- 1Department of Neurology, The First Affiliated Hospital of Soochow University, Suzhou, Jiangsu, China
- 2Suzhou Jiasheng Medical Instrument Co., Ltd., Suzhou, Jiangsu, China
- 3Department of Radiology, The First Affiliated Hospital of Soochow University, Suzhou, Jiangsu, China
Background: White matter hyperintensity (WMH) is often described in acute lacunar stroke (ALS) patients. However, the specific relationship between regional WMH volume and persistent cognitive impairment remains unclear.
Methods: We enrolled patients with ALS who were hospitalized at the First Affiliated Hospital of Soochow University between January 2020 and November 2022. All patients were assessed for global cognitive function using the Montreal Cognitive Assessment (MoCA) scale at 14 ± 2 days and 6 months after the onset of ALS. Manifestations of chronic cerebral small vessel disease (CSVD) were assessed via MRI scan. The distributions of regional WMH were segmented, and their relationship with cognitive impairment was evaluated.
Results: A total of 129 patients were enrolled. Baseline frontal WMH volume (OR = 1.18, P = 0.04) was an independent risk factor for long-term cognitive impairment after ALS. Furthermore, the presence of WMH at the genu of the corpus callosum (GCC) at baseline (OR = 3.1, P = 0.033) was strongly associated with persistent cognitive decline. Multivariable logistic regression analysis showed that depression (OR = 6.252, P = 0.029), NIHSS score (OR = 1.24, P = 0.011), and albumin at admission (OR = 0.841, P = 0.032) were also important determinants of long-term cognitive impairment after ALS.
Conclusions: Our study found that WMH, especially frontal WMH volume and the presence of WMH at the GCC at baseline, independently contributed to long-term cognitive decline in ALS patients. This study provides new evidence of the clinical relationship between regional WMH volume and cognitive impairment in ALS patients.
Introduction
Acute lacunar stroke (ALS) accounts for nearly 25% of all ischemic strokes (1). Cognitive impairment is common after stroke. The volume and location of the lesions, related vascular risk factors, and probable underlying mechanisms all vary among the different stroke subtypes, which may influence the occurrence and severity of post-stroke cognitive impairment (PSCI). Stroke attributed to large-vessel atherosclerosis is generally considered to carry a higher risk of cognitive impairment. However, lacunar stroke with small-artery occlusion may pose a higher risk of PSCI than expected, with affected patients having an 11%−23% risk of developing dementia (2, 3), which is close to the risk level in other stroke subtypes (4). Additionally, cognitive impairments in lacunar stroke patients affect global cognition rather than specific domains (5). Lacunar stroke patients with cognitive impairment are also at a higher risk of unfavorable prognosis, recurrent stroke, and death (6).
Lacunar stroke is one of the subtypes of cerebral small vessel disease (CSVD), which is a systemic pathology of the whole brain (7). Manifestations of CSVD, such as lacunar stroke and white matter hyperintensity (WMH), have been recognized as leading risk factors for cognitive impairment (8, 9) and dementia (10, 11) in older people. Furthermore, CSVD is also an important risk factor for early recurrence of stroke after a TIA or stroke (12). Patients with ALS usually have a relatively high CSVD burden (13) compared to other elderly populations. The presence of cognitive impairment in lacunar stroke patients probably indicates advanced microangiopathy, which might be worsened by preexisting forms of CSVD, such as WMH, enlarged perivascular space (EPVS), cerebral microbleed (CMB), or brain atrophy (14).
In previous studies, many researchers have investigated the effects of total WMH burden or total WMH volume on cognition (15–18). Recently, the effect of WMH on cognitive function has been hypothesized to differ according to the regional distribution of WMH. Biesbroek et al. (19) have also suggested that the location of WMH is a determinant of the nature of cognitive impairment. The regional specificity of WMH has rarely been investigated. S. Kim et al. (20) found that periventricular WMH adjacent to the lateral ventricles might play a larger role in all-cause dementia (ACD) than deep WMH. Regarding brain lobes, one study has reported that WMH in the frontal, occipital, and temporal regions is associated with cognitive dysfunction in patients suffering from Alzheimer's disease (21). Rizvi et al. (22) also found that higher WMH volumes in the frontal, parietal, and occipital lobes were associated with poorer memory performance in a sample of healthy older adults. However, few studies have focused on the relationship between the regional lobar distribution of WMH and cognitive impairment in ALS patients. In this study, we aimed to assess the influence of the volume distribution of WMH on long-term cognition following ALS.
Materials and methods
Study design and participants
This prospective study was conducted at the Department of Neurology at the First Affiliated Hospital of Soochow University. We consecutively recruited patients who were admitted to our department between January 2020 and November 2022 with an imaging-proven lacunar infarction. Acute lacunar stroke was defined as an acute ischemic lesion with a diameter of ≤ 20mm on diffusion-weighted imaging (DWI) sequence in the perforator artery supply area, with its TOAST classification considered to be small artery occlusion, which has also reported in previous articles (23, 24). We excluded patients to whom any of the following criteria applied: (1) a cortical and/or cortico-subcortical non-lacunar infarct (an axial diameter of > 20 mm on diffusion-weighted MRI sequences); (2) macroangiopathy or cardioembolism; (3) preexisting disability [modified Rankin Scale score (mRS) >1]; (4) a history of severe depression (17-item Hamilton Depression Rating Scale score≥24) or other mental illness; (5) cognitive disorder attributed to severe systemic or other diseases; (6) WMH due to other specific causes; (7) conditions causing inability to cooperate with the clinical assessment, such as disturbance of consciousness, severe language disorders, or blindness; or (8) inability to undergo complete MRI scanning. The study was approved by the ethics committee of the First Affiliated Hospital of Soochow University (approval number 222315), and all participants gave written informed consent.
Data acquisition
Demographic and clinical baseline data were collected within 48 h of admission; these included age, gender, education level, previous medical history [stroke, hypertension, diabetes mellitus, dyslipidemia, and coronary heart disease (CHD)], baseline National Institutes of Health Stroke Scale (NIHSS) score, baseline mRS score, baseline blood glucose and lipid levels, homocysteinemia, albumin, fibrinogen, and other relevant blood indexes.
Neuropsychological assessment and follow-up visit
Neuropsychological tests were conducted by two experienced investigators (SSD and YZ) certified to administer neuropsychological assessments at 14 ± 2 days after stroke onset. The Montreal Cognitive Assessment (MoCA) has high sensitivity and specificity for mild cognitive impairment. According to the guidelines from the Vascular Impairment of Cognition Classification Consensus Study (VICCCS) of the Chinese Revision Plan, cognitive impairment is defined as follows: for individuals with no education, a MoCA score of ≤13 is indicative; for those with 1–6 years of education, a MoCA score of ≤19 is indicative; and for those with 7 or more years of education, a score of ≤24 is indicative (25). Additionally, the 17-item Hamilton Depression Scale (HAMD-17) was used to assess depression severity. A score of < 7 indicates a normal condition (26). A follow-up visit, including neuropsychological evaluations, mRS score assessment, and medication status, was conducted at 6 months (day 182 ± 7) after stroke onset in the form of an outpatient visit or by video. None of the physicians knew the baseline information about the patients.
