- 1Department of Emergency, Longhua Hospital, Shanghai University of Traditional Chinese Medicine, Shanghai, China
- 2Shanghai Skin Disease Hospital, Skin Disease Hospital of Tongji University, Shanghai, China
- 3Department of Neurosurgery, Union Hospital, Tongji Medical College, Huazhong University of Science and Technology, Wuhan, Hubei, China
- 4School of Acupuncture-Moxibustion and Tuina, Shanghai University of Traditional Chinese Medicine, Shanghai, China
- 5Institute of Critical Care, Shanghai University of Traditional Chinese Medicine, Shanghai, China
The endovascular intervention technique has gained prominence in the treatment of intracranial aneurysms due to its minimal invasiveness and shorter recovery time. A critical step of the intervention is the shaping of the microcatheter, which ensures its accurate placement and stability within the aneurysm sac. This is vital for enhancing coil placement and minimizing the risk of catheter kickback during the coiling process. Currently, microcatheter shaping is primarily reliant on the operator's experience, who shapes them based on the curvature of the target vessel and aneurysm location, utilizing 3D rotational angiography or CT angiography. Some researchers have documented their experiences with conventional shaping methods. Additionally, some scholars have explored auxiliary techniques such as 3D printing and computer simulations to facilitate microcatheter shaping. However, the shaping of microcatheters can still pose challenges, especially in cases with complex anatomical structures or very small aneurysms, and even experienced operators may encounter difficulties, and there has been a lack of a holistic summary of microcatheter shaping techniques in the literature. In this article, we present a review of the literature from 1994 to 2023 on microcatheter shaping techniques in endovascular aneurysm embolization. Our review aims to present a thorough overview of the various experiences and techniques shared by researchers over the last 3 decades, provides an analysis of shaping methods, and serves as an invaluable resource for both novice and experienced practitioners, highlighting the significance of understanding and mastering this technique for successful endovascular intervention in intracranial aneurysms.
Introduction
In light of recent advancements in technology and material sciences, endovascular intervention techniques have emerged as significant therapeutic modalities for managing intracranial aneurysms (1). These techniques have garnered preference from both patients and clinicians owing to their minimally invasive nature and expedited recovery period (2, 3). Nonetheless, the execution of endovascular embolization therapy, whether it employs simple coil embolization or is supplemented by stents or balloons, faces a principal technical challenge in accurately navigating the microcatheter into the aneurysm sac and ensuring its stability therein (4). Optimal shaping of the microcatheter enhances its stability within the aneurysm sac, promotes effective coil placement, and curtails the risk of catheter kickback during the coiling process. Therefore, in order to successfully complete intracranial aneurysm embolization, shaping the microcatheter into an appropriate form is crucial, and achieving proper microcatheter shaping is a critical step (5, 6).
At present, the procedure of microcatheter shaping primarily depends on the experience of the operator, who bends them to approximate the desired shape according to the curvature of the target vessel and the position of the aneurysm with three-dimensional (3D) rotational angiography or computed tomography angiography (CTA) (7). Notably, in exceptional instances involving complex anatomical structures or diminutive aneurysms, even experienced operators might have to reshape the microcatheter multiple times or face challenges in accomplishing successful shaping (8). Additionally, in the process of evolving from an inexperienced novice to a skilled interventionalist, understanding comprehensively the technique of microcatheter shaping, and gradually accumulating experience, is also an important step in learning the technique of endovascular intervention (9). Hence, despite microcatheter shaping being a routine step in endovascular aneurysm embolization, its implementation can be challenging.
Concerning the topic of microcatheter shaping, diverse researchers have contributed their experiences with conventional shaping methods. Moreover, certain scholars have utilized auxiliary techniques such as 3D printing and computer simulations to assist with microcatheter shaping (10, 11). Despite these contributions, a comprehensive synthesis of microcatheter shaping techniques, to the best of our knowledge, is still lacking in scholarly literature. Consequently, this article aims to collate and review previously reported techniques in microcatheter shaping.
Methods and search strategy
The literature search was performed to identify studies regarding the application of microcatheter shaping in intracranial aneurysm embolization. We identified key terms and phrases related to microcatheter shaping in the treatment of intracranial aneurysms, such as “Microcatheter,” “Shaping,” “Intracranial Aneurysms,” “Endovascular Treatment,” and “Neurointervention.” We combined these terms using Boolean operators to formulate our search strings. The literature search was conducted based on the PubMed, Web of Science, Scopus, ScienceDirect, J-STAGE, and CNKI databases for relevant publications from January 1994 to May 2023. Additionally, we utilized Google Scholar to ensure broader coverage. Literature types included all English and Chinese articles, such as original articles, case reports, and reviews. Meta-analyses were not included as there were no published ones in this field. After completing the data retrieval, two authors independently screened the titles and abstracts of the literature, while all authors collectively assessed the full texts of potentially relevant articles and whether they could be included in our study (illustrated in Figure 1).
The shapability of different microcatheters
Initiating the microcatheter shaping process necessitates an in-depth comprehension of the shapability performance of different microcatheter types. In the past, several publications have reported scholarly research focusing on this particular aspect (Table 1). The first literature report on the comparative study of shapability performance of different types of microcatheters was illustrated by Abe et al. (12). In their study, the distal tip of five types of microcatheters were shaped into a 90° turn with distal straight segment lengths of 3, 5, or 7 mm, respectively. The authors investigated the modifications in the curvature of the shaped microcatheters under various conditions: post-insertion into a guiding catheter, post-microguidewire insertion through the microcatheter, and post-Guglielmi detachable coil (GDC) placement through the microcatheter. Kiyosue et al. (13) conducted an evaluation and comparison of 12 microcatheter types. Following the insertion of shaping mandrels into the microcatheters, the distal tips were flexed at 90° and 150°, respectively, and measured using a goniometer. The microcatheters were then exposed to steam for 20 s, followed by immersion in 17°C water for an additional 20 s. Upon the removal of the shaping mandrels, the shaping angles were measured and subjected to further analysis.
