- 1Department of Neurology, Medical University of South Carolina, Charleston, SC, United States
- 2Department of Neurology, Augusta University Medical Center, Augusta, GA, United States
- 3Department of Neurosciences, Medical University of South Carolina, Charleston, SC, United States
- 4Department of Public Health Sciences, Medical University of South Carolina, Charleston, SC, United States
- 5Department of Psychiatry, Medical University of South Carolina, Charleston, SC, United States
Background: In pre-clinical animal models of Parkinson's disease (PD), vagus nerve stimulation (VNS) can rescue motor deficits and protect susceptible neuronal populations. Transcutaneous auricular vagus nerve stimulation (taVNS) has emerged as a non-invasive alternative to traditional invasive cervical VNS. This is the first report summarizing the safety, feasibility, and preliminary efficacy of repeated sessions of taVNS in participants with PD.
Objectives: To evaluate the feasibility, safety, and possible efficacy of taVNS for motor and non-motor symptoms in mild to moderate PD.
Methods: This is a double-blind, sham controlled RCT (NCT04157621) of taVNS in 30 subjects with mild to moderate PD without cognitive impairment. Participants received 10, 1-h taVNS sessions (25 Hz, 200% of sensory threshold, 500 μs pulse width, 60 s on and 30 s off) over a 2-week period. Primary outcome measures were feasibility and safety of the intervention; secondary outcomes included the MDS-UPDRS, cognitive function and self-reported symptom improvement.
Results: taVNS treatment was feasible, however, daily in-office visits were reported as being burdensome for participants. While five participants in the taVNS group and three in the sham group self-reported one or more minor adverse events, no major adverse events occurred. There were no group differences on blood pressure and heart rate throughout the intervention. There were no group differences in MDS-UPDRS scores or self-reported measures. Although global cognitive scores remained stable across groups, there was a reduction in verbal fluency within the taVNS group.
Conclusions: taVNS was safe, and well-tolerated in PD participants. Future studies of taVNS for PD should explore at-home stimulation devices and optimize stimulation parameters to reduce variability and maximize engagement of neural targets.
1. Introduction
Parkinson's disease is the second most common neurodegenerative disorder and is characterized by a loss of nigrostriatal dopamine cells which becomes more widespread across neural networks with disease progression (1). PD is highly heterogeneous in its presentation, but characteristically involves motor symptoms including resting tremor, bradykinesia, rigidity, and postural instability. While clinical diagnosis is based on clinical symptoms, definitive diagnosis can only be performed post-mortem and requires the identification of Lewy bodies produced by protein aggregates including alpha-synuclein (2). Traditional pharmacological treatments for PD such as levodopa/carbidopa primarily target the underlying depletion of nigrostriatal dopamine (3). Limitations of dopaminergic therapy include the development of motor fluctuations, poor efficacy on a subset of non-motor PD symptoms, and no influence on the rate of disease progression (2, 4, 5). Motor fluctuations in PD can be addressed through a neurosurgical neuromodulation technique known as Deep Brain Stimulation (DBS). DBS uses continuous high frequency stimulation to target either the subthalamic nucleus (STN) or globus pallidus internus (GPi). Despite the effectiveness of this approach, it may not be appropriate for all patients and the responsiveness to DBS is strongly dependent upon responsiveness to dopaminergic medications (4). Furthermore, medications and DBS produce limited improvements in non-motor PD symptoms which have significant impact on patient quality of life (5). In addition to neurodegeneration of dopaminergic substantia nigra (SN) neurons, PD results in significant neuronal loss within the noradrenergic Locus Coeruleus (LC) and cholinergic basal forebrain (6). Neuronal degeneration within these regions appear to precede the onset of PD motor symptoms (7). Although adrenergic and cholinergic projections influence motor control (8, 9), they additionally have projections to limbic and cortical regions which, if disrupted, can result in symptoms such as apathy, fatigue, REM behavior disorder and cognitive decline (10, 11), necessitating development of non-dopaminergic approaches to the management of PD.
Several neuromodulation modalities beyond DBS including transcranial magnetic stimulation (TMS) and transcranial direct current stimulation (tDCS) have been investigated as adjuvant therapies to address PD symptoms, however, these approaches are limited in their ability to target deep brain structures affected early in PD pathology (12). The vagus nerve is the longest cranial nerve in the body and carries both sensory afferent information from internal organs to the brain and efferent motor signals from the brain to the body (13). Uniquely, vagus nerve stimulation (VNS) can modulate cholinergic and noradrenergic outputs indirectly via the nucleus tractus solitarius (NTS) (14, 15). Furthermore, preclinical animal models of PD have demonstrated VNS can improve locomotor control, reduce markers of neuroinflammation, decrease intrasomal alpha synuclein, increase brain derived neurotrophic factor (BDNF) and attenuate neuronal damage within the LC and SN (16, 17). Thus, VNS may be an effective approach to improve motor and non-motor symptoms in people with PD. Although cervical VNS is relatively safe, there are risks associated with surgical implantation, and costs of the procedure can be high, reducing patient access (18). Non-invasive forms of brain stimulation can target these structures and minimize the risks associated with electrode implantation. Transcutaneous auricular VNS (taVNS) is a non-invasive form of VNS which delivers electrical stimulation to the auricular branch of the vagus nerve (ABVN) (19, 20). Previous taVNS studies across a range of sites and protocols have reported stimulation to be safe, with limited side effect profiles which most frequently includes transient ear pain, headache, and tingling, however, evidence of safety and efficacy in the PD population is limited (21). Early reports have suggested taVNS treatment may be effective in treating gait disability in PD, however, these studies have been limited to a single session of stimulation (22). The only non-invasive VNS study in PD performed over multiple sessions used transcutaneous cervical VNS in a small sample with limited reporting on specific stimulation parameters and outcomes (Table 1). taVNS can activate cerebral afferents of the vagal pathway and modulate physiological markers (i.e., heart rate) as is observed with traditional, cervically implanted VNS (26–28). These taVNS studies provide preliminary evidence for motor and non-motor benefits but may have been limited by low dosing and single session therapy. The objective of this study was to establish the feasibility, safety, and signals of efficacy of taVNS in mild to moderate PD participants using a comprehensive multiday clinical trial.
