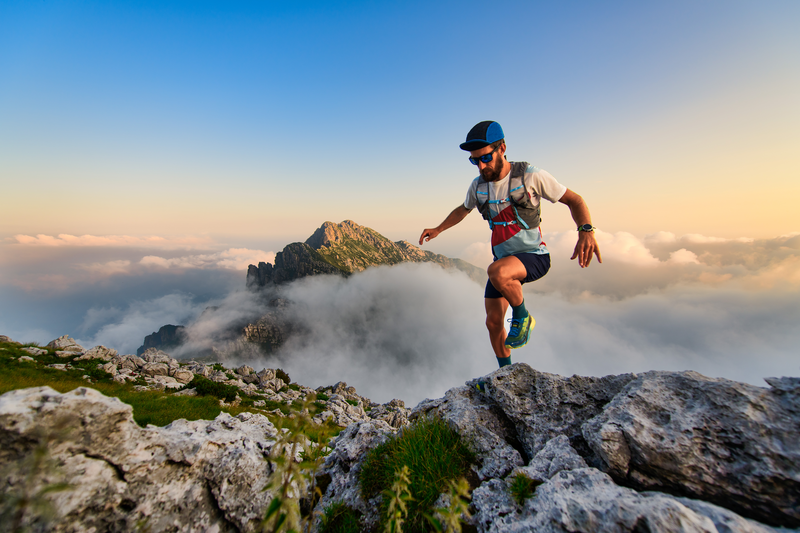
94% of researchers rate our articles as excellent or good
Learn more about the work of our research integrity team to safeguard the quality of each article we publish.
Find out more
HYPOTHESIS AND THEORY article
Front. Neurol. , 03 August 2023
Sec. Neurorehabilitation
Volume 14 - 2023 | https://doi.org/10.3389/fneur.2023.1225924
This article is part of the Research Topic Reviews in Neurorehabilitation View all 17 articles
Background: Stroke results in impairment of motor function of both the upper and lower limbs. However, although it is debatable, motor function of the lower limb is believed to recover faster than that of the upper limb. The aim of this paper is to propose some hypotheses to explain the reasons for that, and discuss their implications for research and practice.
Method: We searched PubMED, Web of Science, Scopus, Embase and CENTRAL using the key words, stroke, cerebrovascular accident, upper extremity, lower extremity, and motor recovery for relevant literature.
Result: The search generated a total of 2,551 hits. However, out of this number, 51 duplicates were removed. Following review of the relevant literature, we proposed four hypotheses: natural instinct for walking hypothesis, bipedal locomotion hypothesis, central pattern generators (CPGs) hypothesis and role of spasticity hypothesis on the subject matter.
Conclusion: We opine that, what may eventually account for the difference, is the frequency of use of the affected limb or intensity of the rehabilitation intervention. This is because, from the above hypotheses, the lower limb seems to be used more frequently. When limbs are used frequently, this will result in use-dependent plasticity and eventual recovery. Thus, rehabilitation techniques that involve high repetitive tasks practice such as robotic rehabilitation, Wii gaming and constraint induced movement therapy should be used during upper limb rehabilitation.
• There is reported difference in the rate of recovery of motor function between upper and lower limbs following stroke. The latter is believed to recover faster than the former.
• One of the reasons attributed to this is that, the cortical homunculus of the upper limb is larger in size due to its higher tactile sensitivity.
• We also proposed natural instinct for walking, bipedal locomotion, central pattern generators hypotheses to further help explain the reasons for the difference.
• However, most importantly, the difference could a factor of intensity or frequency of use of the lower limb compared to the upper limb, and spasticity.
• Therefore, interventions for upper limb motor function should consider increasing the intensity and effective management of spasticity.
Stroke causes impairment in motor, sensory and cognitive functions. For the motor function, its impairment results in disability in carrying out activities of daily living (ADL), which can negatively affect the patient’s quality of life (1–4). Thus, for stroke survivours to regain the ability to carry out ADL such as feeding, bathing, wearing clothes, grooming and picking up the telephone to answer calls, recovery of upper limb motor function is needed (5). Similarly, recovery of lower limb motor function is essential for walking which is required for ADLs such as transfer from one place to another, going for shopping and participating in social and other activities (6). In addition, recovery of motor function, independence in carrying out ADL, and the ability to participate in social and other activities are important in achieving good quality of life (7, 8). Therefore, the importance of upper and lower limb motor function recovery cannot be overemphasized.
However, to date, the rate of recovery of upper and lower limb motor function following stroke is a subject of debate that requires the attention of clinician scientists and researchers. For instance, for a very long time, it has been suggested that, the difference is due mainly to the size of the areas representing the limbs in the cortical homunculus. The area representing the upper limb is larger than that of the lower limb (9, 10); and as such, it was suggested that, its recovery may take a longer time following stroke. Although this could be a possible explanation for the difference, a more recent evidence has however not shown any significant correlation between lesion volume or size and motor function (11); suggesting that, other factors may be responsible for the difference in the rate of recovery between the two.
In addition, although, some researchers opined that, there is essentially no difference in the rate of recovery between the two (12, 13); yet, some studies reported lower limb to recover faster than the upper limb (14–22). However, the fast recovery of the lower limb compared to the upper limb, has been observed to be in a subpopulation of patients with anterior circulation infarct (14). Anterior circulation supplies brain areas that are mainly responsible for the motor and sensory functions of the lower limb, and speech production (23). Moreover, it is noteworthy in the study by Paci and colleagues that, all the participants included in the study received rehabilitation (14). During rehabilitation, it was observed that more attention is usually given to the lower limb compared to the upper limb (24). Thus, allocating attention to the limb may result in intensive practice during rehabilitation, which is important for use-dependent plasticity and recovery (25). Therefore, this could be another reason for the difference.