In another study, Fujimoto et al. (15) assessed the shapability of four microcatheter types, including Excelsior SL-10 (Stryker, Kalamazoo, MI, USA), Echelon-10 (Medtronic, Minneapolis, MN, USA), XT-17 (Stryker, Kalamazoo, MI, USA), and Headway-17 (MicroVention TERUMO, Tustin, CA, USA). In their method, a mandrel was inserted into a microcatheter, and the distal end was coiled with a diameter of 4 mm for 1, 2, and 3 turns, respectively, before being steam-shaped for 30 s. The microcatheter was subsequently immersed in 37°C physiological saline for 10 min. Subsequently, a microguidewire was inserted into the microcatheter, extending 2 cm from the distal end of the microcatheter. Their findings demonstrated that immediately after steam shaping and removal of the mandrel, all four types of microcatheters, when coiled for one turn, displayed a semi-circular shape (204°, average diameter of 8.7 mm). Those coiled for two turns had an average remaining coil of 1.2 turns (472°, average diameter of 7.7 mm), while the ones coiled for three turns had an average remaining coil of 1.8 turns (671°, average diameter of 7.9 mm). No significant differences in morphology were observed among the three shaping conditions. Nevertheless, after immersion in physiological saline for 10 min, there were substantial morphological changes in Excelsior SL-10 and Echelon-10 microcatheters, whereas Headway-17 and XT-17 microcatheters exhibited better shape retention capabilities. Despite the numerous types of microcatheters available in the current market, research on the performance of microcatheters remains limited, and the understanding of shaping techniques is largely dependent on the personal experience of interventionalists. Based on our center's experience, we believe that the Headway-17 microcatheter can achieve a 1:1 shaping ratio based on the angle between the parent artery and the long axis of the aneurysm. Additionally, microcatheters such as Excelsior SL-10, Echelon-10, and Echelon-14 can achieve a 1:2 shaping ratio (illustrated in Figure 2).
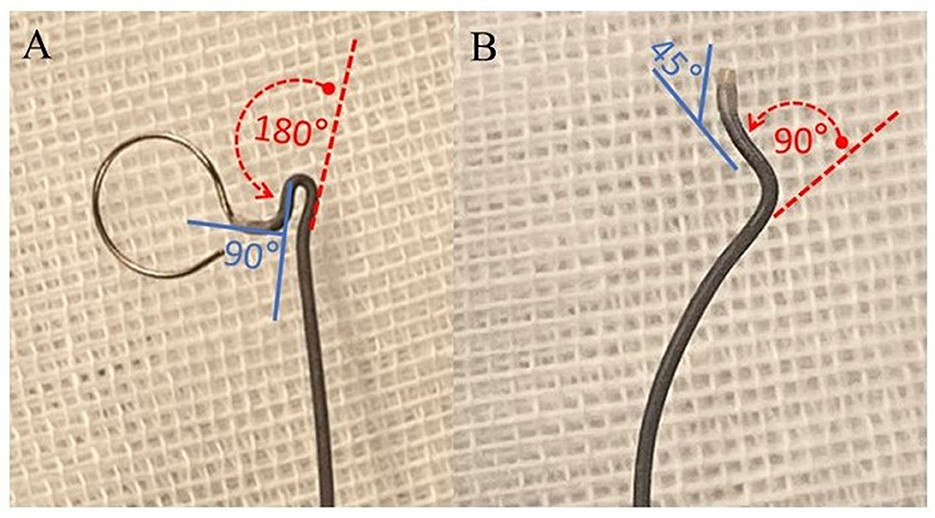
Figure 2. Diagram illustrating the shaping ratio of the microcatheter. (A) Insert the mandrel into a linear Echelon microcatheter. Subsequently, flex the segment proximal to the tip to an angle of 90° (indicated by the blue solid line) and the segment distal to the tip to 180° (denoted by the red dashed arrow). (B) Upon co-heating the microcatheter with the mandrel for shaping and subsequently extracting the mandrel, the segment of the microcatheter proximate to the tip rebounds to an approximate angle of 45° (blue solid line), while the segment distal to the tip rebounds to an angle of 90° (red dashed arrow). This attribute is denominated as the capability to achieve a 1:2 shaping ratio.
The heating condition for shaping
After manipulating the mandrel, the usual procedure for interventionalists involves applying heat for a certain duration, then immersing it in cold water, with the expectation that the distal end of the microcatheter will maintain this particular shape reliably. Akihiko et al. (17) explored this issue of heating methods in their research. They examined the effects of different heating durations on the shaping of the headway microcatheter using four commonly used equipment, namely, a heating plate + kettle, an electric kettle, a steamer, and a hot air gun. The results indicated that under the temperature measurement condition at a distance of 25 mm, the heating plate + kettle, electric kettle, and steamer took a longer time to reach a stable peak temperature, which were 80, 95, and 60°C, respectively. In contrast, the hot air gun reached a stable peak temperature rapidly upon activation though the actual measured temperature was 20°C below the set value. Regarding the effect of heating on the microcatheter, their result indicated that the headway microcatheter retained its optimal shaping after being subjected to heating at 100°C for 90 s, with no significant surface damage observed. Hence, they concluded that using a hot air gun to maintain a heating duration of 90 s at 100°C resulted in the best shaping effect for the microcatheter. In another study, Tomio et al. (18) explored the optimal shaping conditions for Echelon-10 and Excelsior SL-10 microcatheters using a similar methodology. Their result revealed that by subjecting the microcatheters to continuous heating for 30 s at a set temperature of 120°C using a hot air gun, both types of microcatheters achieved the desired shaping curvature under a twice-bending status. Additionally, these microcatheters were able to endure 2–3 reshaping cycles under this heating condition.
Conventional microcatheter shaping method
In the current process of endovascular embolization for intracranial aneurysms, the prevalent method of microcatheter shaping involves the operator, utilizing three-dimensional reconstructed images after completion digital subtraction angiography (DSA) examination, in conjunction with their own experience, to manually shape the microcatheter by inserting a mandrel into the tip of the microcatheter and manipulating it to match the morphological traits of the lesion site. Over the past few decades, some scholars have analyzed and reported on these methods and experiences (Table 2). Kwon et al. (19) shared their experience of microcatheter shaping in the treatment of paraclinoid aneurysms. They encompassed 132 paraclinoid aneurysms and categorized the shaping forms into straight, primary curves (45°, 90°, and J-shaped), C-shaped, pigtail-shaped, and S-shaped. For paraclinoid aneurysms growing superiorly or medially, they found the S-shaped (54.5%) and pigtail-shaped (60.2%) forms to be the most frequently used, respectively. After analysis, they suggested that an S-shaped for superiorly directed aneurysms and a pigtail-shaped for medially directed aneurysms appear to be suitable for microcatheter shapes. In a further delineation, Wang et al. (20) subdivided paraclinoid aneurysms into four subtypes based on location and direction and proposed four corresponding microcatheter shaping strategies: “straight shaped,” “S-shaped,” “C-shaped,” and “primary curve.”