2. Materials and methods
2.1. Study design
From 2018 to 2021, 30 participants with mild to moderate idiopathic PD were recruited from a Movement Disorders Clinic at the Medical University of South Carolina (MUSC) to undergo either taVNS (n = 15) or sham (n = 15) stimulation (Figure 1). The length of participant recruitment was longer than anticipated due to the impact of COVID-19 on in-person visits. Study procedures were reviewed and approved by the MUSC Institutional Review Board and the study was registered on ClinicalTrials.gov (NCT04157621). All participants enrolled in the study were informed of study procedures and provided written consent. Study procedures included an initial screening visit, followed by 10 visits for stimulation over a period of 2 weeks (Figure 1). One week following the final stimulation visit, a follow-up safety assessment was performed. Assessments of motor efficacy were performed in the OFF-medication state in order to avoid any influence of medication response fluctuations on the MDS-UPDRS Part III. Cognitive measures on the contrary were elicited in the ON medication state in order to avoid lack of effort, bradyphrenia, anxiety, and depression, which can be associated with OFF periods, interfering with cognitive assessments.
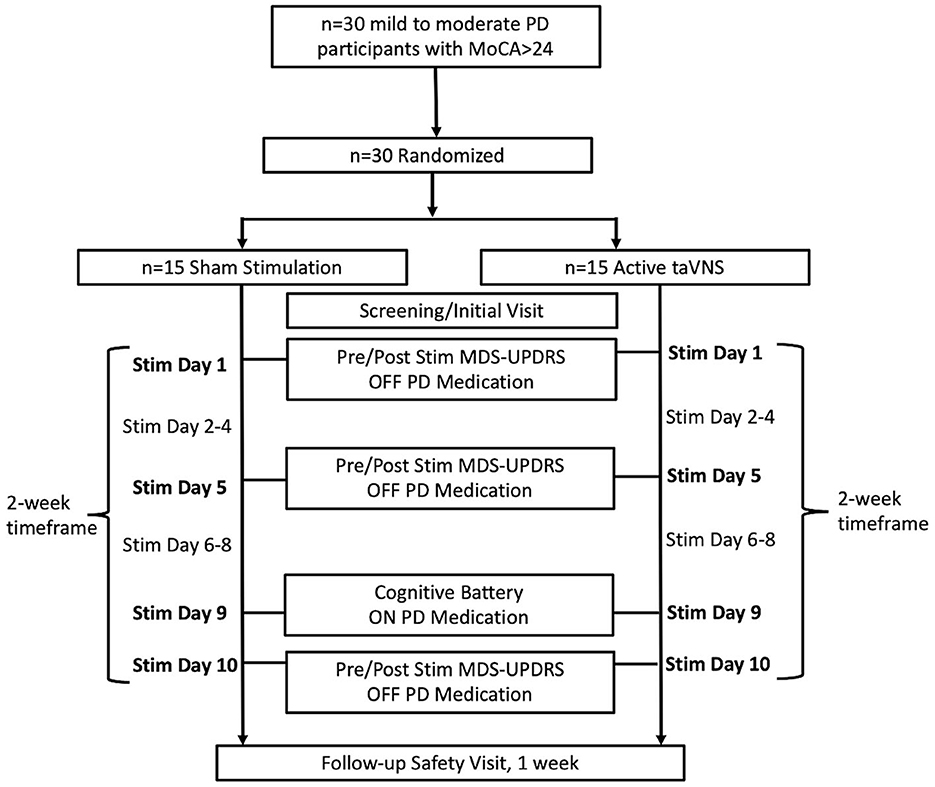
Figure 1. Flow diagram of participant enrollment, treatment group allocation, and timeline of study procedures and assessments.
2.2. Randomization and blinding
Randomization of the 30 participants was performed using the REDCap randomization module in which there was a 1:1 ratio in group assignment (taVNS vs. sham stimulation). All participants and study staff were blinded to which treatment assignment with exception of the Principal Investigator and laboratory personnel performing active or sham stimulation. To maintain objectivity of motor assessments a blinded movement disorders neurologist rated videotaped MDS-UPDRS III examinations.
2.3. Sample size and power calculations
There are no prior studies from which to estimate treatment or placebo effects associated with taVNS in PD. This study was powered to detect a clinically meaningful reduction of 3.25 points on the MDS-UPDRS Part III (29), assuming a small and statistically non-significant change of 1 on the MDS-UPDRS Part III with sham taVNS, and a pooled standard deviation of 2.0. At 80% power and alpha = 0.05, using a two-tailed t-test for difference in means, the total sample size required was 26 (13 per group). Additional participants were recruited to account for attrition.
2.4. Inclusion and exclusion criteria
Individuals meeting UK Brain Bank diagnostic criteria for Parkinson's disease (30) who were between 40 and 79 years of age, taking levodopa three or more times daily, with Hoehn and Yahr staging between 2 and 3 were eligible to participate. Exclusion criteria included prior diagnosis of dementia (31) or mild cognitive impairment on screening [MOCA < 24, (32, 33)], visual hallucinations or other psychotic symptoms, history of ear trauma or facial pain disorder, history of comorbid neurologic disorders or major cardiovascular conditions, history of deep brain stimulation or other brain surgery, neurogenic orthostatic hypotension, chronic respiratory illness, pregnancy, and use of cholinesterase inhibitors or Level 2 and 3 anticholinergic medications (34). All participants were expected to be stable on medication for PD motor and non-motor symptoms for a minimum of 30 days before and for the duration of the trial.