Another reason for the variation could be the type of stroke. This is because, ischemic type of stroke generally shows better functional outcomes compared to the hemorrhagic type (26). This is because, hemorrhagic type of stroke is associated with complications such as expansion of hematoma, increased blood pressure, venous thrombotic events and perihematomal oedema with increased intracranial pressure that can cause further damage to brain cells (27). In addition, other factors such as severity of the impairment and age may be the possible explanation for the difference in rate of recovery (28–30). Furthermore, pattern or rate of recovery that is observed following stroke largely depends on the type of outcome measures used to determine the recovery. The neurophysiological measures of recovery such as the transmagnetic stimulation (TMS), are generally more sensitive than the behavioral measures such as the Fugl-Meyer motor assessment (31). Unfortunately, most studies used the behavioral measures to assess recovery following stroke (15–22).
However, considering that all the above arguments may not be exhaustive on the subject matter, there seems to be many other factors which require further investigation that have not yet been considered in the debate on the variation in the rate of recovery of motor function between the upper and lower limbs following stroke (30). The aim of this paper is to propose several hypotheses for the possible difference in the rate of recovery of motor function between upper and lower limbs following stroke, and their implications for research and practice.
For this purpose, five databases, PubMED, Web of Science, Scopus, Embase and CENTRAL were searched from their inceptions to February, 2023 using the key words, stroke, cerebrovascular accident, upper extremity, lower extremity and motor recovery for relevant literature. The search generated a total of 2,551 hits. However, out of this number, 51 duplicates were removed using Endnote software. Thereafter, relevant articles on recovery of motor function were read, and based on our understanding of the reviewed literature, experience and knowledge of the subject matter, we proposed 4 hypotheses: natural instinct for walking hypothesis, bipedal locomotion hypothesis, central pattern generators (CPGs) hypothesis, and role of spasticity hypothesis on the subject matter to help explain why the difference exists. See Table 1 for the summary of the articles guiding the proposed hypotheses.
Humans seem to have a natural instinct for wanting to walk no matter what. This can be seen even early in life, where stepping/walking reflex, which is the placement of one foot in front of the other when the soles of feet touch ground, is present at birth (32). Although this reflex disappears at age 6 weeks, it voluntarily reappears at age 8–12 months (32). In addition, humans consider walking as a means to an end; and as such they walk to carry out their ADL such as going for shopping, and participating in social and leisure activities (40).
Moreover, historically, it is believed that, “humans made multiple journeys on foot out of Africa to the Eurasian landmass, and dispersing eventually to the Americas and Asia-Pacific region” (41–43). This seems to suggest that, importance of the lower limbs for all human endeavors is as old as the humans themselves. Consequently, in the event of an injury to the nervous system such as after stroke, the natural instinct of the patient is to want to recover walking ability as soon as possible, to help achieve independence in carrying out ADL as much as possible (44, 45). This is probably because, recovery of lower limb motor function significantly influences health-related quality of life (46). Interestingly, early mobilization following stroke results in early recovery (33). In addition, repetitive steps that are taken during walking can help induce recovery of lower limb motor function through use-dependent plasticity (47, 48).
See Figure 1 for the mechanism of the natural instinct for walking hypothesis.
Human locomotion is bipedal, which involves three subtasks, propulsion, limb advancement and body weight support (34). As such, following stroke, the less affected or sound lower limb can be used during propulsion to help force the use of the affected limb (35, 49–51). Forced use of limb following stroke helps with reversing learned non-use, and promoting recovery (49, 52). In addition, bearing weight on the affected limb that generate proprioceptive information in the foot, can serve as important sources of sensory outputs for recovery (53). Consequently, bearing weight on the affected limb helps with the recovery of walking speed and functional mobility (52, 53).
See Figure 2 for the mechanism of the bipedal locomotion hypothesis.
Walking in humans is mainly produced by the combined roles of the reflex circuit, which produces motor patterns triggered by sensory feedback, and the central pattern generators (CPGs), which is a network of neurons capable of generating rhythmic pattern movements even in the absence of command from the higher motor centers (36, 37, 54–57). The CPGs innervate mainly the muscles of the lower limb (54); and they may not be affected following stroke. In addition, the neurons that orchestrates walking reside predominantly in the lumbar spine (58, 59). Consequently, rythmic pattern movement such as stepping during walking can be generated even in the absence of control of the higher centers. Evidence of rhythmic-locomotor activity in the lower limb was seen following epidural stimulation of the spinal cord (60).
See Figure 3 for the mechanism of the central pattern generators hypothesis.
About 25% of patients with stroke develops spasticity, although it depends on the severity of the paresis (61). However, prevalence of the spasticity and its severity, are higher in the upper limb than in the lower limb (38). Presence of severe spasticity in the upper limb, correlates with poor hand dexterity (39). In addition, unlike the lower limb, spasticity in the upper limb is associated with 60, 100, and 33% cases of shoulder pain, elbow pain and wrist pain, respectively, (62). Presence of pain is a significant predictor of poor recovery of function, ability to carry out ADL and quality of life following stroke (63, 64). In contrast, presence of spasticity may not substantially affect functional recovery of the lower limb (65).
In addition, functional specialization of the upper and lower limbs differs. The upper limb is involved in the performance of complex fine motor movement (66). However, as noted earlier, spasticity in the upper limb is significantly associated with poor dexterity, a requirement for fine motor movement ability (39, 65). Moreover, spasticity is associated with decreased joint proprioception (67). Acuity of proprioception in the wrist joint is linked to the control of fine movement (66). Thus, this may be the reason why even in the presence of motor and functional recovery, use of the upper limb in daily activities, which is also an indicator of recovery, may not be easily achieved (68).
Recovery of motor function following stroke has been considered to depend on so many factors such as the size and location of the lesion, and time since stroke (28–30). Similarly, although it is still debatable, the recovery is considered faster in the lower than the upper limb (14–22). However, following review of the literature, we hereby proposed some hypotheses to help explain other possible reasons why the lower limb may recover faster than the upper limb, and discussed the implications of the hypotheses for research and practice. The hypotheses are natural instinct for walking, bipedal locomotion, central pattern generators and role of spasticity hypotheses.
