- 1VIP Department, Longhua Hospital, Shanghai University of Traditional Chinese Medicine, Shanghai, China
- 2Teaching Affairs Department, Yueyang Hospital of Integrated Traditional Chinese and Western Medicine, Shanghai University of Traditional Chinese Medicine, Shanghai, China
- 3Department of Geriatrics, Longhua Hospital, Shanghai University of Traditional Chinese Medicine, Shanghai, China
Background: Cerebral amyloid angiopathy (CAA) is the most common cause of lobar intracerebral hemorrhage (ICH) in the elderly, and its multifocal and recurrent nature leads to high rates of disability and mortality. Therefore, this study aimed to summarize the evidence regarding the recurrence rate and risk factors for CAA-related ICH (CAA-ICH).
Methods: We performed a systematic literature search of all English studies published in PubMed, Embase, Web of Science, Cochrane Library, Scopus, and CINAHL from inception to June 10, 2023. Studies reporting CAA-ICH recurrence rates and risk factors for CAA-ICH recurrence were included. We calculated pooled odds ratios (ORs) with their corresponding 95% confidence intervals (CIs) using a random/fixed-effects model based on the I2 assessment of heterogeneity between studies. Publication bias was assessed using Egger's test.
Results: Thirty studies were included in the final analysis. Meta-analysis showed that the recurrence rate of CAA-ICH was 23% (95% CI: 18–28%, I2 = 96.7%). The risk factors significantly associated with CAA-ICH recurrence were: previous ICH (OR = 2.03; 95% CI: 1.50–2.75; I2 = 36.8%; N = 8), baseline ICH volume (OR = 1.01; 95% CI: 1–1.02; I2 = 0%; N = 4), subarachnoid hemorrhage (cSAH) (OR = 3.05; 95% CI: 1.86–4.99; I2 = 0%; N = 3), the presence of cortical superficial siderosis (cSS) (OR = 2.04; 95% CI: 1.46–2.83; I2 = 0%; N = 5), disseminated cSS (OR = 3.21; 95% CI: 2.25–4.58; I2 = 16.0%; N = 6), and centrum semiovale-perivascular spaces (CSO-PVS) severity (OR = 1.67; 95% CI: 1.14–2.45; I2 = 0%; N = 4).
Conclusion: CAA-ICH has a high recurrence rate. cSAH, cSS (especially if disseminated), and CSO-PVS were significant markers for recurrent CAA-ICH. The onset of ICH in patients with CAA is usually repeated several times, and recurrence is partly related to the index ICH volume. Identifying clinical and neuroimaging predictors of CAA-ICH recurrence is of great significance for evaluating outcomes and improving the prognosis of patients with CAA-ICH.
Systematic review registration: https://www.crd.york.ac.uk/PROSPERO/display_record.php?RecordID=400240, identifier [CRD42023400240].
1. Introduction
Cerebral amyloid angiopathy (CAA) is an age-related cerebral small vessel disease (SVD) characterized by the progressive deposition of amyloid-β in the vessel walls of the middle and small arteries and capillaries of the cerebral cortex, covering the soft meninges (1, 2). Among the various phenotypes of CAA, spontaneous intracerebral hemorrhage (ICH) is one of the most widely studied, accounting for approximately 5–20% of spontaneous ICH in the elderly (3). Spontaneous ICH is a worldwide public health problem with a mortality rate of 50% within 30 days, and only 20% of patients regain functional independence at 6 months (4). Unlike hypertensive ICH, which usually presents as a deep hemorrhage (usually in the basal ganglia, thalamus, and brainstem), cerebral amyloid angiopathy-related ICH (CAA-ICH) preferentially affects the cortical or cortico-subcortical (lobar) regions, with multiple and recurrent lobar hemorrhages as its typical features (5). Compared with other types of ICH, CAA-ICH is milder when it first occurs (6); however, the risk of recurrence is significantly higher (7). Therefore, the prognosis is often worse and can lead to a higher risk of dementia, disability, and even death.
Because of the superficial location of CAA-ICH, surgical removal of the intracerebral hematoma is currently an effective and feasible surgical treatment; however, its safety is still controversial, and its suitability for patients with recurrent ICH remains undetermined (8–12). In addition to surgery, specific treatments are currently lacking. Secondary prevention of spontaneous ICH is currently an accepted conservative treatment that includes hemostasis, strict blood pressure control, and brain support therapy (4). Given the absence of targeted treatment, we should focus on the early identification of potential risk factors for the recurrence of CAA-ICH, which is of great significance in preventing the recurrence of ICH and improving disease prognosis.
There may be a significant unrecognized burden of CAA in older populations until now (13). The number of studies on CAA-ICH is limited and there is much room for future exploration. Recent meta-analyses and systematic reviews have shown that neuroimaging markers of SVD are not only related to the pathophysiology of CAA and participate in the diagnosis of CAA, but also play a significant role in predicting the outcome after the occurrence of CAA-ICH (14–16). Computed tomography (CT) and magnetic resonance imaging (MRI) are essential examinations for patients with acute ICH. Neuroimaging markers provide a great opportunity to explore the risk factors associated with CAA-ICH recurrence and predict rebleeding. MRI-based biomarkers of CAA-associated small vessel injury, including cerebral microbleeds (CMBs), cortical superficial siderosis (cSS), centrum semiovale-perivascular spaces (CSO-PVS), and white matter hyperintensities (WMH), have been widely investigated and considered for the diagnosis of CAA and prediction of ICH recurrence (17, 18). These MRI markers may be closely associated with the pathophysiology of CAA-ICH. Therefore, it has been proposed that setting an ordinal scale representing the total burden of small vessel disease in CAA by considering the four most characteristic MRI markers mentioned above seems to have a greater advantage in predicting CAA-ICH recurrence (19). In addition, for patients with acute ICH, CT is easier to perform within a short period; therefore, the baseline CT imaging characteristics of ICH are also worthy of attention. However, the number of studies on the risk factors for CAA-ICH recurrence is limited, and the factors that strongly suggest the recurrence of CAA-ICH are still inconclusive. This has caused great trouble in the treatment and prevention of recurrence in patients with CAA-ICH.
This study aimed to conduct a systematic review and meta-analysis of previous studies to estimate the CAA-ICH recurrence rate and summarize the risk factors associated with its recurrence to improve the prognosis and treatment outcomes of patients at a high risk of recurrence.
2. Materials and methods
This systematic review followed the Preferred Reporting Items for Systematic Reviews and Meta-Analyses (PRISMA) guidelines (20) (Supplementary Table 1). The study protocol was registered in the International Registry of Prospective Systematic Reviews (PROSPERO; registration no.: CRD42023400240).
2.1. Search strategy
Two authors systematically and independently searched for literature published in PubMed, EMBASE, Web of Science, Cochrane Library, Scopus, and CINAHL from the establishment of the database until June 10, 2023. We combined the subject words “cerebral amyloid angiopathy,” “cerebral hemorrhage,” and related free words to construct a search strategy to obtain relevant literature on the prevalence of recurrence and risk factors for CAA-ICH. The search was not limited to the characteristics of the study population (age, sex, or race). The list of references for each study included in the meta-analysis was manually reviewed to identify other potentially eligible studies. The full search strategy is shown in Supplementary Table 2.
2.2. Inclusion and exclusion criteria
The titles and abstracts of all search results were screened first, and the full text of the qualified literature was independently reviewed by two authors. Disagreements were resolved by consensus and consultation with senior investigators.
Studies included in this review had to meet the following criteria: (1) participants were survivors of acute lobar ICH events, regardless of age or sex; (2) the participants were diagnosed with CAA based on histology or Boston diagnostic criteria (In fact, the Boston criteria encompass histologic diagnosis. Histological diagnosis was emphasized in order to include as many relevant studies as possible); (3) ICH recurrence was defined as a new symptomatic ICH confirmed by imaging after the index ICH; (4) studies reporting CAA-ICH recurrence rates or any risk factor odds ratio (ORs), relative risk ratio (RRs), hazard ratio (HRs), 95% confidence interval (CIs), and equivalent data; (5) retrospective or prospective English original studies.