Brain MRI
All patients underwent a brain MRI scan (Achieva, PHILIPS Medical Systems). We acquired WMH volume using axial 2D T2-fluid-attenuated inversion recovery (T2-FLAIR) images (8000/120 ms for TR/TE; FOV: 220 × 175 × 119 mm; section thickness: 5 mm). Other sequences employed in the study included DWI, 3d-TOF-MRA, T2-weighted, T1-weighted, and susceptibility-weighted imaging (SWI). A total CSVD burden score was calculated according to previously published methods; this consisted of four imaging markers, namely, silent lacuna, WMH, EPVS, and CMB (27). Briefly, WMHs were rated in the FLAIR sequence according to the Fazekas scale. One point was added to the total burden score for either severe periventricular WMH (Fazekas score 3, irregular extension into the deep white matter) or moderate-to-severe deep WMH (Fazekas score 2 or 3, early confluence or confluence). EPVS in the basal ganglia was rated on a semiquantitative scale (grade 0, no EPVS; grade 1, <10 EPVSs; grade 2, 11–20 EPVSs; grade 3, 21–40 EPVSs; grade 4, more than 40 EPVS), and one point was added for EPVS at level 2–4. We used the SWI sequence to evaluate the presence of deep CMBs in the basal ganglia, thalamus, brain stem, and cerebellum. One point was added for the presence of any lacuna or CMB. Total CSVD burden was graded into three levels of severity: mild (0 or 1 point), moderate (2 points), or severe (3 or 4 points) (28, 29).
WMH segment
The regions assessed for WMHs included periventricular white matter hyperintensity (PWMH) and deep white matter hyperintensity (DWMH). If the largest diameter of the WMH was adjacent to the ventricle, we defined it as PWMH; otherwise, it was considered to be DWMH (30). DWMH included WMH in the frontal, parietal, occipital, and temporal regions, as well as infratentorial regions (the brainstem and cerebellum). The segmentation of WMH volumes is shown in Figure 1. An existing lobar atlas was used to obtain regional WMH volumes for the above anatomic sites. The use of this atlas has been described previously (31, 32). PWMH included WMH in three regions, including frontal and occipital caps and bands (33).
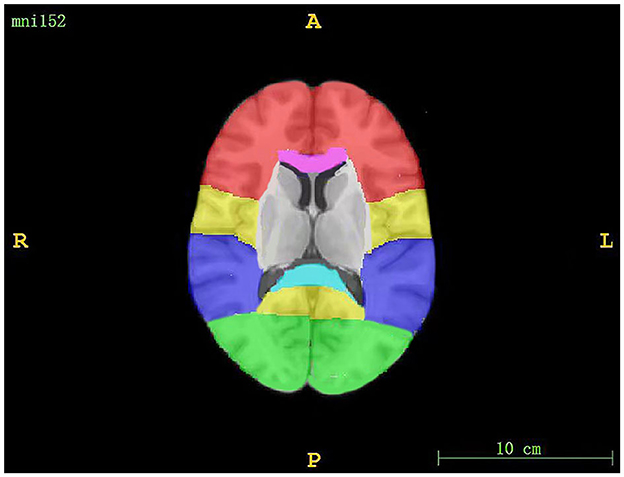
Figure 1. The segmentation of WMH volumes. The red region corresponds to the frontal lobe, the yellow region corresponds to the parietal lobe, the purple region corresponds to the temporal lobe, the green region corresponds to the occipital lobe, the pink region corresponds to the genu of the corpus callosum, and the blue region corresponds to the splenium of the corpus callosum.
The distribution of WMH volume was segmented using MATLAB R2022A (MathWorks, Natick, MA) via the lesion prediction algorithm (LPA; Schmidt, Chapter 6.1), an instrument implemented in the Lesion Segmentation Toolbox version 3.0.0 (www.statistical-modeling.de/lst.html) for SPM12. Large-scale regression models were used to calculate the lesion probability score for each voxel (34).
Statistical analysis
Continuous variables are presented in the form of means ± standard deviation (SD) and were compared using the Student's t-test in the case of normally distributed variables. For non-normally distributed variables, regional WMH volumes are reported in the form of the median (maximum to minimum), other variables are reported in the form of the median (interquartile range), and one-way ANOVA was used for comparisons. Categorical data were examined using the chi-squared test or Fisher's exact test when appropriate and are reported in the form of the constituent ratio. To identify independent predictors of persistent cognitive impairment following lacunar stroke and build a prediction scale, a multivariate logistic regression model was constructed; variables with a p < 0.05 in the univariate analysis were included. SPSS 26.0 was used for statistical analysis.
Results
Baseline characteristics of patients
The study included a total of 129 ALS patients after evaluation (Figure 2), with 24.8% of patients experiencing cognitive impairment during the 6-month follow-up visit. Cognitive trajectories in terms of impairment (cognitive impairment) and preservation from baseline to 6-month follow-up are shown in Figure 3. The participants' characteristics and the differences between the groups with and without cognitive impairment are summarized in Table 1. Compared with the non-cognitive impairment group, the patients in the cognitive impairment group were older (69 ± 10.62 vs. 65 ± 9.33, P = 0.069) and had a higher incidence of depression (21.9% vs. 3.1%, P = 0.002). Patients with higher NIHSS scores (3 vs. 1, P = 0.004) and lower levels of albumin (37.31 ± 3.83 vs. 39.23 ± 3.33, P = 0.012) were more likely to experience cognitive impairment.
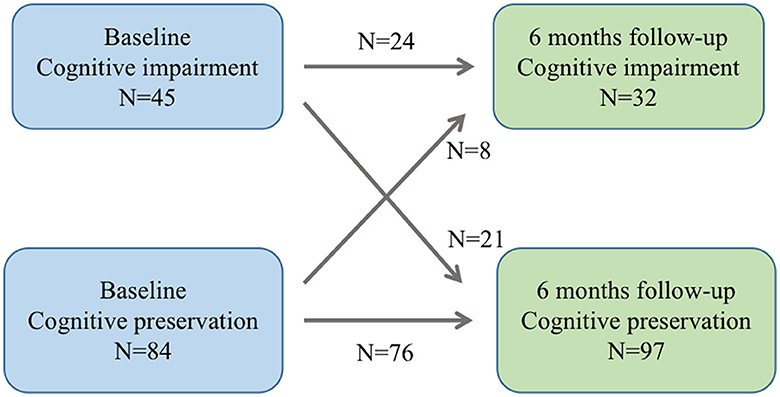
Figure 3. Cognitive trajectories regarding impairment (cognitive impairment and preservation from baseline to 6-month follow-up).