Following stroke, natural instinct for walking, which will result in motor activity with the affected limb; bipedal locomotion, in which weight is borne on the affected limb, while the unaffected limb is used to propel the affected one; role of CPGs in producing rhythmic movement patterns such as the steps needed during walking; and the role of spasticity in impairing movement, suggest that, the lower limb may recover faster than the upper limb because it is used more than the latter in activities. This is because inadequate amount of activity as may often be the case with upper limb compared to the lower limb, may not be able to drive neural reorganization that is required for recovery (69). Interestingly, walking is an ADL, and use of the limb for daily activities in the real world, is a significant predictor of recovery of motor function following stroke (70).
The above argument seems to suggest that, use-dependent plasticity may be the reason for faster recovery of motor function in the lower limb compared to the upper limb. Thus, increasing activity or intensity of practice during upper limb rehabilitation is important to help optimize recovery, by inducing biochemical, physiological and anatomical changes in the brain (71–74). Increasing the intensity of practice of the affected upper limb can be achieved through the use of technology driven rehabilitation interventions such as the Wii gaming and robotic rehabilitation (75, 76). In addition, techniques such as the constraint induced movement therapy, which comprises of massed tasks practice with the affected limb, constraint of the unaffected limb, and transfer package (a contract to ensure continuous use of the affected at home) should be considered (77–79). Already, it is known that, repetitive tasks practice of the upper limb results in greater recovery (80–82); and this will in turn result in increased use of the limb in the real world (83).
In addition, the larger muscles of the lower limb are very important in maintaining standing posture (84). Thus, because of patients’ natural instinct for wanting to regain walking, they would have to be able to stand first before they can walk. In doing so, bearing weight on the two limbs will automatically stimulate the stretch reflex, which will in turn activate the motor cortex (85, 86). When the motor cortex is repeatedly activated, recovery of motor function ensues (87). In addition, even during walking, weight is continuously borne on the lower limbs which helps with the restoration of motor function through the mechanisms already mentioned above. This is because, control of gait and posture are intricately related (86). Moreover, due to the bipedal nature of human locomotion, the unaffected limb forces the affected one into activity during propulsion and limb advancement. Thus, this can result in use-dependent plasticity, and eventual recovery of the lower limb (49).
Similarly, the role the CPGs play in the generation of rhythmic movement pattern such as the steps required for walking, may aid with the faster recovery of the lower limb (37, 54). Thus, considering the roles play by bipedal locomotion in humans, where the unaffected limb forces the affected limb into activity during propulsion and limb advancement; and the potential role of the CPGs in lower limb recovery, use of rhythmic bilateral movement training and bilateral upper limb exercise may help promote recovery of upper limb motor function through use-dependent plasticity (88, 89). Furthermore, as noted earlier, presence of spasticity in the upper limb is associated with poor recovery outcomes (39). Thus, this seems to suggest that, presence of spasticity may account for the difference in the rate of recovery between the upper limb and the lower limb. As such, managing spasticity in the upper limb during early post stroke may help hasten its recovery. Consequently, effective interventions for spasticity in patients with stroke such as active exercises, joint positioning and joint stretching should be used (90).
Although, the 4 theories proposed in this paper tried to explain some of the reasons why the lower limb motor function recovers faster than that of the upper limb, they are not in any way exhaustive, and as such other factors should also be considered. One of these factors is the argument that, upper limb occupies a larger area in the motor homunculus due its high tactile sensitivity, compared to the lower limb (9). Thus, to help recruit more areas of the brain to aid with the recovery of upper limb motor function, sensorimotor stimulation techniques such as the brain and peripheral electrical stimulation and tactile stimulation can be used in combination with other interventions (91–93). Stimulation of the nervous system can result in recovery of the upper limb (91, 94).
Secondly, the timing of rehabilitation is also important. This is because early post stroke is the period when the potential for recovery is higher (8, 95, 96). In addition, it is important also to note that, ability to determine or predict recovery depends on the outcome measure used (97). Furthermore, the difference sometimes may also depend on the psychometric properties of the outcome measures used (20). For instance, most studies use measures of daily function or disability rather than measures of impairment (20). Thus, in determining and predicting recovery of motor function after stroke, a combination of clinical, neurophysiological and imaging outcome measures should be used (20, 98, 99). Moreover, research is needed to be carried out, where practice/ activity will be controlled between upper and lower limbs, to determine if one will recover faster than the other. Similarly, studies should compare patients with the same degree of spasticity in the upper and the lower limbs to determine which one recovers faster.
The lower limb may regain motor function following stroke at a rate faster than the upper limb. Although many factors can help explain the reason why, most importantly the reason majorly has to do with the intensity or frequency or dose of use of the lower limb compared to the upper limb, and presence of spasticity and its significant impact on the upper limb. Therefore, rehabilitation strategies for upper limb motor function following stroke should consider increasing the intensity of practice especially in the real world, and management of spasticity, especially during early post stroke.
In our opinion, the hypotheses we presented are some of the factors that make the lower limb to recover its motor function faster than the upper limb; and that all of them seem to suggest that, the main factor for the difference is intensity of use of the lower limb compared to the upper limb. However, these factors we hypothesized seem not to be yet thoroughly investigated, and as such, future studies should focus on investigating them. For instance, views or opinions of stroke survivours using qualitative research methodology should be collected to explore what they prefer to recover immediately after having a stroke. In addition, ethnography method of qualitative research, whereby a group’s behavior is observed by the researcher without interfering with their behavior, can be used to observe stroke survivours through their recovery journey. That way, the researchers can document the journeys of recovery of upper and lower limbs motor function with the goal of observing which one of them recovers faster.
Similarly, observational studies using objective outcome measures of motor function (physical function) such as the Fugl Meyer motor assessment and Wolf motor function test (WMFT) can also be used to objectively determine the difference over a long period of at least 1 year. In addition, electrophysiological measures of motor function such as the electromyography (EMG) to measure muscle electrical activity, and functional magnetic resonance imaging (fMRI) to measure cortical activity should also be used to determine the difference. Furthermore, biomechanical measurements of aspects of motor function such as movement speed, smoothness, quality and directness should also be considered. Thus, in determining the difference in recovery of motor function between the upper and lower limbs, a combination of outcomes measures of physical function, electrophysiological function, biomechanics, perspectives or views of patients and the caregivers and participants observation should be used to help with more reliable comparison. Moreover, many variables such the participants’ age, sex, time since stroke, side affected, lesion volume, type of stroke, presence of neglect, and handedness before stroke need to be controlled in the studies.