2.5. Transcutaneous auricular vagus nerve stimulation (taVNS) protocol
Active taVNS was applied using custom fabricated ear clip electrodes (1 cm round electrode surface) designed to deliver electrical stimulation to the anterior wall of the left outer ear canal landmarked by the tragus (Figure 2). The reasoning for left ear stimulation is based on conventional, surgically implanted VNS trials which primarily target the left cervical bundle of the vagus nerve. In addition, the afferent effects of taVNS have been primarily established in a left-only fashion (35–37). Sham stimulation utilized the same stimulation parameters; however current was delivered to the left earlobe, a region with limited to no innervation of the ABVN. Electrodes were applied to the ear using a conductive Ten20 paste and connected to an FDA 510 k-cleared constant current electrical nerve stimulation device.
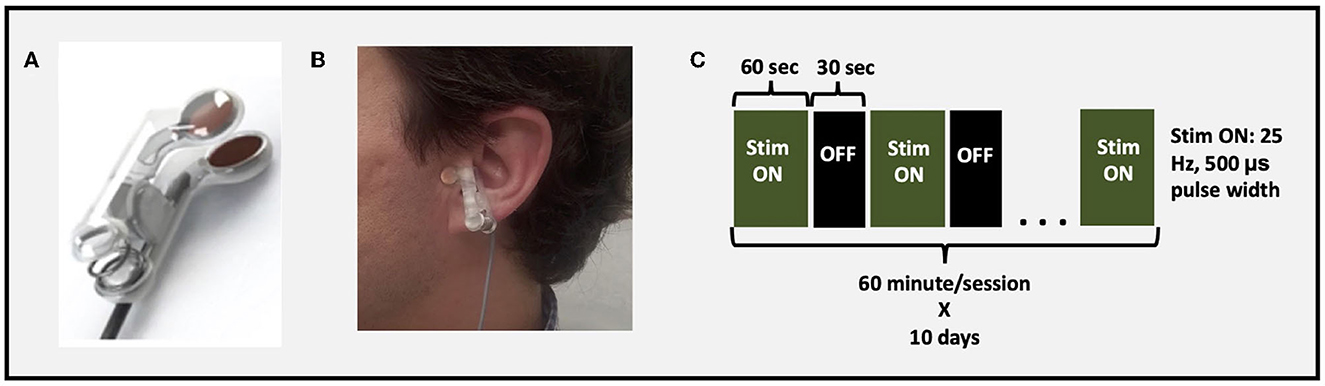
Figure 2. taVNS setup and parameters. (A) taVNS electrodes used to deliver stimulation, (B) example of taVNS electrode device attached to tragus, (C) stimulation parameters and paradigm.
After participants were connected to the stimulation system, they remained supine and were instructed to stay awake and maintain still in a comfortable position. Stimulation was administered for 1-h/day for 10 total days spread over 2 weeks. The stimulation parameters were consistent with prior work suggesting activation of vagal afferent network (36): Pulse Width 500 μs; frequency 25 Hz; duty cycle 60 s On, 30 s OFF; current intensity 200% perceptual threshold (Figure 2). Perceptual threshold was defined by the minimum amount of current required to be perceived by the participant.
2.6. Safety and tolerability evaluation
Safety and tolerability of taVNS stimulation included monitoring of participant reported adverse events, MDS UPDRS Part III examinations, cognitive testing, and the Columbia-Suicide Severity Rating Scale (C-SSRS). Monitoring of vital signs (heart rate and blood pressure) occurred pre-stimulation, 10 min into stimulation and 50 min into stimulation. Neurogenic orthostatic hypotension (NOH) was reported as an adverse event if it was clinically significant. Clinically significant NOH was defined as lightheadedness or syncope in the setting of a 20 mm Hg or more drop in systolic BP or 10 mm Hg or more drop in diastolic BP with standing at 50% or more of assessments. An adverse event was considered related to the study intervention if the event had a reasonable possibility of being causally related to the intervention being administered. Qualitative interviews following study participation were performed to assess participant experiences during the study and feasibility of developing taVNS as a clinical intervention.
2.7. Secondary outcomes
As an early indicator for treatment efficacy, we assessed motor function with the Movement Disorder Society Unified Parkinson's Disease Rating Scale (MDS-UPDRS) Part III, a comprehensive scale that rates the severity of motor symptoms in Parkinson's Disease, modified for videotaped assessments (rigidity testing omitted) (38). Both acute (pre to post stimulation change on a given visit) and subacute (baseline to visit 5 and visit 10 change) assessments were evaluated. All MDS-UPDRS Part III evaluations were performed in the OFF-medication state (defined as at least 12 h without PD medication) to capture the standalone benefits of stimulation independent of dopaminergic medication. MDS-UPDRS Parts I, II, and IV were used to evaluate changes in motor and non-motor aspects of daily living and motor complications. Other secondary outcome measures focused on changes in cognitive function as measured by the Delis-Kaplan Executive Function System (DKEFS) to evaluate letter fluency, category fluency and category switching (39) and the Digit Span (Backward and Forward) assessment to evaluate short-term and working memory (40). Cognitive secondary outcome measures were obtained in the medication ON-state at baseline and visit 9. Patient reported outcomes included the Movement Disorders Society Non-Motor Symptoms Scale for Parkinson's Disease (NMSS), the freezing of gait questionnaire (FOG-Q) (41, 42), and the Conners Adult ADHD Rating Scale short form self-report (CAARS-S:S). The CAARS-S:S served as a patient reported outcome of symptoms associated with inattention and executive dysfunction (43, 44). Additionally, the Patient-Reported Outcomes Measurement Information System (PROMIS) was used to evaluate sleep related impairment (PROMIS-Sleep Related Impairment), applied cognitive abilities (PROMIS-Applied Cognition) and fatigue (PROMIS-Fatigue) (45). Self-reported questionnaires and outcome measures were collected at screening and visit 9.