For proximal anterior cerebral artery aneurysms, Chang et al. (22) underlined the necessity of tailored-shaped or S-shaped microcatheters based on three-dimensional reconstructed images. In another study on endovascular treatment of proximal anterior cerebral artery aneurysms, Lee et al. (23) utilized various microcatheter shaping forms and deduced that a Z-shaped morphology at the tip of the microcatheter facilitated smooth access into the proximal aneurysmal sac in the A1 segment and provided a stable embolization pathway. In another study focused on 11 cases of anterior cerebral artery aneurysms, the authors also documented the application of “S-shaped” or “Z-shaped” microcatheter shaping strategies by the operators (25). Moreover, in a case series reported by Huo et al. (24) involved 15 patients with anterior cerebral artery aneurysms, the microcatheter-shaping strategies were further categorized into several types based on the location and growth direction of the aneurysms.
It is important to note that these conventional shaping methods largely originate from the accumulation of experiences and personal insights of different operators. Additionally, the cases reported in the above literature primarily focus on relatively common sites within the intracranial anterior circulation system. Therefore, the universal applicability of conventional shaping methods in addressing the individual characteristics of different cases, as well as complex cases, and the understanding and learning needs of inexperienced novices still pose considerable challenges.
Improvement attempts on conventional shaping methods
Building upon the previously mentioned microcatheter shaping methods, some scholars have also attempted to make improvements in order to achieve greater stability of the microcatheter. Jia et al. (5) presented a microcatheter shaping technique called “loop technique” based on the principle of the interaction forces between the vessel wall opposite to the aneurysm neck and the curved portion of the proximal vessel wall with the microcatheter for coil embolization of paraclinoid aneurysms. In this technique, the distal part of the microcatheter was steam-shaped into a loop, forming the second curve, while the tip was further shaped to align with the long axis of the aneurysm, creating the first curve. The third curve was designed to align with the cavernous genu portion of the internal carotid artery (ICA). By utilizing the interaction forces, the aim was to enhance the stability of the microcatheter during the embolization procedure. However, the author also pointed out that this approach made it more challenging to adjust the microcatheter tip toward the aneurysmal sac. Additionally, this method carries risks of damaging the vessel wall and getting hooked with the coil loops.
Another improvement came from Ohshima et al. (31). Building upon the conventional shaping techniques, they introduced a modification where the distal 1–2 mm of the microcatheter tip was bent and formed a “Γ” shape. They observed that the microcatheters with the “Γ” tip demonstrated improved movement and oscillation during coiling and reduced coil protrusion into the parent artery and decreased microcatheter kickback. However, their study was limited to in vitro experiments and lacked corresponding clinical case applications. Additionally, based on our experience, the “Γ” tip carries the risk of getting hooked with coil loops, especially when coiling the neck of the aneurysm. If there was no prior plan for a stent-assisted or balloon-assisted approach, dealing with such an issue can be challenging once it arises.
Furthermore, Kwak et al. (32) reported a case of endovascular treatment for an ultrawide-necked circumferential aneurysm of the middle cerebral artery. During the procedure, the authors shaped two microcatheters into a spring form and positioned them at the distal portion of the aneurysm. The intention was for the microcatheters to coil around the stent after its deployment, allowing the coils to distribute evenly within the aneurysm sac. In this article, the authors proposed a novel microcatheter shaping technique for the treatment of circumferential aneurysms or fusiform aneurysms. However, it should be noted that the authors utilized the LVIS BLUE (MicroVention TERUMO, Tustin, CA, USA) stent, which was a flow-diverting device with a tight mesh design and minimized the coil encroachment into the stent. In this study, whether this method is applicable to laser-cut or braided stents was not addressed.
Different attempts in the process of conventional shaping
As mentioned above, in the conventional microcatheter shaping process, the operator shaped and heat the microcatheter with inserting the mandrel into the tip. However, for complex lesion structures or cases requiring significant reshaping, the insertion of the mandrel may cause changes in the curvature of the microcatheter tip, leading to a reduction in shaping accuracy. Therefore, several scholars have attempted different approaches in the shaping process. Tomotaka et al. (33) proposed a shaping process named microcatheter shaping cast. In their report, the operators utilized a metallic introducer to coil the mandrel 4–5 times, creating a stent-like handmade cast. Subsequently, the microcatheter was inserted into the cast, and manual bending was performed according to the morphology of the lesion with a hot air gun for shaping. However, the authors of this article did not provide any demonstration of the practical application of this method in the actual case. Furthermore, we attempted to apply this method during endovascular treatment procedures but encountered difficulties in achieving uniform coiling of the mandrel and bending of the cast, which in turn affected the shaping process.
Another attempted process for forming the shape of the microcatheter was called “intravascular placement” or “endovascular shaping.” In Shinya et al. (34) reported on this method first in a series of 15 cases. They delivered an SL-10 straight microcatheter to the neck of the aneurysm and left it in the parent artery for 5 min. After pulling out the microcatheter, it acquired a certain curvature. Subsequently, based on the 3D reconstructed images of the vessel and aneurysm, the tip of the microcatheter was steam-shaped into the corresponding form. In another report, Katsunari et al. (35) used an XT-17 microcatheter and employed the same method to treat vertebral basilar aneurysms. Ultimately, accurate microcatheter shaping was achieved in all five cases, and the procedures were successfully completed. Moreover, Shinoda et al. (36) employed the same shaping method to treat a case of blister-like aneurysm. Yoshiki et al. (37) reviewed 10 cases of bifurcation aneurysms treated using this shaping method and similarly obtained satisfactory results. We believe that “endovascular shaping” can be attempted for cases with more complex structures or cases where multiple reshaping attempts have been unsatisfactory. However, compared to conventional shaping methods, an additional 5-min microcatheter delivery procedure theoretically increases the risk of complications such as vascular injury and intravascular thrombus formation. Furthermore, this procedure will prolong the duration of microcatheter shaping and overall operational time.
Shaping based on 3D model reconstruction and printing technology
During the microcatheter shaping process, 3D rotational angiography provided assistance to the interventionalists in understanding the structural morphology of the lesion. However, this observation was achieved solely through a two-dimensional computer screen, lacking a correct depth perception (38). Therefore, some scholars have attempted to use in vitro 3D models and printing technology to gain a more comprehensive understanding and analysis of the lesion structure, thus aiding in the precise shaping of the microcatheter (Table 3). Kenichi et al. (39) reported for the first time the creation of a specific silicone vascular model of the lesion site in a patient with anterior communicating artery aneurysm based on 3D rotational angiography. They performed preoperative simulated treatment, focusing particularly on microcatheter navigation and tip shape. In another study, Katsunari et al. (40) reported the use of 3D printing rapid prototyping technology to assist in microcatheter shaping. The authors created a 3D solid aneurysm model based on digital imaging and communications in medicine (DICOM) data to guide microcatheter shaping, followed by the use of a hollow 3D aneurysm model to test the accuracy of the shaping. Subsequently, the pre-shaped microcatheter was used to perform coil embolization treatment. Ultimately, in their series of cases, all 10 patients achieved accurate microcatheter shaping. Toshihiro et al. (10) and Zeng et al. (41) expanded the number of cases using this shaping method. In their report, a total of 27 aneurysms and 31 aneurysms were treated with the assistance of 3D printing technology for microcatheter shaping, respectively, and the authors concluded that this tailor-made shaping method yielded satisfactory outcomes.