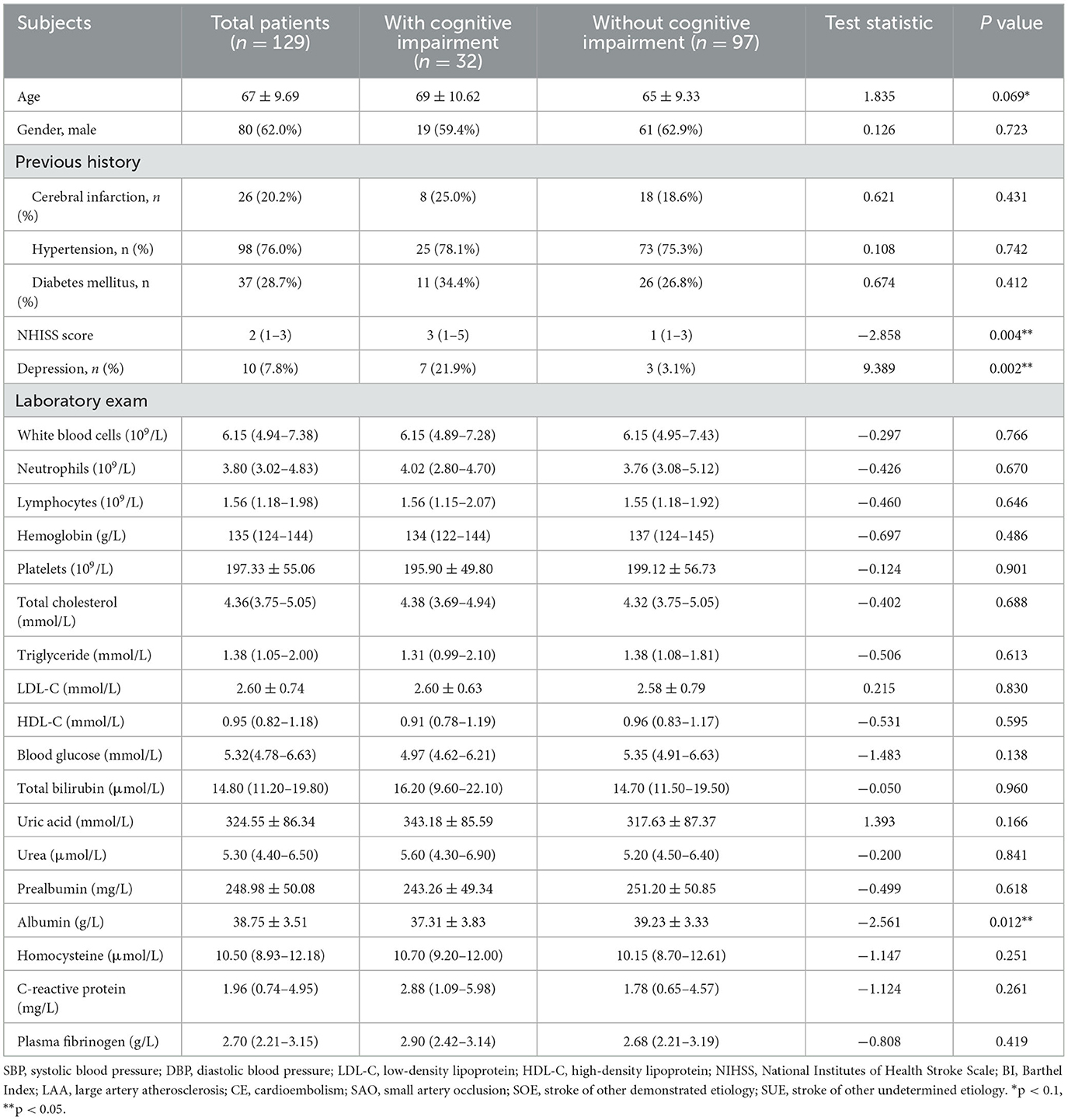
Table 1. Baseline characteristics of acute lacunar stroke patients with and without cognitive impairment.
In a qualitative analysis of CSVD imaging features among the two groups, EPVS (N>10) was found in 21 patients (65.6%) in the cognitive impairment group and 51 patients (52.6%) in the non-cognitive impairment group. Patients with higher total CSVD burden scores suffered cognitive impairment more often at the 6-month follow-up visit. Fazekas scores for periventricular WMH and deep WMH were 3 (2–3) and 2 (1–2) in the cognitive impairment group, respectively. In comparison, these scores were 1 (1–2) and 1 (0-1) in the non-cognitive impairment group. Patients with WMH in the genu of the corpus callosum (GCC) had a higher chance of exhibiting cognitive impairment (53.1% vs. 20.6%, P < 0.001) (Table 2).
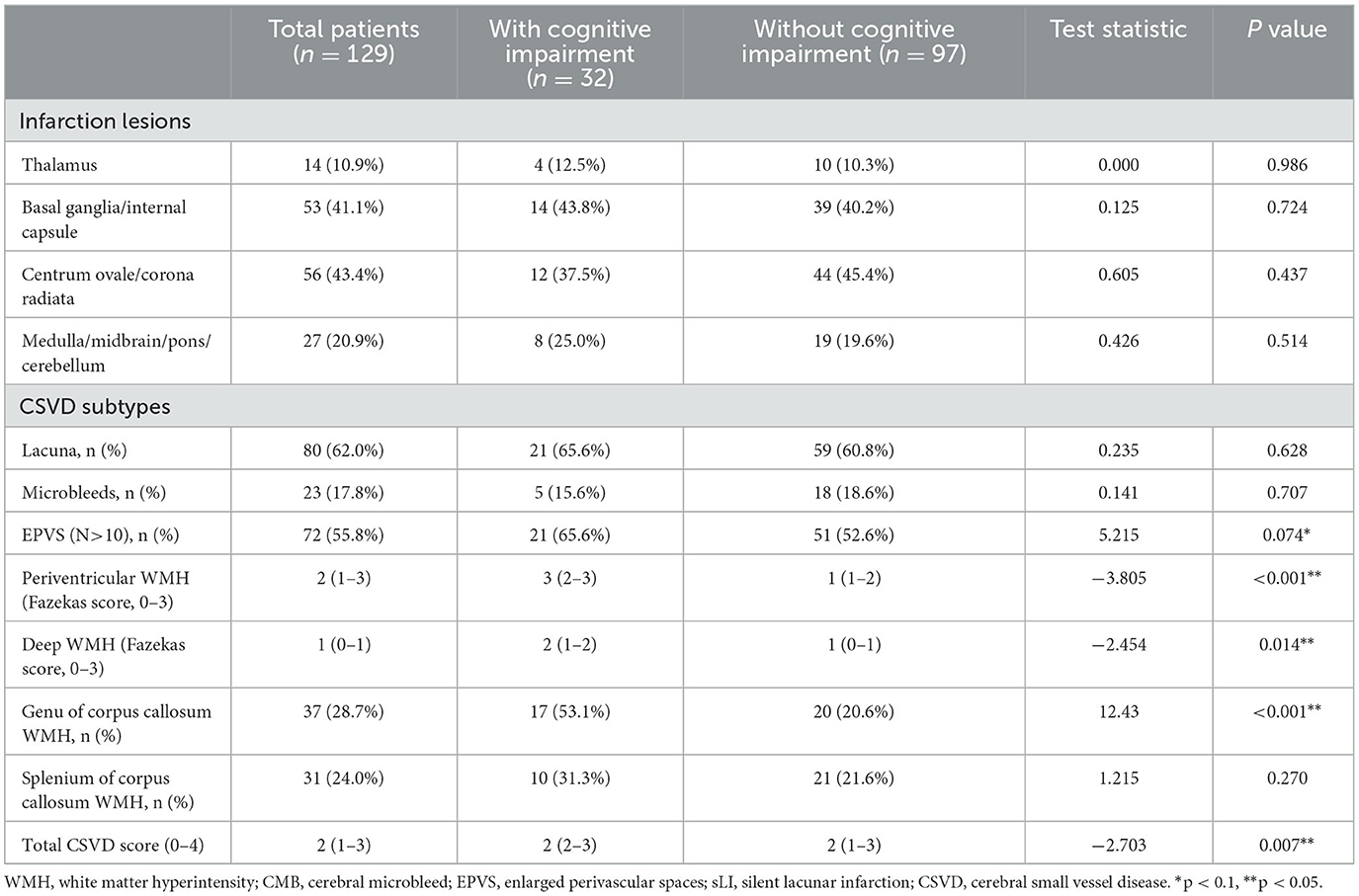
Table 2. Qualitative analysis of imaging features in acute lacunar stroke patients with and without cognitive impairment.