2.8. Statistical analysis
Demographic data, disease severity measures, motor scores at baseline and non-motor scores at baseline were compared between the two treatment groups (active vs. sham taVNS) using chi-squared and two-sided two-sample t-tests. Treatment group differences were considered significant if p-value < 0.05. To assess the effects of taVNS on systolic blood pressure, diastolic blood pressure and heart rate, the change in score before and after 50 min of treatment was compared between the taVNS and sham group using a two-sample t-tests. Data was aggregated from 112 sham sessions and 122 taVNS sessions where complete vital datasets were available. To assess the effects of taVNS on motor (MDS-UPDRS Part III scores) and cognitive outcome measures, the change in score was compared between the active and sham groups using a two sample-sample t-test. Acute effects of taVNS on MDS-UPDRS Part-III scores were evaluated as post-stimulation scores minus pre-stimulation scores at a given visit. Meanwhile subacute effects of taVNS on MDS-UPDRS Part-III scores were evaluated as post-stimulation scores at visit 10 minus pre-stimulation scores at visit 1. The motor outcome measure was considered significant if p-value < 0.05. Cognitive outcome measures were considered significant if p-value < 0.01 (Bonferroni corrected for the five cognitive scores evaluated). To address missing data statistical analyses were performed by excluding participants with missing data and were then repeated using imputation of missing data points.
3. Results
3.1. Demographics and baseline data
Table 2 displays the demographics, disease severity and baseline motor and cognitive scores of participants in the taVNS (n = 15) and sham (n = 15) treatment groups. Fifty percent of participants were female and the mean age was 67. Mean time since symptom onset was 7.7 years (median: 6.5) and mean time since PD diagnosis was 5.1 years (median: 4). Treatment groups did not significantly differ in any demographic characteristics, baseline motor symptom severity (MDS-UPDRS Part-III score active group 25.9 ± 10.3 and sham 24.5 ± 7.3), or baseline cognitive function. Stimulation intensity determined by each participant's sensory threshold was greater in the taVNS group (t = 3.32, df = 28, p-value = 0.0025) than the sham group. Sensory thresholds ranged from 1.4 to 3 in the taVNS group and 0.8–2 in the sham group (Supplementary Figure 1).
3.2. Feasibility, adverse events and tolerability of taVNS
All 30 participants in the taVNS and sham treatment groups completed the on-site stimulation visits. The frequency of visits to the study center for stimulation was reported as burdensome by participants via qualitative interviews following trial participation. taVNS administration was well-tolerated and feasible without technical issues at the study site. As far as safety, during the 10-day period of stimulation, five (33.3%) participants in the active taVNS group and 3 (20%) participants in the sham group self-reported one or more minor adverse event (AE). The most frequently reported AE in the active taVNS group was difficulty sleeping (n = 2) followed by lightheadedness (n = 1), fatigue (n = 1), nausea (n = 1), ringing in the ear (n = 1), grinding teeth (n = 1), fluid in the ear (n = 1), jitteriness/anxiousness (n = 1), and vertigo (n = 1). The most frequently reported AE in the sham group was lightheadedness (n = 2) followed by difficulty sleeping (n = 1), headache (n = 1), fatigue (n = 1), difficulty concentrating (n = 1), and neck pain (n = 1). No pain at the site of stimulation was reported for either treatment group. No serious adverse events (SAEs) were reported for the duration of the study and follow-up. At the 1-week follow-up, 2 participants from the active taVNS group reported AEs including stomach and hip pain, difficulty sleeping, fatigue, and constipation. At follow-up, 1 participant from the sham group described decreased hearing within the left ear. There was no evidence for the development of suicidal thoughts as monitored by the Columbia-Suicide Severity Rating Scale (C-SSRS) (46). Vital signs were monitored and remained stable throughout the duration of the study. Systolic blood pressure (t=0.495, df=232, p=0.621), diastolic blood pressure (t=1.374, df=232, p=0.171) and heart rate (t=0.183, df=232, p=0.138) changes from pre-treatment to 50 minutes into treatment did not differ between the sham and taVNS groups. In the taVNS group there was a 3.6 mm Hg drop in systolic blood pressure and a 0.9 mm Hg drop in diastolic blood pressure and a 3.4 bpm drop in heart rate. Meanwhile in the sham group there was a 2.7 mm Hg drop in systolic blood pressure, a 0.7 mm Hg increase in diastolic blood pressure and a 1.8 bpm drop in heart rate. Orthostatic hypotension was assessed throughout the trial was identified to be clinically significant in 1 (6.6%) participant receiving active taVNS and 1 (6.6%) participant receiving sham stimulation.
3.3. Motor effects of taVNS
Figure 3 displays individual participant responses with regard to motor symptoms. A detailed summary of results is displayed in Table 3. Participants with missing MDS-UPDRS Part III data at visits 1, 5, and 10 were excluded from the analysis (see Table 3 for sample sizes used in the analysis). Analyses were repeated using imputation of missing data points; however, this is not shown since it did not influence significance of the reported results. Acute effects of stimulation did not significantly differ between groups at any of the timepoints (visits 1, 5, and 10) assessed (p-value > 0.05). Both treatment groups showed small reductions in their MDS-UPDRS Part-III scores (2.0 ± 5.6 points reduction in the taVNS group and 2.2 ± 4.3 points reduction in the sham group); however, there were no significant treatment group differences in subacute score changes (p-value = 0.906).