In addition, in practice, clinicians should consider methods and techniques that will help increase the intensity of practice with the upper limb. For instance, transfer package whereby a contract is designed between the clinicians, the patients and their caregivers to make patients practice with the affected limb more in the real world, particularly at home; and home programs to increase the intensity of practice can be used. Furthermore, self-management techniques such as the use of motivational interviewing that will help increase patients’ self-efficacy to enable them practice more with the affected limb should be incorporated in upper limb rehabilitation. Similarly, use of mechanical and computer devices such as the AUTOCITE (automated constraint induced movement extension), Wii games and other robotic devices that can help guarantee increased intensity of practice should also be considered during upper limb rehabilitation. However, the challenges that researchers and clinicians may face in determining whether upper limb or lower limb will recover faster in patients include the role of spontaneous recovery, patients own personal motivation and effort, caregiver support and probably the clinical setting.
The original contributions presented in the study are included in the article/supplementary material, further inquiries can be directed to the corresponding author.
AA, TW, and SN: conception and design, revising it critically for intellectual content, and the final approval of the version to be published. AA: drafting of the paper. All authors contributed to the article and approved the submitted version.
This work was supported by the research funding of the Research Centre for Chinese Medicine Innovation of The Hong Kong Polytechnic University (Ref. No. P0041139) awarded to SN and her team; and PolyU Distinguished Postdoctoral Fellowship Scheme (P0035217).
The authors declare that the research was conducted in the absence of any commercial or financial relationships that could be construed as a potential conflict of interest.
All claims expressed in this article are solely those of the authors and do not necessarily represent those of their affiliated organizations, or those of the publisher, the editors and the reviewers. Any product that may be evaluated in this article, or claim that may be made by its manufacturer, is not guaranteed or endorsed by the publisher.
1. Langhorne, P, Coupar, F, and Pollock, A. Motor recovery after stroke: a systematic review. Lancet Neurol. (2009) 8:741–54. doi: 10.1016/S1474-4422(09)70150-4
2. Morris, JH, Van Wijck, F, Joice, S, and Donaghy, M. Predicting health related quality of life 6 months after stroke: the role of anxiety and upper limb dysfunction. Disabil Rehabil. (2013) 35:291–9. doi: 10.3109/09638288.2012.691942
3. Ekstrand, E, Rylander, L, Lexell, J, and Brogårdh, C. Perceived ability to perform daily hand activities after stroke and associated factors: a cross-sectional study. BMC Neurol. (2016) 16:208–9. doi: 10.1186/s12883-016-0733-x
4. Waddell, KJ, Birkenmeier, RL, Bland, MD, and Lang, CE. An exploratory analysis of the self-reported goals of individuals with chronic upper-extremity paresis following stroke. Disabil Rehabil. (2016) 38:853–7. doi: 10.3109/09638288.2015.1062926
5. Silva, ESM, Pereira, ND, Gianlorenço, ACL, and Camargo, PR. The evaluation of non-use of the upper limb in chronic hemiparesis is influenced by the level of motor impairment and difficulty of the activities-proposal of a new version of the motor activity log. Physiother Theory Pract. (2019) 35:964–74. doi: 10.1080/09593985.2018.1460430
6. Rakesh, N, Boiarsky, D, Athar, A, Hinds, S, and Stein, J. Post-stroke rehabilitation: factors predicting discharge to acute versus subacute rehabilitation facilities. Med. (2019) 98:e15934. doi: 10.1097/MD.0000000000015934
7. Wirz, M, and Dietz, V. European multicenter study of spinal cord injury (EMSCI) network. Recovery of sensorimotor function and activities of daily living after cervical spinal cord injury: the influence of age. J Neurotrauma. (2015) 32:194–9. doi: 10.1089/neu.2014.3335
8. Chanubol, R, Wongphaet, P, Ot, NC, Chira-Adisai, W, Kuptniratsaikul, P, and Jitpraphai, C. Correlation between the action research arm test and the box and block test of upper extremity function in stroke patients. J Med Assoc Thail. (2012) 95:590–7.
