepared a PSD model and found that depressive symptoms in rats could be blocked by the SSRIs citalopram or the 5-HT1A receptor blocker WAY-100635, and detected an increase in newborn neurons in the hippocampal DG region, suggesting that SSRIs act by promoting neural regeneration in the hippocampal DG region. Mak et al. conducted a meta-analysis and found that the 5-HTTLPR LL, LS, and LS genotypes, and the L allele had a positive effect on PSD recovery, but the SS gene in 5-HTTLPR may be a risk factor for PSD (38, 39). Therefore, monoamine neurotransmitters and genes are one of the mechanisms most closely associated with PSD.
After a stroke, acute ischemia/hypoxia occurs in brain tissue, leading to ion transporter dysfunction and ion homeostasis disturbances, which in turn leads to impaired glutamate release and reuptake and intracellular calcium overload, which further contributes to the rapid rise in cerebrospinal fluid glutamate levels. These cascading reactions ultimately lead to neuronal death (40, 41). In addition, excessive glutamate release may lead to synaptic excitotoxicity by exacerbating oxidative stress and inflammation. In contrast, inflammatory mediators may interfere extracellular glutamate levels by decreasing the glutamate scavenging capacity of microglia and astrocytes (42, 43). Many studies have reported higher levels of glutamate and its metabolites in both the blood and cerebrospinal fluid of patients with PSD, especially in the frontal cortex (44, 45). The above experiments suggest that amino acid neurotransmitters are equally involved in developing PSD.
In addition to classical neurotransmitters, astrocytes and microglia in the central nervous system induce cytokine production, including interleukin (IL), tumor necrosis factor (TNF), and interferon (IFN). When the body undergoes an inflammatory response induces the expression of relevant inflammatory cytokines, and an increase in inflammatory cytokines leads to a decrease in the amount of 5-HT or even depletion. Serum inflammatory cytokine levels are elevated in patients with depression, and antidepressant drugs, such as SSRIs, can decrease the pro-inflammatory cytokines IL-6, IL-1β, TNF-α, and IFN-γ, or increase the anti-inflammatory cytokines, such as IL-10, IL-4, IL-13 (46, 47). Inflammation triggers depression by affecting the normal secretion and synthesis of monoamine neurotransmitters, neuronal regeneration, and stimulation of glial cell activation in various ways. Spalletta et al. investigated and put forward the “cytokine hypothesis,” in which pro-inflammatory cytokines interact with 5-HT, leading to the amplification of inflammatory processes and activation of indoleamine-2,3-dioxygenase (IDO) in the limbic region (48). Activation of IDO in the limbic region converts tryptophan to kynurenine, leading to depletion of 5-HT in the paralimbic structures, and the resulting physiological dysfunction may lead to PSD. Elevated levels of inflammatory mediators are thought to be associated with PSD, and increases in pro-inflammatory cytokines IL-1, IL-2, IL-6, IL-17, IL-1β, and TNF-α are strongly associated with PSD (49–51). In another study, a total of 151 patients with acute ischemic stroke were screened at baseline and completed a 1-month follow-up, serum IL-10 levels were measured within 24 h of admission, and depressive symptoms were assessed using the 17-item Hamilton Depression Scale (HAMD-17), with PSD defined as a HAMD score of ≥7. It was found that serum levels of IL-10 were significantly lower in patients with PSD than those in the non-PSD group (52). Alleles associated with reduced anti-inflammatory cytokine function, such as IL-4 + 33C/C and IL-10-1082A/A genotypes, were also associated with PSD (53). In addition, microglia can be distinguished into two phenotypes, the deleterious pro-inflammatory M1 type and the anti-inflammatory M2 type, which represent the dual role of microglia. M1 microglia promote the release of a range of pro-inflammatory cytokines such as TNF-α, IL-1-β, IL-6, and nitric oxide (NO), as well as protein hydrolyzing enzymes, such as matrix metalloproteinase-9 (MMP-9) and MMP-2, which ultimately exacerbate neuronal injury and inhibit neurogenesis in the hippocampus. M2-type microglia express the protective cytokines CD206, IL-10, and scavenger receptors, which have a role in inhibiting inflammation and promoting tissue repair.
NLRP3 inflammatory vesicle is a multiprotein complex of the natural immune system and an upstream regulator of IL-1β. Activation of NLRP3 inflammatory vesicle activates cysteine aspartate lyase-1 via NF-κB and MAPK pathways, induces IL-1β and IL-18 production, and thus promotes inflammatory responses (54, 55). It has been found that lack of NLRP3 attenuates LPS-induced depressive-like symptoms and increases IDO gene expression while inhibiting microglia activation, suggesting that IDO may be a downstream mediator of NLRP3 inflammatory vesicles in inflammation-mediated depressive-like behavior (56). Therefore, Li et al. proposed lowering NLRP3 levels as a treatment for PSD.
Serum growth differentiation factor-15 is a transforming growth factor-β (TGF-β)-related cytokine (57). High levels of serum growth differentiation factor-15 are significantly associated with poor clinical outcomes in acute ischemic stroke, suggesting that serum growth differentiation factor-15 levels can predict the prognosis of ischemic stroke patients (58). High serum growth differentiation factor-15 levels may be associated with an increased risk of suicidal thoughts in depressed patients (59). Many recent studies have pointed out that serum growth differentiation factor-15 levels are nearly one-fold higher in patients with PSD than in patients without depression, and the sensitivity and specificity for predicting PSD were most heightened when the level was 1,660 ng/L (60, 61). MMP-9 is a crucial determinant of extracellular matrix degradation, which is involved in inflammatory response and neuronal plasticity, and it plays a role in the development of brain injury and depression. Elevated serum MMP-9 levels during the acute phase of ischemic stroke were found to be closely associated with the development of depression 3 months later (62). Hypersensitive C-reactive protein (Hs-CRP) is closely related to neurological injury in the acute phase of stroke. It can be used as a serum inflammatory indicator reflecting the intensity of inflammation in the body (63). Hs-CRP can be used as a diagnostic marker for depression, especially for male patients with depression (64). Some studies have found that elevated serum CRP levels on admission are associated with an increased risk of PSD (65). Homocysteine (Hcy) can be used as a biomarker of stroke, and along with abnormally elevated Hcy levels, methylation metabolism is blocked, and NE and 5-HT levels are reduced, thus leading to depression (66, 67). And it has been found that high levels of Hs-CRP and higher Hcy in ischemic stroke patients may also be associated with PSD (68, 69).
