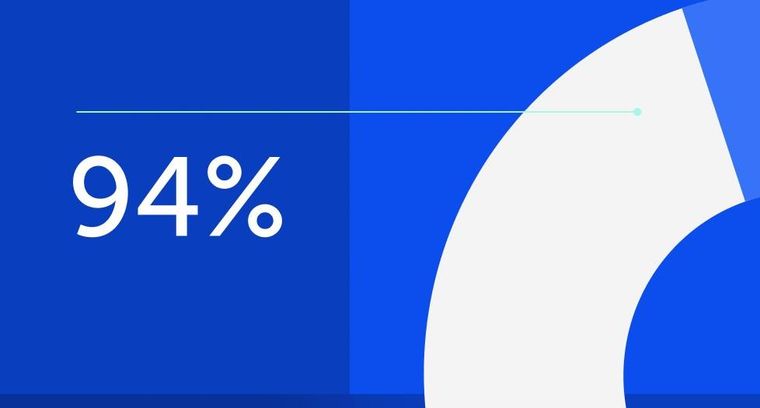
94% of researchers rate our articles as excellent or good
Learn more about the work of our research integrity team to safeguard the quality of each article we publish.
Find out more
HYPOTHESIS AND THEORY article
Front. Neurol., 02 November 2023
Sec. Dementia and Neurodegenerative Diseases
Volume 14 - 2023 | https://doi.org/10.3389/fneur.2023.1239057
Although neurocognitive models have been proposed to explain anosognosia in Alzheimer’s disease (AD), the neural cascade responsible for its origin in the human brain remains unknown. Here, we build on a mechanistic dual-path hypothesis that brings error-monitoring and emotional processing systems as key elements for self-awareness, with distinct impacts on the emergence of anosognosia in AD. Proceeding from the notion of anosognosia as a dimensional syndrome, varying between a lack of concern about one’s own deficits (i.e., anosodiaphoria) and a complete lack of awareness of deficits, our hypothesis states that (i) unawareness of deficits would result from primary damage to the error-monitoring system, whereas (ii) anosodiaphoria would more likely result from an imbalance between emotional processing and error-monitoring. In the first case, a synaptic failure in the error-monitoring system, in which the anterior and posterior cingulate cortices play a major role, would have a negative impact on error (or deficits) awareness, preventing patients from becoming aware of their condition. In the second case, an impairment in the emotional processing system, in which the amygdala and the orbitofrontal cortex play a major role, would prevent patients from monitoring the internal milieu for relevant errors (or deficits) and assigning appropriate value to them, thus biasing their impact on the error-monitoring system. Our hypothesis stems on two scientific premises. One comes from preliminary results in AD patients showing a synaptic failure in the error-monitoring system along with a decline of awareness for cognitive difficulties at the time of diagnosis. Another comes from the somatic marker hypothesis, which proposes that emotional signals are critical to adaptive behavior. Further exploration of these premises will be of great interest to illuminate the foundations of self-awareness and improve our knowledge of the underlying paths of anosognosia in AD and other brain disorders.
Anosognosia – a term derived from the Greek: “a,” absence; “nosos,” disease; “gnosis,” knowledge – was introduced more than a century ago by the French neurologist Joseph Babinski to describe the lack of awareness of a motor deficit resulting from right hemisphere damage (1). Since then, accumulating evidence has shown that anosognosia may affect any type of brain deficits or loss of function (2–4). For instance, it is now well established that unawareness for memory deficits is frequent from the early stages of Alzheimer’s disease (AD) (5), with evidence that it may have a predictive value for worsening of cognition over the disease course (6–8). Moreover, anosognosia typically delays AD diagnosis and causes resistance to treatment and rehabilitation efforts (9, 10), increasing the burden of care (11).
Interestingly, sometimes patients appear aware of their deficits in explicit verbal reports, but show a lack of emotional concern about the deficits and act inappropriately given their condition. This behavior, called anosodiaphoria, has been related to anosognosia in a putative continuum that suggests a dimensional rather than a categorical syndrome, ranging from the lack of concern for the deficits (with implicit unawareness, probably depending on a pre-conscious mechanism) to the complete lack of awareness with explicit denial of the deficits (that is, an explicit unawareness) (12). Note, however, that AD patients may also have preserved emotional reactivity to failure (implicit awareness) despite reduced awareness of performance (explicit unawareness) (13). This double dissociation between implicit and explicit awareness suggests instead a bidirectional unawareness continuum, with two poles, each following its own path, which constitutes the strongest pillar of our dual-path hypothesis. Like anosognosia, anosodiaphoria seems to increase with AD progression (12). Importantly, apathy – a motivational disorder characterized by loss of initiative and lack of emotional reactivity – has been associated with unawareness in AD, but unlike depression, greater apathy seems to correlate with higher levels of anosognosia (4, 14, 15). Additionally, an association between unawareness of deficits and executive dysfunctions in AD has been observed (16–18). More recently, using different neuroimaging techniques, researchers have found metabolic changes, namely reduced glucose metabolism in the posterior cingulate cortex (PCC) and the hippocampus (19), and/or functional disconnections within cortical midline structures involved in self-referential processes, including the medial orbitofrontal cortex (OFC) and the PCC, as well as disconnections between these regions and the medial temporal lobe in prodromal AD patients with anosognosia for memory deficits (20). In other cognitive domains unrelated to memory, anosognosia of deficits has been associated with structural lesions in the anterior cingulate cortex (ACC) (21), a brain region also involved in self-referential processing (22). Nevertheless, though the generation of new hypotheses explaining anosognosia has progressed in recent decades, the neural mechanistic cascade responsible for the emergence of anosognosia in the human brain remains largely unknown.
The Cognitive Awareness Model (CAM) (23), probably the most influential model of anosognosia in AD, is based on a modular framework that accounts for multiple levels at which unawareness phenomena can be generated, including sensory levels and differentiated levels within a hierarchy of memory consolidation processes. Specifically, the CAM predicts that various dissociations can be found in the relationship between awareness and memory (2), which would result in three main types of anosognosia: (1) Mnemonic anosognosia, reflecting a deficit in the consolidation of new information in the personal data base, which is at the origin of the metaphorical “Petrified Self” concept (24, 25); (2) Executive anosognosia, reflecting an impairment in the mechanism that normally allows for comparison between the actual performance and the stored past information; and (3) Primary anosognosia, reflecting the inability of patients to update their knowledge on their own cognitive functioning due to a dysfunction in the metacognitive awareness system (MAS). Specifically, the MAS is anchored in a comparator system that operates in tandem with a personal data base containing semantic representations of the self. Later research integrated the role of emotional processes in the CAM (26), building upon earlier work (27). For more information on this model, see also Tagai et al. (28) and Lenzoni et al. (25).