The following studies were excluded: (1) studies that did not provide the prevalence of CAA-ICH recurrence or could not obtain effect sizes for any risk factor for CAA-ICH recurrence; (2) studies with baseline and follow-up numbers of patients with CAA-ICH <30; (3) studies reporting results from overlapping patient cohorts were included after discussion by two authors, with priority given to studies providing additional or more detailed information; and (4) reviews, letters, abstracts of meetings, Supplementary material, case reports, and case series studies.
2.3. Data extraction and quality assessment
We used a standard pre-extraction table to extract the data required for this meta-analysis and further revised the table based on the preliminary extraction results. Two authors independently extracted the following information: first author, year of publication, study design, geographic region, sample characteristics (e.g., sample size, mean age or range, male proportion, and proportion of defined and probable patients with CAA), duration of follow-up, and number of relapses/total number of follow-ups (Table 1). If multiple articles were published on the same cohort, then the most informative report was included. When a follow-up study included multiple time points, we included the data for the longest follow-up time. We only counted the risk factors that were studied two or more times. In addition, we extracted the necessary imaging methods at baseline, diagnostic criteria for CAA, starting point of the study and relevant risk factors (Table 2).
We preferentially included the results from analyses adjusted for multiple factors to extract OR (95% CI) in the models with the largest number of variables. In the absence of multifactor analysis, the results of the single-factor analysis were included. The Newcastle-Ottawa Scale (NOS) recommended by the Cochrane manual was used to assess the quality of the included studies. It consists of eight items and three components: selection, comparability, and outcomes (cohort studies) or exposure (case-control studies). The highest quality studies scored as high as 9. A study with an NOS score ≥7 indicated high quality. The NOS scores of each study are presented in Supplementary Table 3. Two authors independently evaluated the quality of each included study, and disputes were fully discussed with senior investigators to resolve any differences in the data extraction or quality assessment.
2.4. Statistical analysis
STATA/SE 16.0 was used for all statistical analyses and the significance level was set at 0.05. The “metan” function of STATA/SE 16.0 was used to estimate the recurrence rate of CAA-ICH and the pooled ORs (95% CI) of associated risk factors. Heterogeneity in all included studies was assessed and quantified using Cochrane Q and I2 statistics, respectively. If I2 > 50%, the heterogeneity of the included studies was considered significant, and a random-effects model was used to summarize the results. If I2 ≤ 50%, the fixed-effects model was used. Heterogeneity was also addressed by meta-regression and subgroup analysis. Sensitivity analysis was used to assess the stability of the merger effect. Funnel plot and Egger's test were used to estimate publication bias.
2.5. Studies quality
The quality of evidence was assessed using the Grading of Recommendations, Assessments, Developments and Evaluations (GRADE) system (51), which resulted in a high, moderate, low, or very low level of evidence. Disagreements encountered during the assessment process could be resolved by mutual consultation between the two authors.
3. Results
3.1. Study selection and study characteristics
After conducting the literature search, we initially identified 5,803 articles, including 918 in PubMed, 2,265 in Web of Science, 1,439 in EMBASE, 55 in the Cochrane Library, 138 in Scopus, and 988 in CINAHL. After excluding duplicate studies, 3,311 unrelated studies among the 3,373 were further excluded based on their title/abstract. The remaining 62 studies were eligible for full-text review, of which 30 met the inclusion and exclusion criteria and were included in the meta-analysis (21–50). Figure 1 presents a flowchart of the literature selection process.
Among the included studies, 28 cohort studies (21–24, 26–30, 32–50) and two case-control studies (25, 31) with publication years ranging from 2000–2023, and baseline characteristics are presented in Table 1. Of these, 11 were from Asia (21, 23, 26–30, 37, 40, 41, 48), five from Europe (24, 31, 43, 45, 47), and 14 from the Americas (22, 25, 32–36, 38, 39, 42, 44, 46, 49, 50). The sample size of the included studies varied from 32 (30) to 7,857 (26), and the mean follow-up duration varied from 1 month (21) to 71.2 months (22). Each study reported the number of symptomatic ICH relapses during the study period and the total study population. Sixteen studies were used to further explore the risk factors associated with CAA-ICH recurrence (23, 25, 26, 28, 29, 31–33, 35, 36, 38–40, 46, 48, 50) (Table 2). In addition, the NOS scores for the quality of the included studies ranged from 7 to 9 points, indicating high quality (Supplementary Table 3).
Finally, we analyzed 16 potential risk factors for CAA-ICH recurrence, including general demographic characteristics: age, sex; previous history of hypertension, diabetes, dyslipidemia, previous ICH (i.e., hemorrhagic stroke before the index lobar hemorrhage); use of antithrombotic drugs: antiplatelet or anticoagulation therapy; imaging manifestations visible on CT: baseline ICH volume, presence of subarachnoid hemorrhage (cSAH), presence of intraventricular hemorrhage (IVH), MRI-based imaging markers including lobar CMBs, cSS, CSO-PVS, WMH volume (WMH volume refers to the total volume of WMH measured on liquid-attenuated inversion recovery MRI using a computer-assisted measurement method), and total MRI burden of SVD.
3.2. Recurrence rate
Twenty-eight studies were included to calculate the recurrence rate of CAA-ICH. In the included studies, CAA-ICH recurrence rates ranged from 4.7 to 42.2%, with pooled recurrence rates of 23% (95% CI:18–28%) and significant heterogeneity (I2 = 96.7%; P < 0.001) (Figure 2).
The meta-regression analysis showed that age (P = 0.129), gender (P = 0.362), hypertension (P = 0.857) and diabetes (P = 0.84) were not sources of heterogeneity.
Subgroup analysis by region: The recurrence rate of CAA-ICH in Asian population was 21% (95% CI: 13–29%; I2 = 92.6%; P < 0.001; N = 10); in the Americas, the recurrence rate was 25% (95% CI: 17–33%; I2 = 97.7%; P < 0.001; N = 13); in Europe, the recurrence rate was 20% (95% CI: 11–30%; I2 = 85.8%; P < 0.001; N = 5) (Supplementary Figure 1A).
Subgroup analysis based on follow-up duration: The recurrence rate at 1 year or less was 8% (95% CI: 5–12%; I2 = 77.2%; P = 0.002; N = 5), and the recurrence rate after 1 year was 24% (95% CI: 17–31%; I2 = 84.4%; P < 0.001; N = 21). The subgroup analysis showed that region and follow-up duration were not sources of study heterogeneity in CAA-ICH recurrence rates (Supplementary Figure 1B).
3.3. Risk factors for CAA-ICH recurrence
3.3.1. General demographic characteristics
Eleven studies investigated the association between age and CAA-ICH recurrence. Results were summarized using a random effects model (OR = 1.02; 95% CI: 0.99–1.05; P = 0.19; I2 = 70.4%; P < 0.001). The pooled results of seven studies involving the relationship between sex and CAA-ICH recurrence showed no significant association between sex and CAA-ICH recurrence (OR = 0.95; 95% CI: 0.77–1.17; P = 0.62; I2 = 0%; P = 0.99) (Supplementary Table 4).
3.3.2. Medical history
The association between hypertension and CAA-ICH recurrence was analyzed in seven studies. The pooled results using a fixed-effect model of 7 studies suggested that there was no significant difference in recurrence rate between patients with hypertension and patients without hypertension (OR = 0.81; 95% CI: 0.61–1.08; P = 0.15; I2 = 29.9%; P = 0.20) (Figure 3). Six studies analyzed the influence of diabetes mellitus on CAA-ICH recurrence. Our pooled results of six studies using a fixed-effect model indicated that there was no significant relationship between diabetes mellitus and CAA-ICH recurrence, with no heterogeneity among included studies (OR = 0.87; 95% CI: 0.64–1.19; P = 0.39; I2 = 2.9%; P = 0.40) (Figure 3). Four studies reported an association between dyslipidemia and the recurrence of CAA-ICH. Our pooled results of four studies indicated that there was no significant relationship between dyslipidemia and CAA-ICH recurrence (OR = 1.02; 95% CI: 0.80–1.30; P = 0.88; I2 = 0%; P = 0.80) (Figure 3). Eight studies analyzed the influence of previous ICH on CAA-ICH recurrence. The pooled evidence with a fixed-effect model suggested that patients with CAA-ICH with previous ICH were more susceptible to relapse (OR = 2.03; 95% CI: 1.5–2.75; P < 0.001; I2 = 36.8%; P = 0.14) (Figure 3).
