- 1Pécs Diagnostic Center, Pécs, Hungary
- 2The Hungarian Research Network-Pécsi Tudományegyetem, Clinical Neuroscience Magnetic Resonance Research Group, Pécs, Hungary
- 3Department of Neurosurgery, Medical School, University of Pécs, Pécs, Hungary
- 4Department of Neurology, Medical School, University of Pécs, Pécs, Hungary
- 5Department of Languages for Biomedical Purposes and Communication, University of Pécs, Pécs, Hungary
Background/aim: Migraine is a disabling headache with clinical and radiological complications. The aim of this study was to investigate the volume of the thalamus and hippocampus in migraineurs, the role of white matter lesions (WMLs), and the migraine characteristics in volume changes.
Methods: Brain MRIs of 161 right-handed female episodic migraine patients and 40 right-handed, age-related, healthy women were performed. Left and right thalamus segmentation was performed on the 3D MPRAGE images using the Freesurfer 5.3 image analysis suite. Hippocampal subfield segmentation was based on a novel statistical atlas built primarily upon ultra-high-resolution ex vivo MRI data.
Results: The left hippocampus had a smaller and the left thalamus had a larger total volume than the right one in both the control (p < 0.001) and migraine groups (p <0.001). Patients with white matter lesions (L+) showed smaller right thalamus and right hippocampal tail volumes than patients without lesions (L–) (p = 0.002 and p = 0.015, respectively) and controls (p = 0.039 and p = 0.025, respectively). For the right hippocampal body, we found significantly smaller volume in L+ patients when compared to L– patients (p = 0.018) and a similar trend when compared to the control group (p = 0.064). Patients without aura (A–) showed a larger right hippocampus (p = 0.029), right hippocampal body (p = 0.012), and tail volumes (p = 0.011) than patients with aura (A+). Inverse correlations were found between attack frequency and the volumes of the left and right hippocampal tails (p = 0.018 and p = 0.008, respectively).
Conclusion: These findings indicate that WMLs may influence the volume of the right thalamus and hippocampus, while migraine aura and attack frequency may lead to volume changes in different parts of the hippocampi in migraine patients. These data support the necessity of effective migraine management to limit subcortical volume loss in migraineurs.
Introduction
Migraine is a painful, returning headache disease that lasts for decades (1). Migraine is more prevalent in women than men due to hormonal differences starting in puberty (2–4). In addition, the migraine characteristics of men and women are different. Women report a longer attack duration, increased risk of headache recurrence, higher intensity of headaches, more frequent nausea, phonophobia, and photophobia, greater disability, and a longer time required for recovery (2–4).
Magnetic resonance imaging (MRI) is a useful diagnostic tool in migraine and shows migraine-related structural complications, e.g., hemispheric white matter lesions (WMLs) as well as cortical and subcortical volume changes of different structures (5, 6). The WMLs are clinically mute, mostly progressive microvascular tissue damages, which may influence the intrahemispheric size and volume impairment (5). Previously, we studied the possible influence of WMLs on hemispheric cortical thickness and volume in 161 right-handed female migraineurs, but statistically significant correlations were not found (6).
In healthy adults, the total brain volume was reported to be larger from birth in men than in women by ~11%. This size difference accounts for other reproducible findings: higher white/gray matter ratio, intra- vs. interhemispheric connectivity, and regional cortical and subcortical volumes in men. When structural and lateralization differences are present independent of size, sex/gender explains only ~1% of the total variance (7). Furthermore, there are several documented structural and functional brain differences between men and women, but it is hard to decide which of them is sex hormone-related (8).
The trigeminovascular system is a pain-transmission link between the vascular (dural and cortical) and neuronal (brainstem and thalamus) regions (9). The thalamus is a relay station; thereby, it has high (density) connectivity to many brain structures. It holds an important position in allodynia, central sensitization, and photophobia in migraine (10).
The hippocampus is involved in memory consolidation, spatial navigation, and pain-related stress response (11), as well as in pain processing, pain-related attention, and anxiety (12). Stronger hippocampal-cortico-limbic connectivity in migraineurs is associated with allodynia (11).
Based on the current literature, both structures are functionally impaired in migraine; thereby, we hypothesized that the hemispheric tissue damage (WMLs) and the migraine characteristics (disease duration, frequency, and aura) may negatively affect the volume of both the thalamus and hippocampus.
To test our hypothesis, we reinvestigated our abovementioned migraine headache patients' brain MRI studies (6) to measure the volume of the thalami and hippocampi and to evaluate the potential role of WMLs and the migraine characteristics in volume abnormalities.
Patients and methods
Subjects
A total of 161 women with episodic migraine (mean age 39.3 ± 12.5, range 18–73 years; disease duration 15.6 ± 11.9, range 1–57 years; attack frequency/month 5.6 ± 4.5, range 0.2–20.0; 63 with aura; 52 with white matter lesions; Table 1) were prospectively enrolled in this study. As controls, 40 age-matched healthy female subjects were included (mean age 38.3 ± 10.0, range 19–66 years). Migraineurs had no other types of headaches. None of the included migraine patients' headaches or auras were unilaterally side-locked in nature, but the headaches showed right hemisphere dominance in 68.3% (right-sided or bilateral with right > left pain intensity). An MRI was performed during a headache-free period for each patient; the minimum headache-free period was 1 day after the postdrome headache phase. All migraineurs and controls reported right-handedness. All subjects were strictly investigated for medical comorbidities (e.g., past and current medical history, physical examination, routine and autoimmune blood tests, cardiovascular monitoring, BMI, sleep disease, hypertension, gynecological disease, thyroid gland, and cognitive disease), and only those were enrolled in this study who lacked any medical diseases. Brain MRI studies of healthy participants did not show any structural abnormalities.
MRI acquisition
All subjects were scanned on the same 3T MRI scanner (Magnetom TIM Trio, Siemens AG, Erlangen, Germany) using a 12-channel head matrix coil. A whole-brain T1-weighted three-dimensional axial magnetization-prepared rapid gradient-echo (3D MPRAGE) sequence was acquired using the following parameters: TR/TI/TE = 1,900/900/3.4 ms, BW = 179 Hz/px, flip angle = 9°, FOV = 210 × 240 mm2, matrix size = 224 × 256, slice thickness = 0.94 mm, 176 axial slices, 0.94 × 0.94 × 0.94 mm3 isotropic voxels. Beyond the routine T1- and T2-weighted measurements, the scanning protocol also included fluid-attenuated inversion recovery (FLAIR) imaging (TR/TI/TE = 13,200/2,600/100 ms, BW = 401 Hz/px; echo trains = 14, FOV = 186 × 220 mm2, matrix size = 162 × 192, slice thickness = 1.5 mm, 100 axial slices).