Comparison of regional WMH volumes between the cognitive impairment and non-cognitive impairment groups
Total and regional WMH volumes were quantified for each group (Table 3). The acute lacunar infarction patients with cognitive impairment showed higher total WMH volume relative to the non-cognitive impairment group (median volume of WMH in the cognitive impairment group = 14.45 mm3; median volume of WMH in the non-cognitive impairment group = 5.52 mm3; P = 0.001). Statistically significant differences were also observed in the case of frontal (median volume was 2.96 mm3 in the cognitive impairment group vs. 0.52 mm3 in the non-impairment group; P = 0.006), parietal (median volume was 0.41 mm3 in the cognitive impairment group vs. 0.00 mm3 in the non-cognitive impairment group; P = 0.005), and periventricular WMH volume (median volume was 10.56 mm3 in the cognitive impairment group vs. 3.74 mm3 in the non-cognitive impairment group; P = 0.001). No differences were observed in the case of temporal, occipital, or subtentorial WMH volume (P > 0.05).
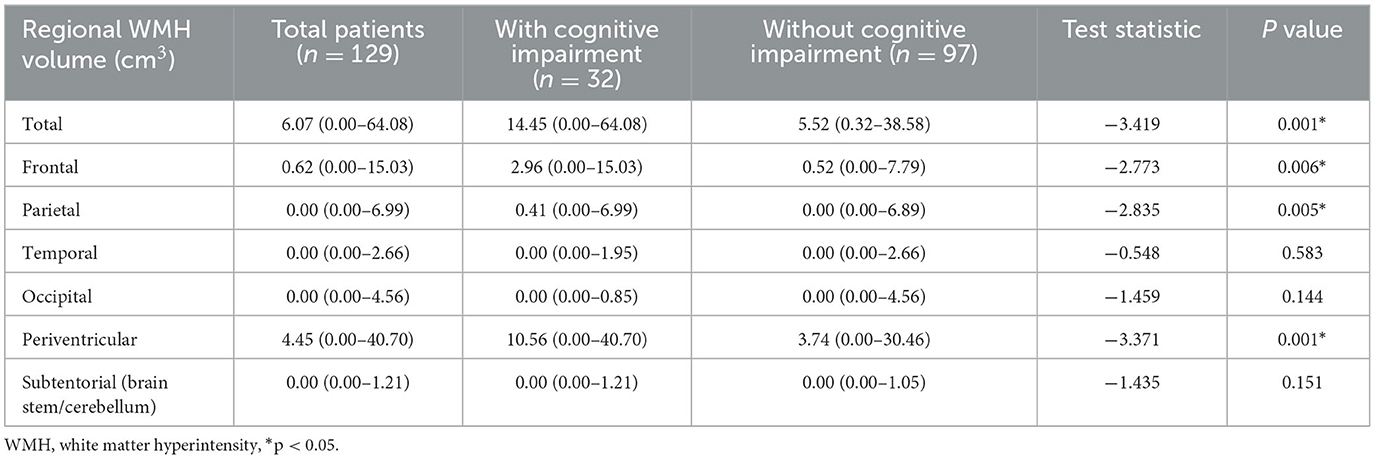
Table 3. Regional WMH volume in acute lacunar stroke patients with and without cognitive impairment.
Independent risk factors for cognitive impairment and the prediction scale
Multivariable logistic regression analysis showed that WMH at the GCC, depression, NIHSS score, and albumin at admission were important determinants of long-term cognitive impairment after ALS (Table 4). Regarding regional WMH volume, only frontal WMH volume was a significant predictor of cognitive impairment, even though both parietal and periventricular WMH volume were also entered into the regression model. Thus, depression (OR = 6.252, P = 0.029), NIHSS score (OR = 1.24, P = 0.011), albumin level (OR = 0.841, P = 0.032), WMH at the GCC (OR = 3.1, P = 0.033), and frontal WMH volume (OR = 1.18, P = 0.04) were used to construct a cognitive prediction scale.
Discussion
This was a prospective study on PSCI, with a 6-month follow-up period, in acute lacunar stroke patients. In this study, we identified depression, plasma albumin, and NIHSS score at admission as independent risk factors for the occurrence of persistent PSCI in the 6-month time course. We further found a significant positive correlation between baseline frontal WMH volume and long-term cognitive impairment, whereas periventricular WMH volume did not correlate with cognition, although this volume was higher among the cognitive impairment group than the non-cognitive impairment group. The study also demonstrated that the occurrence rate of GCC WMH at baseline was statistically significantly different between the cognitive impairment and non-cognitive impairment groups.
Small vessel-related ischemic stroke, or acute lacunar stroke, is one of the common stroke subtypes (35, 36). Although these patients have a small infarct, they usually carry a higher risk of cognitive impairment than would be expected (36). Another recent review summarized domain-specific cognitive impairment after lacunar stroke; the results showed that such dysfunction was comprehensive rather than domain-specific (5). Furthermore, cognitive impairment after lacunar stroke is associated with recurrent stroke and death. Acute lacunar stroke is not solely lacunar infarct; it is a part of the spectrum of CSVD, which is a systemic pathology that influences the brain extensively. The presence of cognitive impairment after lacunar stroke is a symbol of the unmasked microangiopathy (37, 38).