In addition to the well-recognized microglia, other inflammatory markers have attracted widespread attention. A meta-analysis examined whether neutrophil-tolymphocyte ratio (NLR) platelet-lympho-cyte ratio (PLR) and monocyte-to-lymphocyte (MLR) were associated with depression and found that NLR levels were significantly higher in depressed patients than in healthy controls (70). Two hundred and ninety-nine consecutive ischemic stroke patients were enrolled and followed up for 1 month; 26.1% of patients were diagnosed with PSD at 1 month, and patients with PSD had significantly higher NLR levels on admission compared with non-PSD patients and normal controls, with an NLR ≥3.701 independently associated with the development of PSD (71). A recent meta-analysis showed that higher inflammation ratios, especially NLR, were significantly associated with the risk of developing depression and that compared to non-PSD patients, PSD patients had a significantly higher NLR and MLR values were higher in PSD patients (72). Higher platelet count is a predictor of inflammation, and platelet activation and increased platelet counts play an important role in depression, as well as being one of the risk factors for the increased prevalence of cerebrovascular disease, and patients with major depression with psychotic features have a higher PLR than other patients (73). Elevated PLR on admission is an important and independent marker for predicting the development of PSD, and whether it changes over time remains to be thoroughly investigated (74). Sarejloo et al. (75) found that the NLR was higher in patients with PSD than in non-depressed patients with stroke, and the PLR was significantly higher in patients with PSD than in non-depressed patients with stroke.
The HPA axis is the neuroendocrine system that regulates mood; first, when the hypothalamus receives signals from the hippocampus or other tissues, the paraventricular nucleus of the hypothalamus releases corticotropin-releasing hormone (CRH), which induces adrenal cortical hormone (ACTH) and glucocorticoid (GCs).
Adrenocorticotropic Hormone (ACTH) stimulates the synthesis and secretion of GCs in the zona fasciculate by binding to its primary target. As downstream effectors of the HPA axis, GCs enter the circulation and readily cross the blood–brain barrier to regulate physiological changes via intracellular receptors throughout the body. Elevated cortisol concentrations in plasma, urine, and cerebrospinal fluid have been reported in depressed patients, accompanied by downregulation of peripheral 5-HT, hyperactivation of the HPA axis, and upregulation of ACTH (76, 77). Compounds with GC receptor antagonist activity and 5-HT1A receptor agonist activity may be better drugs for treating depression (78, 79). HPA dysfunction is present in 40% of stroke patients, triggering depression, poor prognosis, and increased death are associated (80). Excess cortisol may also be associated with monoamine dysfunction, and in a recent clinical study, Reimold et al. investigated the correlation between cortisol response and thalamic 5-HT transporter levels using positron emission tomography and found that decreased levels of thalamic 5-HT transporters were significantly correlated with elevated cortisol response (81).
Brain Derived Neurotrophic Factor (BDNF) has a variety of biological functions; through the activation of tropomyosin receptor kinase B (TrkB) receptor and p75NTR receptors, TrkB and p75NTR pathway activation lead to opposed effects, BDNF requires signaling through TrkB in neuronal growth and maturation. In contrast, the p75NTR pathway triggers apoptosis and inhibits synapse formation. Activation of the TrkB and p75NTR pathways leads to opposed effects, with BDNF required to signaling through TrkB in neuronal growth and maturation. In contrast, the p75NTR pathway triggers apoptosis and inhibits synapse formation, and BDNF is involved in the physiological and pathological processes of depression and ischemic stroke (82, 83). Yang et al. established a PSD model by oxygen–glucose deprivation and corticosterone treatment of neuronal cells. proBDNF protein levels were significantly elevated in the cortex and hippocampus of rats in the PSD group compared to the control group, suggesting that proBDNF plays a role in PSD pathophysiology (84). In addition, a PSD-like cell model was re-established by recombination of the p75 neurotrophin receptor (p75NTR) or silencing of the c-Jun amino-terminal kinase (JNK) to re-establish a PSD-like cell model and found that p75NTR and silencing of JNK (siJNK) inhibited PSD-induced proBDNF up-regulation and increased apoptosis (84). In the same cohort, higher BDNF methylation status and BDNF val66met polymorphism were independently associated with the prevalence of PSD (85).
Glial Cell Line-derived Neurotrophic Factor (GDNF) is widely distributed in the hypothalamus and other brain parts. The role of GDNF in the brain is essential in the survival, differentiation, and regeneration of neurons in the ischemic hemiparetic zone. GDNF can protect 5-HT and DA neurons from oxidative stress and neuroinflammatory damage and has neurotrophic effects on brain tissue (86). Lower levels of GDNF may be involved in the pathophysiological processes of depression, and GDNF levels increase after antidepressant treatment (87). Some scholars have found that GDNF and mRNA are closely related to PSD, and GDNF can be used as a biomarker for the differential diagnosis of major depression and PSD (88). It further suggests that GDNF may act on neurotransmitters and thus participate in the development of PSD.
IGF-1 has received much attention for its influence on recovery after stroke (89). Ketamine, an n-methyl-d-aspartate receptor antagonist, exerts antidepressant effects, and ketamine also induces sustained massive release of IGF-1 in the prefrontal cortex of male mice (90). A clinical trial by Wei Zhang et al. suggested that low serum IGF-1 levels on admission may be involved in developing PSD (91). Recent studies suggest that carriers of the T allele at the rs9282715 locus of the IGF-1R gene may be susceptible to PSD (92).
Antidepressant medicines can effectively improve patients’ nxiety, depression, and somatization symptoms, the first choice for PSD treatment. The principle of medication is to use the smallest effective dose possible to minimize the adverse effects and improve adherence to the treatment. In the early days, antidepressants such as TCAs, tetracyclines, and monoamine oxidase inhibitors were the mainstay, but these antidepressants had more side effects. SSRIs gradually replaced them with fewer adverse effects and better-tolerated drugs such as NaSSA.
SSRIs, a new class of antidepressant drugs used in clinical applications, began in the 1980s. SSRIs are mainly fluoxetine, paroxetine, sertraline, citalopram, and escitalopram, which can selectively inhibit the presynaptic membrane to the reuptake of 5-HT (93). Studies have demonstrated the efficacy, acceptability, and tolerability of antidepressant medication in patients with PSD (94). There have been small-sample controlled clinical studies showing that fluoxetine (95, 96) and citalopram (96) are effective in the treatment of PSD. Fluoxetine treatment promotes microglial apoptosis (97). Early use of SSRIs such as escitalopram may be a treatment for PSD (98, 99). Zhang et al. (100) retrieved Meta-analyzes up to December 2021. They found that antidepressant co-adjuvant therapy may enhance the efficacy of antidepressant medications, with acupuncture combined with fluoxetine being more efficacious in treating PSD at week 4. In contrast, rTMS combined with paroxetine was more productive in treating PSD at week 8 and was more efficacious. It has been suggested that SSRI treatment may be beneficial in stroke patients to prevent the development of PSD, but treating all stroke patients with SSRI has not proved effective so far. Large, prospective and long-term studies are needed to clarify the possible impact of SSRIs on emotions, cognitive functions, bone fractures and coagulation, as well to detect other possible still neglected side effects (101). Thus, assessing the central serotonergic tone in acute stroke patients through auditory evoked potentials may also help to predict the responsiveness to the SSRI treatment and individuate the subgroup of PSD patients who may benefit from SSRI treatment (102–104).