Our hypothesis derives from a distinct perspective: the core of our rationale is that anosognosia in AD, rather than being modular or domain-specific, would emerge from a critical breakdown in the system responsible for error detection and awareness, not necessarily tied to declarative memory processes (as in the case of the MAS). These errors could be committed in the context of any type of deficits, depending on their level of impairment. Specifically, we predict that AD patients with anosognosia would have a synaptic failure in the error-monitoring system, while AD patients without anosognosia would have this system still intact or, at least, able to compensate for a possible dysfunction through a “more firing, less wiring” mechanism (29) as part of a synaptic plasticity process (30, 31). In particular, this concept refers to the ability of synapses to modify their structure and/or function after persistent electrical activity, which seems the primary mechanism for learning and memory formation (32). Moreover, synaptic failure, resulting from alterations in intrinsic molecular mechanisms or from changes in biochemical reactions in the surrounding neural environment, is a common feature of several neurological and psychiatric disorders (33). Notably, it is one of the earliest pathological events to occur in AD (34–36), even before neuronal loss (37). This is of utmost relevance because it might be at the origin of cognitive deficits (38) and behavioral phenomena, like anosognosia (5), which characterize the prodromal stage of this devastating disorder. As such, cognitive event-related potentials (ERPs) may constitute a valuable biomarker for studying these clinical manifestations (39).
Hence, anosognosia would emerge from a necessary synaptic failure in the error-monitoring system, affecting patients’ awareness for any type of deficits, according to their proneness to produce errors in the concerned domains, as a consequence of (1) direct or (2) indirect damage to that system, as detailed below.
The dual-path hypothesis predicts that the lack of awareness, with explicit denial of deficits (i.e., anosognosia, or explicit unawareness; first case) would result from direct damage to the error-monitoring system; whereas the lack of concern of deficits (i.e., anosodiaphoria, or implicit unawareness; second case) would more likely result from a disturbance in the emotional processing system, with indirect impact on error-monitoring. These (1) direct and/or (2) indirect paths would be at the origin of a neural mechanistic cascade leading to a critical synaptic failure in the error-monitoring system, and would eventually result in the inability of subjects to perceive (explicitly and/or implicitly) their own deficits in any domain (i.e., cognitive, behavioral, functional, etc.).
Specifically, our mechanistic predictions are the following:
In the first case, a failure in the error-monitoring system would have a direct impact on error (or deficit) awareness, thus preventing patients from becoming aware of their condition. Such a failure would reflect local damage to the cingulate cortex, with particular focus on its anterior (ACC) and posterior (PCC) parts, which are the brain generators of ERPs associated with error monitoring, namely the error-related negativity (ERN) for preconscious error detection, and the positivity error (Pe) for error awareness (40) (Figure 1A). It is worth noting that, in AD, the interaction of oligomeric amyloid-β or misfolded tau protein with cell surface receptors might lead to changes in membrane/ion channel activity from its very early stages. This, in turn, could trigger a deterioration in synaptic structure and/or function with negative impact on network connectivity and information processing (47, 48). Remarkably, there is evidence of amyloid-β accumulation (49) and reduced number of synapses (50) in the PCC from the earliest stages of this neurodegenerative disorder. Yet, even in the case of a failure in the error-monitoring system, a preserved emotional processing system would assure some implicit awareness, with possible benefits on implicit learning and behavioral adjustment. In line with this view, recent evidence has found some implicit recognition of difficulties in AD patients despite their inability to explicitly estimate their own cognitive functioning (51).
In the second case, an impairment in the emotional processing system, in which the amygdala (Amy) and OFC play a major role (52, 53), would have an indirect impact on error-monitoring by rendering patients unable to detect relevant errors (or deficits) in the internal milieu, and to assign appropriate value to them. More precisely, such an impairment would bias the impact of deficits on the error-monitoring system, thus possibly affecting the generation of ERPs, and particularly the ERN, a preconscious error detection biomarker, whose amplitude appears modulated by motivational and emotional factors (54). Patients would therefore suffer from an implicit rather than explicit unawareness, being able to identify their own deficits though unable to understand their consequences and adapt their behavior. As such, localized impairment to the Amy and the OFC, along with potential structural and functional disconnections between these regions and critical nodes of the error-monitoring system, could underlie this implicit unawareness. In particular, these disconnections could result from (i) impairments in the integrity of two critical white matter pathways — the uncinate fasciculus (54, 55), which connects the OFC (a central hub for multisensory integration and the generation of somatic markers based on secondary emotions, especially in its medial part and in the adjacent ventromedial prefrontal cortex) to the Amy (which generates somatic markers from primary emotions, particularly involved in emotional arousal processes); and the cingulate bundle (56), an intricate network containing both lengthy fibers linking the cingulate cortex (encompassing the ACC and PCC, integral to error-monitoring); and/or (ii) a functional imbalance within and between two resting state brain networks, namely the Salience Network (SN) (55), anchored in the ACC, the Amy and other limbic structures, critically involved in the detection and response to relevant stimuli, and the Default Mode Network (DMN) (56), whose brain core is the PCC (57), principally involved in self-referential processes (58) (Figure 1B). Remarkably, the bases of impaired self-awareness and anosognosia have been closely linked to DMN functioning in AD (59, 60). In addition to the PCC, other DMN regions include the precuneus, medial prefrontal cortex, and bilateral temporoparietal junction (61). Interestingly, structural-functional connectivity impairments between the precuneus and the PCC, but also beyond the cingulate cortex, seem to play a central role in modeling AD as well as other neurodegenerative and psychiatric disorders (62). We further hypothesize that these disruptions may in part, albeit indirectly, impact the error-monitoring system.