9. Saadon-Grosman, N, Loewenstein, Y, and Arzy, S. The 'creatures' of the human cortical somatosensory system. Brain Commun. (2020) 2:fcaa003. doi: 10.1093/braincomms/fcaa003
10. Reed, CL, and Ziat, M. Haptic perception: from the skin to the brain. In: Reference module in neuroscience and biobehavioral psychology : Neuroscience and Biobehavioral Psychology (2017). doi: 10.1016/B978-0-12-809324-5.21972-X
11. Peters, DM, Fridriksson, J, Richardson, JD, Stewart, JC, Rorden, C, Bonilha, L, et al. Upper and lower limb motor function correlates with Ipsilesional corticospinal tract and red nucleus structural integrity in chronic stroke: a cross-sectional. ROI-Based MRI Study Behav Neurol. (2021) 2021:1–10. doi: 10.1155/2021/3010555
12. Paci, M, Nannetti, L, Moretti, S, Boccaletti, E, and Lombardi, B. Are there differences in motor recovery between upper and lower limbs in hemiplegic patients with hemorrhagic stroke? Ital J Physiother. (2012) 2:59–64. doi: 10.1097/MRR.0000000000000172
13. Verheyden, G, Nieuwboer, A, De Wit, L, Thijs, V, Dobbelaere, J, and Devos, H. Time course of trunk, arm, leg, and functional recovery after ischemic stroke. Neurorehabil Neural Repair. (2018) 22:173–9. doi: 10.1177/1545968307305456
14. Paci, M, Nannetti, L, Casavola, D, and Lombardi, B. Differences in motor recovery between upper and lower limbs: does stroke subtype make the difference? Int J Rehabil Res. (2016) 39:185–7. doi: 10.1097/MRR.0000000000000172
15. Vincent-Onabajo, G, Hamzat, T, and Owolabi, M. Trajectory of motor performance over twelve months in Nigerian stroke survivors. Brain Impairment. (2014) 15:43–50. doi: 10.1017/BrImp.2014.3
16. Skurvydas, A, Juodzbaliene, V, Darbutas, T, and Brazaitis, M. One year after ischemic stroke: changes in leg movement path stability in a speed-accuracy task but no major effects on the hands. Hum Mov Sci. (2018) 57:50–8. doi: 10.1016/j.humov.2017.11.005
17. Lee, KB, Lim, SH, Kim, KH, Kim, KJ, Kim, YR, Chang, WN, et al. Six-month functional recovery of stroke patients. Int J Rehabil Res. (2015) 38:173–80. doi: 10.1097/MRR.0000000000000108
18. Kong, KH, and Lee, J. Temporal recovery and predictors of upper limb dexterity in the first year of stroke: a prospective study of patients admitted to a rehabilitation Centre. NeuroRehabil. (2013) 32:345–50. doi: 10.3233/NRE-130854
19. Rand, D, and Eng, JJ. Disparity between functional recovery and daily use of the upper and lower extremities during subacute stroke rehabilitation. Neurorehabil Neural Repair. (2016) 26:76–84. doi: 10.1177/1545968311408918
20. Desrosiers, J, Malouin, F, Richards, C, Bourbonnais, D, Rochette, A, and Bravo, G. Comparison of changes in upper and lower extremity impairments and disabilities after stroke. Int J Rehabil Res. (2013) 26:109–16. doi: 10.1097/00004356-200306000-00005
21. Hendricks, HT, van Limbeek, J, Geurts, AC, and Zwarts, MJ. Motor recovery after stroke: a systematic review of the literature. Arch Phys Med Rehabil. (2003) 83:1629–37. doi: 10.1053/apmr.2002.35473
22. Dombovy, ML . Rehabilitation and the course of recovery after stroke. In: JP Whisnant , editor. Stroke: Populations, cohorts, and clinical trials, Oxford: Butterworth-Heinemann Ltd (1993). 218–37.
23. Tan, A, and Roberts, D. Cerebral circulation 1: anatomy. BJA Educ. (2021) 21:390–5. doi: 10.1016/j.bjae.2021.05.004
24. Bernhardt, J, Chan, J, Nicola, I, and Collier, JM. Little therapy, little physical activity: rehabilitation within the first 14 days of organized stroke unit care. J Rehabil Med. (2007) 39:43–8. doi: 10.2340/16501977-0013
25. Abdullahi, A, Umar, NA, Ushotanefe, U, Aliyu, AM, Oyeniran, AM, Steven, T, et al. Effects of two different modes of tasks practice during lower limbs constraint-induced movement therapy in people with stroke: a randomized clinical trial. Neural Plast. (2021) 2021:1–9. doi: 10.1155/2021/6664058
26. Salvadori, E, Papi, G, Insalata, G, Rinnoci, V, Donnini, I, Martini, M, et al. Comparison between ischemic and hemorrhagic strokes in functional outcome at discharge from an intensive rehabilitation hospital. Diagnostics. (2020) 11:38. doi: 10.3390/diagnostics11010038
27. Balami, JS, and Buchan, AM. Complications of intracerebral haemorrhage. Lancet Neurol. (2012) 11:101–18. doi: 10.1016/S1474-4422(11)70264-2
28. Persson, HC, Opheim, A, Lundgren-Nilsson, A, Alt Murphy, M, Danielsson, A, and Sunnerhagen, KS. Upper extremity recovery after ischaemic and haemorrhagic stroke: part of the SALGOT study. Eur Stroke J. (2016) 1:310–9. doi: 10.1177/2396987316672809
29. Winters, C, van Wegen, EE, Daffertshofer, A, and Kwakkel, G. Generalizability of the proportional recovery model for the upper extremity after an ischemic stroke. Neurorehabil Neural Repair. (2015) 29:614–22. doi: 10.1177/1545968314562115
30. Ingwersen, T, Wolf, S, Birke, G, Schlemm, E, Bartling, C, Bender, G, et al. Long-term recovery of upper limb motor function and self-reported health: results from a multicenter observational study 1 year after discharge from rehabilitation. Neurol Res Pract. (2021) 3:66. doi: 10.1186/s42466-021-00164-7
31. Hendricks, HT, Pasman, JW, Merx, JL, van Limbeek, J, and Zwarts, MJ. Analysis of recovery processes after stroke by means of transcranial magnetic stimulation. J Clin Neurophysiol. (2003) 20:188–95. doi: 10.1097/00004691-200305000-00004
32. Volpe, JJ . Neurological examination: normal and abnormal features. In: Neurology of the newborn. 5th ed. Philadelphia, PA: Saunders Elsevier (2008).