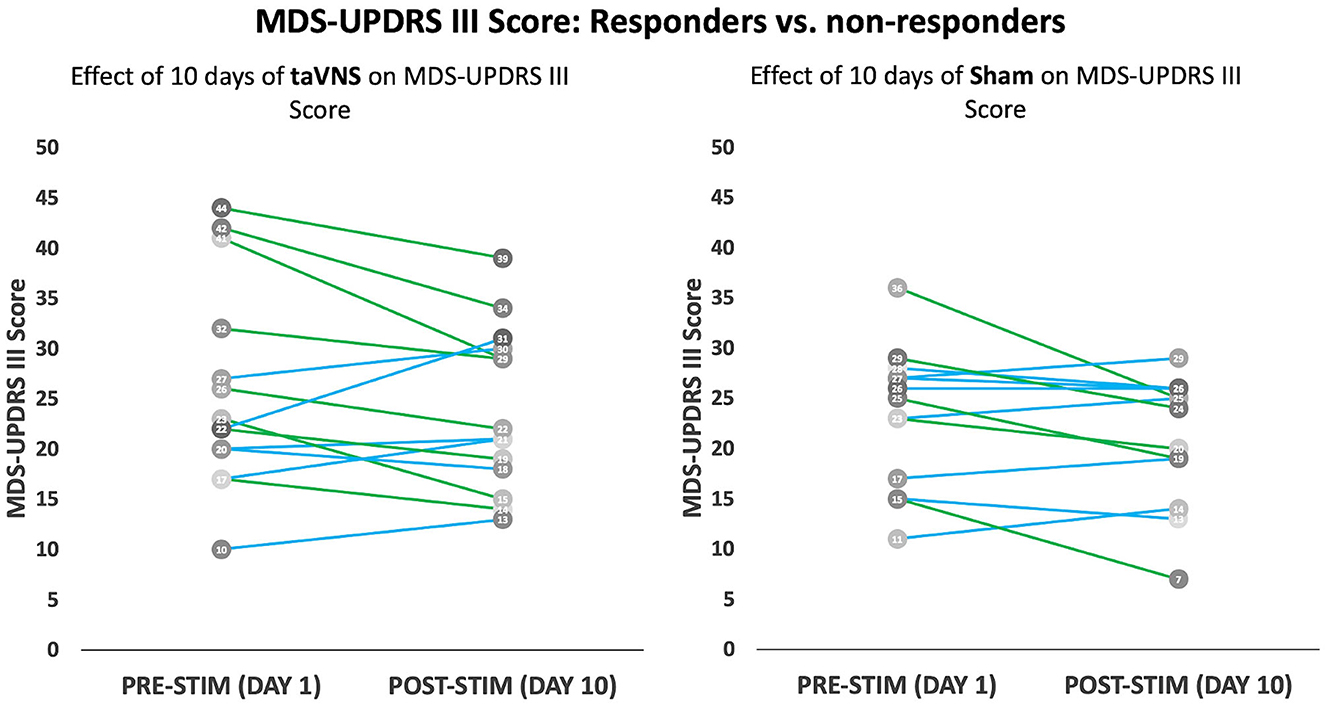
Figure 3. Modified MDS-UPDRS Part III score: MDS-UPDRS Part III scores before and after 10 days of taVNS (left) or sham (right) is shown for individual participants. Participants with a 3-point or greater improvement in UPDRS Part III are shown as green lines, while those with a <3-point drop are shown in blue lines.
3.4. Cognitive effects of taVNS
Cognitive, and patient reported outcomes are presented in Table 3. Cognition: As described for the motor outcome, participants with missing DKEFS (letter fluency, category fluency and category switching) data, and missing Digit Span (Backward and Forward) data were excluded from the analysis (taVNS group n = 1, sham group n = 1). Effects of taVNS on cognitive performance were evaluated by determining treatment group differences in change scores (post-stimulation at visit 9 minus pre-stimulation at baseline). DKEFS letter fluency performance decreased in the taVNS group by 3.8 points but increased in the sham group by 5.4 points. Similarly, DKEFS category fluency performance decreased in the taVNS group by 3.1 points but increased in the sham group by 3.4 points. Change scores in letter fluency (p-value = 0.008, uncorrected) and category fluency (p-value = 0.008, uncorrected) was significantly reduced in the taVNS group relative to the sham group. After Bonferroni correction accounting for number of cognitive tests performed (p-value threshold of 0.01), however, only group differences in category fluency remained significant. DKEFS category switching performance improved in the taVNS group by 1.3 points and was reduced in the sham group by 0.3 points. The Digit Span forward and backward scores increased slightly in the taVNS group (0.2 and 0.1 points, respectively) and increased slightly in the sham group (0.7 and 0.5 point, respectively). There were no significant treatment group differences in change scores for DKEFS category switching, or the digit span forward and backward. Patient reported outcomes: No significant changes from screening to visit 9 were observed for the NMSS, the freezing of gait questionnaire, CAARS or the PROMIS questionnaires.
4. Discussion
To our knowledge, this is the first prospective, randomized, double-blind, sham-controlled study to assess multi-day taVNS in PD participants. In this study of 30 participants with idiopathic PD, 10 days of taVNS stimulation was feasible, safe and well-tolerated. All participants within the taVNS group completed the full course of stimulation and no major AEs were observed. A minority of participants reported minor AEs, however, these were similar in nature and frequency between taVNS (33.3%) and sham (20.0%) treatment groups. No significant improvements or worsening of overall motor symptoms as measured by MDS-UPDRS Part-III score were observed in the taVNS group. In a subset of responders (>3 point improvement in MDS-UPDRS Part-III) within the taVNS group, bradykinesia and tremor symptoms showed the greatest improvements. Measures of cognition were not found to decline or improve in either group apart from verbal fluency measures which declined to a greater extent among the participants that received taVNS.