The representative drug of NaSSA is mirtazapine, which increases the release of 5-HT by directly inhibiting the α2 receptors at the endings of 5-HT neurons and also stimulates the α2 receptors on the cytosolic bodies of 5-HT neurons by increasing the NE content to increase the release of 5-HT further. Li et al. the eighth week of administration of the drug, Mirtazapine may be the best choice for treating PSD patients compared to other antidepressants (105). A study found that ischemic stroke patients who received 30 mg of mirtazapine or no antidepressant treatment starting on day 1 after stroke developed PSD in 40% of the untreated group. In contrast, PSD occurred in only 5.7% of the patients in the group treated with mirtazapine, with 16 patients experiencing PSD, of which 15 resolved after initiating mirtazapine treatment (106).
Anti-inflammatory drugs can increase the concentration of monoaminergic neurotransmitters in the synaptic gap of the neurons involved in the brain in a short period. It has been found that the use of acetylsalicylic acid (ASA), nonsteroidal anti-inflammatory drugs (NSAIDs), or statins in stroke patients reduced the risk of early-onset depression, but a higher risk for late depression (107). Minocycline is widely recognized as a novel agent capable of inhibiting microglial activation (108), It also exerts anti-inflammatory properties (109). A systematic review revealed that minocycline increases the viability of neurons and decreases the infarct volume following cerebral ischemia, the mechanisms included anti-inflammatory, antioxidant, as well as anti-apoptotic effects (110). Bassett et al. (111) studies have found that minocycline reverses the pathogenic phagocytic potential of neurotoxic M1 microglia, and reduces the negative phenotypes associated with reduced neurogenesis caused by mice exposure to chronic mild stress (CMS) induced depressive-like behavior. Camargos et al. (112) used the clamping of the common carotid arteries bilaterally in C57BL/6 mice to prepare a cerebral ischemia–reperfusion injury model, and minocycline improved depression-like behavior in cerebral ischemia mice.
Vitamin D is the only neurosteroid hormone that may regulate 5-HT synthesis via tryptophan hydroxylase 2 (113). Vitamin D may affect the synthesis of neurotransmitters, such as serotonin and dopamine, and is also involved in changes in brain morphology (114, 115). Pertile et al. (114) study continues to establish vitamin D as an important differentiation agent for developing dopamine neurons, and now for the first time shows chronic exposure to the active vitamin D hormone increases the capacity of developing neurons to release dopamine. According to previous research, vitamin D deficiency may be a risk factor for depression (116, 117). A prospective study encompassed 58,646 healthy Japanese adults (23,099 men and 35,547 women) aged of 40 to 79 years in whom dietary vitamin D intake was determined via a self-administered food frequency questionnaire. The median follow-up period was 19.3 years (1989–2009), and dietary vitamin D intake appeared to be negatively correlated with mortality from stroke (118). A Meta-analysis conducted by Zhou et al. (119) found that lower vitamin D levels were associated with an increased risk of ischemic stroke. Berghout et al. (120) conducted a prospective study measuring serum 25-hydroxyvitamin D concentrations in 9,680 participants (56.8% female) aged ≥45 years from 1997 to 2008, and lower serum 25-hydroxyvitamin D concentrations were not associated with a higher risk of stroke. It was not associated with a higher risk of stroke, and only severe vitamin D deficiency was associated with incident stroke. Gu et al. found a higher prevalence of vitamin D deficiency and insufficiency in patients with acute stroke. Low serum vitamin D levels were associated with the development of PSD (121, 122). Another study found that 55 (29.1%) patients with acute ischemic stroke were diagnosed with PSD at 1 month, and lower serum vitamin D within 24 h of admission was associated with PSD development and predicted PSD at 1 month (123). The stimulatory effects of vitamin D3 on the BDNF signaling pathway and neuroplasticity may play a role in the recovery of neurological function and the amelioration of PSD (124).
Multiple mechanisms are interrelated and interact in PSD. Monoamine neurotransmitters are the most important pathogenetic mechanism in PSD, and inflammatory cytokines and microglia can cause a decrease in 5-HT in the brain; altered ratios of monocytes, neutrophils, and lymphocytes can be used as a predictive biomarker for PSD, and there is a correlation between higher proinflammatory factors, NLRP3, TGF-β, Hs-CRP, Hcy with PSD, and the PSD interconnection between neurotrophic factor, neuroendocrine abnormalities, and The interconnections between stroke lesion sites and neurotransmitters involve multiple systems in the body thereby inducing PSD. Currently, PSD is mainly treated with SSRIs, NASSA, anti-inflammatory drugs, and medications such as vitamin D. In the future, many studies are needed to find more critical mechanisms for clinical reference, with the aim of early prediction of PSD and intervention to reduce the morbidity and mortality of PSD.
Q-YZ: Formal analysis, Resources, Writing – original draft, Writing – review & editing. F-YK: Formal analysis, Methodology, Supervision, Validation, Writing – original draft, Writing – review & editing.
The author(s) declare that no financial support was received for the research, authorship, and/or publication of this article.
The authors declare that the research was conducted in the absence of any commercial or financial relationships that could be construed as a potential conflict of interest.
All claims expressed in this article are solely those of the authors and do not necessarily represent those of their affiliated organizations, or those of the publisher, the editors and the reviewers. Any product that may be evaluated in this article, or claim that may be made by its manufacturer, is not guaranteed or endorsed by the publisher.