WMH is widely recognized as an independent risk factor for cognitive impairment. A meta-analysis showed that WMH is associated with substantially increased risks of long-term cognitive impairment and all-cause dementia (7). WMH may cause cognitive impairment via several mechanisms. First, white matter lesions may disrupt the subcortical neural networks, such as the frontal subcortical circuit, the frontal thalamus circuit, and the dorsolateral prefrontal and cingulate circuits (39–41). Second, WMH has been shown to be related to dementia-related pathological processes, including Wallerian degeneration or lack of myelin occurring secondary to neuronal loss (42). Furthermore, WMH may contribute to the accumulation of amyloid-β proteins, which is secondary to the impaired drainage of extravascular proteins along blood vessels (43).
Regarding the influence of CSVD-related WMH on cognitive impairment in ALS patients, our study indicated that baseline Fazekas grades of both periventricular WMH and deep WMH were relevant; this result was similar to those of other studies (44). Measurement of the severity of WMH is mostly based on semiquantitative visual rating scales such as the Fazekas visual grade or Scheltens grade (45). Many population-based studies have identified an association between WMH severity and cognitive decline. Recently, volumetric measures of WMH were employed to assess contributions to cognitive impairment sensitively and reliably, which added additional value to WMH grades. Cross-sectional studies that have used WMH volume have suggested that a higher volume of WMH is associated with greater impairments in global cognition as well as perceptional speed, memory, processing speed, and executive function; however, these studies have showed a weak evidential effect (46–48). While some studies have investigated the effects of total WMH volume on cognition, the regional specificity of WMH volume has rarely been explored. Sheline et al. reported that the strategic location of WMH is critical in late-life depression (49). Another study discovered that periventricular hyperintensity in the frontal caps and occipital WMHs were strong predictors of balance and gait impairment (50).
This study focused on the effect of baseline regional WMH volume on long-term cognitive impairment in ALS patients. We found that frontal WMH volume was statistically significantly associated with long-term cognitive impairment in ALS patients after adjustment for NIHSS score, albumin, and depression. Frontal lobe WMH volume is correlated with age, but this is not true for other lobes, such as the temporal lobes (51). Furthermore, CSVD usually causes underlying dysfunction of frontal-subcortical circuits; a higher frontal WMH volume may represent a preexisting strike against cognitive reserves. Frontal lobe WMHs have been reported to be associated with cognition in certain other populations, such as PD patients (52), patients with objectively defined subtle cognitive decline (Obj-SCD) (53), and healthy older adults (22). However, the findings of the current study were contrary to those of a previous study suggesting that WMH volume in the parietal lobe, rather than the frontal lobe, is a predictor of the timing of onset of dementia in the community (21). Therefore, more systematic and large-scale studies are needed in the future. Regional WMH volume would provide more quantitative information for clinical research, and the researchers could obtain WMH topography using regional WMH volume in order to better understand the underlying mechanisms.
In this study, we additionally found that the baseline presence of GCC WMH was independently related to persistent cognitive impairment in ALS patients. Pozorski et al. found that impaired memory in PD patients was associated with a higher probability of WMH in and adjacent to the GCC (52). However, Garnier-Crussard et al. showed that WMH in the larger splenium of the corpus callosum (SCC) is a core feature in Aβ-positive AD patients (54). This differs from the findings presented here. Owing to the extensive structural connections of the corpus callosum with the frontal, temporal, parietal, and occipital lobes of the bilateral cerebral hemispheres, it plays a crucial role in the functions of movement, sensation, language, visual discrimination and perception, auditory localization, and accommodation and coordination of information. A study by Raghavan et al. has reported reduced fractional anisotropy (FA) of the GCC to be a cerebrovascular disease marker and a predictor of longitudinal cognitive impairment with the use of diffusion tensor imaging (DTI) (55). Additionally, several lines of evidence indicate the existence of a relationship between CC and vascular disease (56), suggesting that the GCC is the region most vulnerable to the effects of vascular disease, as the SCC would be an important hub in AD, located in the vicinity of brain areas that are the most sensitive to AD pathology (57).
Our study has both strengths and limitations. A main strength was the homogenous sample of recruited patients with symptomatic CSVD-related lacunar stroke. There have been a few comparable studies analyzing the effect of baseline CSVD on PSCI in cohorts who have experienced heterogeneous forms of stroke with different etiologies and infarct lesion sizes (58–61). Moreover, our longitudinal design allowed assessment for long-term cognitive impairment.
However, our study also had several limitations. First, this was a monocentric and small-sample study. We defined several exclusion criteria, which might affect our results and conclusions. Therefore, sampling bias and possible selection bias should be considered. Second, some participants were lost to follow-up, and no comparison of the baseline characteristics was made between the patients lost to follow-up and those included in the study. Thus, withdrawal bias could not be ruled out. Third, cognitive evaluation was performed only using the MoCA scale for global cognitive status; more extensive neuropsychological assessments for distinct cognitive domains should be carried out. Fourth, we did not know the patients' pre-stroke cognitive status, although we excluded those who had severe cognitive impairment or dementia before falling ill.
Furthermore, several patients were excluded for reasons of aphasia or disturbance of consciousness at admission, and yet, these patients may suffer from more severe stroke. Therefore, patients included in our study may have had relatively mild stroke attacks. Finally, the cross-sectional and observational design of the study prevented us from assessing the dynamic evolution of WMH.
Conclusion
Our study is one of a few existing studies to observe an association between volumetric analyses of regional WMH and cognitive impairment, providing new evidence that frontal WMH volume and the presence of WMH at the GCC at baseline are the core features of long-term cognitive impairment after ALS. Carefully established insights into regional WMH via brain magnetic resonance may greatly help to diagnose ALS patients with a higher risk of long-term cognitive impairment.
Data availability statement
The raw data supporting the conclusions of this article will be made available by the authors, without undue reservation.
Ethics statement
The studies involving humans were approved by Research Ethics Committee of First Affiliated Hospital of Soochow University. The studies were conducted in accordance with the local legislation and institutional requirements. The participants provided their written informed consent to participate in this study.
Author contributions
TL: Data curation, Investigation, Writing—original draft. MY: Investigation, Writing—original draft. GY: Methodology, Software, Writing—review and editing. SD: Methodology, Writing—review and editing. YZ: Data curation, Methodology, Writing—review and editing. YQ: Methodology, Writing—review and editing, Investigation. DD: Formal analysis, Investigation, Writing—review and editing. MZ: Software, Supervision, Writing—review and editing. QF: Supervision, Validation, Visualization, Writing—review and editing.
Funding
The author(s) declare financial support was received for the research, authorship, and/or publication of this article. This work was supported by the General Project of the National Natural Science Foundation of China (82071300).
Acknowledgments
The authors thank all the participating individuals for their contributions to this study. Further, the authors would like to thank all the centers and their staff members involved in the study.
Conflict of interest
GY was employed by Suzhou Jiasheng Medical Instrument Co., Ltd.