3.3.3. Use of antithrombotic drugs
The impact of anticoagulation therapy on CAA-ICH recurrence has been reported in three studies. We used a random effects model to summarize the results, which showed that there was no significant relationship between anticoagulation and CAA-ICH recurrence (OR = 1.72; 95% CI: 0.16–18.13; P = 0.651; I2 = 74.2%; P = 0.02) (Supplementary Table 4).
Four studies analyzed the influence of antiplatelet therapy on CAA-ICH recurrence. Our analysis results suggested no significant relationship between antiplatelet therapy and the recurrence of CAA-ICH. We used a random-effect model because of the significant heterogeneity observed among these studies (OR = 1.66; 95% CI: 0.66–4.15; P = 0.28; I2 = 69.7%; P = 0.02) (Supplementary Table 4).
3.3.4. ICH imaging manifestations at baseline based on CT
Four studies reported an association between baseline ICH volume and CAA-ICH recurrence. The pooled results with a fixed-effects model showed that the larger the baseline ICH volume, the higher the risk of CAA-ICH recurrence (OR = 1.01; 95% CI: 1–1.02; P = 0.004; I2 = 0%; P = 0.51) (Figure 4). A fixed-effect model was used to summarize the results of two studies and showed that there was no significant association between the presence of IVH and CAA-ICH recurrence (OR = 1.35; 95% CI: 0.49–3.78; P = 0.56; I2 = 0%; P = 0.36) (Figure 4). The pooled results with a fixed-effect model of three studies suggested that patients with CAA-ICH along with cSAH were more susceptible to relapse (OR = 3.05; 95% CI: 1.86–4.99; P < 0.001; I2 = 0%; P = 0.44) (Figure 4).
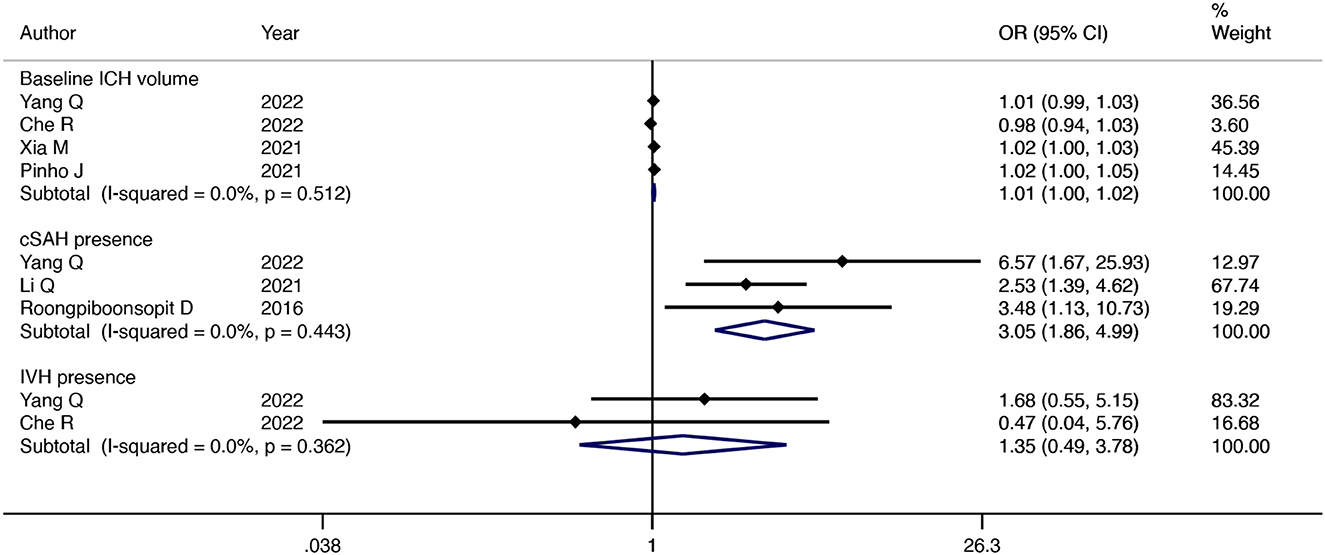
Figure 4. Forest plot showing the association between ICH imaging manifestations at baseline based on CT and CAA-ICH recurrence.
3.3.5. MRI imaging markers and total MRI burden of SVD
Five studies investigated the association between lobar CMBs presence and CAA-ICH recurrence. Our pooled results of five studies indicated that there was no significant relationship between lobar CMBs count and CAA-ICH recurrence, with no heterogeneity among included studies (OR = 1.00; 95% CI: 0.99–1.00; P = 0.57; I2 = 0%; P = 0.52) (Figure 5A). In addition, the pooled results of four studies showed that lobar CMBs>5 was not significantly associated with CAA-ICH recurrence (OR = 1.61; 95% CI: 0.54–4.81; P = 0.39; I2 = 75.3%; P = 0.007) (Figure 5B).
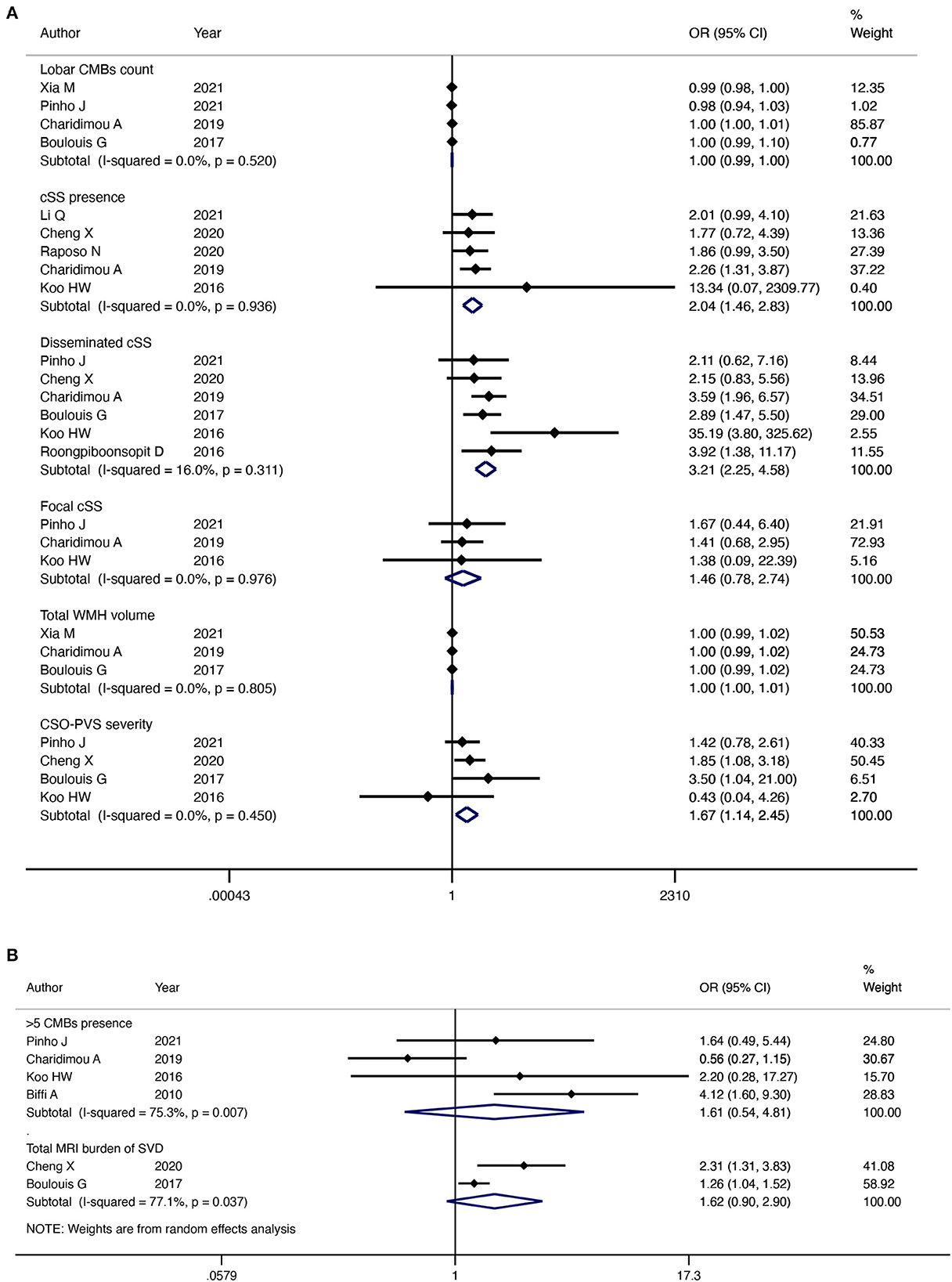
Figure 5. Forest plot showing the association between MRI imaging markers and CAA-ICH recurrence. I2 ≤ 50%, the fixed effects model was used (A), I2 > 50%, the random effects model was used (B).