Xu et al. (8) adopted a more ingenious approach to utilize 3D printing technology in assisting microcatheter shaping. They created hollow and translucent 3D models that were immersed in water. Subsequently, the microcatheter was introduced into the target position in the models, and the water temperature was heated to 50°C for 5 min to achieve the desired shape of the microcatheter. Finally, the embolization procedures were successfully performed. In another study, Song et al. (11) applied the same method to treat 16 patients with aneurysms. Additionally, they made a small improvement to this method by creating perforators on the surface of the model to facilitate steam heating. In a similar manner, Nakajima et al. (44) inserted a mandrel into a 3D-printed hollow model to create an ideal shape to assist in shaping the microcatheter under the condition of heating with a hot air gun.
3D reconstruction modeling and printing technology provided operators with a relatively accurate and customized microcatheter shaping aid for specific patients, which theoretically facilitated the implementation of endovascular treatment procedure and improved its safety. However, this method still had certain limitations. Due to the process of obtaining DICOM data and forming the models, as well as the time required for model sterilization, it typically takes ~1 day. Therefore, for patients requiring emergency operations for ruptured aneurysms, the application of 3D printing technology posed difficulties.
Computer simulation technology assisted shaping
In recent years, with the continuous development of computer technology, computer algorithm-based simulation techniques have gradually been applied in the field of cerebral endovascular therapy (45–47). Song et al. (48) introduced an intelligent microcatheter shaping method platform named UKnow, developed by Union Strong (Beijing) Technology Co. Ltd., based on a computer algorithm model, and validated it on the in vitro silicone models, which provided a novel method and system for assisting intracranial aneurysm embolization. In their algorithm model, the computer software performed a 3D reconstruction of the parent artery and aneurysm based on the acquired DICOM data, utilized “collision detection” and “centerline constraint” algorithms for path simulation and correction, as well as automatically generated the expected microcatheter shape and mandrel shape. Finally, the interventionalist applied the computer-generated solution to the physical microcatheter and mandrel for shaping operations.
Based on this software and algorithm (illustrated in Figure 3) (6), we conducted a preliminary study on its clinical application. We utilized this microcatheter shaping technique to treat a total of 30 aneurysms in 24 patients. Ultimately, all 30 microcatheters accurately entered the aneurysmal sac, while 25 achieved satisfactory results in terms of position within the sac and intraoperative stability (illustrated in Figure 4) (6). In a recently published multicenter randomized controlled study on the clinical application of this software (49), 101 patients underwent treatment with computer-assisted microcatheter shaping technology (CAMS group), while another 100 patients underwent conventional manual microcatheter shaping methods (MMS group). The result indicated that the CAMS group showed significant superiority over the MMS group in terms of the success rate of the first attempt (96.0 vs. 66.0%, P < 0.001), success rate of microcatheter placement within 5 min (96.0 vs. 72.0%, P < 0.001), microcatheter stability (97.0 vs. 84.0%, P = 0.002), and excellent delivery performance without microwire guidance (45.5 vs. 24.0%, P < 0.001). At the same time, Wu et al. (50). reported on another computer simulation-assisted microcatheter shaping system, AneuShapeTM, which was developed by ArteryFlow Technology Corporation, Hangzhou, China. They applied this system to treat 55 aneurysms, achieved favorable results in terms of accessibility (45/55, 81.8%), positioning (47/55, 85.5%), and stability (46/55, 83.6%), and demonstrated a promising outcome. Furthermore, Gangqin Xu et al. (51) reported a method based on computational fluid dynamics for simulating cerebral blood flow streamline to assist microcatheter shaping, which was successfully applied to seven patients.
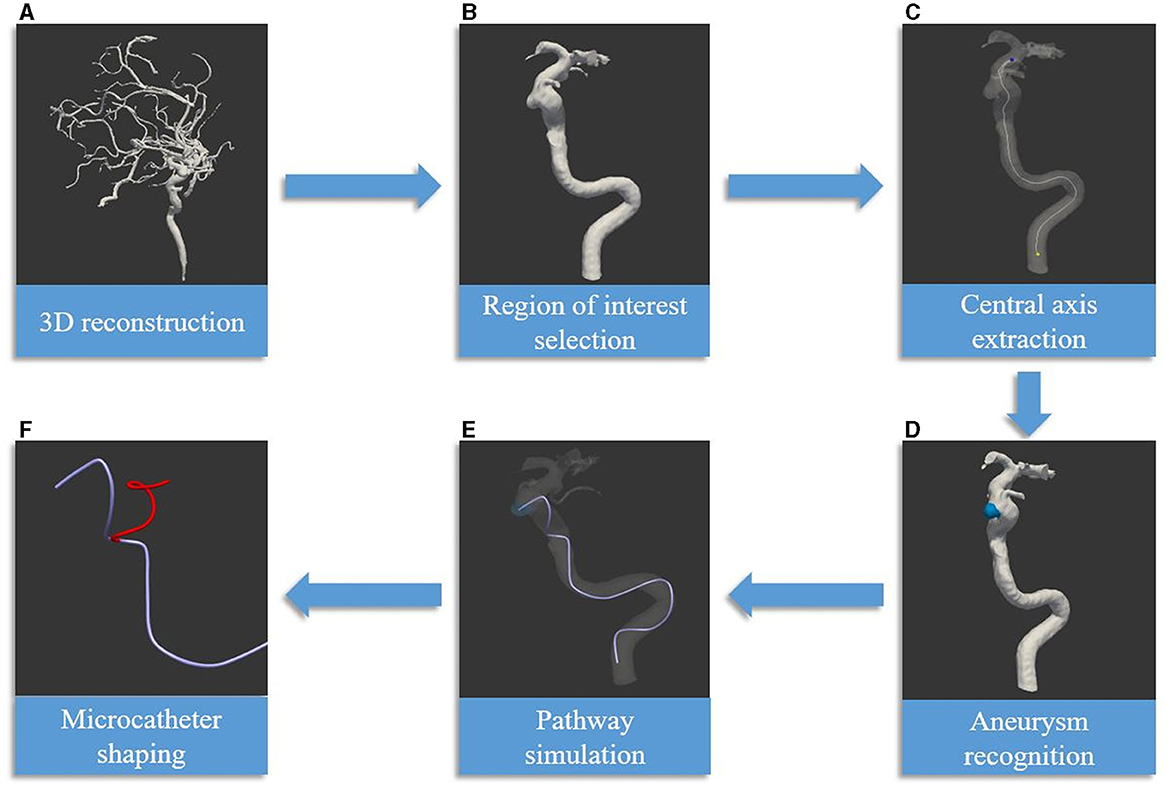
Figure 3. Delineates the process of employing artificial intelligence technology in the shaping of microcatheters [reprinted with permission from Liu et al. (6), © 2022 Elsevier Inc.] (A) The procedure begins with the generation of a three-dimensional vascular image, constructed using DICOM data obtained from CTA or DSA. (B) The subsequent phase involves the segmentation of arteries within the defined region of interest. (C) The centerline is then extracted, serving dual purposes: as the simulation path and as the central axis of the parent artery. (D) The process progresses with the detection of the aneurysm, along with the determination of the target for the microcatheter tip. (E) The artificial intelligence algorithm is then utilized to simulate the path of the microcatheter. (F) The final phase encompasses the creation of the mandrel and microcatheter shapes. This is based on the simulated path and the characteristics of various microcatheter types. The red line symbolizes the optimal mandrel shape, determined through software-calculated parameters and the elasticity features of the microcatheter, exemplified here by the Echelon microcatheter.