A third prediction, following a synergistic A plus B mechanism (Figures 1A,B), would result in the most severe case of anosognosia, affecting both explicit and implicit awareness, with pronounced consequences, up to and including a full inability of patients to learn from their errors (or deficits) and to adapt their behavior.
Figure 1. Schematic representation of the Dual-path hypothesis for the emergence of anosognosia in AD. (A, Direct path) Synaptic failure in the error-monitoring system, due to direct damage to the ACC and/or the PCC, the brain generators of the ERN and the Pe (40), with negative impact on explicit awareness. Note, reduced metabolism has been reported in the PCC from the early stages of AD (41), with further evidence of accentuated hypometabolism in AD patients whose the onset of symptoms was before 65 years old (42). (B, Indirect path) Structural and/or functional disconnections between the emotional processing and the error-monitoring systems, with negative impact on implicit awareness. The schematic diagram illustrates the cingulate fibers that travel along the anterior–posterior axis of the cingulum bundle (in blue) to reach various brain regions, including the medial temporal lobe, the Amy, and the OFC (43). The Amy and OFC are further interconnected via the uncinate fasciculus (in red) (44). Note, these two major white matter tracts have been shown to be impaired in the early stage of Alzheimer’s disease (45). Further illustrated are the ACC and the Amy, which constitute key brain areas of the SN; and the PCC, which constitutes a key region of the DMN [see Menon (46) for literature review on large-scale brain networks in psychopathology, highlighting potential parallels across neurological and psychiatric disorders].
Our hypothesis stems on two scientific premises: the one comes from our preliminary results on ERPs showing a synaptic failure in the error-monitoring system of AD patients at the time of their diagnosis (63). In particular, by studying ERPs elicited by errors during a word memory recognition task in two groups of cognitively normal amyloid-positive individuals at baseline, we have recently shown direct evidence of an error-monitoring failure, along with a cognitive awareness decline, only in subjects who progressed to AD during the five-year study period. Specifically, we measured the ERN, mainly related to error detection, and the Pe, mainly related to error awareness, in 51 amyloid positive individuals who presented only subjective memory complaints at study entry, while they performed a word memory recognition task. Of these, 15 individuals progressed to AD within the five-year study period (PROG group), and 36 remained cognitively normal (CTRL group). We observed opposite longitudinal effects for the PROG and CTRL groups. Notably, we found a reduction of the Pe amplitude for the PROG group over time, in contrast with the increase of the Pe amplitude for the CTRL group. Moreover, contrary to the CTRL group, subjects who progressed to AD showed a cognitive awareness decline, with signs of anosognosia for their cognitive deficits at the moment of AD diagnosis (63). Importantly, there is evidence that error-monitoring impairments in AD patients, and consequent unawareness of errors (including errors committed as a consequence of memory recognition deficits), are not merely a byproduct of their typical memory impairment (64).
The second premise comes from the “somatic marker hypothesis,” proposed by Damasio (65, 66). In fact, our rationale converges to some extent with that hypothesis, which states that one must “feel” the consequences of one’s own actions, assigning them an affective value, in order to make the right decisions. In particular, Damasio proposes that decision-making (like self-awareness in the case of our dual-path hypothesis) requires the interplay between two specific brain systems: the executive and the emotional processing systems, in which the OFC (67) and particularly the amygdala (68) are necessary for triggering somatic states (53). Specifically, Damasio argues that autonomic reactions, such as electrodermal responses (EDR; see Sequeira et al. (69) for literature review on electrical autonomic correlates of emotion) to stimuli, might prepare the subject to adapt attentionally and physically to changes in the environment (66). Other researchers have also pointed to an important role for emotional dysregulation in producing unawareness, as errors may require an affective signature to motivate self-monitoring (27). Supporting evidence exists for severe AD pathology in autonomic-related cortices, such as the OFC, which suggests that it could contribute to the emotional and autonomic dysregulations that often accompany this neurodegenerative disorder (70).
In line with these premises, anosognosia of memory deficits has been associated with either hypoperfusion or hypometabolism in the PCC (7, 20, 71), the ACC (71), and the OFC (20, 71). Congruently, additional research has shown reduced within- and between-network connectivity in the DMN in AD patients with anosognosia (60, 72), with a pertinent association between hypometabolism in this network and an increased risk of progression to dementia in anosognosic patients (73).
Interestingly, evidence from another study found an association between memory monitoring and motor monitoring in AD patients, but observed that anosognosia for memory deficits was associated only with memory monitoring, not motor monitoring (74). The authors interpreted their results within a hierarchical model of awareness, suggesting that local self-monitoring processes (based on domain specific monitors) were associated across different domains, but only contributed to overall levels of awareness in a domain-specific manner. We interpret their results in a new light. Our rationale proposes that anosognosia is not domain-specific and can occur in any domain that is conducive to error. In early stages of AD, with a typical amnestic presentation, a dysfunction in error-monitoring would largely concern memory deficits, rather than other less affected or even unaffected functions, explaining why overall unawareness (or anosognosia for memory deficits as assessed through an offline, clinical interview method in that study) would be related to a failure in memory monitoring, but not to motor monitoring. Or, to put it another way, we postulate that if there were no errors, there would be no anosognosia. Therefore, a failure in the error-monitoring system would explain why anosognosia tends to worsen during the course of AD, following the level of impairment (and, consequently, the probability of making errors) in various cognitive, behavioral and functional domains, beyond memory.
A new line of research is thus needed to explore the dual-path hypothesis for the emergence of anosognosia. We aim at investigating this hypothesis by studying a group of AD patients, at distinct stages of the disease, presenting different levels of anosognosia, versus a group of healthy elderly controls, while performing a computer-based error-monitoring task. To characterize our study population, we will use a comprehensive neuropsychological battery, including several cognitive tests to assess global cognition, memory, executive and instrumental functions, as well as both online performance discrepancy measures (based on our computerized task) and the offline Healthy Aging Brain Care Monitor questionnaire to detect anosognosia in distinct cognitive, psychological/behavioral and functional domains (63, 75, 76). In addition, standard scales will be used to evaluate depression, anxiety and apathy, which is highly relevant because these neuropsychiatric conditions can have an impact on the amplitude of our electrophysiological biomarkers of interest (54, 77, 78), and so must be taken into account in the statistical models. Specifically, we will focus our research on the study of erroneous responses and their possible correlations with the level of anosognosia and both central (i.e., the ERN and the Pe) and peripheral (i.e., the EDR) biomarkers of error awareness and emotional arousal, measured simultaneously during the computer-based task. Because of their extremely high temporal resolution, cognitive ERPs, such as ERN and Pe, are a valuable, non-invasive tool for assessment of synaptic dysfunction (39, 79). Also, we will investigate to what extent the level of anosognosia can be correlated with the amplitudes of these electrophysiological biomarkers, as well as with our hypothesis’ main regions (as illustrated in Figure 1: the PCC, the ACC, the OFC and the Amy), which have been associated with neural mechanisms of autonomic, emotional, and cognitive integration (67, 68, 80), and their interconnections, through structural and functional neuroimaging methods.