Five studies reported an association between the presence of cSS and recurrence of CAA-ICH. The pooled evidence with a fixed-effect model indicated that patients with CAA-ICH and cSS were more susceptible to relapse (OR = 2.04; 95% CI: 1.46–2.83; P < 0.001; I2 = 0%; P = 0.94) (Figure 5A). Six studies investigated the association between disseminated cSS and CAA-ICH recurrence separately. The pooled evidence indicated that disseminated cSS was highly correlated with the recurrence of CAA-ICH (OR = 3.21; 95% CI: 2.25–4.58; P < 0.001; I2 = 16%; P = 0.31) (Figure 5A). However, the pooled results of the three studies suggested that there was no significant relationship between focal cSS and CAA-ICH recurrence (OR = 1.46; 95% CI: 0.78–2.74; P = 0.24; I2 = 0%; P = 0.98) (Figure 5A). As no significant heterogeneity was found, a fixed-effects model was used in both cases.
Three studies analyzed the relationship between WMH volume and CAA-ICH recurrence, and pooled results with a fixed-effect model showed no significant association between them (OR = 1.00; 95% CI: 1.00–1.01; P = 0.52; I2 = 0%; P = 0.45) (Figure 5A).
Four studies analyzed the association between CSO-PVS and CAA-ICH recurrence. The pooled evidence of four studies with a fixed-effect model suggested that the risk of recurrence of CAA-ICH was positively associated with the severity of CSO-PVS (OR = 1.67; 95% CI: 1.14–2.45; P = 0.009; I2 = 0%; P = 0.45) (Figure 5A).
The association between the total MRI burden of SVD and CAA-ICH recurrence has been reported in two studies. Our pooled results of two studies indicated that there was no significant relationship between the total MRI burden of SVD and the risk of CAA-ICH recurrence (OR = 1.62; 95% CI: 0.90–2.90; P = 0.11; I2 = 77.1%; P = 0.04) (Figure 5B). A random-effects model was used because significant heterogeneity was detected between the studies.
3.4. Sensitivity analysis and publication bias
In sensitivity analysis, no individual study significantly influenced the pooled CAA-ICH recurrence rate (Figure 6). The funnel plots visually assess publication bias and the horizontal line represents the summary effect estimates. The funnel plots and Egger's tests of relapse risk factors, except for previous ICH (P = 0.008) (Figure 7F), showed no statistically significant publication bias for other risk factors, including baseline ICH volume (P = 0.364) (Figure 7A), cSAH presence (P = 0.224) (Figure 7B), CSO-PVS (P = 0.798) (Figure 7C), cSS presence (P = 0.343) (Figure 7D) and disseminated cSS (P = 0.333) (Figure 7E).
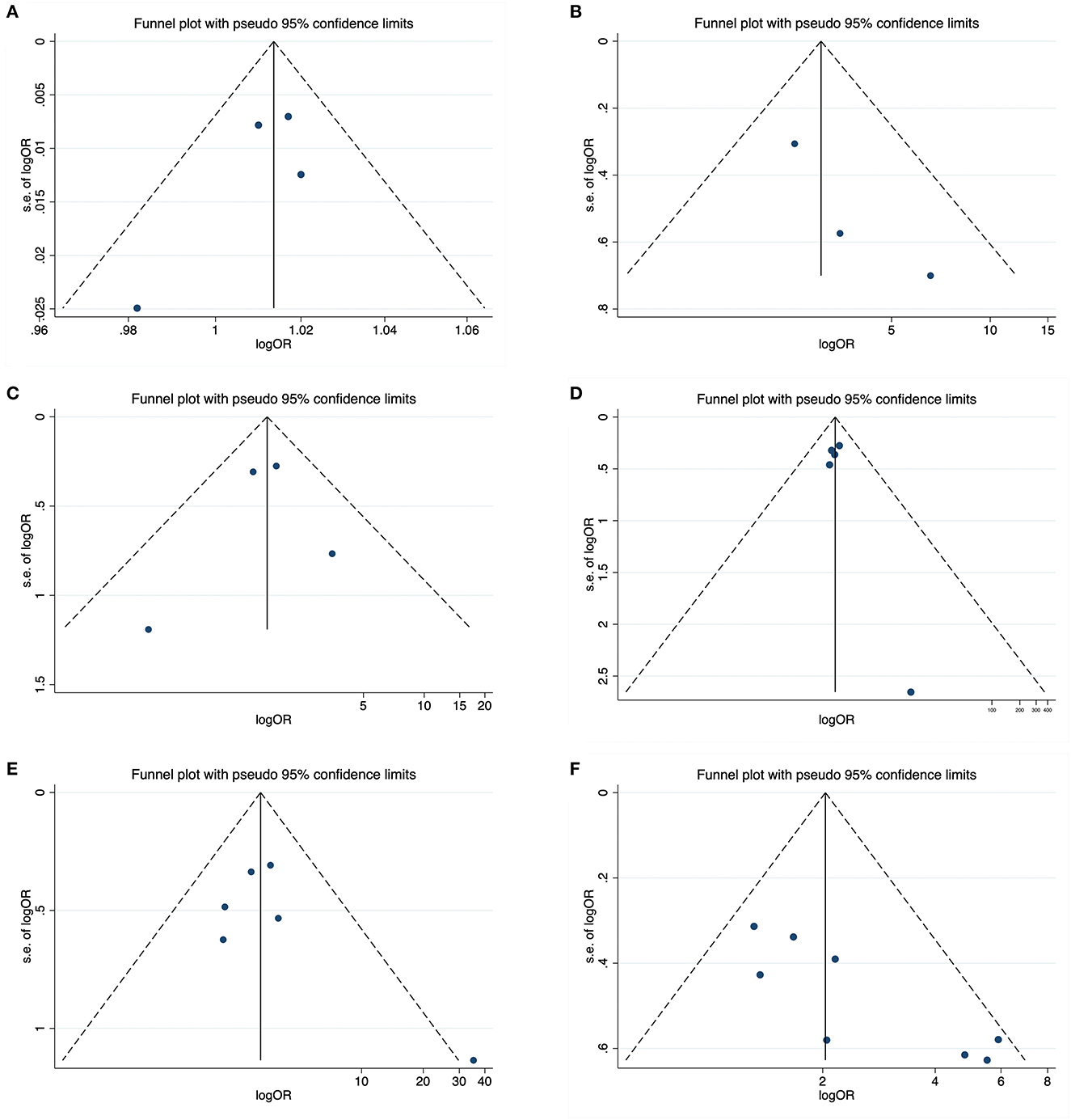
Figure 7. Funnel plots about potential publication bias for six recurrence risk factors including baseline ICH volume (A), cSAH presence (B), CSO-PVS (C), cSS presence (D), disseminated cSS (E), and previous ICH (F).