33. Yen, HC, Jeng, JS, Chen, WS, Pan, GS, Chuang, PT, BS, WY, Lee, YY, et al. Early mobilization of mild-moderate intracerebral hemorrhage patients in a stroke center: a randomized controlled trial. Neurorehabil Neural Repair. (2020) 34:72–81. doi: 10.1177/1545968319893294
34. Awad, LN, Lewek, MD, Kesar, TM, Franz, JR, and Bowden, MG. These legs were made for propulsion: advancing the diagnosis and treatment of post-stroke propulsion deficits. J Neuroeng Rehabil. (2020) 17:139. doi: 10.1186/s12984-020-00747-6
35. Abdullahi, A, Truijen, S, Umar, NA, Useh, U, Egwuonwu, VA, van Criekinge, T, et al. Effects of lower limb constraint induced movement therapy in people with stroke: a systematic review and Meta-analysis. Front Neurol. (2021) 12:638904. doi: 10.3389/fneur.2021.638904
36. Ryu, HX, and Kuo, AD. An optimality principle for locomotor central pattern generators. Sci Rep. (2021) 11:13140. doi: 10.1038/s41598-021-91714-1
37. Minassian, K, Hofstoetter, US, Dzeladini, F, Guertin, PA, and Ijspeert, A. The human central pattern generator for locomotion: does it exist and contribute to walking? Neuroscientist. (2017) 23:649–63. doi: 10.1177/1073858417699790
38. Katoozian, L, Tahan, N, Zoghi, M, and Bakhshayesh, B. The onset and frequency of spasticity after first ever stroke. J Natl Med Assoc. (2018) 110:547–52. doi: 10.1016/j.jnma.2018.01.008
39. Kong, KH, Chua, KS, and Lee, J. Recovery of upper limb dexterity in patients more than 1 year after stroke: frequency, clinical correlates and predictors. NeuroRehabilitation. (2011) 28:105–11. doi: 10.3233/NRE-2011-0639
40. O'Mara, S . Biopsychosocial functions of human walking and adherence to Behaviourally demanding belief systems: a narrative review. Front Psychol. (2021) 12:654122. doi: 10.3389/fpsyg.2021.654122
41. Hershkovitz, I, Weber, GW, Quam, R, Duval, M, Grün, R, Kinsley, L, et al. The earliest modern humans outside Africa. Science. (2018) 359:456–9. doi: 10.1126/science.aap8369
42. Bae, CJ, Douka, K, and Petraglia, MD. On the origin of modern humans: Asian perspectives. Science. (2017) 358:eaai9067. doi: 10.1126/science.aai9067
43. López, S, van Dorp, L, and Hellenthal, G. Human dispersal out of Africa: a lasting debate. Evol Bioinformatics Online. (2016) 11:57–68. doi: 10.4137/EBO.S33489
44. Törnbom, K, Sunnerhagen, KS, and Danielsson, A. Perceptions of physical activity and walking in an early stage after stroke or acquired brain injury. PLoS One. (2017) 12:e0173463. doi: 10.1371/journal.pone.0173463
45. Bohannon, RW, Andrews, AW, and Smith, MB. Rehabilitation goals of patients with hemiplegia. Int J Rehabil Res. (1988) 11:181–4. doi: 10.1097/00004356-198806000-00012
46. Martino Cinnera, A, Bonnì, S, Pellicciari, MC, Giorgi, F, Caltagirone, C, and Koch, G. Health-related quality of life (HRQoL) after stroke: positive relationship between lower extremity and balance recovery. Top Stroke Rehabil. (2020) 27:534–40. doi: 10.1080/10749357.2020.1726070
47. Enzinger, C, Johansen-Berg, H, Dawes, H, Bogdanovic, M, Collett, J, Guy, C, et al. Functional MRI correlates of lower limb function in stroke victims with gait impairment. Stroke. (2008) 39:1507–13. doi: 10.1161/STROKEAHA.107.501999
48. Yang, YR, Chen, I, Liao, KK, Huang, CC, and Wang, RY. Cortical reorganization induced by body weight-supported treadmill training in patients with hemiparesis of different stroke durations. Arch Phys Med Rehabil. (2010) 91:513–8. doi: 10.1016/j.apmr.2009.11.021
49. Mahtani, GB, Kinnaird, CR, Connolly, M, Holleran, CL, Hennessy, PW, Woodward, J, et al. Altered sagittal- and frontal-plane kinematics following high-intensity stepping training versus conventional interventions in subacute stroke. Phys Ther. (2016) 97:320–9. doi: 10.2522/ptj.20160281
50. Roelker, S, Bowden, M, Kautz, S, and Neptune, RR. Paretic propulsion as a measure of walking performance and functional motor recovery post-stroke: a review. Gait Posture. (2018) 68:6–14. doi: 10.1016/j.gaitpost.2018.10.027
51. Yu, WH, Liu, WY, Wong, AM, Wang, TC, Li, YC, and Lien, HY. Effect of forced use of the lower extremity on gait performance and mobility of post-acute stroke patients. J Phys Ther Sci. (2015) 27:421–5. doi: 10.1589/jpts.27.421
52. Macleod, CA, Meng, L, Conway, BA, and Porr, B. Reflex control of robotic gait using human walking data. PLoS One. (2014) 9:e109959. doi: 10.1371/journal.pone.0109959
53. Ravichandran, H, Shetty, KS, and Janakiraman, B. Effect of gait-specific weight-bearing interventions on physical performance among subjects with stroke: a systematic review and Meta-analysis. J Stroke Med. (2022) 5:107–18. doi: 10.1177/25166085221115605
54. Klarner, T, and Zehr, EP. Sherlock Holmes and the curious case of the human locomotor central pattern generator. J Neurophysiol. (2018) 120:53–77. doi: 10.1152/jn.00554.2017
55. Grillner, S . Biological pattern generation: the cellular and computational logic of networks in motion. Neuron. (2006) 52:751–66. doi: 10.1016/j.neuron.2006.11.008
56. Yang, JF, and Gorassini, M. Spinal and brain control of human walking: implications for retraining of walking. Neuroscientist. (2006) 12:379–89. doi: 10.1177/1073858406292151
57. Kiehn, O . Locomotor circuits in the mammalian spinal cord. Annu Rev Neurosci. (2006) 29:279–306. doi: 10.1146/annurev.neuro.29.051605.112910
58. Kathe, C, Skinnider, MA, Hutson, TH, Regazzi, N, Gautier, M, Demesmaeker, R, et al. The neurons that restore walking after paralysis. Nature. (2022) 611:540–7. doi: 10.1038/s41586-022-05385-7