While new hypotheses have been put forward over the last decades to explain anosognosia, there is still no evidence of a neural mechanistic understanding of this phenomenon. Here, we hypothesize that anosognosia might emerge from a critical synaptic failure in the error-monitoring system, thus preventing patients from detecting (explicitly and/or implicitly) their own errors (or deficits). This failure could result from either (i) direct damage to the error-monitoring system (i.e., Direct path, Figure 1A) or from (ii) the lack of emotional feedback on errors arriving to that system (i.e., Indirect path, Figure 1B), reflecting, in this case, local impairment in key structures of the emotional processing system and/or a disconnection between this system and the error-monitoring system.
We have focused our hypothesis on AD as a pathological model, but our rationale implies that it can be applied to any other brain condition in which anosognosia occurs. Indeed, anosognosia is prone to main deficits, according to their level of impairment, in several brain disorders. As it is well known, in chronic, progressive disorders, like AD, anosognosia tends to worsen over the course of the disease. We interpret this association in a particular way. We propose that anosognosia probably follows the appearance of errors committed in the context of a given deficit, such as deficits in episodic memory and the consequent forgetfulness (that is, the error), which generally occur from the early stages in AD patients. Since errors would become more frequent as the disease progresses, the level of anosognosia would correspondingly increase. On the contrary, in non-progressive disorders such as acute stroke, anosognosia is often a transient phenomenon, probably benefiting from a cascade of mechanisms leading to synaptic plasticity as frequently observed within the first hours after the onset of cerebral ischemia (81). To provide a few examples, anosognosia is mostly related to episodic memory deficits in the early stages of AD, but it can also affect other deficits following the severity of the disease (82); as it is also mostly related to personality changes in the behavioral variant of frontotemporal dementia (83); or to hemiplegia, particularly in the acute phase after a right-hemisphere stroke (84); etc. Altogether, this strongly suggests that a common inability to monitor errors (committed in the context of a given deficit), more than a specific memory consolidation impairment typical of AD, may be at the origin of this intriguing syndrome. Importantly, this would hold true regardless of the type of deficits (cognitive, behavioral, motor, etc.) or the neurological condition (neurodegenerative disorder, stroke, etc.).
For instance, in the context of stroke, there is evidence of action-monitoring deficits in patients with anosognosia for hemiplegia (AHP) (85, 86). Interestingly, these action-monitoring deficits seem to relate to monitoring deficits in distinct cognitive domains (87), thus supporting the existence of an error-monitoring impairment in stroke patients with AHP. Moreover, these patients appear unable to monitor self-performed actions, while able to monitor others’ actions or their own actions as if they were a third person (88), which seems to indicate impairment in their self-referential systems. As clearly established, the DMN – and the PCC, as a central node of this network – play a primordial role in self-referential processes, with additional evidence indicating that damage to brain white matter tracts involved in these processes may foster the appearance of anosognosia in stroke patients (89).
A growing body of evidence has shown that synaptic failure is a common pathological finding in several brain conditions, including neurodevelopmental and neurodegenerative diseases (33), as well as ischemic cerebral damage. Interestingly, although not focused on anosognosia, further evidence has suggested that prolonged synaptic failure may be a cause of persistent symptoms in patients with cerebral ischemia (90). Moving research from correlation to understanding the mechanistic causation is crucial for the development of successful therapies in the neurological and psychiatric fields. ERPs primarily reflect synaptic transmission processes, and may thus provide sensitive biomarkers to improve our knowledge on the neural substrates and mechanisms underlying brain disorders and their clinical manifestations.
Finally, elucidating the neural mechanistic cascade leading to anosognosia in AD may have two major clinical and scientific outcomes: first, contribute to a deeper understanding of the pathophysiology of this neurodegenerative disorder; second, refine current models of anosognosia with the goal of improving rehabilitation strategies allowing anosognosic patients to adhere to healthcare measures, which could maintain their autonomy for longer and reduce the burden of care. Very important, the rationale of our hypothesis extends beyond AD. To validate it, novel research is required not only in Alzheimer’s patients, but also in other neurological (and even psychiatric) populations, in which anosognosia has been frequently reported. Such a line of research can still illuminate the theoretical foundations of human self-awareness.
The original contributions presented in the study are included in the article/supplementary material, further inquiries can be directed to the corresponding author.
KA: conception of the hypothesis, manuscript writing (first draft and final version). DP: manuscript review and editing, design of the “hypothesis figure,” conceptualized with KA, with further contributions of TG and TM. All authors: discussion of arguments in support of the hypothesis.
This work was supported by a grant from the ANR (Agence Nationale de la Recherche): NOT_AWARE: ANR-17-CE37-0017-01 (to KA) and the National Institute on Aging of the National Institutes of Health under award numbers RF1AG074204 and RF1AG079324 (to DP).
The authors declare that the research was conducted in the absence of any commercial or financial relationships that could be construed as a potential conflict of interest.
All claims expressed in this article are solely those of the authors and do not necessarily represent those of their affiliated organizations, or those of the publisher, the editors and the reviewers. Any product that may be evaluated in this article, or claim that may be made by its manufacturer, is not guaranteed or endorsed by the publisher.