In the current study we demonstrated the safety of taVNS in individuals with PD as there were no group differences in the effects of taVNS and sham stimulation on heart rate and blood pressure. This safety profile is particularly important for individuals with PD due to the susceptibility of this population to cardiovascular autonomic dysfunction which can occur with mild to moderate disease progression (47). Furthermore, there were no significant acute or subacute changes in measures of motor function between the taVNS and sham stimulation groups; however, within the taVNS group several participants demonstrated clinically meaningful improvements. These findings indicate that there is a substantial degree of variability in taVNS response, which may include individual differences in nerve anatomy, and differences in baseline brain structure and function. For example, changes in brain structure and function resulting from PD progression might influence taVNS response. There is evidence that non-invasive brain stimulation may lose its effectiveness in modulating monosynaptic targets when white-matter pathways undergo degeneration (48). In contrast with previous non-invasive VNS studies, the results from the current study suggest that bradykinesia and tremor symptoms are the most responsive to stimulation, while gait and posture scores remained relatively unchanged. While improvement on gait and posture items from the UPDRS were not seen in our study, in the only other published taVNS trial for PD, a single 30-min session of stimulation produced a 2.4 point reduction in UPDRS-III and significant improvements in reaction time, gait speed, stride length and swing amplitude (23). In a randomized, sham-controlled transcutaneous cervical VNS trial, a single 2-min session of stimulation produced small improvements in spatiotemporal gait parameters including step length variability and step time (22). Differences in symptom response between this study and others may have been influenced by the overall small numbers of subjects, differences in study inclusion criteria, form of VNS stimulation (auricular vs. cervical) and the parameters used to perform stimulation including intensity, frequency, pulse width, waveform shape, and cycle duration. It is important to consider that while the current taVNS study is the first to use multiple days of stimulation in participants with PD, invasive VNS trials for treatment-resistant depression have demonstrated response rates build over time and can take months to produce meaningful clinical results. Thus, consideration for longer duration trials may be necessary to observe clinically meaningful results (49). A recent pilot study assessed the safety and feasibility of at-home, remotely monitored taVNS for participants to manage long COVID-19 symptoms (50). This remotely monitored approach may offer a solution for providing larger, clinically impactful doses of taVNS and avoid frequent stimulation visits at the study center.
In this study, we did not observe effects on fatigue, sleep impairment, or overall self-reported measures of cognitive functioning; however, a decline in verbal fluency was observed for the taVNS group. Interestingly, this finding shows similarities to the subthalamic nucleus (STN) deep brain stimulation (DBS) literature, wherein the most consistently reported cognitive effect of stimulation is a decline in verbal fluency. This points to the possibility that PD participants may be particularly susceptible to the interruption of brain networks involved in language and speech production. In other patient populations, such as those with treatment-resistant depression, traditional VNS improved verbal fluency (51). Notably, verbal fluency testing was performed at visit 9 shortly within minutes of completing stimulation. Given that there was no indication of subjective decline in cognition on formal scales (CAARS-S:S or PROMIS Applied Cognition), we suspect that this finding might represent an acute effect of stimulation. However, because we did not reassess at the safety follow-up, the time course for resolution is unknown.
A few limitations should be considered when interpreting result from this clinical trial. In this study, taVNS stimulation parameters (i.e., amplitude, frequency, electrode montage, and duty cycle) were chosen based off a systematic assessment in a small group of healthy controls (27). As a result, it is unknown whether stimulation administration was optimized for the PD population where neurodegeneration may impact the dose and stimulation parameters required to achieve therapeutic effects. Although the duration of stimulation in this taVNS study was significantly longer than previous clinical trials, preclinical studies have utilized longer stimulation to achieve physiological benefits. To account for the influence of disease state on response to stimulation, future studies should consider a systematic approach to identify optimal parameters in PD participants. Target engagement studies using neuroimaging and neurophysiological measures can be used to determine stimulation parameters as well as ear target (left vs. right vs. bilateral) which optimally engage afferent targets (26, 52). For example, iterative testing of these various parameters can be evaluated in the context of their ability to elicit changes in markers of vagal tone (i.e., pupil dilation) or blood oxygen level-dependent response within specific brain regions or networks (53). Furthermore, studies using direct neurophysiological measures of neural activity in the subthalamic nucleus via local field potential recordings have been proposed to provide mechanistic insights (54). Thus, future taVNS clinical trials should consider the use of objective these biomarkers to quantify target engagement.
To our knowledge, this is the first study which has evaluated the feasibility, safety, tolerability, and efficacy of multiday taVNS in PD participants. These results suggest the need for an improved mechanistic understanding of taVNS and optimization of stimulation parameters to effectively engage relevant pathophysiological targets (i.e., LC) for the development of future non-invasive VNS clinical trials for PD.
5. Conclusions
taVNS is a feasible, well-tolerated and safe neuromodulation approach for individuals with mild to moderate PD. Ten days of taVNS stimulation does not significantly improve global PD motor symptom severity; however, bradykinesia and tremor may be improved by stimulation in a subset of patients. Verbal fluency may be susceptible to transient worsening and should be closely monitored in future taVNS studies. Future randomized clinical trials of taVNS which aim to improve motor and non-motor symptoms in PD will benefit from the establishment of stimulation parameters which optimally engage neural targets, and an at-home treatment paradigm to improve patient centered treatment delivery.
Data availability statement
The raw data supporting the conclusions of this article will be made available by the authors, without undue reservation.