3.5. Rating the quality of evidence
According to the GRADE scores, the strength of evidence for the six risk factors mentioned above ranged between very low and low. All studies reported outcome indicators directly. The reason for the downgrade is shown in Figure 8.
4. Discussion
Our study confirmed a high recurrence rate of CAA-ICH. However, the significant heterogeneity among the included studies may have limited the reliability of the findings. Vascular risk factors, region and follow-up duration were not sources of heterogeneity. Furthermore, our study found that the risk factors associated with the recurrence of CAA-ICH included previous ICH, baseline ICH volume, presence of cSAH, cSS (especially if disseminated), and the severity of CSO-PVS (P < 0.05). However, the associations between age, sex, hypertension, diabetes, dyslipidemia, anticoagulation or antiplatelet therapy, presence of IVH, lobar CMBs, WMH volume, total MRI burden of SVD (P>0.05), and CAA-ICH recurrence require further investigation.
Our study showed that cSAH on CT, and cSS (especially if disseminated) and CSO-PVS on MRI are significant neuroimaging markers associated with CAA-ICH recurrence. They can serve as baseline predictors of ICH to identify patients at high risk for recurrence. The generation of cSS is attributed to the excessive deposition of amyloid-β, which leads to increased brittleness of the cortical or pia meningeal blood vessels (52). This brittleness can cause vessel rupture, resulting in chronic bleeding, and the deposition of hemosiderin and other blood decomposition substances in the superficial cortical surface and subarachnoid space (53). The incidence of cSS is high in patients with CAA-ICH but not in those with other types of ICH (54). According to the modified Boston criteria, the cSS is an important, clinically relevant imaging marker for the diagnosis of CAA (55). Some studies have found that the site of subsequent substantial cerebral hemorrhage in patients with CAA is often the same as the site of the initial cSS (56). A previous meta-analysis has also confirmed that cSS strongly predicts increased risk of recurrent lobar hemorrhage (35). Our study also showed that patients with more severe cSS (disseminated cSS: affecting three brain sulci and more) had a higher risk of future independent bleeding.
Similar to cSS, the mechanism by which cSAH can predict the recurrence of CAA-ICH may be related to the increased brittleness of blood vessels caused by the high deposition of amyloid proteins in the pia, leading to rupture. cSS and cSAH are likely two different imaging markers in a single pathological process (57). Focal convex linear SAH (acute cSAH) marks acute bleeding events and chronic cerebral sulci blood degradation products (cSS) mark previous episodes of cSAH (58). Previous studies have confirmed that the presence of MRI-detected cSHA is closely associated with the recurrence of CAA-ICH (54, 59). Specifically, adjacent cSAH is often associated with acute lobar ICH, which may extend from or develop directly from lobar ICH (60). This indicates that the area near the hematoma is more susceptible compared to distant areas. Sometimes, cSAH is clinically characterized by mild or asymptomatic symptoms that are easy to miss; however, its frequent onset eventually leads to symptomatic ICH (57). Owing to the limited number of available studies, in our study, cSAH was based on CT scans. CT scan is widely used in most medical institutions for the evaluation of acute ICH. Therefore, the discovery of CT-based cSAH has a greater advantage in determining early ICH recurrence.
The PVS is defined as a fluid-filled space that acts as a pre-lymphatic system for removing substances (40, 61). Owing to aging, the deterioration of vascular conditions, and other factors, the deposited amyloid-β obstructs the function of the original cleaning substance of the PVS, causing the expansion of the PVS to further hinder the drainage of soluble amyloid-β, leading to arterial load rupture and ICH (62). High CSO-PVS have been shown to be closely related to cSS and are highly prevalent in sporadic CAA (63). They hold as much potential as cSS for both diagnosing CAA and predicting ICH recurrence.
In our study, the two other MRI-based SVD markers, lobar CMBs and WMH volume, were not significantly associated with CAA-ICH recurrence. A previous meta-analysis showed that the presence and number of lobar CMBs were associated with a high risk of ICH recurrence, especially when more than 10 CMBs were counted (64). Our pooled results showed that neither the presence of lobar CMBs >5 nor the number of lobar CMBs was significantly associated with the recurrence of CAA-ICH. This may be due to the fact that a smaller number of CMBs implies milder cerebral microangiopathy and is less predictive of rebleeding compared with lobar CMBs >10. In addition, the included studies contained different group-level data, which may have affected our pooled results. However, it has also been suggested that specific disease phenotypes (e.g., more bleeding or active disease selected by cSS) are more valuable in predicting CAA-ICH recurrence than the overall disease burden (lobar CMBs count and other SVD markers) (53), which is largely consistent with the results of our meta-analysis. The difficulty of detecting WMH by conventional MRI may explain the lack of correlation between WMH and ICH recurrence. Therefore, the predictive value of CMB and WMH for CAA-ICH should be studied using larger and more comprehensive imaging datasets. In the CT-based imaging findings, except for cSAH, the recurrence of CAA-ICH correlated with the baseline ICH volume, which reflects the severity of the index ICH; however, there was no significant correlation with the presence or absence of IVH.
The use of antithrombotic drugs in CAA-ICH survivors remains controversial. Previous studies have shown that avoiding anticoagulant use is a safer option for patients with CAA prone to recurrent ICH (65, 66). They suggested that patients with both non-valvular atrial fibrillation and CAA should consider alternative therapies (such as left atrial appendage occlusion) after bleeding or replace warfarin with a relatively safe vitamin K antagonist (67). Antiplatelet agents used in most ischemic strokes and transient ischemic attacks appear to have a better safety profile than anticoagulants (68). Although antiplatelet drugs do not directly increase the risk of bleeding, they are associated with an increased prevalence of lobar microbleeds, which further increases the risk of developing symptomatic ICH (69). Our meta-analysis showed that neither anticoagulant nor antiplatelet therapy was significantly associated with CAA-ICH recurrence, a conclusion consistent with an existing study (70). Ischemic lesions are common in patients with CAA (71). For survivors of CAA-ICH, the timing of discontinuation or re-initiation of antithrombotic therapy depends on whether the risk of bleeding or ischemia is higher. The decision made by the clinician after fully weighing the benefits and risks is particularly critical and of great significance for patients who require the continuous use of antithrombotic drugs. The safety of antithrombotic therapy in patients with CAA-ICH should be determined by studying a larger established population.
Patients with a history of hemorrhagic stroke are at a higher risk of rebleeding. However, there was no significant correlation between the history of other diseases and CAA-ICH recurrence. A previous study suggested that hypertension may be the only significant clinical factor affecting the recurrence of CAA-ICH (48, 72). However, our statistical results showed that a history of hypertension was not associated with the recurrence of CAA-ICH. Although patients with CAA-ICH may show clinical or pathological evidence of hypertension, hypertension-associated ICH primarily presents as arteriolar sclerosis and fibrinoid necrosis, which differ significantly from the characteristics and mechanism of CAA-ICH (73, 74). Therefore, hypertension may affect the prognosis of patients with CAA-ICH in other ways, which requires further investigation. However, there is currently no better treatment for CAA-ICH survivors. Therefore, strict blood pressure control is recommended.
This study has several strengths that contribute to its robustness and reliability. There are a limited number of studies specifically on CAA-ICH, and this study included as many studies as possible related to CAA-ICH recurrence. The study populations were from different geographic regions, and the studies spanned a long period of time. We screened for several risk factors for clinical and imaging manifestations that are strongly associated with ICH recurrence, which can be very helpful for clinicians in making decisions about disease assessment and medication. In addition, the study used a standardized study scoring tool and adhered to the relevant guidelines for systematic reviews and meta-analyses, which ensured the rigor and validity of the study.
Our study had several limitations. First, the number of included studies and the small sample size may have limited the reliability of our results for certain risk factors. Second, considering the limited literature available, we broadened the search scope to include all prospective or retrospective studies involving the CAA-ICH recurrence rate or any recurrence factors. The duration of follow-up varied widely among the included studies. These limitations prevent us from extending our conclusions to other populations. Therefore, large-scale, prospective, high-quality studies in different countries are necessary to confirm our findings.