MR image analysis
Left and right thalamus segmentation was performed on the high-resolution three-dimensional 3D MPRAGE images using the Freesurfer 5.3 image analysis suite (https://surfer.nmr.mgh.harvard.edu/fswiki). The technical details were described previously (13). Quality control was performed throughout the automatic processing stream. When the reconstruction was inaccurate, error correction was performed based on the recommended workflow (http://surfer.nmr.mgh.harvard.edu/fswiki/RecommendedReconstruction). The left and right hippocampus and their subfields (head, body, and tail) were segmented using the development version of FreeSurfer on 31 August 2017 (https://surfer.nmr.mgh.harvard.edu/fswiki/DownloadAndInstall). This new hippocampal subfield segmentation method is based on a novel statistical atlas built primarily upon ultra-high-resolution (~0.1 mm isotropic) ex vivo MRI data. This ex vivo atlas outperforms the in vivo counterpart that was distributed with Freesurfer 5.3 and yields subregion volumes that agree better with those from histological studies. The technical details of this new method were described earlier (14).
Analysis of white matter lesions
WMLs were considered if visible as hyperintensity on T2-weighted and FLAIR MRI but without a low-signal intensity lesion on T1-weighted MRI and larger than 3 mm, appearing in at least two consecutive slices (Figure 1). Supratentorial WMLs were delineated manually on the FLAIR images using the 3D Slicer software (http://www.slicer.org, Version 4.6.2, Figure 2). Total and lobar hemispheric WML numbers/volumes were calculated for all L+ subjects. The borders of the lobes were defined as previously described (6).
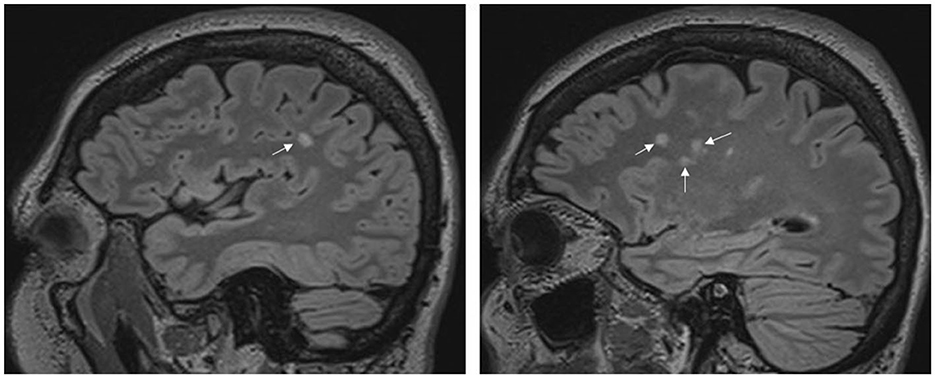
Figure 1. Migraine patient with intrahemispheric white matter lesions. The sagittal fluid-attenuated inversion recovery (FLAIR) images belong to one patient and show high signal intensities in the white matter. The arrows show the lesions.
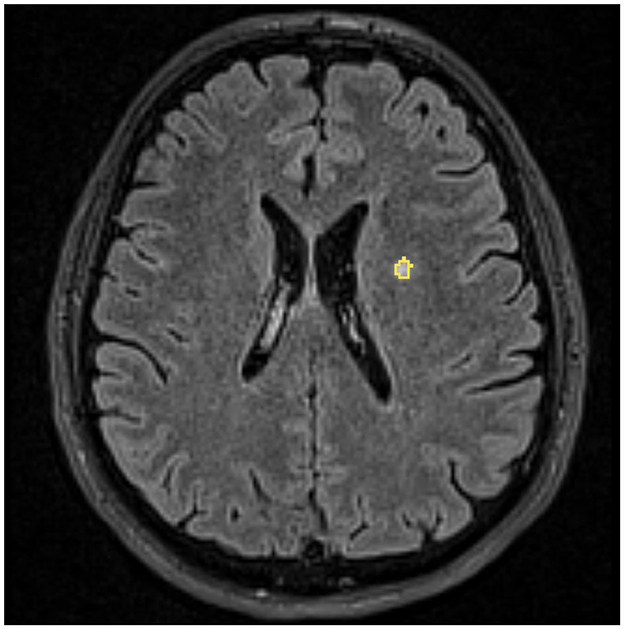
Figure 2. Delineation of a white matter lesion in a female migraineur. An example of an axial FLAIR image used to assess white matter lesion is shown in the background. Yellow indicates the outline of a manually delineated white matter lesion.
Statistical analysis
Statistical analyses were performed using the SPSS 20.0 software (IBM Corp., Armonk, NY, United States).
Differences in age between the whole migraine group and healthy controls were assessed using the Mann-Whitney U-test. Age differences among migraine subgroups (L+ and L–) and the healthy control group were assessed using the Kruskal–Wallis test followed by the Mann-Whitney U-test. The age difference between migraine patients without aura (A–) and those with aura (A+) was assessed using the Mann-Whitney U-test.
A Wilcoxon signed-rank test was used to identify whether there were statistically significant differences between the total volume of the left and right hippocampus, as well as between the left and right thalamus, separately in the healthy control group and the migraine group. Volume differences between the healthy control group and the migraine group in the left and right hippocampus, as well as the thalamus, were assessed by ANCOVA using age and total intracranial volume (ICV) as covariates.
Differences in continuous migraine-related variables (i.e., disease duration, migraine attack frequency) between migraine subgroups (L+ and L–) were compared using the Mann–Whitney U-test, while differences in the rate of aura (A+ or A–) between the same subgroups were assessed using Fisher's exact test. Within the migraine group with lesions (L+), a Wilcoxon signed-rank test was used to test for differences between left and right hemispheres in total and lobar hemispheric WML numbers/volumes. These differences were not tested for the temporal and occipital lobes because lesions were rare in these lobes, with a median lesion number of 0.