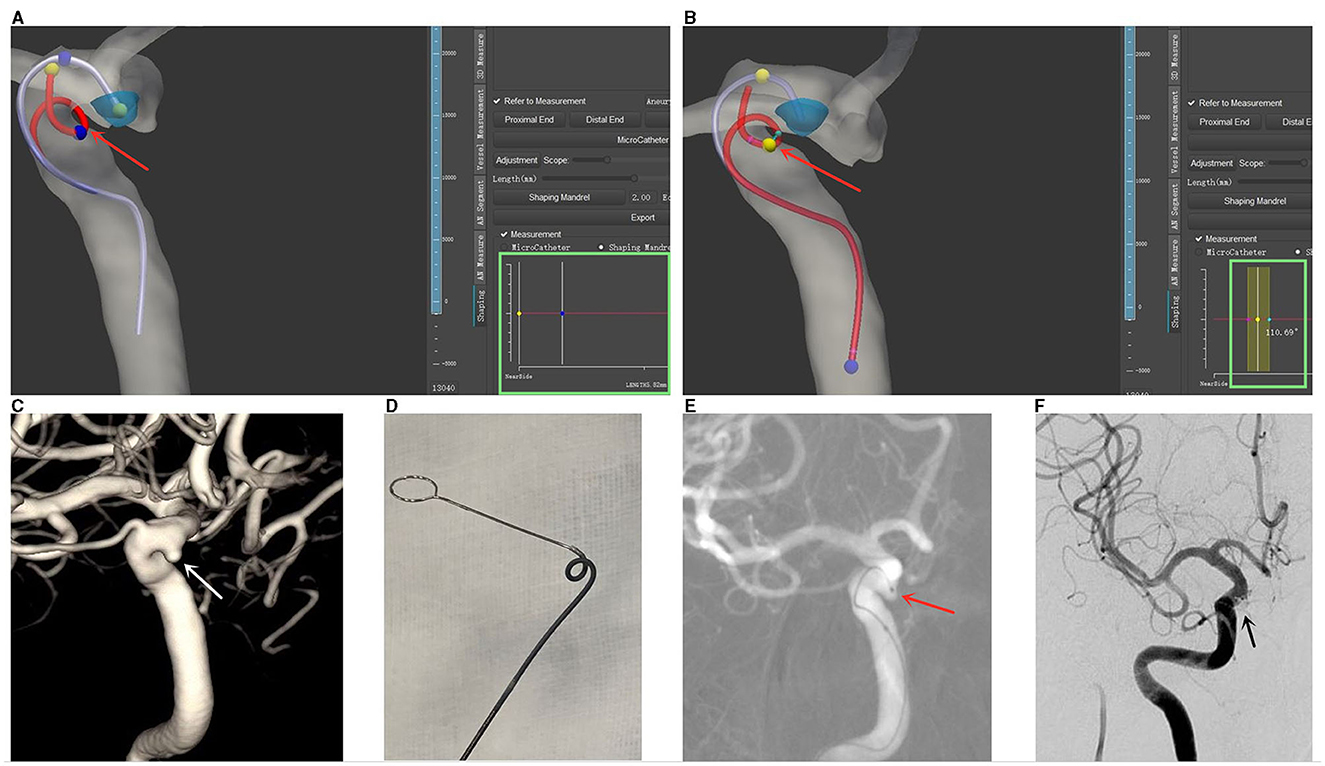
Figure 4. Illustrative case demonstrating computer-assisted microcatheter shaping [reprinted with permission from Liu et al. (6), © 2022 Elsevier Inc.]. (A) Three-dimensional (3D) reconstructions of the aneurysm and the parent artery were generated in the software. The planned trajectory and form of an Echelon microcatheter are denoted by a purple line, while the simulated shaping mandrel is represented by a red line. Adjustable markers in yellow and blue (highlighted by a red arrow) permit the calculation of the distance between them, which is displayed in the green box (measured distance: 5.82 mm). (B) Angular measurements are also enabled at each curve of the simulated shaping mandrel, as indicated by a red arrow. The calculated angle is displayed in the green box (measured angle: 110.69°). (C) The aneurysm is visualized through 3D-DSA, marked by a white arrow. (D) The microcatheter is shaped in vitro, adhering to the pre-calculated trajectory and form. (E) The shaped microcatheter is successfully utilized to access the aneurysm sac on the first attempt, and the tip positioning is deemed satisfactory, as indicated by a red arrow. (F) Successful embolization of the aneurysm is achieved, as denoted by a black arrow.
The emergence of computer algorithm-assisted solution has provided convenience for microcatheter shaping and decision-making during the neurointerventional procedure, particularly for insufficiently experienced interventionalists. It overcame the limitations of the conventional shaping method that heavily relies on the interventionalist's experience, resulting in less accurate shaping. Additionally, it offered a potential advantage in enhancing the safety of procedure. However, it should be noted that how to precisely translate computer-generated shaping schemes to actual mandrels and microcatheters on a 1:1 scale, rather than relying on observation and manual manipulation by the interventionalist, remains an issue that computer simulation technology-assisted shaping needs to confront.