The remaining authors declare that the research was conducted in the absence of any commercial or financial relationships that could be construed as a potential conflict of interest.
Publisher's note
All claims expressed in this article are solely those of the authors and do not necessarily represent those of their affiliated organizations, or those of the publisher, the editors and the reviewers. Any product that may be evaluated in this article, or claim that may be made by its manufacturer, is not guaranteed or endorsed by the publisher.
Abbreviations
ACD, all-cause dementia; ALS, acute lacunar stroke; CHD, coronary heart disease; CMB, cerebral microbleed; CSVD, cerebral small vessel disease; DTI, diffusion tensor imaging; DWI, diffusion-weighted imaging; DWMH, deep white matter hyperintensity; EPVS, enlarged perivascular space; FA, fractional anisotropy; GCC, genu of the corpus callosum; HAMD-17, 17-item Hamilton Depression Scale; MoCA, Montreal Cognitive Assessment; mRS, modified Rankin Scale; NIHSS, National Institutes of Health Stroke Scale; Obj-SCD, objectively defined subtle cognitive decline; PSCI, post-stroke cognitive impairment; PWMH, periventricular white matter hyperintensity; SCC, splenium of the corpus callosum; SD, standard deviation; SWI, susceptibility-weighted imaging; T2-FLAIR, T2-fluid-attenuated inversion recovery; WMH, white matter hyperintensity.
References
1. Jiang S, Wu S, Zhang S, Wu B. Advances in understanding the pathogenesis of lacunar stroke: from pathology and pathophysiology to neuroimaging. Cerebrovasc Dis. (2021) 50:588–96. doi: 10.1159/000516052
2. Ross GW, Petrovitch H, White LR, Masaki KH, Li CY, Curb JD, et al. Characterization of risk factors for vascular dementia: the Honolulu-Asia Aging Study. Neurology. (1999) 53:337–43. doi: 10.1212/WNL.53.2.337
3. Barba R, Martinez-Espinosa S, Rodriguez-Garcia E, Pondal M, Vivancos J, Del Ser T. Poststroke dementia: clinical features and risk factors. Stroke. (2000) 31:1494–501. doi: 10.1161/01.STR.31.7.1494
4. Kwon HS, Lee D, Lee MH, Yu S, Lim JS, Yu KH, et al. Post-stroke cognitive impairment as an independent predictor of ischemic stroke recurrence: PICASSO sub-study. J Neurol. (2020) 267:688–93. doi: 10.1007/s00415-019-09630-4
5. Edwards JD, Jacova C, Sepehry AA, Pratt B, Benavente OR. A quantitative systematic review of domain-specific cognitive impairment in lacunar stroke. Neurology. (2013) 80:315–22. doi: 10.1212/WNL.0b013e31827deb85
6. Kwan A, Wei J, Dowling NM, Power MC, Nadareishvili Z, Group SPSS. Cognitive Impairment after Lacunar Stroke and the Risk of Recurrent Stroke and Death. Cerebrovasc Dis. (2021) 50:383–9. doi: 10.1159/000514261
7. Hu HY, Ou YN, Shen XN, Qu Y, Ma YH, Wang ZT, et al. White matter hyperintensities and risks of cognitive impairment and dementia: a systematic review and meta-analysis of 36 prospective studies. Neurosci Biobehav Rev. (2021) 120:16–27. doi: 10.1016/j.neubiorev.2020.11.007
8. Greenberg SM. Small vessels, big problems. N Engl J Med. (2006) 354:1451–3. doi: 10.1056/NEJMp068043
9. Pantoni L. Cerebral small vessel disease: from pathogenesis and clinical characteristics to therapeutic challenges. Lancet Neurol. (2010) 9:689–701. doi: 10.1016/S1474-4422(10)70104-6
10. Shaaban CE, Jorgensen DR, Gianaros PJ, Mettenburg J, Rosano C. Cerebrovascular disease: neuroimaging of cerebral small vessel disease. Prog Mol Biol Transl Sci. (2019) 165:225–55. doi: 10.1016/bs.pmbts.2019.07.008
11. Vermeer SE, Prins ND, den Heijer T, Hofman A, Koudstaal PJ, Breteler MM. Silent brain infarcts and the risk of dementia and cognitive decline. N Engl J Med. (2003) 348:1215–22. doi: 10.1056/NEJMoa022066
12. Foschi M, Pavolucci L, Rondelli F, Spinardi L, Favaretto E, Filippini M, et al. Prospective observational cohort study of early recurrent TIA: features, frequency, and outcome. Neurology. (2020) 95:e1733–44. doi: 10.1212/WNL.0000000000010317
13. Staals J, Makin SD, Doubal FN, Dennis MS, Wardlaw JM. Stroke subtype, vascular risk factors, and total MRI brain small-vessel disease burden. Neurology. (2014) 83:1228–34. doi: 10.1212/WNL.0000000000000837
14. Yamamoto Y, Ohara T, Nagakane Y, Tanaka E, Morii F, Koizumi T, et al. Chronic kidney disease, 24-h blood pressure and small vessel diseases are independently associated with cognitive impairment in lacunar infarct patients. Hypertens Res. (2011) 34:1276–82. doi: 10.1038/hr.2011.118
15. de Groot JC, de Leeuw FE, Oudkerk M, van Gijn J, Hofman A, Jolles J, et al. Cerebral white matter lesions and cognitive function: the Rotterdam Scan Study. Ann Neurol. (2000) 47:145–51. doi: 10.1002/1531-8249(200002)47:2<145::aid-ana3>3.3.co;2-g
16. Inzitari D, Pracucci G, Poggesi A, Carlucci G, Barkhof F, Chabriat H, et al. Changes in white matter as determinant of global functional decline in older independent outpatients: three year follow-up of LADIS (leukoaraiosis and disability) study cohort. BMJ. (2009) 339:b2477. doi: 10.1136/bmj.b2477
17. Stenset V, Hofoss D, Johnsen L, Skinningsrud A, Berstad AE, Negaard A, et al. White matter lesion severity is associated with reduced cognitive performances in patients with normal CSF Abeta42 levels. Acta Neurol Scand. (2008) 118:373–8. doi: 10.1111/j.1600-0404.2008.01045.x
18. Wang YL, Chen W, Cai WJ, Hu H, Xu W, Wang ZT, et al. Associations of white matter hyperintensities with cognitive decline: a longitudinal study. J Alzheimers Dis. (2020) 73:759–68. doi: 10.3233/JAD-191005
19. Biesbroek JM, Weaver NA, Hilal S, Kuijf HJ, Ikram MK, Xu X, et al. Impact of strategically located white matter hyperintensities on cognition in memory clinic patients with small vessel disease. PLoS ONE. (2016) 11:e0166261. doi: 10.1371/journal.pone.0166261
20. Kim S, Choi SH, Lee YM, Kim MJ, Kim YD, Kim JY, et al. Periventricular white matter hyperintensities and the risk of dementia: a CREDOS study. Int Psychogeriatr. (2015) 27:2069–77. doi: 10.1017/S1041610215001076