Differences in the thalamus, hippocampal, and hippocampal subfield volumes among the three groups (L+, L–, and control) were assessed using ANCOVA with age and ICV as covariates. In the case of significance, the least significant difference post-hoc analyses were conducted for pairwise comparison of the three groups.
Within the whole migraine group, the same volumes were compared between patients with aura (A+) and without aura (A–) using ANCOVA with age and ICV as covariates. The possible effects of other migraine characteristics (i.e., disease duration and migraine attack frequency) on the examined volumes were tested using stepwise multiple linear regression analyses. In these models, age, ICV, lesion, and aura group variables were also included as possible predictors.
Within the migraine group with lesions (L+), we assessed the potential relationships between left and right hemispheric brain structure volumes and the total and lobar lesion numbers/volumes in the corresponding hemisphere. Since the lesion number/volume data were not normally distributed (right-skewed with several extreme values), to perform powerful statistical analyses, the effects of lesion numbers/volumes were assessed by creating binarized subgroup variables based on the median split of them, e.g., the subgroup with a low number/volume (below or equal to the median) vs. the subgroup with a high number/volume of left hemispheric frontal lobe lesions. The effects of these subgroup variables on the examined volumes were tested using stepwise multiple linear regression analyses, including all these subgroup variables, age, and ICV as possible predictors. Since lesions were rare in the temporal and occipital lobes with a median lesion number of 0, the effects of lesion number/volume in these lobes were not assessed. Results were considered significant at p ≤ 0.05.
Results
There was no significant difference in age between the whole migraine group and the control group (p = 0.736). The Kruskal–Wallis test revealed significant age differences among the migraine subgroups (L+ and L–) and controls (p = 0.001). Post-hoc testing indicated that the L+ subgroup was significantly older than the other two groups (Table 1). There was no significant difference in age between patients without aura (A–) and with aura (A+) (p = 0.816, two-sided exact p-value).
The Wilcoxon signed-rank test showed that the left hippocampus had a smaller total volume when compared with the right hippocampus in both the healthy control group (3,353 ± 252 mm3 vs. 3,517 ± 289 mm3, Z = −4.968, p < 0.001) and the migraine group (3,379 ± 293 mm3 vs. 3,494 ± 317 mm3, Z = −8.653, p < 0.001). On the other hand, the left thalamus had a bigger volume when compared with the right thalamus in both the healthy control group (7,708 ± 747 mm3 vs. 6,984 ± 562 mm3; Z = −5.275, p < 0.001) and the migraine group (7,675 ± 817 mm3 vs. 6,951 ± 689 mm3, Z = −10.835, p < 0.001). There were no significant differences between the healthy control group and the whole migraine group in the total volume of the left hippocampus (p = 0.703), right hippocampus (p = 0.436; one outlier patient was excluded based on standardized residuals > 3), left thalamus (p = 0.801; one outlier patient was excluded based on standardized residuals < −3), and right thalamus (p = 0.726).
Disease duration and the rate of aura were significantly higher in L+ patients, while migraine attack frequency was not significantly different between the L+ and L– subgroups (Table 1). Results for the number and volumes of supratentorial white matter lesions in L+ patients in different hemispheric lobes and the total left and right hemispheres are presented in Table 2. The Wilcoxon signed-rank test indicated that there were no differences between the left and right hemispheres in total (p = 0.341), frontal (p = 0.084), and parietal (p = 0.264) WML numbers, and no differences were found for the total (p = 0.448) and frontal (p = 0.226) lesion volumes. However, the lesion volume was bigger in the left compared to the right-hemispheric parietal lobe (Z = −2.097, p = 0.036).
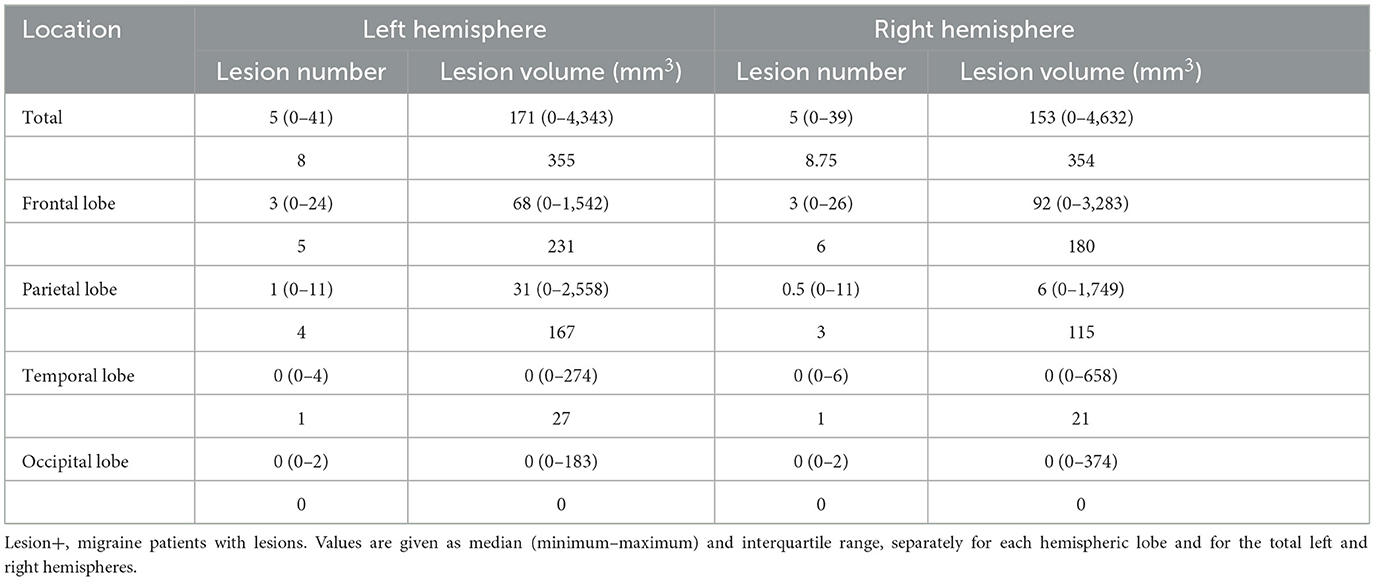
Table 2. Number and volumes of supratentorial white matter lesions in the Lesion+ migraine subgroup.