1. Langer, KG, Levine, DN, and Babinski, J. Contribution to the study of the mental disorders in hemiplegia of organic cerebral origin (Anosognosia). Translated by KG Langer & DN Levine: translated from the original contribution à l’Étude des troubles Mentaux dans l'Hémiplégie Organique Cérébrale (Anosognosie). Cortex. (1914) 61:5–8. doi: 10.1016/j.cortex.2014.04.019
2. Mograbi, DC, and Morris, RG. Anosognosia. Cortex. (2018) 103:385–6. doi: 10.1016/j.cortex.2018.04.001
3. McGlynn, SM, and Schacter, DL. Unawareness of deficits in neuropsychological syndromes. J Clin Exp Neuropsychol. (1989) 11:143–205. doi: 10.1080/01688638908400882
4. Starkstein, SE. Anosognosia in Alzheimer’s disease: diagnosis, frequency, mechanism and clinical correlates. Cortex. (2014) 61:64–73. doi: 10.1016/j.cortex.2014.07.019
5. Bastin, C, Giacomelli, F, Miévis, F, Lemaire, C, Guillaume, B, and Salmon, E. Anosognosia in mild cognitive impairment: lack of awareness of memory difficulties characterizes prodromal Alzheimer’s disease. Front Psych. (2021) 12:631518. doi: 10.3389/fpsyt.2021.631518
6. Vannini, P, Amariglio, R, Hanseeuw, B, Johnson, KA, McLaren, DG, Chhatwal, J, et al. Memory self-awareness in the preclinical and prodromal stages of Alzheimer’s disease. Neuropsychologia. (2017) 99:343–9. doi: 10.1016/j.neuropsychologia.2017.04.002
7. Gerretsen, P, Chung, JK, Shah, P, Plitman, E, Iwata, Y, Caravaggio, F, et al. Alzheimer’s Disease Neuroimaging Initiative. Anosognosia is an independent predictor of conversion from mild cognitive impairment to Alzheimer’s disease and is associated with reduced brain metabolism. J Clin Psychiatry. (2017) 78:e1187–96. doi: 10.4088/JCP.16m11367
8. Starkstein, SE, Jorge, R, Mizrahi, R, and Robinson, RG. A diagnostic formulation for anosognosia in Alzheimer’s disease. J Neurol Neurosurg Psychiatry. (2006) 77:719–25. doi: 10.1136/jnnp.2005.085373
9. Cosentino, S, Metcalfe, J, Cary, MS, De Leon, J, and Karlawish, J. Memory awareness influences everyday decision making capacity about medication management in Alzheimer’s disease. Int J Alzheimers Dis. (2011) 2011:483897. doi: 10.4061/2011/483897
10. Clare, L. Awareness in early-stage Alzheimer’s disease: a review of methods and evidence. Br J Clin Psychol. (2004) 43:177–96. doi: 10.1348/014466504323088042
11. Turró-Garriga, O, Garre-Olmo, J, Vilalta-Franch, J, Conde-Sala, JL, de Gracia, BM, and López-Pousa, S. Burden associated with the presence of anosognosia in Alzheimer’s disease. Int J Geriatr Psychiatry. (2013) 28:291–7. doi: 10.1002/gps.3824
12. Lindau, M, and Bjork, R. Anosognosia and anosodiaphoria in mild cognitive impairment and Alzheimer’s disease. Dement Geriatr Cogn Dis Extra. (2014) 4:465–80. doi: 10.1159/000369132
13. Mograbi, DC, Brown, RG, Salas, C, and Morris, RG. Emotional reactivity and awareness of task performance in Alzheimer’s disease. Neuropsychologia. (2012) 50:2075–84. doi: 10.1016/j.neuropsychologia.2012.05.008
14. Azocar, I, Livingston, G, and Huntley, J. The association between impaired awareness and depression, anxiety, and apathy in mild to moderate Alzheimer’s disease: a systematic review. Front Psych. (2021) 12:633081. doi: 10.3389/fpsyt.2021.633081
15. Mograbi, DC, and Morris, RG. On the relation among mood, apathy, and anosognosia in Alzheimer’s disease. J Int Neuropsychol Soc. (2014) 20:2–7. doi: 10.1017/S1355617713001276
16. Amanzio, M, Vase, L, Leotta, D, Miceli, R, Palermo, S, and Geminiani, G. Impaired awareness of deficits in Alzheimer’s disease: the role of everyday executive dysfunction. J Int Neuropsychol Soc. (2013) 19:63–72. doi: 10.1017/S1355617712000896
17. Amanzio, M, Torta, DME, Sacco, K, Cauda, F, D’Agata, F, Duca, S, et al. Unawareness of deficits in Alzheimer’s disease: role of the cingulate cortex. Brain. (2011) 134:1061–76. doi: 10.1093/brain/awr020
18. Michon, A, Deweer, B, Pillon, B, Agid, Y, and Dubois, B. Relation of anosognosia to frontal lobe dysfunction in Alzheimer’s disease. J Neurol Neurosurg Psychiatry. (1994) 57:805–9. doi: 10.1136/jnnp.57.7.805
19. Vannini, P, Hanseeuw, B, Munro, CE, Amariglio, RE, Marshall, GA, Rentz, DM, et al. Anosognosia for memory deficits in mild cognitive impairment: Insight into the neural mechanism using functional and molecular imaging. Neuroimage Clin. (2017) 15:408–14. doi: 10.1016/j.nicl.2017.05.020
20. Perrotin, A, Desgranges, B, Landeau, B, Mézenge, F, La Joie, R, Egret, S, et al. Anosognosia in Alzheimer disease: disconnection between memory and self-related brain networks. Ann Neurol. (2015) 78:477–86. doi: 10.1002/ana.24462