In conclusion, our study confirmed a high recurrence rate of CAA-ICH. cSAH on CT, and cSS (especially if disseminated) and CSO-PVS on MRI were neuroimaging markers significantly associated with CAA-ICH recurrence. The onset of CAA-ICH usually occurs several times, and recurrence is partly related to the index ICH volume. Therefore, it should be highly valued and prevented after the initial bleeding event. Screening for effective baseline predictors can help develop rational prevention strategies to reduce the occurrence of rebleeding events and improve the prognosis of patients with CAA-ICH, which is currently the best option in the absence of specific treatment.
Data availability statement
The original contributions presented in the study are included in the article/Supplementary material, further inquiries can be directed to the corresponding authors.
Author contributions
XJ: Formal analysis, Writing—original draft, Software. MB: Formal analysis, Writing—original draft, Software. HZ: Data curation, Writing—review & editing. JX: Writing—review & editing, Supervision. LP: Methodology, Funding acquisition, Writing—review & editing. ZL: Funding acquisition, Methodology, Resources, Writing—review & editing.
Funding
The author (s) declare financial support was received for the research, authorship, and/or publication of this article. This work was supported by grants from the National Natural Science Foundation of China (Grant Nos. 81973803 and 82274431), Shanghai Healthcare Research Special Project (Grant No. 202240039), and the Science and Technology Innovation Cultivation Program of Longhua Hospital Affiliated to Shanghai University of Traditional Chinese Medicine (Grant Nos. YD202210 and YD202219).
Acknowledgments
We extend our gratitude to all the authors of the included studies.
Conflict of interest
The authors declare that the research was conducted in the absence of any commercial or financial relationships that could be construed as a potential conflict of interest.
Publisher's note
All claims expressed in this article are solely those of the authors and do not necessarily represent those of their affiliated organizations, or those of the publisher, the editors and the reviewers. Any product that may be evaluated in this article, or claim that may be made by its manufacturer, is not guaranteed or endorsed by the publisher.
Supplementary material
The Supplementary Material for this article can be found online at: https://www.frontiersin.org/articles/10.3389/fneur.2023.1265693/full#supplementary-material
References
1. Viswanathan A, Greenberg SM. Cerebral amyloid angiopathy in the elderly. Ann Neurol. (2011) 70:871–80. doi: 10.1002/ana.22516
2. Vinters HV. Cerebral amyloid angiopathy. A critical review. Stroke. (1987) 18:311–24. doi: 10.1161/01.STR.18.2.311
3. Jellinger KA. Alzheimer disease and cerebrovascular pathology: an update. J Neural Transm. (2002) 109:813–36. doi: 10.1007/s007020200068
4. Broderick J, Connolly S, Feldmann E, Hanley D, Kase C, Krieger D, et al. Guidelines for the management of spontaneous intracerebral hemorrhage in adults: 2007 update: a guideline from the American Heart Association/American Stroke Association Stroke Council, high blood pressure research council, and the quality of care and outcomes in research interdisciplinary working group. Stroke. (2007) 38:2001–23. doi: 10.1161/STROKEAHA.107.183689
5. Ikram MA, Wieberdink RG, Koudstaal PJ. International epidemiology of intracerebral hemorrhage. Curr Atheroscler Rep. (2012) 14:300–6. doi: 10.1007/s11883-012-0252-1
6. Charidimou A, Gang Q, Werring DJ. Sporadic cerebral amyloid angiopathy revisited: recent insights into pathophysiology and clinical spectrum. J Neurol Neurosurg Psychiatry. (2012) 83:124–37. doi: 10.1136/jnnp-2011-301308
7. Passero S, Burgalassi L, D'Andrea P, Battistini N. Recurrence of bleeding in patients with primary intracerebral hemorrhage. Stroke. (1995) 26:1189–92. doi: 10.1161/01.STR.26.7.1189
8. Leblanc R, Preul M, Robitaille Y, Villemure JG, Pokrupa R. Surgical considerations in cerebral amyloid angiopathy. Neurosurgery. (1991) 29:712–8. doi: 10.1227/00006123-199111000-00012
9. Ishii N, Nishihara Y, Horie A. Amyloid angiopathy and lobar cerebral haemorrhage. J Neurol Neurosurg Psychiatry. (1984) 47:1203–10. doi: 10.1136/jnnp.47.11.1203
10. Mehndiratta P, Manjila S, Ostergard T, Eisele S, Cohen ML, Sila C, et al. Cerebral amyloid angiopathy-associated intracerebral hemorrhage: pathology and management. Neurosurg Focus. (2012) 32:E7. doi: 10.3171/2012.1.FOCUS11370
11. Izumihara A, Ishihara T, Iwamoto N, Yamashita K, Ito H. Postoperative outcome of 37 patients with lobar intracerebral hemorrhage related to cerebral amyloid angiopathy. Stroke. (1999) 30:29–33. doi: 10.1161/01.STR.30.1.29
12. Zhang Y, Wang X, Schultz C, Lanzino G, Rabinstein AA. Postoperative outcome of cerebral amyloid angiopathy-related lobar intracerebral hemorrhage: case series and systematic review. Neurosurgery. (2012) 70:125–30. doi: 10.1227/NEU.0b013e31822ea02a
13. Weber SA, Patel RK, Lutsep HL. Cerebral amyloid angiopathy: diagnosis and potential therapies. Expert Rev Neurother. (2018) 18:503–13. doi: 10.1080/14737175.2018.1480938
14. Charidimou A, Boulouis G, Greenberg SM, Viswanathan A. Cortical superficial siderosis and bleeding risk in cerebral amyloid angiopathy: a meta-analysis. Neurology. (2019) 93:e2192–e202. doi: 10.1212/WNL.0000000000008590
15. Malhotra K, Theodorou A, Katsanos AH, Zompola C, Shoamanesh A, Boviatsis E, et al. Prevalence of clinical and neuroimaging markers in cerebral amyloid angiopathy: a systematic review and meta-analysis. Stroke. (2022) 53:1944–53. doi: 10.1161/STROKEAHA.121.035836
16. Xu M, Zhu Y, Song X, Zhong X, Yu X, Wang D, et al. Pathological changes of small vessel disease in intracerebral hemorrhage: a systematic review and meta-analysis. Transl Stroke Res. (2023). doi: 10.1007/s12975-023-01154-4
17. Charidimou A, Boulouis G, Gurol ME, Ayata C, Bacskai BJ, Frosch MP, et al. Emerging concepts in sporadic cerebral amyloid angiopathy. Brain. (2017) 140:1829–50. doi: 10.1093/brain/awx047
18. Boulouis G, Charidimou A, Greenberg SM. Sporadic cerebral amyloid angiopathy: pathophysiology, neuroimaging features, and clinical implications. Semin Neurol. (2016) 36:233–43. doi: 10.1055/s-0036-1581993
19. Charidimou A, Martinez-Ramirez S, Reijmer YD, Oliveira-Filho J, Lauer A, Roongpiboonsopit D, et al. Total magnetic resonance imaging burden of small vessel disease in cerebral amyloid angiopathy: an imaging-pathologic study of concept validation. JAMA Neurol. (2016) 73:994–1001. doi: 10.1001/jamaneurol.2016.0832