After correcting for the confounding effects of age and ICV, the patients with lesions (L+) showed significantly smaller right thalamus and right hippocampal tail volumes than patients without lesions (L–) and controls (Table 3). For the right hippocampal body, the ANCOVA showed a strong trend (p = 0.051); therefore, we performed the post-hoc tests, which indicated a significantly smaller volume in L+ patients when compared to L– patients and a trend when compared to the control group (Table 3).
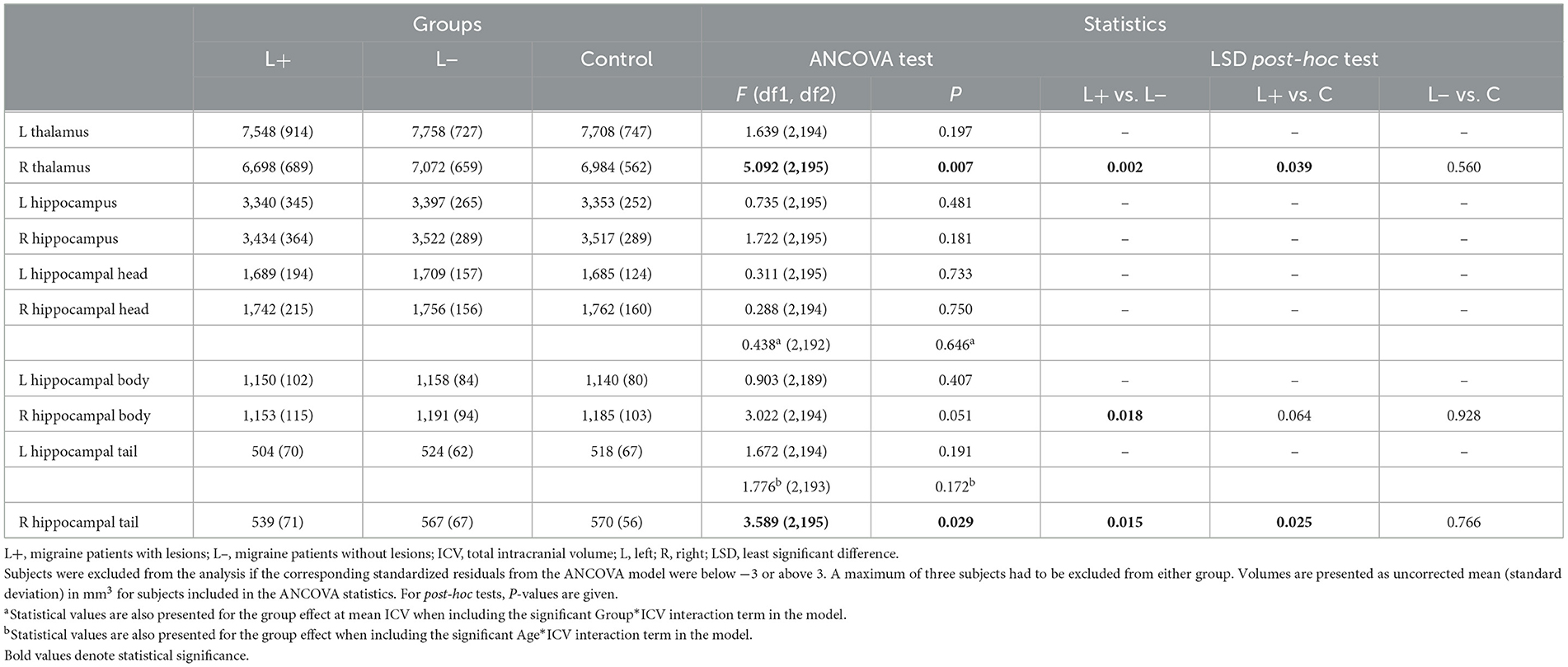
Table 3. Group differences in the volumes of examined structures among lesion-related migraine subgroups and controls.
Focusing on the migraine group, patients without aura (A–) showed a larger right hippocampus, right hippocampal body, and tail than patients with aura (A+) (Table 4).
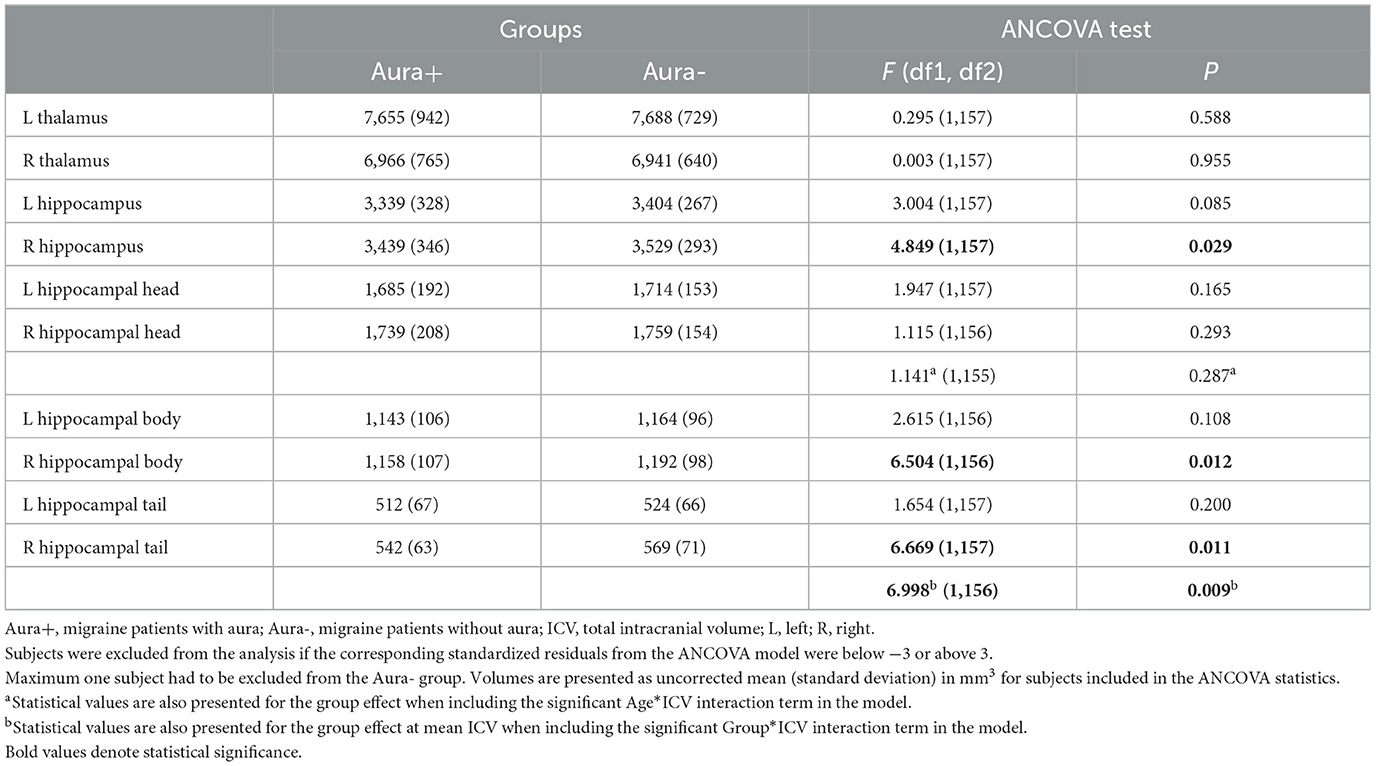
Table 4. Group differences in the volumes of examined structures between aura-related migraine subgroups.