21. Northoff, G, Heinzel, A, de Greck, M, Bermpohl, F, Dobrowolny, H, and Panksepp, J. Self-referential processing in our brain--a meta-analysis of imaging studies on the self. Neuroimage. (2006) 31:440–57. doi: 10.1016/j.neuroimage.2005.12.002
22. Valera-Bermejo, JM, De Marco, M, Mitolo, M, McGeown, WJ, and Venneri, A. Neuroanatomical and cognitive correlates of domain-specific anosognosia in early Alzheimer’s disease. Cortex. (2020) 129:236–46. doi: 10.1016/j.cortex.2020.04.026
23. Agnew, SK, and Morris, RG. The heterogeneity of anosognosia for memory impairment in Alzheimer’s disease: a review of the literature and a proposed model. Aging Ment Health. (1998) 2:7–19. doi: 10.1080/13607869856876
24. Mograbi, DC, Brown, RG, and Morris, RG. Anosognosia in Alzheimer’s disease – the petrified self. Conscious Cogn. (2009) 18:989–1003. doi: 10.1016/j.concog.2009.07.005
25. Lenzoni, S, Morris, RG, and Mograbi, DC. The petrified self 10 years after: current evidence for mnemonic anosognosia. Front Psychol. (2020) 11:465. doi: 10.3389/fpsyg.2020.00465
26. Morris, RG, and Mograbi, DC. Anosognosia, autobiographical memory and self knowledge in Alzheimer’s disease. Cortex. (2013) 49:1553–65. doi: 10.1016/j.cortex.2012.09.006
27. Rosen, HJ. Anosognosia in neurodegenerative disease. Neurocase. (2011) 17:231–41. doi: 10.1080/13554794.2010.522588
28. Tagai, K, Nagata, T, Shinagawa, S, and Shigeta, M. Anosognosia in patients with Alzheimer’s disease: current perspectives. Psychogeriatrics. (2020) 20:345–52. doi: 10.1111/psyg.12507
29. Daselaar, SM, Iyengar, V, Davis, SW, Eklund, K, Hayes, SM, and Cabeza, RE. Less wiring, more firing: low-performing older adults compensate for impaired white matter with greater neural activity. Cereb Cortex. (2015) 25:983–90. doi: 10.1093/cercor/bht289
30. Sjöström, PJ, Turrigiano, GG, and Nelson, SB. Rate, timing, and cooperativity jointly determine cortical synaptic plasticity. Neuron. (2001) 32:1149–64. doi: 10.1016/S0896-6273(01)00542-6
31. Keck, T, Toyoizumi, T, Chen, L, Doiron, B, Feldman, DE, Fox, K, et al. Integrating Hebbian and homeostatic plasticity: the current state of the field and future research directions. Philos Trans R Soc Lond Ser B Biol Sci. (2017) 372:20160158. doi: 10.1098/rstb.2016.0158
32. Hebb, DO. The organization of behavior: a neuropsychological theory. New York: Psychology Press (2005). 378 p.
33. Taoufik, E, Kouroupi, G, Zygogianni, O, and Matsas, R. Synaptic dysfunction in neurodegenerative and neurodevelopmental diseases: an overview of induced pluripotent stem-cell-based disease models. Open Biol. (2018) 8:180138. doi: 10.1098/rsob.180138
34. Terry, RD, Masliah, E, Salmon, DP, Butters, N, DeTeresa, R, Hill, R, et al. Physical basis of cognitive alterations in Alzheimer’s disease: synapse loss is the major correlate of cognitive impairment. Ann Neurol. (1991) 30:572–80. doi: 10.1002/ana.410300410
35. Selkoe, DJ. Alzheimer’s disease is a synaptic failure. Science. (2002) 298:789–91. doi: 10.1126/science.1074069
36. Chen, Y, Fu, AKY, and Ip, NY. Synaptic dysfunction in Alzheimer’s disease: mechanisms and therapeutic strategies. Pharmacol Ther. (2019) 195:186–98. doi: 10.1016/j.pharmthera.2018.11.006
37. Wishart, TM, Parson, SH, and Gillingwater, TH. Synaptic vulnerability in neurodegenerative disease. J Neuropathol Exp Neurol. (2006) 65:733–9. doi: 10.1097/01.jnen.0000228202.35163.c4
38. Pelucchi, S, Gardoni, F, Di Luca, M, and Marcello, E. Synaptic dysfunction in early phases of Alzheimer’s disease. Handb Clin Neurol. (2022) 184:417–38. doi: 10.1016/B978-0-12-819410-2.00022-9
39. Olichney, JM, Yang, J-C, Taylor, J, and Kutas, M. Cognitive event-related potentials: biomarkers of synaptic dysfunction across the stages of Alzheimer’s disease. J Alzheimers Dis. (2011) 26:215–28. doi: 10.3233/JAD-2011-0047
40. O’Connell, RG, Dockree, PM, Bellgrove, MA, Kelly, SP, Hester, R, Garavan, H, et al. The role of cingulate cortex in the detection of errors with and without awareness: a high-density electrical mapping study. Eur J Neurosci. (2007) 25:2571–9. doi: 10.1111/j.1460-9568.2007.05477.x
41. Nestor, PJ, Fryer, TD, Smielewski, P, and Hodges, JR. Limbic hypometabolism in Alzheimer’s disease and mild cognitive impairment. Ann Neurol. (2003) 54:343–51. doi: 10.1002/ana.10669
42. Rabinovici, GD, Furst, AJ, Alkalay, A, Racine, CA, O’Neil, JP, Janabi, M, et al. Increased metabolic vulnerability in early-onset Alzheimer’s disease is not related to amyloid burden. Brain. (2010) 133:512–28. doi: 10.1093/brain/awp326
43. Bubb, EJ, Metzler-Baddeley, C, and Aggleton, JP. The cingulum bundle: anatomy, function, and dysfunction. Neurosci Biobehav Rev. (2018) 92:104–27. doi: 10.1016/j.neubiorev.2018.05.008