59. Sherrington, CS . The integrative action of the nervous system, New Heaven: Yale University Press (1906).
60. Dimitrijevic, MR, Gerasimenko, Y, and Pinter, MM. Evidence for a spinal central pattern generator in humans. Ann N Y Acad Sci. (1998) 860:360–76. doi: 10.1111/j.1749-6632.1998.tb09062.x
61. Zeng, H, Chen, J, Guo, Y, and Tan, S. Prevalence and risk factors for spasticity after stroke: a systematic review and Meta-analysis. Front Neurol. (2021) 11:616097. doi: 10.3389/fneur.2020.616097
62. Wissel, J, Schelosky, LD, Scott, J, Christe, W, Faiss, JH, and Mueller, J. Early development of spasticity following stroke: a prospective, observational trial. J Neurol. (2010) 257:1067–72. doi: 10.1007/s00415-010-5463-1
63. Payton, H, and Soundy, A. The experience of post-stroke pain and the impact on quality of life: an integrative review. Behav Sci (Basel). (2020) 10:128. doi: 10.3390/bs10080128
64. Westerlind, E, Singh, R, Persson, HC, and Sunnerhagen, KS. Experienced pain after stroke: a cross-sectional 5-year follow-up study. BMC Neurol. (2020) 20:4. doi: 10.1186/s12883-019-1584-z
65. Singer, JC, Nishihara, K, and Mochizuki, G. Does Poststroke lower-limb spasticity influence the recovery of standing balance control? A 2-year multilevel growth model. Neurorehabil Neural Repair. (2016) 30:626–34. doi: 10.1177/1545968315613862
66. Tseng, YT, Chen, FC, Tsai, CL, and Konczak, J. Upper limb proprioception and fine motor function in young pianists. Hum Mov Sci. (2021) 75:102748. doi: 10.1016/j.humov.2020.102748
67. Mahmoudzadeh, A, Nakhostin Ansari, N, Naghdi, S, Ghasemi, E, Motamedzadeh, O, Shaw, BS, et al. Role of spasticity severity in the balance of post-stroke patients. Front Hum Neurosci. (2021) 15:783093. doi: 10.3389/fnhum.2021.783093
68. Rand, D, and Eng, JJ. Predicting daily use of the affected upper extremity 1 year after stroke. J Stroke Cerebrovasc Dis. (2015) 24:274–83. doi: 10.1016/j.jstrokecerebrovasdis.2014.07.039
69. Lang, CE, Macdonald, JR, Reisman, DS, Boyd, L, Jacobson Kimberley, T, Schindler-Ivens, SM, et al. Observation of amounts of movement practice provided during stroke rehabilitation. Arch Phys Med Rehabil. (2009) 90:1692–8. doi: 10.1016/j.apmr.2009.04.005
70. Abdullahi, A, Sabo, B, Badaru, UM, Saeys, W, and Truijen, S. Factors influencing recovery of upper limb motor function during constraint-induced movement therapy for people with stroke. Transl Neurosci. (2022) 13:453–9. doi: 10.1515/tnsci-2022-0260
71. Ward, NS, Brander, F, and Kelly, K. Intensive upper limb neurorehabilitation in chronic stroke: outcomes from the queen square programme. J Neurol Neurosurg Psychiatry. (2019) 90:498–506. doi: 10.1136/jnnp-2018-319954
72. Sehatzadeh, S . Effect of increased intensity of physiotherapy on patient outcomes after stroke: an evidence-based analysis. Ont Health Technol Assess Ser. (2015) 15:1–42.
73. Pang, MY, Harris, JE, and Eng, JJ. A community-based upper-extremity group exercise program improves motor function and performance of functional activities in chronic stroke: a randomized controlled trial. Arch Phys Med Rehabil. (2006) 87:1–9. doi: 10.1016/j.apmr.2005.08.113
74. Abdullahi, A, Truijen, S, and Saeys, W. Neurobiology of recovery of motor function after stroke: the central nervous system biomarker effects of constraint-induced movement therapy. Neural Plast. (2020) 2020:1–12. doi: 10.1155/2020/9484298
75. Aprile, I, Germanotta, M, Cruciani, A, Loreti, S, Pecchioli, C, Cecchi, F, et al. Upper limb robotic rehabilitation after stroke: a multicenter, randomized clinical trial. J Neurol Phys Ther. (2020) 44:3–14. doi: 10.1097/NPT.0000000000000295
76. McNulty, PA, Thompson-Butel, AG, Faux, SG, Lin, G, Katrak, PH, and Harris, LR. The efficacy of Wii-based movement therapy for upper limb rehabilitation in the chronic poststroke period: a randomized controlled trial. Int J Stroke. (2015) 10:1253–60. doi: 10.1111/ijs.12594
77. Abdullahi, A, Van Criekinge, T, Umar, NA, Zakari, UU, Truijen, S, and Saeys, W. Effect of constraint-induced movement therapy on persons-reported outcomes of health status after stroke: a systematic review and meta-analysis. Int J Rehabil Res. (2021) 44:15–23. doi: 10.1097/MRR.0000000000000446
78. Etoom, M, Hawamdeh, M, Hawamdeh, Z, Alwardat, M, Giordani, L, Bacciu, S, et al. Constraint-induced movement therapy as a rehabilitation intervention for upper extremity in stroke patients: systematic review and meta-analysis. Int J Rehabil Res. (2016) 39:197–210. doi: 10.1097/MRR.0000000000000169
79. Kwakkel, G, Veerbeek, JM, van Wegen, EE, and Wolf, SL. Constraint-induced movement therapy after stroke. Lancet Neurol. (2015) 14:224–34. doi: 10.1016/S1474-4422(14)70160-7
80. Rocha, LSO, Gama, GCB, Rocha, RSB, Rocha, LB, Dias, CP, Santos, LLS, et al. Constraint induced movement therapy increases functionality and quality of life after stroke. J Stroke Cerebrovasc Dis. (2021) 30:105774. doi: 10.1016/j.jstrokecerebrovasdis.2021.105774
81. Abdullahi, A . Effects of number of repetitions and number of hours of shaping practice during constraint-induced movement therapy: a randomized controlled trial. Neurol Res Int. (2018) 2018:1–9. doi: 10.1155/2018/5496408
82. Birkenmeier, RL, Prager, EM, and Lang, CE. Translating animal doses of task-specific training to people with chronic stroke in 1-hour therapy sessions: a proof-of-concept study. Neurorehabil Neural Repair. (2010) 24:620–35. doi: 10.1177/1545968310361957