Within the whole migraine group, neither the disease duration nor migraine attack frequency was selected by stepwise linear regression as significant predictors of the examined structural volumes, except for the volumes of hippocampal tails. Using multiple linear regression, including the volume of the left hippocampal tail as the dependent variable and migraine attack frequency, age, and ICV as independent variables, we found a significant negative correlation between attack frequency and the volume of the left hippocampal tail (p = 0.018, t = −2.385, Figure 3A). Based on our results reported in Tables 3, 4, for the right hippocampal tail, we also included the lesion (L– vs. L+) and aura (A– vs. A+) in addition to migraine attack frequency, age, and ICV as independent variables in the multiple linear regression model. We found a negative correlation of attack frequency with the right hippocampal tail volume (p = 0.008, t = −2.688, Figure 3B).
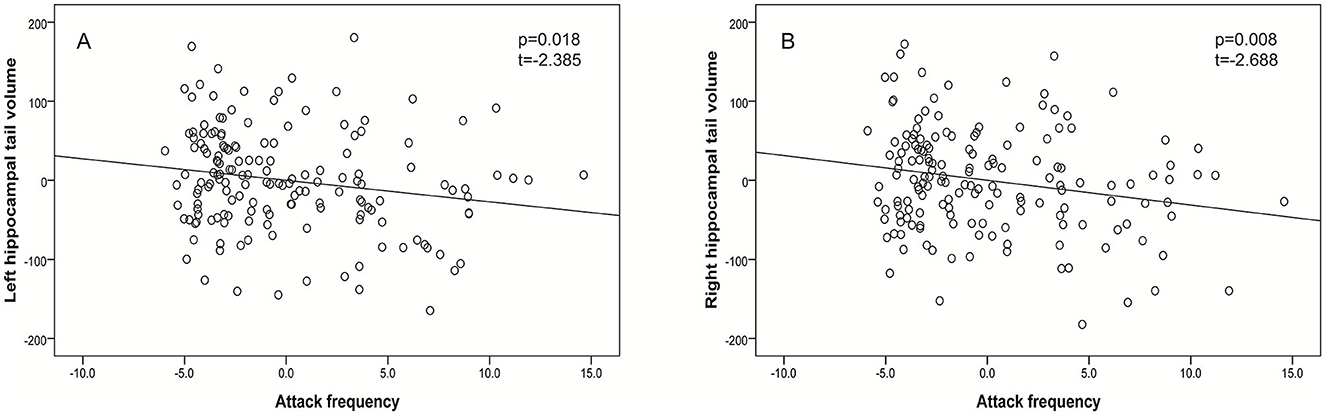
Figure 3. Partial regression plots demonstrating that more frequent migraine attacks were associated with decreased volumes of the left hippocampal tail (after controlling for the effects of age and ICV, A) and the right hippocampal tail (after controlling for the effects of age, ICV, lesion, and aura, B).
In the L+ migraine group, none of the binarized total or lobar hemispheric lesion number/volume variables were selected by stepwise linear regression as significant predictors of the examined structural volumes.
Discussion
In this rather homogenous patient population, we investigated 161 right-handed episodic migraineurs. To eliminate the confounding effect of sex-related differences in headache characteristics and brain size, only women were enrolled in the study. The total volumes of the left or right thalami and hippocampi were not different between migraineurs and controls. The volume of the right thalamus was smaller than the left one within the migraine and control groups. Patients with WMLs showed a smaller right thalamus than in lesion-negative migraineurs. The hippocampi were smaller on the left side in both groups. The hippocampus subgroup analysis of migraine patients and the subfield volume values of all three groups showed statistically significant differences. The white matter lesions, the presence of aura, and the attack frequency appeared to be risk factors for smaller volumes. It is also possible that the dominant unilateral headache side with more attacks on the right side has a role in the volume differences of the thalami. Since the patients' memory was only reliable for the last month, we did not use these data for statistical analysis.
Thalamic and hippocampal volume asymmetries in healthy subjects
In our right-handed healthy female control group, significant volume differences were detected between the thalami (R < L) and hippocampi (R > L) of the two hemispheric sides. Since in the cited morphometric studies (15–17), the participants were not separated by sex, and handedness was not documented in one study, these findings are just partly comparable with our data. However, handedness may influence the hippocampus volume on the same side.
Manual dominance in migraine
In a large retrospective study, the authors collected data from 546 patients with migraine aged between 16 and 65 years, reporting manual dominance according to the Edinburgh test. They included 466 right-handed and 80 left-handed subjects with migraine and registered 4,215 unilateral painful attacks. It was concluded that manual dominance influences the side of pain lateralization in migraine (18). Considering our 1-month-long database, the right-handed data are congruent.
The volume of the thalamus in migraine
In a study of 35 patients with migraine without aura and 40 healthy controls, the authors found thalamic nuclei with significantly increased and decreased volumes. The authors concluded that the result might contribute to the underlying pathogenesis of migraine (19).
In another study, significant volume reductions of three thalamic nuclei were observed in migraineurs: the central nuclear complex, the anterior nucleus, and the lateral dorsal nucleus (20). The thalamic nuclei with reduced volumes were connected to the limbic system.