21. Brickman AM, Provenzano FA, Muraskin J, Manly JJ, Blum S, Apa Z, et al. Regional white matter hyperintensity volume, not hippocampal atrophy, predicts incident Alzheimer disease in the community. Arch Neurol. (2012) 69:1621–7. doi: 10.1001/archneurol.2012.1527
22. Rizvi B, Narkhede A, Last BS, Budge M, Tosto G, Manly JJ, et al. The effect of white matter hyperintensities on cognition is mediated by cortical atrophy. Neurobiol Aging. (2018) 64:25–32. doi: 10.1016/j.neurobiolaging.2017.12.006
23. Adams HP, Bendixen BH, Kappelle LJ, Biller J, Love BB, Gordon DL, et al. Classification of subtype of acute ischemic stroke. Definitions for use in a multicenter clinical trial TOAST Trial of Org 10172 in acute stroke treatment. Stroke. (1993) 24:35–41. doi: 10.1161/01.STR.24.1.35
24. Lawrence AJ, Brookes RL, Zeestraten EA, Barrick TR, Morris RG, Markus HS. Pattern and rate of cognitive decline in cerebral small vessel disease: a prospective study. PLoS ONE. (2015) 10:e0135523. doi: 10.1371/journal.pone.0135523
25. Allan LM, Rowan EN, Firbank MJ, Thomas AJ, Parry SW, Polvikoski TM, et al. Long term incidence of dementia, predictors of mortality and pathological diagnosis in older stroke survivors. Brain. (2011) 134:3716–27. doi: 10.1093/brain/awr273
26. Tang A, Liu S, Wang Z, Shao H, Cai X, Li T. A new nomogram model for individualized prediction of cognitive impairment in patients with acute ischemic stroke. J Stroke Cerebrovasc Dis. (2022) 31:106515. doi: 10.1016/j.jstrokecerebrovasdis.2022.106515
27. Zhou L, Chen L, Ma L, Diao S, Qin Y, Fang Q, et al. A new nomogram including total cerebral small vessel disease burden for individualized prediction of early-onset depression in patients with acute ischemic stroke. Front Aging Neurosci. (2022) 14:922530. doi: 10.3389/fnagi.2022.922530
28. Arba F, Inzitari D, Ali M, Warach SJ, Luby M, Lees KR, et al. Small vessel disease and clinical outcomes after IV rt-PA treatment. Acta Neurol Scand. (2017) 136:72–7. doi: 10.1111/ane.12745
29. Liu X, Li T, Diao S, Cai X, Kong Y, Zhang L, et al. The global burden of cerebral small vessel disease related to neurological deficit severity and clinical outcomes of acute ischemic stroke after IV rt-PA treatment. Neurol Sci. (2019) 40:1157–66. doi: 10.1007/s10072-019-03790-x
30. Akisaki T, Sakurai T, Takata T, Umegaki H, Araki A, Mizuno S, et al. Cognitive dysfunction associates with white matter hyperintensities and subcortical atrophy on magnetic resonance imaging of the elderly diabetes mellitus Japanese elderly diabetes intervention trial (J-EDIT). Diabetes Metab Res Rev. (2006) 22:376–84. doi: 10.1002/dmrr.632
31. Bangen KJ, Thomas KR, Weigand AJ, Sanchez DL, Delano-Wood L, Edmonds EC, et al. Pattern of regional white matter hyperintensity volume in mild cognitive impairment subtypes and associations with decline in daily functioning. Neurobiol Aging. (2020) 86:134–42. doi: 10.1016/j.neurobiolaging.2019.10.016
32. DeCarli C, Massaro J, Harvey D, Hald J, Tullberg M, Au R, et al. Measures of brain morphology and infarction in the framingham heart study: establishing what is normal. Neurobiol Aging. (2005) 26:491–510. doi: 10.1016/j.neurobiolaging.2004.05.004
33. Saba L, Lucatelli P, Anzidei M, di Martino M, Suri JS, Montisci R. Volumetric distribution of the white matter hyper-intensities in subject with mild to severe carotid artery stenosis: does the side play a role? J Stroke Cerebrovasc Dis. (2018) 27:2059–66. doi: 10.1016/j.jstrokecerebrovasdis.2018.02.065
34. Wardlaw JM, Valdes Hernandez MC, Munoz-Maniega S. What are white matter hyperintensities made of? Relevance to vascular cognitive impairment. J Am Heart Assoc. (2015) 4:001140. doi: 10.1161/JAHA.114.001140
35. Gorelick PB, Scuteri A, Black SE, Decarli C, Greenberg SM, Iadecola C, et al. Vascular contributions to cognitive impairment and dementia: a statement for healthcare professionals from the american heart association/american stroke association. Stroke. (2011) 42:2672–713. doi: 10.1161/STR.0b013e3182299496
36. Ornello R, Degan D, Tiseo C, Di Carmine C, Perciballi L, Pistoia F, et al. Distribution and temporal trends from 1993 to 2015 of ischemic stroke subtypes: a systematic review and meta-analysis. Stroke. (2018) 49:814–9. doi: 10.1161/STROKEAHA.117.020031
37. Berry C, Sidik N, Pereira AC, Ford TJ, Touyz RM, Kaski JC, et al. Small-vessel disease in the heart and brain: current knowledge, unmet therapeutic need, and future directions. J Am Heart Assoc. (2019) 8:e011104. doi: 10.1161/JAHA.118.011104
38. Toyoda K. Cerebral small vessel disease and chronic kidney disease. J Stroke. (2015) 17:31–7. doi: 10.5853/jos.2015.17.1.31
39. Cummings JL. Frontal-subcortical circuits and human behavior. Arch Neurol. (1993) 50:873–80. doi: 10.1001/archneur.1993.00540080076020
40. Duering M, Gonik M, Malik R, Zieren N, Reyes S, Jouvent E, et al. Identification of a strategic brain network underlying processing speed deficits in vascular cognitive impairment. Neuroimage. (2013) 66:177–83. doi: 10.1016/j.neuroimage.2012.10.084
41. Mamiya PC, Richards TL, Kuhl PK. Right forceps minor and anterior thalamic radiation predict executive function skills in young bilingual adults. Front Psychol. (2018) 9:118. doi: 10.3389/fpsyg.2018.00118
42. Romero-Sevilla R, Casado-Naranjo I, Portilla-Cuenca JC, Duque-de San Juan B, Fuentes JM, Lopez-Espuela F. Vascular risk factors and lesions of vascular nature in magnetic resonance as predictors of progression to dementia in patients with mild cognitive impairment. Curr Alzheimer Res. (2018) 15:671–8. doi: 10.2174/1567205015666180119100840
43. van Westen D, Lindqvist D, Blennow K, Minthon L, Nagga K, Stomrud E, et al. Cerebral white matter lesions - associations with Abeta isoforms and amyloid PET. Sci Rep. (2016) 6:20709. doi: 10.1038/srep20709