44. Thiebaut de Schotten, M, Dell’Acqua, F, Valabregue, R, and Catani, M. Monkey to human comparative anatomy of the frontal lobe association tracts. Cortex. (2012) 48:82–96. doi: 10.1016/j.cortex.2011.10.001
45. Kiuchi, K, Morikawa, M, Taoka, T, Nagashima, T, Yamauchi, T, Makinodan, M, et al. Abnormalities of the uncinate fasciculus and posterior cingulate fasciculus in mild cognitive impairment and early Alzheimer’s disease: a diffusion tensor tractography study. Brain Res. (2009) 1287:184–91. doi: 10.1016/j.brainres.2009.06.052
46. Menon, V. Large-scale brain networks and psychopathology: a unifying triple network model. Trends Cogn Sci. (2011) 15:483–506. doi: 10.1016/j.tics.2011.08.003
47. Gulisano, W, Maugeri, D, Baltrons, MA, Fà, M, Amato, A, Palmeri, A, et al. Role of amyloid-β and tau proteins in Alzheimer’s disease: confuting the amyloid cascade. J Alzheimers Dis. (2018) 64:S611–31. doi: 10.3233/JAD-179935
48. Budak, M, and Zochowski, M. Synaptic failure differentially affects pattern formation in heterogenous networks. Front Neural Circuits. (2019) 13:31. doi: 10.3389/fncir.2019.00031
49. Collij, LE, Heeman, F, Salvadó, G, Ingala, S, Altomare, D, de Wilde, A, et al. Multitracer model for staging cortical amyloid deposition using PET imaging. Neurology. (2020) 95:e1538–53. doi: 10.1212/WNL.0000000000010256
50. Scheff, SW, Price, DA, Ansari, MA, Roberts, KN, Schmitt, FA, Ikonomovic, MD, et al. Synaptic change in the posterior cingulate gyrus in the progression of Alzheimer’s disease. J Alzheimers Dis. (2015) 43:1073–90. doi: 10.3233/JAD-141518
51. Geurten, M, Salmon, E, and Bastin, C. Impaired explicit self-awareness but preserved behavioral regulation in patients with Alzheimer disease. Aging Ment Health. (2021) 25:142–8. doi: 10.1080/13607863.2019.1675142
52. Rolls, ET, Deco, G, Huang, C-C, and Feng, J. Human amygdala compared to orbitofrontal cortex connectivity, and emotion. Prog Neurobiol. (2023) 220:102385. doi: 10.1016/j.pneurobio.2022.102385
53. Šimić, G, Tkalčić, M, Vukić, V, Mulc, D, Španić, E, Šagud, M, et al. Understanding emotions: origins and roles of the amygdala. Biomol Ther. (2021) 11:823. doi: 10.3390/biom11060823
54. Hajcak, G, McDonald, N, and Simons, RF. Error-related psychophysiology and negative affect. Brain Cogn. (2004) 56:189–97. doi: 10.1016/j.bandc.2003.11.001
55. Seeley, WW, Menon, V, Schatzberg, AF, Keller, J, Glover, GH, Kenna, H, et al. Dissociable intrinsic connectivity networks for salience processing and executive control. J Neurosci. (2007) 27:2349–56. doi: 10.1523/JNEUROSCI.5587-06.2007
56. Raichle, ME, MacLeod, AM, Snyder, AZ, Powers, WJ, Gusnard, DA, and Shulman, GL. A default mode of brain function. Proc Natl Acad Sci U S A. (2001) 98:676–82. doi: 10.1073/pnas.98.2.676
57. Leech, R, Kamourieh, S, Beckmann, CF, and Sharp, DJ. Fractionating the default mode network: distinct contributions of the ventral and dorsal posterior cingulate cortex to cognitive control. J Neurosci. (2011) 31:3217–24. doi: 10.1523/JNEUROSCI.5626-10.2011
58. Jilka, SR, Scott, G, Ham, T, Pickering, A, Bonnelle, V, Braga, RM, et al. Damage to the salience network and interactions with the default mode network. J Neurosci. (2014) 34:10798–807. doi: 10.1523/JNEUROSCI.0518-14.2014
59. Antoine, N, Bahri, MA, Bastin, C, Collette, F, Phillips, C, Balteau, E, et al. Anosognosia and default mode subnetwork dysfunction in Alzheimer’s disease. Hum Brain Mapp. (2019) 40:5330–40. doi: 10.1002/hbm.24775
60. Mondragón, JD, Maurits, NM, and Deyn, D. Functional neural correlates of anosognosia in mild cognitive impairment and Alzheimer’s disease: a systematic review. Neuropsychol Rev. (2019) 29:139–65. doi: 10.1007/s11065-019-09410-x
61. Utevsky, AV, Smith, DV, and Huettel, SA. Precuneus is a functional core of the default-mode network. J Neurosci. (2014) 34:932–40. doi: 10.1523/JNEUROSCI.4227-13.2014
62. Dadario, NB, and Sughrue, ME. The functional role of the precuneus. Brain. (2023) 146:3598–607. doi: 10.1093/brain/awad181
63. Razafimahatratra, S, Guieysse, T, Lejeune, F-X, Houot, M, Medani, T, Dreyfus, G, et al. Can a failure in the error-monitoring system explain unawareness of memory deficits in Alzheimer’s disease? Cortex. (2023) 166:428–40. doi: 10.1016/j.cortex.2023.05.014
64. Dodson, CS, Spaniol, M, O’Connor, MK, Deason, RG, Ally, BA, and Budson, AE. Alzheimer’s disease and memory-monitoring impairment: Alzheimer’s patients show a monitoring deficit that is greater than their accuracy deficit. Neuropsychologia. (2011) 49:2609–18. doi: 10.1016/j.neuropsychologia.2011.05.008