In measurements of thalamic subregions in 77 migraineurs with episodic migraine and 30 controls, selective functional hypoconnectivity in the thalamic subregions was detected (21). The data provided neuroimaging evidence of thalamocortical pathway dysfunction in episodic migraines, including emotion and personality traits in migraineurs.
In a recent thalamic study, the volume was investigated by analyzing MRI data obtained from a large study, which specifically included women migraineurs with aura, unrelated migraine-free matched controls, and migraine aura-free co-twins. None of the analyses showed any between-group differences in the volume of the thalamus or of individual thalamic nuclei. These results indicated that the pathophysiology of migraine with aura did not involve alteration of thalamic volumes (22). According to our findings, thalamic volume abnormalities were not detected in migraine aura patients in the latter publication, but we found WML-associated smaller volume.
The volume of the hippocampus in migraine
In migraine patients, after a long follow-up for 2 years, the right hippocampus volume was positively associated with a good migraine outcome after adjustment of headache frequency (OR 4.72, p = 0.024) (23).
In a review of hippocampal volume, a longitudinal study discovered decreased volume in newly diagnosed migraine patients after 1 year (24). A cross-sectional study also suggested an adaptive increase in volume at low headache frequency and a maladaptive decrease in volume at higher headache frequency (25). These results were interpreted as either initial adaptive plasticity of the hippocampus or a larger hippocampus (i.e., a pre-existing condition) in migraineurs that then decreases with increased attack frequency (26). Evidence for the first interpretation has come from animal models of neurogenesis in the structure that is known to persist into adulthood. Stress (e.g., migraine) may mediate adaptive structural plasticity through the remodeling of dendrites and synapses (27). With repeated stress involved in migraine attacks, including pain, glucocorticoids, cortical spreading depression, and gonadal hormones, elevated and prolonged levels of excitatory amino acids are likely released (28, 29). Moreover, the aging rat hippocampus displays elevated and prolonged levels of excitatory amino acids released during acute stress. It is probable that structural plasticity in response to repeated stress starts as an adaptive and protective response but ends up as damage if the imbalance in the regulation of the key mediators is not resolved. It is likely that morphological rearrangements in the hippocampus impair memory functions, and it is conceivable that these may also have a role in chronic pain perception (30). In another study, the authors concluded that migraineurs had smaller hippocampal volume and stronger hippocampal-cortico-limbic connectivity compared to healthy subjects. Hippocampal volumes and measures of hippocampal volume connectivity with other cortico-limbic network regions are associated with symptoms of allodynia (11). These studies show hippocampal volume changes and support the role of migraine frequency in volume abnormalities. Since we also found a hippocampal tail volume association with migraine frequency, these findings are consistent with our results.
WMLs and migraine characteristics
The thalamic and hippocampal volume changes are likely the consequence of recurrent migraine headaches and accumulate with migraine years (5, 31). The WMLs are worsening with longer migraine disease duration, but they can be found at any age of migraineurs (5). A previous MRI study of supratentorial white matter hyperintensities in migraine patients demonstrated tissue damage with axonal loss, decreased glial cell density with impaired energy metabolism, enlarged extracellular space with an increased extracellular water fraction, and decreased blood flow and volume (31). In addition, reactive oxygen species (ROS) are the contributors to oxidative stress, which can cause vascular endothelial dysfunction in migraines and may lead to tissue damage in WMLs (32).
Both the thalamus and hippocampus are involved in migraine bout-related pain processing, which thereby causes stress. These include headache intensity, frequency, and the dominant hemisphere attack. The pain may have a role in the volume changes, especially in the hippocampi.
Migraine aura is usually unilateral and visual, but it can also be sensory, motor, brainstem, and retinal or cause speech/language disturbance. The cortical spreading depression (CSD) is a slowly propagated wave of depolarization of neurons and glial cells, followed by a subsequent sustained suppression of spontaneous neuronal activity, accompanied by complex and variable changes in vascular caliber, blood flow, and energy metabolism. Although spreading depression (SD) has been most extensively studied in the cortex, the phenomenon may occur in all neural tissues, including the hippocampus, cerebellum, and retina, among other regions (33, 34). Growing evidence suggests that an increased propensity to CSD could be a mechanism involved in the increased prevalence of migraine in women. Both estrogen and progesterone have been reported to increase the frequency of CSD (35).
Beyond the careful new migraine patient selection, the short- or long-term follow-up studies give hope for longitudinal remeasurements of the same patient group, to see further volume changes.
Limitations
The main limitation of this study is that migraine patients were not followed for at least 1 year to obtain precise data on the pain side. Another limitation is the cross-sectional nature of the study, which does not allow for an evaluation of whether the observed volume changes are progressive during the disease course.
We did not adjust p-values for multiple comparisons. However, to avoid data dredging, our investigation was restricted to a small number of brain regions predefined based on a priori hypothesis. The choice of thalamus and hippocampus was based on previous studies, and we have good reasons to believe that both could be involved in migraine. Adjusting p-values for multiple comparisons is still controversial and not always the right choice, especially in studies with a clearly defined primary hypothesis (36).
Thalamus segmentation was performed by Freesurfer 5.3. Having analyzed quite a few of our initial measurements with this version, we did not want to switch to another major version released in the meantime. Such an update is discouraged without repeating all the analyses already completed by Freesurfer 5.3.
Conclusion
The major findings of this study are the following: (1) the volume of the right thalamus is decreased in the white matter lesion migraine subgroup, (2) the volume of the right hippocampus is smaller in migraineurs with aura and WMLs, and (3) the volume of both hippocampus tails is smaller in patients with a higher migraine frequency. These structural abnormalities are likely to be the consequence of recurrent migraine headaches and their negative impact on the traditional migraine characteristics and progressive nature of WMLs. These processes may negatively influence the function of the thalamus and hippocampus in the human brain. These data further support the importance of effective migraine management; briefly, the patients need to follow a healthy lifestyle and avoid almost all migraine risk factors, and the doctors need to investigate co-morbidities if suspected and control the attacks with the most effective therapy.
Data availability statement
The raw data supporting the conclusions of this article will be made available by the authors, without undue reservation.