Ethics statement
The studies involving human participants were reviewed and approved by Medical University of South Carolina IRB. The patients/participants provided their written informed consent to participate in this study.
Author contributions
DL: statistical analysis: execution, review, and critique and manuscript: writing of the first draft. TT: research project: execution, statistical analysis: review and critique, and manuscript: review and critique. CM and LL: research project: execution and manuscript: review and critique. HB and BB: research project: conception and manuscript: review and critique. LH: research project: organization and execution and manuscript: review and critique. JE and AP: statistical analysis: design and execution. VH: research project: conception and organization and manuscript: review and critique. All authors contributed to the article and approved the submitted version.
Funding
This work was supported by a grant from the MUSC Center on Aging.
Acknowledgments
Firstly, we would like to thank all the participants who were willing to take part in this study. Additionally, we would like to thank the staff at MUSC's Murray Center for Research on Parkinson's and Related Disorders for supporting the execution of this study.
Conflict of interest
The authors declare that the research was conducted in the absence of any commercial or financial relationships that could be construed as a potential conflict of interest.
Publisher's note
All claims expressed in this article are solely those of the authors and do not necessarily represent those of their affiliated organizations, or those of the publisher, the editors and the reviewers. Any product that may be evaluated in this article, or claim that may be made by its manufacturer, is not guaranteed or endorsed by the publisher.
Supplementary material
The Supplementary Material for this article can be found online at: https://www.frontiersin.org/articles/10.3389/fneur.2023.1210103/full#supplementary-material
Supplementary Figure 1. Individual sensory thresholds used for dosing across participants.
References
1. Kouli A, Torsney KM, Kuan WL. Parkinson's disease: etiology, neuropathology, and pathogenesis. In:TB Stoker, JC Greenland, editors, Parkinson's Disease: Pathogenesis and Clinical Aspects. Brisbane, QLD: Codon Publications (2018).
2. Stefanis L. alpha-Synuclein in Parkinson's disease. Cold Spring Harb Perspect Med. (2012) 2:a009399. doi: 10.1101/cshperspect.a009399
3. Dauer W, Przedborski S. Parkinson's disease: mechanisms and models. Neuron. (2003) 39:889–909. doi: 10.1016/S0896-6273(03)00568-3
4. Lin Z, Zhang C, Li D, Sun B. Preoperative levodopa response and deep brain stimulation effects on motor outcomes in Parkinson's disease: a systematic review. Mov Disord Clin Pract. (2022) 9:140–55. doi: 10.1002/mdc3.13379
5. Prakash KM, Nadkarni NV, Lye WK, Yong MH, Tan EK. The impact of non-motor symptoms on the quality of life of Parkinson's disease patients: a longitudinal study. Eur J Neurol. (2016) 23:854–60. doi: 10.1111/ene.12950
6. Muller ML, Bohnen NI. Cholinergic dysfunction in Parkinson's disease. Curr Neurol Neurosci Rep. (2013) 13:377. doi: 10.1007/s11910-013-0377-9
7. Espay AJ, LeWitt PA, Kaufmann H. Norepinephrine deficiency in Parkinson's disease: the case for noradrenergic enhancement. Mov Disord. (2014) 29:1710–9. doi: 10.1002/mds.26048
8. Srinivasan J, Schmidt WJ. Potentiation of parkinsonian symptoms by depletion of locus coeruleus noradrenaline in 6-hydroxydopamine-induced partial degeneration of substantia nigra in rats. Eur J Neurosci. (2003) 17:2586–92. doi: 10.1046/j.1460-9568.2003.02684.x
9. Morris R, Martini DN, Madhyastha T, Kelly VE, Grabowski TJ, Nutt J, et al. Overview of the cholinergic contribution to gait, balance and falls in Parkinson's disease. Parkinsonism Relat Disord. (2019) 63:20–30. doi: 10.1016/j.parkreldis.2019.02.017
10. Prasuhn J, Prasuhn M, Fellbrich A, Strautz R, Lemmer F, Dreischmeier S, et al. Association of locus coeruleus and substantia nigra pathology with cognitive and motor functions in patients with Parkinson's disease. Neurology. (2021) 97:e1007–e16. doi: 10.1212/WNL.0000000000012444
11. Blesa J, Foffani G, Dehay B, Bezard E, Obeso JA. Motor and non-motor circuit disturbances in early Parkinson's disease: which happens first? Nat Rev Neurosci. (2022) 23:115–28. doi: 10.1038/s41583-021-00542-9
12. Zhang W, Deng B, Xie F, Zhou H, Guo JF, Jiang H, et al. Efficacy of repetitive transcranial magnetic stimulation in Parkinson's disease: a systematic review and meta-analysis of randomised controlled trials. EClinicalMedicine. (2022) 52:101589. doi: 10.1016/j.eclinm.2022.101589
13. George MS, Sackeim HA, Rush AJ, Marangell LB, Nahas Z, Husain MM, et al. Vagus nerve stimulation: a new tool for brain research and therapy. Biol Psychiatry. (2000) 47:287–95. doi: 10.1016/S0006-3223(99)00308-X