1. Hankey, GJ. Secondary stroke prevention. Lancet Neurol. (2014) 13:178–94. doi: 10.1016/s1474-4422(13)70255-2
2. Ni, X, Su, H, Lv, Y, Li, R, Chen, C, Zhang, D, et al. The major risk factor for depression in the Chinese middle-aged and elderly population: a cross-sectional study. Front Psych. (2022) 13:986389. doi: 10.3389/fpsyt.2022.986389
3. Medeiros, GC, Roy, D, Kontos, N, and Beach, SR. Post-stroke depression: a 2020 updated review. Gen Hosp Psychiatry. (2020) 66:70–80. doi: 10.1016/j.genhosppsych.2020.06.011
4. Guo, J, Wang, J, Sun, W, and Liu, X. The advances of post-stroke depression: 2021 update. J Neurol. (2022) 269:1236–49. doi: 10.1007/s00415-021-10597-4
5. Cai, W, Mueller, C, Li, YJ, Shen, WD, and Stewart, R. Post stroke depression and risk of stroke recurrence and mortality: a systematic review and meta-analysis. Ageing Res Rev. (2019) 50:102–9. doi: 10.1016/j.arr.2019.01.013
6. Herrman, H, Kieling, C, McGorry, P, Horton, R, Sargent, J, and Patel, V. Reducing the global burden of depression: a lancet-world psychiatric association commission. Lancet. (2019) 393:e42–3. doi: 10.1016/s0140-6736(18)32408-5
7. Malhi, GS, and Mann, JJ. Depression. Lancet. (2018) 392:2299–312. doi: 10.1016/s0140-6736(18)31948-2
8. Schöttke, H, Gerke, L, Düsing, R, and Möllmann, A. Post-stroke depression and functional impairments - a 3-year prospective study. Compr Psychiatry. (2020) 99:152171. doi: 10.1016/j.comppsych.2020.152171
9. Stein, LA, Goldmann, E, Zamzam, A, Luciano, JM, Messé, SR, Cucchiara, BL, et al. Association between anxiety, depression, and post-traumatic stress disorder and outcomes after ischemic stroke. Front Neurol. (2018) 9:890. doi: 10.3389/fneur.2018.00890
10. Gainotti, G, Azzoni, A, and Marra, C. Frequency, phenomenology and anatomical-clinical correlates of major post-stroke depression. Br J Psychiatry. (1999) 175:163–7. doi: 10.1192/bjp.175.2.163
11. Tsouna-Hadjis, E, Vemmos, KN, Zakopoulos, N, and Stamatelopoulos, S. First-stroke recovery process: the role of family social support. Arch Phys Med Rehabil. (2000) 81:881–7. doi: 10.1053/apmr.2000.4435
12. Malliarou, M, Tsionara, C, Patsopoulou, A, Bouletis, A, Tzenetidis, V, Papathanasiou, I, et al. Investigation of factors that affect the quality of life after a stroke. Adv Exp Med Biol. (2023) 1425:437–42. doi: 10.1007/978-3-031-31986-0_42
13. Soares, JC, and Mann, JJ. The anatomy of mood disorders--review of structural neuroimaging studies. Biol Psychiatry. (1997) 41:86–106. doi: 10.1016/s0006-3223(96)00006-6
14. Robinson, RG, and Jorge, RE. Post-stroke depression: a review. Am J Psychiatry. (2016) 173:221–31. doi: 10.1176/appi.ajp.2015.15030363
15. Loubinoux, I, Kronenberg, G, Endres, M, Schumann-Bard, P, Freret, T, Filipkowski, RK, et al. Post-stroke depression: mechanisms, translation and therapy. J Cell Mol Med. (2012) 16:1961–9. doi: 10.1111/j.1582-4934.2012.01555.x
16. Rupprechter, S, Romaniuk, L, Series, P, Hirose, Y, Hawkins, E, Sandu, AL, et al. Blunted medial prefrontal cortico-limbic reward-related effective connectivity and depression. Brain. (2020) 143:1946–56. doi: 10.1093/brain/awaa106
17. Li, G, Liu, Y, Zheng, Y, Wu, Y, Li, D, Liang, X, et al. Multiscale neural modeling of resting-state fMRI reveals executive-limbic malfunction as a core mechanism in major depressive disorder. Neuroimage Clin. (2021) 31:102758. doi: 10.1016/j.nicl.2021.102758
18. Terroni, L, Amaro, E, Iosifescu, DV, Tinone, G, Sato, JR, Leite, CC, et al. Stroke lesion in cortical neural circuits and post-stroke incidence of major depressive episode: a 4-month prospective study. World J Biol Psychiatry. (2011) 12:539–48. doi: 10.3109/15622975.2011.562242
19. Brydon, L, Harrison, NA, Walker, C, Steptoe, A, and Critchley, HD. Peripheral inflammation is associated with altered substantia nigra activity and psychomotor slowing in humans. Biol Psychiatry. (2008) 63:1022–9. doi: 10.1016/j.biopsych.2007.12.007
20. Starkstein, SE, Robinson, RG, and Price, TR. Comparison of cortical and subcortical lesions in the production of poststroke mood disorders. Brain. (1987) 110:1045–59. doi: 10.1093/brain/110.4.1045
21. Kim, JS, and Choi-Kwon, S. Poststroke depression and emotional incontinence: correlation with lesion location. Neurology. (2000) 54:1805–10. doi: 10.1212/wnl.54.9.1805
22. Hama, S, Yamashita, H, Shigenobu, M, Watanabe, A, Hiramoto, K, Kurisu, K, et al. Depression or apathy and functional recovery after stroke. Int J Geriatr Psychiatry. (2007) 22:1046–51. doi: 10.1002/gps.1866
23. Klingbeil, J, Brandt, ML, Wawrzyniak, M, Stockert, A, Schneider, HR, Baum, P, et al. Association of Lesion Location and Depressive Symptoms Poststroke. Stroke. (2021) 52:830–7. doi: 10.1161/STROKEAHA.120.031889
24. Wei, N, Yong, W, Li, X, Zhou, Y, Deng, M, Zhu, H, et al. Post-stroke depression and lesion location: a systematic review. J Neurol. (2015) 262:81–90. doi: 10.1007/s00415-014-7534-1
25. Ribeiro, NF, and Madruga, L. A sudden and severe depressive episode after a left cingulate gyrus stroke: a case report of post-stroke depression and review of literature. J Neural Transm (Vienna). (2021) 128:711–6. doi: 10.1007/s00702-021-02334-y
26. Hong, W, Zhao, Z, Wang, D, Li, M, Tang, C, Li, Z, et al. Altered gray matter volumes in post-stroke depressive patients after subcortical stroke. Neuroimage Clin. (2020) 26:102224. doi: 10.1016/j.nicl.2020.102224
27. Carnes-Vendrell, A, Deus, J, Molina-Seguin, J, Pifarré, J, and Purroy, F. Depression and apathy after transient ischemic attack or minor stroke: prevalence, evolution and predictors. Sci Rep. (2019) 9:16248. doi: 10.1038/s41598-019-52721-5
28. Tziaka, E, Christidi, F, Tsiptsios, D, Sousanidou, A, Karatzetzou, S, Tsiakiri, A, et al. Leukoaraiosis as a predictor of depression and cognitive impairment among stroke survivors: a systematic review. Neurol Int. (2023) 15:238–72. doi: 10.3390/neurolint15010016
29. Sullivan, GM, Ogden, RT, Huang, YY, Oquendo, MA, Mann, JJ, and Parsey, RV. Higher in vivo serotonin-1a binding in posttraumatic stress disorder: a PET study with [11C]WAY-100635. Depress Anxiety. (2013) 30:197–206. doi: 10.1002/da.22019
30. Spasojevic, N, Jovanovic, P, and Dronjak, S. Chronic fluoxetine treatment affects gene expression of catecholamine enzymes in the heart of depression model rats. Indian J Exp Biol. (2012) 50:771–5.