Ethics statement
The studies involving humans were approved by Regional Research Ethics Committee of the Medical Center, Pécs. The studies were conducted in accordance with the local legislation and institutional requirements. The participants provided their written informed consent to participate in this study.
Author contributions
MH: Conceptualization, Formal analysis, Methodology, Writing—review and editing. GK-J: Data curation, Investigation, Writing—review and editing. HK: Formal analysis, Writing—review and editing. GP: Conceptualization, Data curation, Formal analysis, Investigation, Methodology, Writing—original draft, Writing—review and editing. GO: Formal analysis, Writing—review and editing. EB: Data curation, Investigation, Writing—review and editing. RR: Data curation, Investigation, Writing—review and editing. FJ: Data curation, Investigation, Writing—review and editing. AT: Data curation, Investigation, Writing—review and editing. KE: Writing—original draft, Writing—review and editing. ZP: Conceptualization, Data curation, Investigation, Methodology, Writing—original draft, Writing—review and editing.
Funding
This study was supported by grants from EEA/Norwegian Financial Mechanism HU 0114—Save what can be saved—applied neurological research using high-field magnetic resonance imaging, by TÁMOP-4.2.1/B, EFOP-3.6.2-16-2017-00008. The role of neuroinflammation in neurodegeneration: from molecules to clinics, TKP2021-EGA-16 and TKP-2021-EGA-13. Project Nos. TKP2021-EGA-16 and TKP-2021-EGA-13 have been implemented with the support provided by the National Research, Development and Innovation Fund of Hungary, financed under the TKP2021-EGA funding scheme. This study was also supported by the Hungarian Brain Research Program 2 and 3 (2017-1.2.1-NKP-2017-00002 and NAP 3.0) and the Translational Neuroscience National Laboratory (RRF-2.3.1-21-2022-00011). ZP was funded by PTE ÁOK-KA-2017-23 and EFOP-3.6.3-VEKOP_00009.
Conflict of interest
The authors declare that the research was conducted in the absence of any commercial or financial relationships that could be construed as a potential conflict of interest.
Publisher's note
All claims expressed in this article are solely those of the authors and do not necessarily represent those of their affiliated organizations, or those of the publisher, the editors and the reviewers. Any product that may be evaluated in this article, or claim that may be made by its manufacturer, is not guaranteed or endorsed by the publisher.
Abbreviations
ANCOVA, analysis of covariance; CSD, cortical spreading depression; FLAIR, fluid-attenuated inversion recovery; ICV, intracranial volume; 3D MPRAGE, three-dimensional magnetization-prepared rapid gradient-echo; MRI, magnetic resonance imaging; SPSS, statistical package for the social sciences; ROS, reactive oxygen species; SD, spreading depression; WMLs, white matter lesions.
References
1. Headache Classification Committee of the International Headache Society (IHS). The International Classification of Headache Disorders, 3rd edition. Cephalalgia. (2018) 38:1–211. doi: 10.1177/0333102417738202
2. Macgregor EA, Rosenberg JD, Kurth T. Sex-related differences in epidemiological and clinic-based headache studies. Headache. (2011) 51:843–59. doi: 10.1111/j.1526-4610.2011.01904.x
3. Finocchi C, Strada L. Sex-related differences in migraine. Neurol Sci. (2014) 35 (Suppl. 1):207–13. doi: 10.1007/s10072-014-1772-y
4. Vetvik KG, MacGregor EA. Sex differences in the epidemiology, clinical features, and pathophysiology of migraine. Lancet Neurol. (2017) 16:76–87. doi: 10.1016/S1474-4422(16)30293-9
5. Erdélyi-Bótor S, Aradi M, Kamson DO, Kovács N, Perlaki G, Orsi G, et al. Changes of migraine-related white matter hyperintensities after 3 years: a longitudinal MRI study. Headache. (2015) 55:55–70. doi: 10.1111/head.12459
6. Komáromy H, He M, Perlaki G, Orsi G, Nagy SA, Bosnyák E, et al. Influence of hemispheric white matter lesions and migraine characteristics on cortical thickness and volume. J Headache Pain. (2019) 20:4. doi: 10.1186/s10194-019-0959-2
7. Eliot L, Ahmed A, Khan H, Patel J. Dump the “dimorphism”: comprehensive synthesis of human brain studies reveals few male-female differences beyond size. Neurosci Biobehav Rev. (2021) 125:667–97. doi: 10.1016/j.neubiorev.2021.02.026
8. DeCasien AR, Guma E, Liu S, Raznahan A. Sex differences in the human brain: a roadmap for more careful analysis and interpretation of a biological reality. Biol Sex Differ. (2022) 13:43. doi: 10.1186/s13293-022-00448-w
9. Moskowitz MA. Trigeminovascular system. Cephalalgia. (1992) 12:127. doi: 10.1046/j.1468-2982.1992.1203127.x
10. Younis S, Hougaard A, Noseda R, Ashina M. Current understanding of thalamic structure and function in migraine. Cephalalgia. (2019) 39:1675–82. doi: 10.1177/0333102418791595
11. Chong CD, Dumkrieger GM, Schwedt TJ. Structural co-variance patterns in migraine: a cross-sectional study exploring the role of the hippocampus. Headache. (2017) 57:1522–31. doi: 10.1111/head.13193
12. Liu HY, Chou KH, Chen WT. Migraine and the hippocampus. Curr Pain Headache Rep. (2018) 22:13. doi: 10.1007/s11916-018-0668-6
13. Fischl B, Salat DH, Busa E, Albert M, Dieterich M, Haselgrove C, et al. Whole brain segmentation: automated labeling of neuroanatomical structures in the human brain. Neuron. (2002) 33:341–55. doi: 10.1016/S0896-6273(02)00569-X
14. Iglesias JE, Augustinack JC, Nguyen K, Player CM, Player A, Wright M, et al. A computational atlas of the hippocampal formation using ex vivo, ultra-high-resolution MRI: application to adaptive segmentation of in vivo MRI. Neuroimage. (2015) 115:117–37. doi: 10.1016/j.neuroimage.2015.04.042
15. Keller SS, Gerdes JS, Mohammadi S, Kellinghaus C, Kugel H, Deppe K, et al. Volume estimation of the thalamus using freesurfer and stereology: consistency between methods. Neuroinformatics. (2012) 10:341–50. doi: 10.1007/s12021-012-9147-0
16. Szabo CA, Xiong J, Lancaster JL, Rainey L, Fox P. Amygdalar and hippocampal volumetry in control participants: differences regarding handedness. Am J Neuroradiol. (2001) 22:1342–5.