Shaping with steerable articulating tip
In recent years, the introduction of the steerable guidewire and microcatheter has provided a new option for interventional procedures (52, 53). Due to the characteristic of a manipulable bending tip, the steerable guidewire and microcatheter also present a new paradigm for neurointerventionalists in terms of microcatheter shaping and navigation technique. Recently, studies have reported the clinical applications of a microcatheter named Bendit in the field of neurointervention, especially for cases that are traditionally challenging to manage, such as those where intravascular navigation is difficult (54–56). The steerable microcatheter has demonstrated commendable controllability, impressively navigating through intricate vascular structures. Currently, while the application of steerable microcatheters in neurointervention is still in its preliminary phase with small sample sizes, it is believed that with continuous improvements in material and design, along with accumulating clinical experience, steerable microcatheters will offer more possibilities in neurointerventional techniques, ultimately benefiting patients.
Conclusion
In recent years, with the development of neurointerventional theory, techniques, and materials, microcatheter shaping, as an important part of neurointerventional procedure, has undergone various advancements. The shaping methods have evolved from conventional manual shaping to incorporating composite bending, then to 3D model assistance, and now to the emergence of computer algorithm-assisted techniques. Researchers and interventionalists have been continuously exploring and improving these techniques. Accurate insertion and stable placement of microcatheters within aneurysmal sacs are crucial for the success of interventional embolization procedures. Therefore, achieving greater accuracy in microcatheter shaping is an ongoing goal for every interventionalist. In future, the combination of computer algorithm assistance and 3D model printing under sterile conditions in the operating room may represent a new direction and application for the microcatheter shaping method. We believe that the development of these methods and technologies will bring more benefits to interventionalists and patients.
Author contributions
BF, XH, and CL: conception and design. CL and XW: analysis, interpretation of the data, article drafting, and review of the submitted version of manuscript. BF and SZ: article revision. BF, SZ, and CL: final approval of the version to be published. XH, LW, and KG: technical and material support. All authors contributed to the article and approved the submitted version.
Conflict of interest
The authors declare that the research was conducted in the absence of any commercial or financial relationships that could be construed as a potential conflict of interest.
Publisher's note
All claims expressed in this article are solely those of the authors and do not necessarily represent those of their affiliated organizations, or those of the publisher, the editors and the reviewers. Any product that may be evaluated in this article, or claim that may be made by its manufacturer, is not guaranteed or endorsed by the publisher.
Abbreviations
3D, three-dimensional; CTA, computed tomography angiography; GDC, Guglielmi detachable coil; DSA, digital subtraction angiography; ICA, internal carotid artery; DICOM, digital imaging and communications in medicine.
References
1. Molyneux AJ, Kerr RS Yu LM, Clarke M, Sneade M, Yarnold JA, et al. International subarachnoid aneurysm trial (ISAT) of neurosurgical clipping versus endovascular coiling in 2143 patients with ruptured intracranial aneurysms: a randomised comparison of effects on survival, dependency, seizures, rebleeding, subgroups, and aneurysm occlusion. Lancet. (2005) 366:809–17. doi: 10.1016/S0140-6736(05)67214-5
2. Liu C, Guo K, Wu X, Wu L, Cai Y, Hu X, et al. Utility of low-profile visualized intraluminal support (LVIS™) stent for treatment of acutely ruptured bifurcation aneurysms: a single-center study. Front Neurol. (2023) 14:1050369. doi: 10.3389/fneur.2023.1050369
3. Zhao J, Lin H, Summers R, Yang M, Cousins BG, Tsui J. Current treatment strategies for intracranial aneurysms: an overview. Angiology. (2018) 69:17–30. doi: 10.1177/0003319717700503
4. Hanel RA, Kallmes DF, Lopes DK, Nelson PK, Siddiqui A, Jabbour P, et al. Prospective study on embolization of intracranial aneurysms with the pipeline device: the PREMIER study 1 year results. J Neurointerv Surg. (2020) 12:62–6. doi: 10.1136/neurintsurg-2019-015091
5. Jia ZY, Song YS, Sheen JJ, Kim JG, Lee CW, Suh DC. Loop microcatheter technique for coil embolization of paraclinoid aneurysms. Acta Neurochir. (2018) 160:1755–60. doi: 10.1007/s00701-018-3642-5
6. Liu C, Shen Y, Wu X, Qian K, Hu X, Yang H. Artificial intelligence-assisted microcatheter shaping for intracranial aneurysm coiling: a preliminary study. Ann Vasc Surg. (2022) 85:228–36. doi: 10.1016/j.avsg.2022.03.013
7. Pakbaz RS, Kerber CW. Complex curve microcatheters for berry aneurysm endovascular therapy. AJNR. (2007) 28:179–80.
8. Xu Y, Tian W, Wei Z, Li Y, Gao X, Li W, et al. Microcatheter shaping using three-dimensional printed models for intracranial aneurysm coiling. J Neurointerv Surg. (2020) 12:308–10. doi: 10.1136/neurintsurg-2019-015346
9. Nawka MT, Fiehler J, Spallek J, Buhk JH, Frölich AM. Current status of training environments in neuro-interventional practice: are animal models still contemporary? J Neurointerv Surg. (2019) 11:283–9. doi: 10.1136/neurintsurg-2018-014036
10. Ishibashi T, Takao H, Suzuki T, Yuki I, Kaku S, Kan I, et al. Tailor-made shaping of microcatheters using three-dimensional printed vessel models for endovascular coil embolization. Comput Biol Med. (2016) 77:59–63. doi: 10.1016/j.compbiomed.2016.07.005
11. Song X, Qiu H, Tu W, Wang S, Cao Y, Li C, et al. Three-dimensional printing-assisted precision microcatheter shaping in intracranial aneurysm coiling. Neurosurg Rev. (2022) 45:1773–82. doi: 10.1007/s10143-021-01703-1
12. Abe T, Hirohata M, Tanaka N, Uchiyama Y, Fujimoto K, Fujimura N, et al. Distal-tip shape-consistency testing of steam-shaped microcatheters suitable for cerebral aneurysm coil placement. AJNR. (2004) 25:1058–61.
13. Kiyosue H, Hori Y, Matsumoto S, Okahara M, Tanoue S, Sagara Y, et al. Shapability, memory, and luminal changes in microcatheters after steam shaping: a comparison of 11 different microcatheters. AJNR. (2005) 26:2610–6.