31. Robinson, RG, Kubos, KL, Starr, LB, Rao, K, and Price, TR. Mood disorders in stroke patients. Importance of location of lesion. Brain. (1984) 107:81–93. doi: 10.1093/brain/107.1.81
32. Rocco, A, Afra, J, Toscano, M, Sirimarco, G, Di Clemente, L, Altieri, M, et al. Acute subcortical stroke and early serotonergic modification: a IDAP study. Eur J Neurol. (2007) 14:1378–82. doi: 10.1111/j.1468-1331.2007.01985.x
33. Newberg, AR, Davydow, DS, and Lee, HB. Cerebrovascular disease basis of depression: post-stroke depression and vascular depression. Int Rev Psychiatry. (2006) 18:433–41. doi: 10.1080/09540260600935447
34. Toscano, M, Viganò, A, Puledda, F, Verzina, A, Rocco, A, Lenzi, GL, et al. Serotonergic correlation with anger and aggressive behavior in acute stroke patients: an intensity dependence of auditory evoked potentials (IDAP) study. Eur Neurol. (2014) 72:186–92. doi: 10.1159/000362268
35. Kim, JM, Stewart, R, Kang, HJ, Kim, SW, Shin, IS, Kim, HR, et al. A longitudinal study of SLC6A4 DNA promoter methylation and poststroke depression. J Psychiatr Res. (2013) 47:1222–7. doi: 10.1016/j.jpsychires.2013.04.010
36. Starr, LR, Hammen, C, Brennan, PA, and Najman, JM. Serotonin transporter gene as a predictor of stress generation in depression. J Abnorm Psychol. (2012) 121:810–8. doi: 10.1037/a0027952
37. Wang, SH, Zhang, ZJ, Guo, YJ, Sui, YX, and Sun, Y. Involvement of serotonin neurotransmission in hippocampal neurogenesis and behavioral responses in a rat model of post-stroke depression. Pharmacol Biochem Behav. (2010) 95:129–37. doi: 10.1016/j.pbb.2009.12.017
38. Wang, Y, Liu, H, Jiang, Y, Shi, X, Shao, Y, and Xu, ZX. Meta-analysis of 5-hydroxytryptamine transporter gene promoter region polymorphism and post-stroke depression. J Int Med Res. (2020) 48:300060520925943. doi: 10.1177/0300060520925943
39. Mak, KK, Kong, WY, Mak, A, Sharma, VK, and Ho, RC. Polymorphisms of the serotonin transporter gene and post-stroke depression: a meta-analysis. J Neurol Neurosurg Psychiatry. (2013) 84:322–8. doi: 10.1136/jnnp-2012-303791
40. Suzuki, H, Kawakita, F, Asada, R, Nakano, F, Nishikawa, H, and Fujimoto, M. Old but still hot target, glutamate-mediated neurotoxicity in stroke. Transl Stroke Res. (2022) 13:216–7. doi: 10.1007/s12975-021-00958-6
41. Gruenbaum, BF, Kutz, R, Zlotnik, A, and Boyko, M. Blood glutamate scavenging as a novel glutamate-based therapeutic approach for post-stroke depression. Ther Adv Psychopharmacol. (2020) 10:2045125320903951. doi: 10.1177/2045125320903951
42. Tomasetti, C, Iasevoli, F, Buonaguro, EF, De Berardis, D, Fornaro, M, Fiengo, AL, et al. Treating the synapse in major psychiatric disorders: the role of postsynaptic density network in dopamine-glutamate interplay and psychopharmacologic drugs molecular actions. Int J Mol Sci. (2017) 18:135. doi: 10.3390/ijms18010135
43. Haroon, E, Miller, AH, and Sanacora, G. Inflammation, glutamate, and glia: a trio of trouble in mood disorders. Neuropsychopharmacology. (2017) 42:193–215. doi: 10.1038/npp.2016.199
44. Madeira, C, Vargas-Lopes, C, Brandão, CO, Reis, T, Laks, J, Panizzutti, R, et al. Elevated glutamate and glutamine levels in the cerebrospinal fluid of patients with probable Alzheimer's disease and depression. Front Psych. (2018) 9:561. doi: 10.3389/fpsyt.2018.00561
45. Sanacora, G, Treccani, G, and Popoli, M. Towards a glutamate hypothesis of depression: an emerging frontier of neuropsychopharmacology for mood disorders. Neuropharmacology. (2012) 62:63–77. doi: 10.1016/j.neuropharm.2011.07.036
46. Ogłodek, E. Changes in the serum levels of cytokines: IL-1β, IL-4, IL-8 and IL-10 in depression with and without posttraumatic stress disorder. Brain Sci. (2022) 12:387. doi: 10.3390/brainsci12030387
47. Wang, L, Wang, R, Liu, L, Qiao, D, Baldwin, DS, and Hou, R. Effects of SSRIs on peripheral inflammatory markers in patients with major depressive disorder: a systematic review and meta-analysis. Brain Behav Immun. (2019) 79:24–38. doi: 10.1016/j.bbi.2019.02.021
48. Spalletta, G, Bossù, P, Ciaramella, A, Bria, P, Caltagirone, C, and Robinson, RG. The etiology of poststroke depression: a review of the literature and a new hypothesis involving inflammatory cytokines. Mol Psychiatry. (2006) 11:984–91. doi: 10.1038/sj.mp.4001879
49. Korostynski, M, Hoinkis, D, Piechota, M, Golda, S, Pera, J, Slowik, A, et al. Toll-like receptor 4-mediated cytokine synthesis and post-stroke depressive symptoms. Transl Psychiatry. (2021) 11:246. doi: 10.1038/s41398-021-01359-x
50. Wu, D, Zhang, G, Zhao, C, Yang, Y, Miao, Z, and Xu, X. Interleukin-18 from neurons and microglia mediates depressive behaviors in mice with post-stroke depression. Brain Behav Immun. (2020) 88:411–20. doi: 10.1016/j.bbi.2020.04.004
51. Li, R, Fan, W, Li, D, and Liu, X. Correlation of common inflammatory cytokines with cognition impairment, anxiety, and depression in acute ischemic stroke patients. Braz J Med Biol Res. (2022) 55:e11517. doi: 10.1590/1414-431X2021e11517
52. Chi, CH, Huang, YY, Ye, SZ, Shao, MM, Jiang, MX, Yang, MY, et al. Interleukin-10 level is associated with post-stroke depression in acute ischaemic stroke patients. J Affect Disord. (2021) 293:254–60. doi: 10.1016/j.jad.2021.06.037
53. Jm, K, Hj, K, Jw, K, Ky, B, Sw, K, Jt, K, et al. Associations of tumor necrosis factor-α and interleukin-1β levels and polymorphisms with post-stroke depression. Am J Geriatr Psychiatry. (2017) 25:1300–8. doi: 10.1016/j.jagp.2017.07.012
54. Place, DE, and Kanneganti, TD. Recent advances in inflammasome biology. Curr Opin Immunol. (2018):32–8. doi: 10.1016/j.coi.2017.10.011
55. Shen, HH, Yang, YX, Meng, X, Luo, XY, Li, XM, Shuai, ZW, et al. NLRP3: a promising therapeutic target for autoimmune diseases. Autoimmun Rev. (2018) 17:694–702. doi: 10.1016/j.autrev.2018.01.020