44. Fruhwirth V, Enzinger C, Fandler-Hofler S, Kneihsl M, Eppinger S, Ropele S, et al. Baseline white matter hyperintensities affect the course of cognitive function after small vessel disease-related stroke: a prospective observational study. Eur J Neurol. (2021) 28:401–10. doi: 10.1111/ene.14593
45. Chen Y, Wang X, Guan L, Wang Y. Role of white matter hyperintensities and related risk factors in vascular cognitive impairment: a review. Biomolecules. (2021) 11:8. doi: 10.3390/biom11081102
46. Debette S, Markus HS. The clinical importance of white matter hyperintensities on brain magnetic resonance imaging: systematic review and meta-analysis. BMJ. (2010) 341:c3666. doi: 10.1136/bmj.c3666
47. Kloppenborg RP, Nederkoorn PJ, Geerlings MI, van den Berg E. Presence and progression of white matter hyperintensities and cognition: a meta-analysis. Neurology. (2014) 82:2127–38. doi: 10.1212/WNL.0000000000000505
48. Sivakumar L, Riaz P, Kate M, Jeerakathil T, Beaulieu C, Buck B, et al. White matter hyperintensity volume predicts persistent cognitive impairment in transient ischemic attack and minor stroke. Int J Stroke. (2017) 12:264–72. doi: 10.1177/1747493016676612
49. Sheline YI, Price JL, Vaishnavi SN, Mintun MA, Barch DM, Epstein AA, et al. Regional white matter hyperintensity burden in automated segmentation distinguishes late-life depressed subjects from comparison subjects matched for vascular risk factors. Am J Psychiat. (2008) 165:524–32. doi: 10.1176/appi.ajp.2007.07010175
50. Ogama N, Sakurai T, Shimizu A, Toba K. Regional white matter lesions predict falls in patients with amnestic mild cognitive impairment and Alzheimer's disease. J Am Med Dir Assoc. (2014) 15:36–41. doi: 10.1016/j.jamda.2013.11.004
51. Kim JH, Hwang KJ, Kim JH, Lee YH, Rhee HY, Park KC. Regional white matter hyperintensities in normal aging, single domain amnestic mild cognitive impairment, and mild Alzheimer's disease. J Clin Neurosci. (2011) 18:1101–6. doi: 10.1016/j.jocn.2011.01.008
52. Pozorski V, Oh JM, Okonkwo O, Krislov S, Barzgari A, Theisen F, et al. Cross-sectional and longitudinal associations between total and regional white matter hyperintensity volume and cognitive and motor function in Parkinson's disease. Neuroimage Clin. (2019) 23:101870. doi: 10.1016/j.nicl.2019.101870
53. Calcetas AT, Thomas KR, Edmonds EC, Holmqvist SL, Edwards L, Bordyug M, et al. Increased regional white matter hyperintensity volume in objectively-defined subtle cognitive decline and mild cognitive impairment. Neurobiol Aging. (2022) 118:1–8. doi: 10.1016/j.neurobiolaging.2022.06.002
54. Garnier-Crussard A, Bougacha S, Wirth M, Dautricourt S, Sherif S, Landeau B, et al. White matter hyperintensity topography in Alzheimer's disease and links to cognition. Alzheimers Dement. (2022) 18:422–33. doi: 10.1002/alz.12410
55. Raghavan S, Przybelski SA, Reid RI, Graff-Radford J, Lesnick TG, Zuk SM, et al. Reduced fractional anisotropy of the genu of the corpus callosum as a cerebrovascular disease marker and predictor of longitudinal cognition in MCI. Neurobiol Aging. (2020) 96:176–83. doi: 10.1016/j.neurobiolaging.2020.09.005
56. Vemuri P, Lesnick TG, Przybelski SA, Graff-Radford J, Reid RI, Lowe VJ, et al. Development of a cerebrovascular magnetic resonance imaging biomarker for cognitive aging. Ann Neurol. (2018) 84:705–16. doi: 10.1002/ana.25346
57. La Joie R, Perrotin A, Barre L, Hommet C, Mezenge F, Ibazizene M, et al. Region-specific hierarchy between atrophy, hypometabolism, and beta-amyloid (Abeta) load in Alzheimer's disease dementia. J Neurosci. (2012) 32:16265–73. doi: 10.1523/JNEUROSCI.2170-12.2012
58. Auriat AM, Ferris JK, Peters S, Ramirez J, Black SE, Jacova C, et al. The impact of covert lacunar infarcts and white matter hyperintensities on cognitive and motor outcomes after stroke. J Stroke Cerebrovasc Dis. (2019) 28:381–8. doi: 10.1016/j.jstrokecerebrovasdis.2018.10.009
59. Dickie DA, Gardner K, Wagener A, Wyss A, Arba F, Wardlaw JM, et al. Cortical thickness, white matter hyperintensities, and cognition after stroke. Int J Stroke. (2020) 15:46–54. doi: 10.1177/1747493019851291
60. Molad J, Kliper E, Korczyn AD, Ben Assayag E, Ben Bashat D, Shenhar-Tsarfaty S, et al. Only White matter hyperintensities predicts post-stroke cognitive performances among cerebral small vessel disease markers: results from the TABASCO study. J Alzheimers Dis. (2017) 56:1293–9. doi: 10.3233/JAD-160939
Keywords: regional white matter hyperintensity volume, persistent cognitive impairment, acute lacunar infarct, cerebral small vessel disease, neuropsychology
Citation: Li T, Ye M, Yang G, Diao S, Zhou Y, Qin Y, Ding D, Zhu M and Fang Q (2023) Regional white matter hyperintensity volume predicts persistent cognitive impairment in acute lacunar infarct patients. Front. Neurol. 14:1265743. doi: 10.3389/fneur.2023.1265743
Received: 23 July 2023; Accepted: 15 September 2023;
Published: 10 October 2023.
Edited by:
Matteo Foschi, Azienda Unità Sanitaria Locale (AUSL) della Romagna, ItalyReviewed by:
Andrea Surcinelli, Santa Maria delle Croci Hospital, ItalyZhigang Liang, Yantai Yuhuangding Hospital, China
Copyright © 2023 Li, Ye, Yang, Diao, Zhou, Qin, Ding, Zhu and Fang. This is an open-access article distributed under the terms of the Creative Commons Attribution License (CC BY). The use, distribution or reproduction in other forums is permitted, provided the original author(s) and the copyright owner(s) are credited and that the original publication in this journal is cited, in accordance with accepted academic practice. No use, distribution or reproduction is permitted which does not comply with these terms.
*Correspondence: Qi Fang, ZmFuZ3FpXzAwOEAxMjYuY29t; Mo Zhu, c29vY2hvd3podW1vQDE2My5jb20=; Dongxue Ding, d2ludGVyc25vdzE5ODhAMTI2LmNvbQ==
†These authors have contributed equally to this work