14. Abe T, Hirohata M, Tanaka N, Uchiyama Y, Morimitsu H, Fujimura N, et al. Stability of microcatheter for cerebral aneurysm embolization after steam shaping. Interv Neuroradiol. (2004) 10 Suppl 1:117–20. doi: 10.1177/15910199040100S120
15. Fujimoto M, Itokawa H, Moriya M, Okamoto N, Sasanuma J. Comparison of shaping properties among 4 microcatheters—shapability into spirals and shape consistency. J Neuroendovas Ther. (2016) 10:1–11. doi: 10.5797/jnet.tn.2016-0011
16. Wattanasatesiri T, Chung JW, Choi TW, Kim HC, Lee M, Hur S. Shaping the tip of microcatheters for superselective catheterization: steam vs. manual methods. Diag Interv Radiol. (2020) 26:456–63. doi: 10.5152/dir.2020.19314
17. Adachi A, Kobayashi E, Kado K, Saeki N. The optimal conditions for microcatheter shaping. J Neuroendovas Ther. (2016) 10:236–42. doi: 10.5797/jnet.oa.2015-0009
18. Tomio R, Uesugi T, Akaji K. Optimal heating temperature and time for echelon 10 and excelsior SL-10 shaping using a heat gun. J Neuroendovas Ther. (2020) 14:80. doi: 10.5797/jnet.oa.2019-0080
19. Kwon BJ, Im SH, Park JC, Cho YD, Kang HS, Kim JE, et al. Shaping and navigating methods of microcatheters for endovascular treatment of paraclinoid aneurysms. Neurosurgery. (2010) 67:34–40. doi: 10.1227/01.NEU.0000370891.67129.2F
20. Wang Y, Li Y, Jiang C, Wu Z, Jiang F, Meng H, et al. Could the types of paraclinoid aneurysm be used as a criterion in choosing endovascular treatment? Neuro-Radiol View Acta Neurochir. (2013) 155:2019–27. doi: 10.1007/s00701-013-1830-x
21. Toyota S, Fujimoto Y, Iwamoto F, Wakayama A, Yoshimine T. Technique for shaping microcatheter tips in coil embolization of paraclinoid aneurysms using full-scale volume rendering images of 3D rotational angiography. Minim Invasive Neurosurg. (2009) 52:201–3. doi: 10.1055/s-0029-1239588
22. Chang HW, Youn SW, Jung C, Kang HS, Sohn CH, Kwon BJ, et al. Technical strategy in endovascular treatment of proximal anterior cerebral artery aneurysms. Acta Neurochir. (2011) 153:279–85. doi: 10.1007/s00701-010-0804-5
23. Lee HY, Ahn JS, Suh DC, Lee DH. Z-shaped microcatheter tip shaping for embolization of aneurysms at the proximal A1 segment of the anterior cerebral artery: a technical note. Neurointervention. (2011) 6:95–9. doi: 10.5469/neuroint.2011.6.2.95
24. Xiaochuan H, Xiaoyun S, Youxiang L, Ning G, Wenshi G, Junsheng L. Endovascular treatment of unruptured A1 segment aneurysms. Interv Neuroradiol. (2013) 19:186–94. doi: 10.1177/159101991301900207
25. Ko JK, Cha SH, Lee TH, Choi CH, Lee SW, Lee JI. Endovascular treatment of aneurysms arising from the proximal segment of the anterior cerebral artery. J Korean Neurosurg Soc. (2013) 54:75–80. doi: 10.3340/jkns.2013.54.2.75
26. Cho YD, Ahn JH, Jung SC, Kim CH, Kang HS, Kim JE, et al. Coil embolization in precommunicating (A1) segment aneurysms of anterior cerebral artery. Neuroradiology. (2014) 56:219–25. doi: 10.1007/s00234-014-1319-1
27. Ahn JH, Cho YD, Kang HS, Kim JE, Cho WS, Jung SC, et al. Endovascular treatment of ophthalmic artery aneurysms: assessing balloon test occlusion and preservation of vision in coil embolization. AJNR. (2014) 35:2146–52. doi: 10.3174/ajnr.A3999
28. Cho YD, Rhim JK, Park JJ, Jeon JS, Yoo RE, Kang HS, et al. Microcatheter looping to facilitate aneurysm selection in coil embolization of paraclinoid aneurysms. Korean J Radiol. (2015) 16:899–905. doi: 10.3348/kjr.2015.16.4.899
29. Shiwei D, Jian C, Yang L, Liyong S, Guilin L, Hongqi Z. Application of multiple shaping microcatheter in coil embolization for ruptured posterior communicating artery aneurysms associated with embryonic type of posterior cerebral artery. J Interv Radiol. (2018) 27:495–9.
30. Chung Y, Ryu J, Kim EJ, Lee SH, Choi SK. Geometric classification of paraclinoid aneurysms for microcatheter superselection in coil embolization. Turk Neurosurg. (2020) 30:651–7. doi: 10.5137/1019-5149.JTN.27371-19.2
31. Ohshima T, Miyachi S, Matsuo N, Kawaguchi R, Maejima R, Takayasu M, et al. novel approach involving a microcatheter tip during aneurysmal coil embolization: the Γ (gamma) tip method. Interv Neuroradiol. (2019) 25:681–4. doi: 10.1177/1591019919850849
32. Kwak HS, Park JS, Koh EJ. Stent-assisted coil embolization on down-the-barrel view with spring-shaped microcatheter in patient with M1 ultrawide necked circumferential aneurysm. J Neurointerv Surg. (2018) 10:e13. doi: 10.1136/neurintsurg-2017-013597.rep
33. Ohshima T, Kawaguchi R, Maejima R, Matsuo N, Miyachi S. A novel technique for microcatheter additional shaping during intracranial aneurysmal coil embolization: microcatheter shaping cast. Asian J Neurosurg. (2019) 14:759–61. doi: 10.4103/ajns.AJNS_130_19
34. Yamaguchi S, Ito O, Koyanagi Y, Iwaki K, Matsukado K. Microcatheter shaping using intravascular placement during intracranial aneurysm coiling. Interv Neuroradiol. (2017) 23:249–54. doi: 10.1177/1591019917689926
35. Namba K, Higaki A, Kaneko N, Nemoto S, Kawai K. Precision microcatheter shaping in vertebrobasilar aneurysm coiling. Interv Neuroradiol. (2019) 25:423–9. doi: 10.1177/1591019918824012
36. Shinoda N, Mori M, Tamura S, Korosue K, Kose S, Kohmura E. Three-dimensional shaping technique for coil placement using the steam-shaped microcatheter for ruptured blood blister-like aneurysm. Neurochirurgie. (2018) 64:216–8. doi: 10.1016/j.neuchi.2018.04.006
37. Hanaoka Y, Koyama JI, Yamazaki D, Miyaoka Y, Fujii Y, Nakamura T, et al. Editors' choice initial experience with in vivo “endovascular shaping” technique that utilizes the vascular configuration for small cerebral aneurysm coiling. Nagoya J Med Sci. (2020) 82:355–62. doi: 10.18999/nagjms.82.2.355
38. Spottiswoode BS, van den Heever DJ, Chang Y, Engelhardt S, Du Plessis S, Nicolls F, et al. Preoperative three-dimensional model creation of magnetic resonance brain images as a tool to assist neurosurgical planning. Stereotact Funct Neurosurg. (2013) 91:162–9. doi: 10.1159/000345264
39. Kono K, Shintani A, Okada H, Terada T. Preoperative simulations of endovascular treatment for a cerebral aneurysm using a patient-specific vascular silicone model. Neurol Med Chir. (2013) 53:347–51. doi: 10.2176/nmc.53.347
40. Namba K, Higaki A, Kaneko N, Mashiko T, Nemoto S, Watanabe E. Microcatheter shaping for intracranial aneurysm coiling using the 3-dimensional printing rapid prototyping technology: preliminary result in the first 10 consecutive cases. World Neurosurg. (2015) 84:178–86. doi: 10.1016/j.wneu.2015.03.006
41. Zeng Y, Li X, Zhang J, Zhou S, Lin Z. Shaping and application of microcatheters based on 3D-printed hollow aneurysm model: a pilot feasibility study. Clin Neurol Neurosurg. (2022) 218:107277. doi: 10.1016/j.clineuro.2022.107277
42. Xu C, Wang B, Han JY, Li ZZ, Shi HZ, Li ZF. The application of 3D printing-assisted microcatheter shaping in the embolization of intracranial aneurysms. J Interv Radiol. (2017) 26:1–5.