65. Damasio, A. (1994). Descartes’ error: emotion, reason, and the human brain. New York: GP Putnam’s Sons.
66. Damasio, AR. The somatic marker hypothesis and the possible functions of the prefrontal cortex. Philos Trans R Soc Lond Ser B Biol Sci. (1996) 351:1413–20. doi: 10.1098/rstb.1996.0125
67. Bechara, A, Damasio, H, and Damasio, AR. Emotion, decision making and the orbitofrontal cortex. Cereb Cortex. (2000) 10:295–307. doi: 10.1093/cercor/10.3.295
68. Bechara, A, Damasio, H, and Damasio, AR. Role of the amygdala in decision-making. Ann N Y Acad Sci. (2003) 985:356–69. doi: 10.1111/j.1749-6632.2003.tb07094.x
69. Sequeira, H, Hot, P, Silvert, L, and Delplanque, S. Electrical autonomic correlates of emotion. Int J Psychophysiol. (2009) 71:50–6. doi: 10.1016/j.ijpsycho.2008.07.009
70. Chu, CC, Tranel, D, Damasio, AR, and Van Hoesen, GW. The autonomic-related cortex: pathology in Alzheimer’s disease. Cereb Cortex. (1997) 7:86–95. doi: 10.1093/cercor/7.1.86
71. Hanyu, H, Sato, T, Akai, T, Shimizu, S, Hirao, K, Kanetaka, H, et al. Neuroanatomical correlates of unawareness of memory deficits in early Alzheimer’s disease. Dement Geriatr Cogn Disord. (2008) 25:347–53. doi: 10.1159/000119594
72. Mondragón, JD, Maurits, NM, and Deyn, D. Functional connectivity differences in Alzheimer’s disease and amnestic mild cognitive impairment associated with AT(N) classification and anosognosia. Neurobiol Aging. (2021) 101:22–39. doi: 10.1016/j.neurobiolaging.2020.12.021
73. Therriault, J, Ng, KP, Pascoal, TA, Mathotaarachchi, S, Kang, MS, Struyfs, H, et al. Anosognosia predicts default mode network hypometabolism and clinical progression to dementia. Neurology. (2018) 90:e932–9. doi: 10.1212/WNL.0000000000005120
74. Chapman, S, Colvin, LE, Vuorre, M, Cocchini, G, Metcalfe, J, Huey, ED, et al. Cross domain self-monitoring in anosognosia for memory loss in Alzheimer’s disease. Cortex. (2018) 101:221–33. doi: 10.1016/j.cortex.2018.01.019
75. Monahan, P, Alder, C, Khan, B, Stump, T, and Boustani, M. The healthy aging brain care (HABC) monitor: validation of the patient self-report version of the clinical tool designed to measure and monitor cognitive, functional, and psychological health. Clin Interv Aging. (2014):2123. doi: 10.2147/CIA.S64140
76. Guieysse, T, Lamothe, R, Houot, M, Razafimahatratra, S, Medani, T, Lejeune, F-X, et al. Detecting anosognosia from the prodromal stage of Alzheimer’s disease. J Alzheimers Dis. (2023) 95:1723–33. doi: 10.3233/JAD-230552
77. Andersson, S, and Finset, A. Electrodermal responsiveness and negative symptoms in brain injured patients. J Psychophysiol. (1999) 13:109–16. doi: 10.1027//0269-8803.13.2.109
78. Holmes, AJ, and Pizzagalli, DA. Spatiotemporal dynamics of error processing dysfunctions in major depressive disorder. Arch Gen Psychiatry. (2008) 65:179–88. doi: 10.1001/archgenpsychiatry.2007.19
79. Horvath, A, Szucs, A, Csukly, G, Sakovics, A, Stefanics, G, and Kamondi, A. EEG and ERP biomarkers of Alzheimer’s disease: a critical review. Front Biosci. (2018) 23:183–220. doi: 10.2741/4587
80. Critchley, HD. Neural mechanisms of autonomic, affective, and cognitive integration. J Comp Neurol. (2005) 493:154–66. doi: 10.1002/cne.20749
81. Grefkes, C, and Fink, GR. Recovery from stroke: current concepts and future perspectives. Neurol Res Pract. (2020) 2:17. doi: 10.1186/s42466-020-00060-6
82. Leicht, H, Berwig, M, and Gertz, H-J. Anosognosia in Alzheimer’s disease: the role of impairment levels in assessment of insight across domains. J Int Neuropsychol Soc. (2010) 16:463–73. doi: 10.1017/S1355617710000056
83. Rankin, KP, Baldwin, E, Pace-Savitsky, C, Kramer, JH, and Miller, BL. Self awareness and personality change in dementia. J Neurol Neurosurg Psychiatry. (2005) 76:632–9. doi: 10.1136/jnnp.2004.042879
84. Orfei, MD, Caltagirone, C, and Spalletta, G. The evaluation of anosognosia in stroke patients. Cerebrovasc Dis. (2009) 27:280–9. doi: 10.1159/000199466
85. Saj, A, Vocat, R, and Vuilleumier, P. Action-monitoring impairment in anosognosia for hemiplegia. Cortex. (2014) 61:93–106. doi: 10.1016/j.cortex.2014.10.017
86. Vocat, R, Saj, A, and Vuilleumier, P. The riddle of anosognosia: does unawareness of hemiplegia involve a failure to update beliefs? Cortex. (2013) 49:1771–81. doi: 10.1016/j.cortex.2012.10.009
87. Jenkinson, PM, Edelstyn, NMJ, Drakeford, JL, and Ellis, SJ. Reality monitoring in anosognosia for hemiplegia. Conscious Cogn. (2009) 18:458–70. doi: 10.1016/j.concog.2008.12.005
88. Fotopoulou, A, Rudd, A, Holmes, P, and Kopelman, M. Self-observation reinstates motor awareness in anosognosia for hemiplegia. Neuropsychologia. (2009) 47:1256–60. doi: 10.1016/j.neuropsychologia.2009.01.018
89. Pacella, V, Foulon, C, Jenkinson, PM, Scandola, M, Bertagnoli, S, Avesani, R, et al. Anosognosia for hemiplegia as a tripartite disconnection syndrome. Elife. (2019) 8:e46075. doi: 10.7554/eLife.46075
Keywords: anosognosia, Alzheimer’s disease, error-monitoring system, emotional processing, neural mechanism, synaptic failure, error-related potentials, self-awareness
Citation: Andrade K, Guieysse T, Medani T, Koechlin E, Pantazis D and Dubois B (2023) The dual-path hypothesis for the emergence of anosognosia in Alzheimer’s disease. Front. Neurol. 14:1239057. doi: 10.3389/fneur.2023.1239057
Received: 12 June 2023; Accepted: 20 October 2023;
Published: 02 November 2023.
Edited by:
Madepalli Krishnappa Lakshmana, Florida International University, United StatesReviewed by:
Jessica J. Zakrzewski, University of California, San Diego, United StatesCopyright © 2023 Andrade, Guieysse, Medani, Koechlin, Pantazis and Dubois. This is an open-access article distributed under the terms of the Creative Commons Attribution License (CC BY). The use, distribution or reproduction in other forums is permitted, provided the original author(s) and the copyright owner(s) are credited and that the original publication in this journal is cited, in accordance with accepted academic practice. No use, distribution or reproduction is permitted which does not comply with these terms.
*Correspondence: Katia Andrade, a2F0aWEuc2FudG9zYW5kcmFkZUBnbWFpbC5jb20=
Disclaimer: All claims expressed in this article are solely those of the authors and do not necessarily represent those of their affiliated organizations, or those of the publisher, the editors and the reviewers. Any product that may be evaluated in this article or claim that may be made by its manufacturer is not guaranteed or endorsed by the publisher.
Research integrity at Frontiers
Learn more about the work of our research integrity team to safeguard the quality of each article we publish.