56. Zhang, S, Zong, Y, Ren, Z, Hu, J, Wu, X, Xiao, H, et al. Regulation of indoleamine 2, 3-dioxygenase in hippocampal microglia by NLRP3 inflammasome in lipopolysaccharide-induced depressive-like behaviors. Eur J Neurosci. (2020) 52:4586–601. doi: 10.1111/ejn.15016
57. Li, Z, Xu, H, Xu, Y, Lu, G, Peng, Q, Chen, J, et al. Morinda officinalis oligosaccharides alleviate depressive-like behaviors in post-stroke rats via suppressing NLRP3 inflammasome to inhibit hippocampal inflammation. CNS Neurosci Ther. (2021) 27:1570–86. doi: 10.1111/cns.13732
58. Yin, J, Zhu, Z, Guo, D, Wang, A, Zeng, N, Zheng, X, et al. Increased growth differentiation factor 15 is associated with unfavorable clinical outcomes of acute ischemic stroke. Clin Chem. (2019) 65:569–78. doi: 10.1373/clinchem.2018.297879
59. Peng, R, Li, D, Mei, SQ, and Li, Y. The association among serum growth differentiation factor 15 level and suicidal ideation is dependent on testosterone deficiency in depressive patients. J Inflamm Res. (2021) 14:2723–30. doi: 10.2147/JIR.S313200
60. Lu, X, Duan, J, Cheng, Q, and Lu, J. The association between serum growth differentiation factor-15 and 3-month depression after acute ischemic stroke. J Affect Disord. (2020) 260:695–702. doi: 10.1016/j.jad.2019.09.037
61. Zang, Y, Zhu, Z, Xie, Y, Liu, Z, Yin, J, Yang, P, et al. Serum growth differentiation factor 15 levels are associated with depression after ischemic stroke. J Am Heart Assoc. (2022) 11:e022607. doi: 10.1161/JAHA.121.022607
62. Che, B, Zhu, Z, Bu, X, Yin, J, Han, L, Xu, T, et al. Multiple biomarkers covering several pathways for the prediction of depression after ischemic stroke. J Affect Disord. (2021) 280:442–9. doi: 10.1016/j.jad.2020.10.075
63. Cai, Z, He, W, Zhuang, FJ, and Chen, Y. The role of high high-sensitivity C-reactive protein levels at admission on poor prognosis after acute ischemic stroke. Int J Neurosci. (2019) 129:423–9. doi: 10.1080/00207454.2018.1538139
64. Tayefi, M, Shafiee, M, Kazemi-Bajestani, SMR, Esmaeili, H, Darroudi, S, Khakpouri, S, et al. Depression and anxiety both associate with serum level of hs-CRP: a gender-stratified analysis in a population-based study. Psychoneuroendocrinology. (2017) 81:63–9. doi: 10.1016/j.psyneuen.2017.02.035
65. Yang, Y, Zhu, L, Zhang, B, Gao, J, Zhao, T, and Fang, S. Higher levels of C-reactive protein in the acute phase of stroke indicate an increased risk for post-stroke depression: a systematic review and meta-analysis. Neurosci Biobehav Rev. (2022) 134:104309. doi: 10.1016/j.neubiorev.2021.08.018
66. Wang, M, Liang, X, Cheng, M, Yang, L, Liu, H, Wang, X, et al. Homocysteine enhances neural stem cell autophagy in in vivo and in vitro model of ischemic stroke. Cell Death Dis. (2019) 10:561. doi: 10.1038/s41419-019-1798-4
67. Moradi, F, Lotfi, K, Armin, M, Clark, CCT, Askari, G, and Rouhani, MH. The association between serum homocysteine and depression: a systematic review and meta-analysis of observational studies. Eur J Clin Investig. (2021) 51:e13486. doi: 10.1111/eci.13486
68. Cheng, LS, Tu, WJ, Shen, Y, Zhang, LJ, and Ji, K. Combination of high-sensitivity C-reactive protein and homocysteine predicts the post-stroke depression in patients with ischemic stroke. Mol Neurobiol. (2018) 55:2952–8. doi: 10.1007/s12035-017-0549-8
69. Tang, CZ, Zhang, YL, Wang, WS, Li, WG, and Shi, JP. Serum levels of high-sensitivity C-reactive protein at admission are more strongly associated with Poststroke depression in acute ischemic stroke than homocysteine levels. Mol Neurobiol. (2016) 53:2152–60. doi: 10.1007/s12035-015-9186-2
70. Su, M, Ouyang, X, and Song, Y. Neutrophil to lymphocyte ratio, platelet to lymphocyte ratio, and monocyte to lymphocyte ratio in depression: a meta-analysis. J Affect Disord. (2022) 308:375–83. doi: 10.1016/j.jad.2022.04.038
71. Chen, H, Luan, X, Zhao, K, Qiu, H, Liu, Y, Tu, X, et al. The association between neutrophil-to-lymphocyte ratio and post-stroke depression. Clin Chim Acta. (2018) 486:298–302. doi: 10.1016/j.cca.2018.08.026
72. Cheng, Y, Wang, Y, Wang, X, Jiang, Z, Zhu, L, and Fang, S. Neutrophil-to-lymphocyte ratio, platelet-to-lymphocyte ratio, and monocyte-to-lymphocyte ratio in depression: an updated systematic review and Meta-analysis. Front Psych. (2022) 13:893097. doi: 10.3389/fpsyt.2022.893097
73. Kayhan, F, Gündüz, Ş, Ersoy, SA, Kandeğer, A, and Annagür, BB. Relationships of neutrophil-lymphocyte and platelet-lymphocyte ratios with the severity of major depression. Psychiatry Res. (2017) 247:332–5. doi: 10.1016/j.psychres.2016.11.016
74. Huang, G, Chen, H, Wang, Q, Hong, X, Hu, P, Xiao, M, et al. High platelet-to-lymphocyte ratio are associated with post-stroke depression. J Affect Disord. (2019) 246:105–11. doi: 10.1016/j.jad.2018.12.012
75. Sarejloo, S, Abadifard, E, Othman, ZJ, Zafarani, F, Khanzadeh, M, Sadigh-Eteghad, S, et al. Neutrophil to lymphocyte ratio and platelet to lymphocyte ratio in Poststroke depression: a systematic review and Meta-analysis. Dis Markers. (2022) 2022:5911408–10. doi: 10.1155/2022/5911408
76. Holsboer, F. The corticosteroid receptor hypothesis of depression. Neuropsychopharmacology. (2000) 23:477–501. doi: 10.1016/S0893-133X(00)00159-7
77. De Kloet, ER, Vreugdenhil, E, Oitzl, MS, and Joëls, M. Brain corticosteroid receptor balance in health and disease. Endocr Rev. (1998) 19:269–301. doi: 10.1210/edrv.19.3.0331
78. Lee, SR, Choi, B, Paul, S, Seo, JH, Back, DB, Han, JS, et al. Depressive-like behaviors in a rat model of chronic cerebral hypoperfusion. Transl Stroke Res. (2015) 6:207–14. doi: 10.1007/s12975-014-0385-3