20. Liberati A, Altman DG, Tetzlaff J, Mulrow C, Gøtzsche PC, Ioannidis JP, et al. The prisma statement for reporting systematic reviews and meta-analyses of studies that evaluate healthcare interventions: explanation and elaboration. BMJ. (2009) 339:b2700. doi: 10.1136/bmj.b2700
21. Yanagawa T, Sato H, Suzuki K, Ooigawa H, Takao M, Kurita H. Association of antithrombotic therapy with postoperative rebleeding in patients with cerebral amyloid angiopathy. Chin Neurosurg J. (2023) 9:12. doi: 10.1186/s41016-023-00324-5
22. Koemans EA, Castello JP, Rasing I, Abramson JR, Voigt S, Perosa V, et al. Sex differences in onset and progression of cerebral amyloid angiopathy. Stroke. (2023) 54:306–14. doi: 10.1161/STROKEAHA.122.040823
23. Yang Q, Zeng X, Yu Z, Liu X, Tang L, Zhang G, et al. Ct-visible convexity subarachnoid hemorrhage predicts early recurrence of lobar hemorrhage. Front Neurol. (2022) 13:843851. doi: 10.3389/fneur.2022.843851
24. Goeldlin MB, Mueller A, Siepen BM, Mueller M, Strambo D, Michel P, et al. Etiology, 3-month functional outcome and recurrent events in non-traumatic intracerebral hemorrhage. J Stroke. (2022) 24:266–77. doi: 10.5853/jos.2021.01823
25. Garg A, Ortega-Gutierrez S, Farooqui M, Nagaraja N. Recurrent intracerebral hemorrhage in patients with cerebral amyloid angiopathy: a propensity-matched case-control study. J Neurol. (2022) 269:2200–5. doi: 10.1007/s00415-021-10937-4
26. Che R, Zhang M, Sun H, Ma J, Hu W, Liu X, et al. Long-term outcome of cerebral amyloid angiopathy-related hemorrhage. CNS Neurosci Ther. (2022) 28:1829–37. doi: 10.1111/cns.13922
27. Xu T, Feng Y, Wu W, Shen F, Ma X, Deng W, et al. The predictive values of different small vessel disease scores on clinical outcomes in mild ich patients. J Atheroscler Thromb. (2021) 28:997–1008. doi: 10.5551/jat.61267
28. Xia M, Su Y, Gao F, Shen Y, Dong Q, Cheng X. The use of serum matrix metalloproteinases in cerebral amyloid angiopathy-related intracerebral hemorrhage and cognitive impairment. Eur Stroke J. (2021) 6(Suppl. 1):444. doi: 10.1177/23969873211034932
29. Cheng X, Su Y, Wang Q, Gao F, Ye X, Wang Y, et al. Neurofilament light chain predicts risk of recurrence in cerebral amyloid angiopathy-related intracerebral hemorrhage. Aging-Us. (2020) 12:23727–38. doi: 10.18632/aging.103927
30. Tsai HH, Chen SJ, Tsai LK, Pasi M, Lo YL, Chen YF, et al. Long-term vascular outcomes in patients with mixed location intracerebral hemorrhage and microbleeds. Neurology. (2021) 96:e995–e1004. doi: 10.1212/WNL.0000000000011378
31. Pinho J, Araujo JM, Costa AS, Silva F, Francisco A, Quintas-Neves M, et al. Intracerebral hemorrhage recurrence in patients with and without cerebral amyloid angiopathy. Cerebrovasc Dis Extra. (2021) 11:15–21. doi: 10.1159/000513503
32. Li Q, Zanon Zotin MC, Warren AD, Ma Y, Gurol E, Goldstein JN, et al. Ct-visible convexity subarachnoid hemorrhage is associated with cortical superficial siderosis and predicts recurrent ich. Neurology. (2021) 96:e986–e94. doi: 10.1212/WNL.0000000000011052
33. Raposo N, Charidimou A, Roongpiboonsopit D, Onyekaba M, Gurol ME, Rosand J, et al. Convexity subarachnoid hemorrhage in lobar intracerebral hemorrhage: a prognostic marker. Neurology. (2020) 94:e968–e77. doi: 10.1212/WNL.0000000000009036
34. Pasi M, Charidimou A, Boulouis G, Auriel E, Ayres A, Schwab KM, et al. Mixed-location cerebral hemorrhage/microbleeds: underlying microangiopathy and recurrence risk. Neurology. (2018) 90:e119–e26. doi: 10.1212/WNL.0000000000004797
35. Charidimou A, Boulouis G, Roongpiboonsopit D, Xiong L, Pasi M, Schwab KM, et al. Cortical superficial siderosis and recurrent intracerebral hemorrhage risk in cerebral amyloid angiopathy: large prospective cohort and preliminary meta-analysis. Int J Stroke. (2019) 14:723–33. doi: 10.1177/1747493019830065
36. Charidimou A, Boulouis G, Roongpiboonsopit D, Auriel E, Pasi M, Haley K, et al. Cortical superficial siderosis multifocality in cerebral amyloid angiopathy: a prospective study. Neurology. (2017) 89:2128–35. doi: 10.1212/WNL.0000000000004665
37. Xia L, Min W, Lu X, Wang C, Jiang Z, Zhang Y, et al. Subdural hemorrhage from cerebral amyloid angiopathy-related intracerebral hemorrhage: a risk factor for postoperative hemorrhage. World Neurosurg. (2017) 107:103–14. doi: 10.1016/j.wneu.2017.07.099
38. Boulouis G, Charidimou A, Pasi M, Roongpiboonsopit D, Xiong L, Auriel E, et al. Hemorrhage recurrence risk factors in cerebral amyloid angiopathy: comparative analysis of the overall small vessel disease severity score versus individual neuroimaging markers. J Neurol Sci. (2017) 380:64–7. doi: 10.1016/j.jns.2017.07.015
39. Roongpiboonsopit D, Charidimou A, William CM, Lauer A, Falcone GJ, Martinez-Ramirez S, et al. Cortical superficial siderosis predicts early recurrent lobar hemorrhage. Neurology. (2016) 87:1863–70. doi: 10.1212/WNL.0000000000003281
40. Koo HW, Jo KI, Yeon JY, Kim JS, Hong SC. Clinical features of high-degree centrum semiovale-perivascular spaces in cerebral amyloid angiopathy. J Neurol Sci. (2016) 367:89–94. doi: 10.1016/j.jns.2016.05.040
41. Yeh S-J, Tang S-C, Tsai L-K, Jeng J-S. Pathogenetical subtypes of recurrent intracerebral hemorrhage designations by Smash-U Classification System. Stroke. (2014) 45:2636. doi: 10.1161/STROKEAHA.114.005598
42. van Etten ES, Auriel E, Haley KE, Ayres AM, Vashkevich A, Schwab KM, et al. Incidence of symptomatic hemorrhage in patients with lobar microbleeds. Stroke. (2014) 45:2280–5. doi: 10.1161/STROKEAHA.114.005151
43. Charidimou A, Peeters AP, Jäger R, Fox Z, Vandermeeren Y, Laloux P, et al. Cortical superficial siderosis and intracerebral hemorrhage risk in cerebral amyloid angiopathy. Neurology. (2013) 81:1666–73. doi: 10.1212/01.wnl.0000435298.80023.7a
44. Biffi A, Shulman JM, Jagiella JM, Cortellini L, Ayres AM, Schwab K, et al. Genetic variation at Cr1 increases risk of cerebral amyloid angiopathy. Neurology. (2012) 78:334–41. doi: 10.1212/WNL.0b013e3182452b40
45. Domingues-Montanari S, Hernandez-Guillamon M, Fernandez-Cadenas I, Mendioroz M, Boada M, Munuera J, et al. Ace variants and risk of intracerebral hemorrhage recurrence in amyloid angiopathy. Neurobiol Aging. (2011) 32:551.e13–22. doi: 10.1016/j.neurobiolaging.2010.01.019
46. Biffi A, Halpin A, Towfighi A, Gilson A, Busl K, Rost N, et al. Aspirin and recurrent intracerebral hemorrhage in cerebral amyloid angiopathy. Neurology. (2010) 75:693–8. doi: 10.1212/WNL.0b013e3181eee40f
47. Petridis AK, Barth H, Buhl R, Hugo HH, Mehdorn HM. Outcome of cerebral amyloid angiopathic brain haemorrhage. Acta Neurochir. (2008) 150:889–95. doi: 10.1007/s00701-008-0001-y
48. Izumihara A, Suzuki M, Ishihara T. Recurrence and extension of lobar hemorrhage related to cerebral amyloid angiopathy: multivariate analysis of clinical risk factors. Surg Neurol. (2005) 64:160–4. doi: 10.1016/j.surneu.2004.09.010