17. Jang H, Lee JY, Lee KI, Park KM. Are there differences in brain morphology according to handedness? Brain Behav. (2017) 7:e00730. doi: 10.1002/brb3.730
18. La Pegna GB, Quatrosi G, Vetri L, Reina F, Galati C, Manzo ML, et al. Migraine and handedness. Neurol Sci. (2021) 42:2965–8. doi: 10.1007/s10072-021-05111-7
19. Shin KJ, Lee HJ, Park KM. Alterations of individual thalamic nuclei volumes in patients with migraine. J Headache Pain. (2019) 20:112. doi: 10.1186/s10194-019-1063-3
20. Magon S, May A, Stankewitz A, Goadsby PJ, Tso AR, Ashina M, et al. Morphological abnormalities of thalamic subnuclei in migraine: a multicenter MRI study at 3 tesla. J Neurosci. (2015) 35:13800–6. doi: 10.1523/JNEUROSCI.2154-15.2015
21. Yang Y, Xu H, Deng Z, Cheng W, Zhao X, Wu Y, et al. Functional connectivity and structural changes of thalamic subregions in episodic migraine. J Headache Pain. (2022) 23:119. doi: 10.1186/s10194-022-01491-z
22. Hougaard A, Nielsen SH, Gaist D, Puonti O, Garde E, Reislev NL, et al. Migraine with aura in women is not associated with structural thalamic abnormalities. Neuroimage Clin. (2020) 28:102361. doi: 10.1016/j.nicl.2020.102361
23. Liu HY, Chou KH, Lee PL, Fuh JL, Niddam DM, Lai KL, et al. Hippocampus and amygdala volume in relation to migraine frequency and prognosis. Cephalalgia. (2017) 37:1329–36. doi: 10.1177/0333102416678624
24. Liu J, Lan L, Li G, Yan X, Nan J, Xiong S, et al. Migraine-related gray matter and white matter changes at a 1-year follow-up evaluation. J Pain. (2013) 14:1703–8. doi: 10.1016/j.jpain.2013.08.013
25. Maleki N, Becerra L, Brawn J, McEwen B, Burstein R, Borsook D. Common hippocampal structural and functional changes in migraine. Brain Struct Funct. (2013) 218:903–12. doi: 10.1007/s00429-012-0437-y
26. Glasper ER, Schoenfeld TJ, Gould E. Adult neurogenesis: optimizing hippocampal function to suit the environment. Behav Brain Res. (2012) 227:380–3. doi: 10.1016/j.bbr.2011.05.013
27. McEwen BS, Kalia M. The role of corticosteroids and stress in chronic pain conditions. Metabolism. (2010) 59 (Suppl. 1):S9–15. doi: 10.1016/j.metabol.2010.07.012
28. Milde-Busch A, Blaschek A, Heinen F, Borggräfe I, Koerte I, Straube A, et al. Associations between stress and migraine and tension-type headache: results from a school-based study in adolescents from grammar schools in Germany. Cephalalgia. (2011) 31:774–85. doi: 10.1177/0333102410390397
29. Craft RM, Mogil JS, Aloisi AM. Sex differences in pain and analgesia: the role of gonadal hormones. Eur J Pain. (2004) 8:397–411. doi: 10.1016/j.ejpain.2004.01.003
30. McEwen BS. Plasticity of the hippocampus: adaptation to chronic stress and allostatic load. Ann N Y Acad Sci. (2001) 933:265–77. doi: 10.1111/j.1749-6632.2001.tb05830.x
31. Aradi M, Schwarcz A, Perlaki G, Orsi G, Kovács N, Trauninger A, et al. Quantitative MRI studies of chronic brain white matter hyperintensities in migraine patients. Headache. (2013) 53:752–63. doi: 10.1111/head.12013
32. Erdélyi-Bótor S, Komáromy H, Kamson DO, Kovács N, Perlaki G, Orsi G, et al. Serum L-arginine and dimethylarginine levels in migraine patients with brain white matter lesions. Cephalalgia. (2017) 37:571–80. doi: 10.1177/0333102416651454
33. Lauritzen M. Cortical spreading depression in migraine. Cephalalgia. (2001) 21:757–60. doi: 10.1177/033310240102100704
34. Charles A, Brennan K. Cortical spreading depression-new insights and persistent questions. Cephalalgia. (2009) 29:1115–24. doi: 10.1111/j.1468-2982.2009.01983.x
35. Sachs M, Pape HC, Speckmann EJ, Gorji A. The effect of estrogen and progesterone on spreading depression in rat neocortical tissues. Neurobiol Dis. (2007) 25:27–34. doi: 10.1016/j.nbd.2006.08.013
Keywords: episodic migraine, white matter lesions, migraine headache characteristics, volume of thalamus and hippocampus, right-handed female migraineurs
Citation: He M, Kis-Jakab G, Komáromy H, Perlaki G, Orsi G, Bosnyák E, Rozgonyi R, John F, Trauninger A, Eklics K and Pfund Z (2023) The volume of the thalamus and hippocampus in a right-handed female episodic migraine group. Front. Neurol. 14:1254628. doi: 10.3389/fneur.2023.1254628
Received: 07 July 2023; Accepted: 25 September 2023;
Published: 19 October 2023.
Edited by:
Raffaele Ornello, University of L'Aquila, ItalyReviewed by:
Ana Starcevic, University of Belgrade, SerbiaCsaba Juhasz, Wayne State University, United States
David Olayinka Kamson, Johns Hopkins Medicine, United States
Mansoureh Togha, Tehran University of Medical Sciences, Iran
Copyright © 2023 He, Kis-Jakab, Komáromy, Perlaki, Orsi, Bosnyák, Rozgonyi, John, Trauninger, Eklics and Pfund. This is an open-access article distributed under the terms of the Creative Commons Attribution License (CC BY). The use, distribution or reproduction in other forums is permitted, provided the original author(s) and the copyright owner(s) are credited and that the original publication in this journal is cited, in accordance with accepted academic practice. No use, distribution or reproduction is permitted which does not comply with these terms.
*Correspondence: Zoltán Pfund, cGZ1bmQuem9sdGFuQHB0ZS5odQ==