43. Quan L, Yanzhao L, Dongfeng D. Application of profile 3D printing assisted microcatheter shaping in interventional embolization of intracranial aneurysms. Chin J Neurotrauma Surg. (2019) 5:284–8.
44. Nakajima S, Sakamoto M, Yoshioka H, Uno T, Kurosaki M. A new method of microcatheter heat-forming for cerebral aneurysmal coiling using stereolithography three-dimensional printed hollow vessel models. Yonago Acta Med. (2021) 64:113–9. doi: 10.33160/yam.2021.02.001
45. Zhu W, Li W, Tian Z, Zhang Y, Wang K, Zhang Y, et al. Stability assessment of intracranial aneurysms using machine learning based on clinical and morphological features. Transl Stroke Res. (2020) 11:1287–95. doi: 10.1007/s12975-020-00811-2
46. Turhon M, Li M, Kang H, Huang J, Zhang F, Zhang Y, et al. Development and validation of a deep learning model for prediction of intracranial aneurysm rupture risk based on multi-omics factor. Eur Radiol. (2023) 26:1–12. doi: 10.1007/s00330-023-09672-3
47. Feng J, Zeng R, Geng Y, Chen Q, Zheng Q, Yu F, et al. Automatic differentiation of ruptured and unruptured intracranial aneurysms on computed tomography angiography based on deep learning and radiomics. Insights Imaging. (2023) 14:76. doi: 10.1186/s13244-023-01423-8
48. Song L, Ni W, Wang W, Yang G, Li J, Feng X, et al. A novel intelligent microcatheter-shaping method for embolization of intracranial aneurysm. J Neurorestoratol. (2021) 9:196–207. doi: 10.26599/JNR.2021.9040022
49. Yang H, Ni W, Xu L, Geng J, He X, Ba H, et al. Computer-assisted microcatheter shaping for intracranial aneurysm embolization: evaluation of safety and efficacy in a multicenter randomized controlled trial. J Neurointerv Surg. (2023). doi: 10.1136/jnis-2023-020104
50. Wu ZB, Zeng Y, Zhang HQ, Shu K, Li GH, Xiang JP, et al. Virtual simulation with AneuShape™ software for microcatheter shaping in intracranial aneurysm coiling: a validation study. Front Neurol. (2023) 14:1095266. doi: 10.3389/fneur.2023.1095266
51. Gangqin Xu KZ, Dongyang C, Bowen Y, Tongyuan Z, Jiangyu X, Tianxiao L. Application of microcatheter shaping technique based on CFD simulation of cerebral blood flow lines in interventional treatment of internal carotid-posterior communicating artery aneurysms. J Interv Radiol. (2022) 31:651–4.
52. Inaba Y, Arai Y, Sone M, Aramaki T, Osuga K, Tanaka H, et al. Clinical trial for development of a steerable microcatheter. MITAT Off J Soc Minimally Inv Ther. (2019) 28:1–5. doi: 10.1080/13645706.2018.1467458
53. von Hessling A, Reyes Del Castillo T, Lehmann L, Roos JE, Karwacki G. Applications of the Columbus steerable guidewire. J Neurointerv Surg. (2022) 14:1045. doi: 10.1136/neurintsurg-2021-018120
54. Qiao Y, Zhang YJ, Tsappidi S, Mehta TI, Hui FK. Initial clinical experience with the Bendit steerable articulating tip microcatheter for intracranial access and intervention. Interv Neuroradiol J Perither Neuroradiol Surg Proc Neurosci. (2023) 14:15910199231193931. doi: 10.1177/15910199231193931
55. Berenstein A, Chapot R, Orion D, Barr JD, Cabiri O, Killer-Oberpfalzer M. Clinical and simulated experiences with the bendit articulating/steerable microcatheter in endovascular treatment: 2-dimensional operative video. Operative Neurosurg. (2023) 25:e36–e7. doi: 10.1227/ons.0000000000000679
Keywords: endovascular, intracranial aneurysm, microcatheter shaping, techniques, embolization
Citation: Liu C, Wu X, Hu X, Wu L, Guo K, Zhou S and Fang B (2023) Navigating complexity: a comprehensive review of microcatheter shaping techniques in endovascular aneurysm embolization. Front. Neurol. 14:1245817. doi: 10.3389/fneur.2023.1245817
Received: 23 June 2023; Accepted: 26 September 2023;
Published: 19 October 2023.
Edited by:
Kaustubh Limaye, Indiana University Bloomington, United StatesReviewed by:
Philipp Gruber, Aarau Cantonal Hospital, SwitzerlandVincent Nguyen, University of Southern California, United States
Copyright © 2023 Liu, Wu, Hu, Wu, Guo, Zhou and Fang. This is an open-access article distributed under the terms of the Creative Commons Attribution License (CC BY). The use, distribution or reproduction in other forums is permitted, provided the original author(s) and the copyright owner(s) are credited and that the original publication in this journal is cited, in accordance with accepted academic practice. No use, distribution or reproduction is permitted which does not comply with these terms.
*Correspondence: Shuang Zhou, emhvdXNodWFuZzgwMDgmI3gwMDA0MDsxNjMuY29t; Bangjiang Fang, ZmFuZ2JqaSYjeDAwMDQwOzE2My5jb20=
†These authors have contributed equally to this work and share first authorship
‡These authors have contributed equally to this work and share last authorship