79. Setiawan, E, Attwells, S, Wilson, AA, Mizrahi, R, Rusjan, PM, Miler, L, et al. Association of translocator protein total distribution volume with duration of untreated major depressive disorder: a cross-sectional study. Lancet Psychiatry. (2018) 5:339–47. doi: 10.1016/S2215-0366(18)30048-8
80. Xu, T, Pu, S, Ni, Y, Gao, M, Li, X, and Zeng, X. Elevated plasma macrophage migration inhibitor factor as a risk factor for the development of post-stroke depression in ischemic stroke. J Neuroimmunol. (2018) 320:58–63. doi: 10.1016/j.jneuroim.2018.04.003
81. Pace, TW, Hu, F, and Miller, AH. Activation of cAMP-protein kinase a abrogates STAT5-mediated inhibition of glucocorticoid receptor signaling by interferon-alpha. Brain Behav Immun. (2011) 25:1716–24. doi: 10.1016/j.bbi.2011.07.227
82. Li, Y, Li, F, Qin, D, Chen, H, Wang, J, Wang, J, et al. The role of brain derived neurotrophic factor in central nervous system. Front Aging Neurosci. (2022) 14:986443. doi: 10.3389/fnagi.2022.986443
83. Podyma, B, Parekh, K, Güler, AD, and Deppmann, CD. Metabolic homeostasis via BDNF and its receptors. Trends Endocrinol Metab. (2021) 32:488–99. doi: 10.1016/j.tem.2021.04.005
84. Yang, B, Wang, L, Nie, Y, Wei, W, and Xiong, W. proBDNF expression induces apoptosis and inhibits synaptic regeneration by regulating the RhoA-JNK pathway in an in vitro post-stroke depression model. Transl Psychiatry. (2021) 11:578. doi: 10.1038/s41398-021-01667-2
85. Kim, JM, Stewart, R, Kang, HJ, Kim, SY, Kim, SW, Shin, IS, et al. A longitudinal study of BDNF promoter methylation and genotype with poststroke depression. J Affect Disord. (2013) 149:93–9. doi: 10.1016/j.jad.2013.01.008
86. Beker, M, Caglayan, AB, Beker, MC, Altunay, S, Karacay, R, Dalay, A, et al. Lentivirally administered glial cell line-derived neurotrophic factor promotes post-ischemic neurological recovery, brain remodeling and contralesional pyramidal tract plasticity by regulating axonal growth inhibitors and guidance proteins. Exp Neurol. (2020) 331:113364. doi: 10.1016/j.expneurol.2020.113364
87. Sun, J, Kong, L, Wu, F, Wei, Y, Zhu, Y, Yin, Z, et al. Decreased plasma glial cell line-derived neurotrophic factor level in major depressive disorder is associated with age and clinical severity. J Affect Disord. (2019) 245:602–7. doi: 10.1016/j.jad.2018.11.068
88. Zhang, Y, Jiang, H, Yue, Y, Yin, Y, Zhang, Y, Liang, J, et al. The protein and mRNA expression levels of glial cell line-derived neurotrophic factor in post stroke depression and major depressive disorder. Sci Rep. (2017) 7:8674. doi: 10.1038/s41598-017-09000-y
89. Hayes, CA, Valcarcel-Ares, MN, and Ashpole, NM. Preclinical and clinical evidence of IGF-1 as a prognostic marker and acute intervention with ischemic stroke. J Cereb Blood Flow Metab. (2021) 41:2475–91. doi: 10.1177/0271678X211000894
90. Deyama, S, Kondo, M, Shimada, S, and Kaneda, K. IGF-1 release in the medial prefrontal cortex mediates the rapid and sustained antidepressant-like actions of ketamine. Transl Psychiatry. (2022) 12:178. doi: 10.1038/s41398-022-01943-9
91. Zhang, W, Wang, W, and Kuang, L. The relation between insulin-like growth factor 1 levels and risk of depression in ischemic stroke. Int J Geriatr Psychiatry. (2018) 33:e228–33. doi: 10.1002/gps.4774
92. Yue, Y, You, L, Zhao, F, Zhang, K, Shi, Y, Tang, H, et al. Common susceptibility variants of KDR and IGF-1R are associated with poststroke depression in the Chinese population. Gen Psychiatr. (2023) 36:e100928. doi: 10.1136/gpsych-2022-100928
93. García-García, ML, Tovilla-Zárate, CA, Villar-Soto, M, Juárez-Rojop, IE, González-Castro, TB, Genis-Mendoza, AD, et al. Fluoxetine modulates the pro-inflammatory process of IL-6, IL-1β and TNF-α levels in individuals with depression: a systematic review and meta-analysis. Psychiatry Res. (2022) 307:114317. doi: 10.1016/j.psychres.2021.114317
94. Chollet, F, Rigal, J, Marque, P, Barbieux-Guillot, M, Raposo, N, Fabry, V, et al. Serotonin selective reuptake inhibitors (SSRIs) and stroke. Curr Neurol Neurosci Rep. (2018) 18:100. doi: 10.1007/s11910-018-0904-9
95. Mortensen, JK, and Andersen, G. Pharmacological management of post-stroke depression: an update of the evidence and clinical guidance. Expert Opin Pharmacother. (2021) 22:1157–66. doi: 10.1080/14656566.2021.1880566
96. Tay, J, Mårtensson, B, Markus, HS, and Lundström, E. Does fluoxetine reduce apathetic and depressive symptoms after stroke? An analysis of the efficacy oF fluoxetine-a randomized controlled trial in stroke trial data set. Int J Stroke. (2023) 18:285–95. doi: 10.1177/17474930221124760
97. Dhami, KS, Churchward, MA, Baker, GB, and Todd, KG. Fluoxetine and its metabolite norfluoxetine induce microglial apoptosis. J Neurochem. (2019) 148:761–78. doi: 10.1111/jnc.14661
98. Deng, L, Sun, X, Qiu, S, Xiong, Y, Li, Y, Wang, L, et al. Interventions for management of post-stroke depression: a Bayesian network meta-analysis of 23 randomized controlled trials. Sci Rep. (2017) 7:16466. doi: 10.1038/s41598-017-16663-0
99. Feng, RF, Ma, R, Wang, P, Ji, X, Zhang, ZX, Li, MM, et al. Efficacy of escitalopram for poststroke depression: a systematic review and meta-analysis. Sci Rep. (2022) 12:3304. doi: 10.1038/s41598-022-05560-w
100. Zhang, J, Song, Z, Gui, C, Jiang, G, Cheng, W, You, W, et al. Treatments to post-stroke depression, which is more effective to HAMD improvement? A network meta-analysis Front Pharmacol. (2022) 13:1035895. doi: 10.3389/fphar.2022.1035895
101. Marazziti, D, Mucci, F, Tripodi, B, Carbone, MG, Muscarella, A, Falaschi, V, et al. Emotional blunting, cognitive impairment, bone fractures, and bleeding as possible side effects of long-term use of SSRIs. Clin Neuropsychiatry. (2019) 16:75–85.