49. Greenberg SM, Eng JA, Ning MM, Smith EE, Rosand J. Hemorrhage burden predicts recurrent intracerebral hemorrhage after lobar hemorrhage. Stroke. (2004) 35:1415–20. doi: 10.1161/01.STR.0000126807.69758.0e
50. O'Donnell HC, Rosand J, Knudsen KA, Furie KL, Segal AZ, Chiu RI, et al. Apolipoprotein E genotype and the risk of recurrent lobar intracerebral hemorrhage. N Engl J Med. (2000) 342:240–5. doi: 10.1056/NEJM200001273420403
51. Guyatt GH, Oxman AD, Vist GE, Kunz R, Falck-Ytter Y, Alonso-Coello P, et al. Grade: an emerging consensus on rating quality of evidence and strength of recommendations. BMJ. (2008) 336:924–6. doi: 10.1136/bmj.39489.470347.AD
52. Linn J, Herms J, Dichgans M, Brückmann H, Fesl G, Freilinger T, et al. Subarachnoid hemosiderosis and superficial cortical hemosiderosis in cerebral amyloid angiopathy. AJNR Am J Neuroradiol. (2008) 29:184–6. doi: 10.3174/ajnr.A0783
53. Charidimou A, Linn J, Vernooij MW, Opherk C, Akoudad S, Baron JC, et al. Cortical superficial siderosis: detection and clinical significance in cerebral amyloid angiopathy and related conditions. Brain. (2015) 138:2126–39. doi: 10.1093/brain/awv162
54. Linn J, Halpin A, Demaerel P, Ruhland J, Giese AD, Dichgans M, et al. Prevalence of superficial siderosis in patients with cerebral amyloid angiopathy. Neurology. (2010) 74:1346–50. doi: 10.1212/WNL.0b013e3181dad605
55. Greenberg SM, Charidimou A. Diagnosis of cerebral amyloid angiopathy: evolution of the boston criteria. Stroke. (2018) 49:491–7. doi: 10.1161/STROKEAHA.117.016990
56. Linn J, Wollenweber FA, Lummel N, Bochmann K, Pfefferkorn T, Gschwendtner A, et al. Superficial siderosis is a warning sign for future intracranial hemorrhage. J Neurol. (2013) 260:176–81. doi: 10.1007/s00415-012-6610-7
57. Beitzke M, Enzinger C, Wünsch G, Asslaber M, Gattringer T, Fazekas F. Contribution of convexal subarachnoid hemorrhage to disease progression in cerebral amyloid angiopathy. Stroke. (2015) 46:1533–40. doi: 10.1161/STROKEAHA.115.008778
58. Ni J, Auriel E, Jindal J, Ayres A, Schwab KM, Martinez-Ramirez S, et al. The characteristics of superficial siderosis and convexity subarachnoid hemorrhage and clinical relevance in suspected cerebral amyloid angiopathy. Cerebrovasc Dis. (2015) 39:278–86. doi: 10.1159/000381223
59. Charidimou A, Jäger RH, Fox Z, Peeters A, Vandermeeren Y, Laloux P, et al. Prevalence and mechanisms of cortical superficial siderosis in cerebral amyloid angiopathy. Neurology. (2013) 81:626–32. doi: 10.1212/WNL.0b013e3182a08f2c
60. Smith EE, Maas MB. To predict recurrence in cerebral amyloid angiopathy, look to the subarachnoid space. Neurology. (2020) 94:375–6. doi: 10.1212/WNL.0000000000009029
61. Marín-Padilla M, Knopman DS. Developmental aspects of the intracerebral microvasculature and perivascular spaces: insights into brain response to late-life diseases. J Neuropathol Exp Neurol. (2011) 70:1060–9. doi: 10.1097/NEN.0b013e31823ac627
62. Weller RO, Subash M, Preston SD, Mazanti I, Carare RO. Perivascular drainage of amyloid-beta peptides from the brain and its failure in cerebral amyloid angiopathy and Alzheimer's disease. Brain Pathol. (2008) 18:253–66. doi: 10.1111/j.1750-3639.2008.00133.x
63. Charidimou A, Jäger RH, Peeters A, Vandermeeren Y, Laloux P, Baron JC, et al. White matter perivascular spaces are related to cortical superficial siderosis in cerebral amyloid angiopathy. Stroke. (2014) 45:2930–5. doi: 10.1161/STROKEAHA.114.005568
64. Charidimou A, Imaizumi T, Moulin S, Biffi A, Samarasekera N, Yakushiji Y, et al. Brain hemorrhage recurrence, small vessel disease type, and cerebral microbleeds: a meta-analysis. Neurology. (2017) 89:820–9. doi: 10.1212/WNL.0000000000004259
65. Eckman MH, Rosand J, Knudsen KA, Singer DE, Greenberg SM. Can patients be anticoagulated after intracerebral hemorrhage? A decision analysis. Stroke. (2003) 34:1710–6. doi: 10.1161/01.STR.0000078311.18928.16
66. Wilson D, Werring DJ. Antithrombotic therapy in patients with cerebral microbleeds. Curr Opin Neurol. (2017) 30:38–47. doi: 10.1097/WCO.0000000000000411
67. Ruff CT, Giugliano RP, Braunwald E, Hoffman EB, Deenadayalu N, Ezekowitz MD, et al. Comparison of the efficacy and safety of new oral anticoagulants with warfarin in patients with atrial fibrillation: a meta-analysis of randomised trials. Lancet. (2014) 383:955–62. doi: 10.1016/S0140-6736(13)62343-0
68. He J, Whelton PK, Vu B, Klag MJ. Aspirin and risk of hemorrhagic stroke: a meta-analysis of randomized controlled trials. JAMA. (1998) 280:1930–5. doi: 10.1001/jama.280.22.1930
69. Vernooij MW, Haag MD, van der Lugt A, Hofman A, Krestin GP, Stricker BH, et al. Use of antithrombotic drugs and the presence of cerebral microbleeds: the rotterdam scan study. Arch Neurol. (2009) 66:714–20. doi: 10.1001/archneurol.2009.42
70. Viswanathan A, Rakich SM, Engel C, Snider R, Rosand J, Greenberg SM, et al. Antiplatelet use after intracerebral hemorrhage. Neurology. (2006) 66:206–9. doi: 10.1212/01.wnl.0000194267.09060.77
71. Reijmer YD, van Veluw SJ, Greenberg SM. Ischemic brain injury in cerebral amyloid angiopathy. J Cereb Blood Flow Metab. (2016) 36:40–54. doi: 10.1038/jcbfm.2015.88
72. Biffi A, Anderson CD, Battey TW, Ayres AM, Greenberg SM, Viswanathan A, et al. Association between blood pressure control and risk of recurrent intracerebral hemorrhage. JAMA. (2015) 314:904–12. doi: 10.1001/jama.2015.10082
73. Biessels GJ. Cerebral amyloid angiopathy: still a syndromal diagnosis. Stroke. (2020) 51:3487–8. doi: 10.1161/STROKEAHA.120.032703
Keywords: intracerebral hemorrhage, cerebral amyloid angiopathy, cerebral small vessel disease, cortical superficial siderosis, recurrence
Citation: Jia X, Bo M, Zhao H, Xu J, Pan L and Lu Z (2023) Risk factors for recurrent cerebral amyloid angiopathy-related intracerebral hemorrhage. Front. Neurol. 14:1265693. doi: 10.3389/fneur.2023.1265693
Received: 23 July 2023; Accepted: 23 October 2023;
Published: 07 November 2023.
Edited by:
Xin Cheng, Fudan University, ChinaCopyright © 2023 Jia, Bo, Zhao, Xu, Pan and Lu. This is an open-access article distributed under the terms of the Creative Commons Attribution License (CC BY). The use, distribution or reproduction in other forums is permitted, provided the original author(s) and the copyright owner(s) are credited and that the original publication in this journal is cited, in accordance with accepted academic practice. No use, distribution or reproduction is permitted which does not comply with these terms.
*Correspondence: Zhengyu Lu, lu.zhengyu@shutcm.edu.cn; Luqian Pan, panluqian@shutcm.edu.cn
†These authors have contributed equally to this work and share first authorship
‡These authors have contributed equally to this work