83. Otaki, R, Oouchida, Y, Aizu, N, Sudo, T, Sasahara, H, Saito, Y, et al. Relationship between body-specific attention to a paretic limb and real-world arm use in stroke patients: a longitudinal study. Front Syst Neurosci. (2022) 15:806257. doi: 10.3389/fnsys.2021.806257
84. Lee, Y-J, Liang, JN, and Wen, Y-T. Characteristics of postural muscle activity in response to a motor-motor task in elderly. Appl Sci. (2019) 9:4319. doi: 10.3390/app9204319
85. Petersen, TH, Willerslev-Olsen, M, Conway, BA, and Nielsen, JB. The motor cortex drives the muscles during walking in human subjects. J Physiol. (2012) 590:2443–52. doi: 10.1113/jphysiol.2012.227397
86. Pulverenti, TS, Zaaya, M, Grabowski, M, Grabowski, E, Islam, MA, Li, J, et al. Neurophysiological changes after paired brain and spinal cord stimulation coupled with locomotor training in human spinal cord injury. Front Neurol. (2021) 12:627975. doi: 10.3389/fneur.2021.627975
87. Bergfeldt, U, Jonsson, T, Bergfeldt, L, and Julin, P. Cortical activation changes and improved motor function in stroke patients after focal spasticity therapy--an interventional study applying repeated fMRI. BMC Neurol. (2015) 15:52. doi: 10.1186/s12883-015-0306-4
88. Han, KJ, and Kim, JY. The effects of bilateral movement training on upper limb function in chronic stroke patients. J Phys Ther Sci. (2016) 28:2299–302. doi: 10.1589/jpts.28.2299
89. Stinear, JW, and Byblow, WD. Rhythmic bilateral movement training modulates corticomotor excitability and enhances upper limb motricity poststroke: a pilot study. J Clin Neurophysiol. (2004) 21:124–31. doi: 10.1097/00004691-200403000-00008
90. Monaghan, K, Horgan, F, Blake, C, Cornall, C, Hickey, PPM, Lyons, BE, et al. Physical treatment interventions for managing spasticity after stroke. Cochrane Database Syst Rev. (2017) 2017:CD009188. doi: 10.1002/14651858.CD009188.pub2
91. Abdullahi, A, Wong, TW, Van Criekinge, T, and Ng, SS. Combination of non-invasive brain stimulation and constraint induced movement therapy in patients with stroke: a systematic review and Meta-analysis. Expert Rev Neurother. (2023) 23:187–203. doi: 10.1080/14737175.2023.2177154
92. Abdullahi, A, Wong, TWL, and Ng, SSM. Rehabilitation of severe impairment in motor function after stroke: suggestions for harnessing the potentials of Mirror neurons and the Mentalizing systems to stimulate recovery. Brain Sci. (2022) 12:1311. doi: 10.3390/brainsci12101311
93. Rosenfeldt, AB, Linder, SM, Davidson, S, Clark, C, Zimmerman, NM, Lee, JJ, et al. Combined aerobic exercise and task practice improve health-related quality of life Poststroke: a preliminary analysis. Arch Phys Med Rehabil. (2019) 100:923–30. doi: 10.1016/j.apmr.2018.11.011
94. Wu, CW, Seo, HJ, and Cohen, LG. Influence of electric somatosensory stimulation on paretic-hand function in chronic stroke. Arch Phys Med Rehabil. (2006) 87:351–7. doi: 10.1016/j.apmr.2005.11.019
95. Kwakkel, G, Kollen, B, and Twisk, J. Impact of time on improvement of outcome after stroke. Stroke. (2008) 37:2348–53. doi: 10.1161/01.STR.0000238594.91938.1e
96. Dromerick, AW, Lang, CE, Birkenmeier, RL, Wagner, JM, Miller, JP, Videen, TO, et al. Very early constraint-induced movement during stroke rehabilitation (VECTORS): a single-center RCT. Neurol. (2009) 73:195–201. doi: 10.1212/WNL.0b013e3181ab2b27
97. Woldag, H, Gerhold, LL, de Groot, M, Wohlfart, K, Wagner, A, and Hummelsheim, H. Early prediction of functional outcome after stroke. Brain Inj. (2006) 20:1047–52. doi: 10.1080/02699050600915422
98. Stinear, CM, Byblow, WD, and Ward, SH. An update on predicting motor recovery after stroke. Ann Phys Rehabil Med. (2014) 57:489–98. doi: 10.1016/j.rehab.2014.08.006
Keywords: stroke, upper extremity, lower extremity, motor recovery, natural instinct for walking hypothesis, bipedal locomotion hypothesis, central pattern generators hypothesis, role of spasticity hypothesis
Citation: Abdullahi A, Wong TWL and Ng SSM (2023) Variation in the rate of recovery in motor function between the upper and lower limbs in patients with stroke: some proposed hypotheses and their implications for research and practice. Front. Neurol. 14:1225924. doi: 10.3389/fneur.2023.1225924
Received: 20 May 2023; Accepted: 24 July 2023;
Published: 03 August 2023.
Edited by:
Teresa Paolucci, University of Studies G. d'Annunzio Chieti and Pescara, ItalyReviewed by:
Jolanta Dorszewska, Poznan University of Medical Sciences, PolandCopyright © 2023 Abdullahi, Wong and Ng. This is an open-access article distributed under the terms of the Creative Commons Attribution License (CC BY). The use, distribution or reproduction in other forums is permitted, provided the original author(s) and the copyright owner(s) are credited and that the original publication in this journal is cited, in accordance with accepted academic practice. No use, distribution or reproduction is permitted which does not comply with these terms.
*Correspondence: Shamay S. M. Ng, c2hhbWF5Lm5nQHBvbHl1LmVkdS5oaw==
†ORCID: Auwal Abdullahi https://orcid.org/0000-0001-9214-3313
Thomson W. L. Wong https://orcid.org/0000-0002-6267-9034
Shamay S. M. Ng https://orcid.org/0000-0003-1660-0548
Disclaimer: All claims expressed in this article are solely those of the authors and do not necessarily represent those of their affiliated organizations, or those of the publisher, the editors and the reviewers. Any product that may be evaluated in this article or claim that may be made by its manufacturer is not guaranteed or endorsed by the publisher.
Research integrity at Frontiers
Learn more about the work of our research integrity team to safeguard the quality of each article we publish.