102. Juckel, G, Pogarell, O, Augustin, H, Mulert, C, Müller-Siecheneder, F, Frodl, T, et al. Differential prediction of first clinical response to serotonergic and noradrenergic antidepressants using the loudness dependence of auditory evoked potentials in patients with major depressive disorder. J Clin Psychiatry. (2007) 68:1206–12. doi: 10.4088/jcp.v68n0806
103. Lee, BH, Park, YM, Lee, SH, and Shim, M. Prediction of long-term treatment response to selective serotonin reuptake inhibitors (SSRIs) using scalp and source loudness dependence of auditory evoked potentials (LDAEP) analysis in patients with major depressive disorder. Int J Mol Sci. (2015) 16:6251–65. doi: 10.3390/ijms16036251
104. Ip, CT, Ganz, M, Ozenne, B, Olbrich, S, Beliveau, V, Dam, VH, et al. Association between the loudness dependence of auditory evoked potential, serotonergic neurotransmission and treatment outcome in patients with depression. Eur Neuropsychopharmacol. (2023) 70:32–44. doi: 10.1016/j.euroneuro.2023.02.008
105. Li, X, and Zhang, C. Comparative efficacy of nine antidepressants in treating Chinese patients with post-stroke depression: a network meta-analysis. J Affect Disord. (2020) 266:540–8. doi: 10.1016/j.jad.2020.02.005
106. Niedermaier, N, Bohrer, E, Schulte, K, Schlattmann, P, and Heuser, I. Prevention and treatment of poststroke depression with mirtazapine in patients with acute stroke. J Clin Psychiatry. (2004) 65:1619–23. doi: 10.4088/jcp.v65n1206
107. Wium-Andersen, IK, Wium-Andersen, MK, Jørgensen, MB, and Osler, M. Anti-inflammatory treatment and risk for depression after first-time stroke in a cohort of 147 487 Danish patients. J Psychiatry Neurosci. (2017) 42:320–30. doi: 10.1503/jpn160244
108. Han, QQ, Shen, SY, Chen, XR, Pilot, A, Liang, LF, Zhang, JR, et al. Minocycline alleviates abnormal microglial phagocytosis of synapses in a mouse model of depression. Neuropharmacology. (2022) 220:109249. doi: 10.1016/j.neuropharm.2022.109249
109. Romero-Miguel, D, Lamanna-Rama, N, Casquero-Veiga, M, Gómez-Rangel, V, Desco, M, and Soto-Montenegro, ML. Minocycline in neurodegenerative and psychiatric diseases: an update. Eur J Neurol. (2021) 28:1056–81. doi: 10.1111/ene.14642
110. Naderi, Y, Panahi, Y, Barreto, GE, and Sahebkar, A. Neuroprotective effects of minocycline on focal cerebral ischemia injury: a systematic review. Neural Regen Res. (2020) 15:773–82. doi: 10.4103/1673-5374.268898
111. Bassett, B, Subramaniyam, S, Fan, Y, Varney, S, Pan, H, Carneiro, AMD, et al. Minocycline alleviates depression-like symptoms by rescuing decrease in neurogenesis in dorsal hippocampus via blocking microglia activation/phagocytosis. Brain Behav Immun. (2021) 91:519–30. doi: 10.1016/j.bbi.2020.11.009
112. Camargos, QM, Silva, BC, Silva, DG, Toscano, ECB, Oliveira, BDS, Bellozi, PMQ, et al. Minocycline treatment prevents depression and anxiety-like behaviors and promotes neuroprotection after experimental ischemic stroke. Brain Res Bull. (2020) 155:1–10. doi: 10.1016/j.brainresbull.2019.11.009
113. Patrick, RP, and Ames, BN. Vitamin D hormone regulates serotonin synthesis. Part 1: relevance for autism. FASEB J. (2014) 28:2398–413. doi: 10.1096/fj.13-246546
114. Pertile, RAN, Brigden, R, Raman, V, Cui, X, Du, Z, and Eyles, D. Vitamin D: a potent regulator of dopaminergic neuron differentiation and function. J Neurochem. (2023) 166:779–89. doi: 10.1111/jnc.15829
115. Somoza-Moncada, MM, Turrubiates-Hernández, FJ, Muñoz-Valle, JF, Gutiérrez-Brito, JA, Díaz-Pérez, SA, Aguayo-Arelis, A, et al. Vitamin D in depression: A potential bioactive agent to reduce suicide and suicide attempt risk. Nutrients. (2023) 15:1765. doi: 10.3390/nu15071765
116. Ceolin, G, Matsuo, LH, Confortin, SC, D'Orsi, E, Rieger, DK, and Moreira, JD. Lower serum 25-hydroxycholecalciferol is associated with depressive symptoms in older adults in southern Brazil. Nutr J. (2020) 19:123. doi: 10.1186/s12937-020-00638-5
117. Grudet, C, Lindqvist, D, Malm, J, Westrin, Å, and Ventorp, F. 25(OH)D levels are decreased in patients with difficult-to-treat depression. Compr Psychoneuroendocrinol. (2022) 10:100126. doi: 10.1016/j.cpnec.2022.100126
118. Sheerah, HA, Eshak, ES, Cui, R, Imano, H, Iso, H, and Tamakoshi, A. Relationship between dietary vitamin D and deaths from stroke and coronary heart disease: the Japan collaborative cohort study. Stroke. (2018) 49:454–7. doi: 10.1161/strokeaha.117.019417
119. Zhou, R, Wang, M, Huang, H, Li, W, Hu, Y, and Wu, T. Lower vitamin D status is associated with an increased risk of ischemic stroke: a systematic review and Meta-analysis. Nutrients. (2018) 10:277. doi: 10.3390/nu10030277
120. Berghout, BP, Fani, L, Heshmatollah, A, Koudstaal, PJ, Ikram, MA, Zillikens, MC, et al. Vitamin D status and risk of stroke: the Rotterdam study. Stroke. (2019) 50:2293–8. doi: 10.1161/strokeaha.119.025449
121. Gu, Y, Zhu, Z, Luan, X, and He, J. Vitamin D status and its association with season, depression in stroke. Neurosci Lett. (2019) 690:99–105. doi: 10.1016/j.neulet.2018.09.046
122. Hung, KC, Wu, JY, Illias, AM, Chiu, CC, Chang, YJ, Liao, SW, et al. Association of a low vitamin D status with risk of post-stroke depression: a meta-analysis and systematic review. Front Nutr. (2023) 10:1142035. doi: 10.3389/fnut.2023.1142035
123. Han, B, Lyu, Y, Sun, H, Wei, Y, and He, J. Low serum levels of vitamin D are associated with post-stroke depression. Eur J Neurol. (2015) 22:1269–74. doi: 10.1111/ene.12607
Keywords: stroke, depression, mechanisms, treatment, 5-HT, PSD, SSRIs, vitamin D
Citation: Zhan Q and Kong F (2023) Mechanisms associated with post-stroke depression and pharmacologic therapy. Front. Neurol. 14:1274709. doi: 10.3389/fneur.2023.1274709
Received: 08 August 2023; Accepted: 13 October 2023;
Published: 02 November 2023.
Edited by:
Raffaele Ornello, University of L'Aquila, ItalyReviewed by:
Massimiliano Toscano, Sapienza University of Rome, ItalyCopyright © 2023 Zhan and Kong. This is an open-access article distributed under the terms of the Creative Commons Attribution License (CC BY). The use, distribution or reproduction in other forums is permitted, provided the original author(s) and the copyright owner(s) are credited and that the original publication in this journal is cited, in accordance with accepted academic practice. No use, distribution or reproduction is permitted which does not comply with these terms.
*Correspondence: Fanyi Kong, a2Z5MTEwNjU0NzcyN0AxNjMuY29t
Disclaimer: All claims expressed in this article are solely those of the authors and do not necessarily represent those of their affiliated organizations, or those of the publisher, the editors and the reviewers. Any product that may be evaluated in this article or claim that may be made by its manufacturer is not guaranteed or endorsed by the publisher.
Research integrity at Frontiers
Learn more about the work of our research integrity team to safeguard the quality of each article we publish.