14. Nemeroff CB, Mayberg HS, Krahl SE, McNamara J, Frazer A, Henry TR, et al. VNS therapy in treatment-resistant depression: clinical evidence and putative neurobiological mechanisms. Neuropsychopharmacology. (2006) 31:1345–55. doi: 10.1038/sj.npp.1301082
15. Bowles S, Hickman J, Peng X, Williamson WR, Huang R, Washington K, et al. Vagus nerve stimulation drives selective circuit modulation through cholinergic reinforcement. Neuron. (2022) 110:2867–85e7. doi: 10.1016/j.neuron.2022.06.017
16. Farrand AQ, Verner RS, McGuire RM, Helke KL, Hinson VK, Boger HA. Differential effects of vagus nerve stimulation paradigms guide clinical development for Parkinson's disease. Brain Stimul. (2020) 13:1323–32. doi: 10.1016/j.brs.2020.06.078
17. Farrand AQ, Helke KL, Gregory RA, Gooz M, Hinson VK, Boger HA. Vagus nerve stimulation improves locomotion and neuronal populations in a model of Parkinson's disease. Brain Stimul. (2017) 10:1045–54. doi: 10.1016/j.brs.2017.08.008
18. Revesz D, Rydenhag B, Ben-Menachem E. Complications and safety of vagus nerve stimulation: 25 years of experience at a single center. J Neurosurg Pediatr. (2016) 18:97–104. doi: 10.3171/2016.1.PEDS15534
19. Peuker ET, Filler TJ. The nerve supply of the human auricle. Clin Anat. (2002) 15:35–7. doi: 10.1002/ca.1089
20. Kreisberg E, Esmaeilpour Z, Adair D, Khadka N, Datta A, Badran BW, et al. High-resolution computational modeling of the current flow in the outer ear during transcutaneous auricular Vagus Nerve Stimulation (taVNS). Brain Stimul. (2021) 14:1419–30. doi: 10.1016/j.brs.2021.09.001
21. Kim AY, Marduy A, de Melo PS, Gianlorenco AC, Kim CK, Choi H, et al. Safety of transcutaneous auricular vagus nerve stimulation (taVNS): a systematic review and meta-analysis. Sci Rep. (2022) 12:22055. doi: 10.1038/s41598-022-25864-1
22. Morris R, Yarnall AJ, Hunter H, Taylor JP, Baker MR, Rochester L. Noninvasive vagus nerve stimulation to target gait impairment in Parkinson's disease. Mov Disord. (2019) 34:918–9. doi: 10.1002/mds.27664
23. Marano M, Anzini G, Musumeci G, Magliozzi A, Pozzilli V, Capone F, et al. Transcutaneous auricular vagus stimulation improves gait and reaction time in Parkinson's disease. Mov Disord. (2022) 37:2163–4. doi: 10.1002/mds.29166
24. Mondal B, Choudhury S, Simon B, Baker MR, Kumar H. Noninvasive vagus nerve stimulation improves gait and reduces freezing of gait in Parkinson's disease. Mov Disord. (2019) 34:917–8. doi: 10.1002/mds.27662
25. Kaut O, Janocha L, Weismüller TJ, Wüllner U. Transcutaneous vagal nerve stimulation improves gastroenteric complaints in Parkinson's disease patients. NeuroRehabilitation. (2019) 45:449–51. doi: 10.3233/NRE-192909
26. Badran BW, Dowdle LT, Mithoefer OJ, LaBate NT, Coatsworth J, Brown JC, et al. Neurophysiologic effects of transcutaneous auricular vagus nerve stimulation (taVNS) via electrical stimulation of the tragus: a concurrent taVNS/fMRI study and review. Focus. (2022) 20:80–9. doi: 10.1176/appi.focus.20110
27. Badran BW, Mithoefer OJ, Summer CE, LaBate NT, Glusman CE, Badran AW, et al. Short trains of transcutaneous auricular vagus nerve stimulation (taVNS) have parameter-specific effects on heart rate. Brain Stimul. (2018) 11:699–708. doi: 10.1016/j.brs.2018.04.004
28. Badran BW, Brown JC, Dowdle LT, Mithoefer OJ, LaBate NT, Coatsworth J, et al. Tragus or cymba conchae? Investigating the anatomical foundation of transcutaneous auricular vagus nerve stimulation (taVNS). Brain Stimul. (2018) 11:947–8. doi: 10.1016/j.brs.2018.06.003
29. Horvath K, Aschermann Z, Acs P, Deli G, Janszky J, Komoly S, et al. Minimal clinically important difference on the Motor Examination part of MDS-UPDRS. Parkinsonism Relat Disord. (2015) 21:1421–6. doi: 10.1016/j.parkreldis.2015.10.006
30. Hughes AJ, Daniel SE, Kilford L, Lees AJ. Accuracy of clinical diagnosis of idiopathic Parkinson's disease: a clinico-pathological study of 100 cases. J Neurol Neurosurg Psychiatry. (1992) 55:181–4. doi: 10.1136/jnnp.55.3.181
31. Emre M, Aarsland D, Brown R, Burn DJ, Duyckaerts C, Mizuno Y, et al. Clinical diagnostic criteria for dementia associated with Parkinson's disease. Mov Disord. (2007) 22:1689–707. doi: 10.1002/mds.21507
32. Hoops S, Nazem S, Siderowf AD, Duda JE, Xie SX, Stern MB, et al. Validity of the MoCA and MMSE in the detection of MCI and dementia in Parkinson disease. Neurology. (2009) 73:1738–45. doi: 10.1212/WNL.0b013e3181c34b47
33. Dalrymple-Alford JC, MacAskill MR, Nakas CT, Livingston L, Graham C, Crucian GP, et al. The MoCA: well-suited screen for cognitive impairment in Parkinson disease. Neurology. (2010) 75:1717–25. doi: 10.1212/WNL.0b013e3181fc29c9
34. Hilmer SN, Mager DE, Simonsick EM, Cao Y, Ling SM, Windham BG, et al. A drug burden index to define the functional burden of medications in older people. Arch Intern Med. (2007) 167:781–7. doi: 10.1001/archinte.167.8.781
35. Frangos E, Ellrich J, Komisaruk BR. Non-invasive access to the Vagus Nerve central projections via electrical stimulation of the external ear: fMRI evidence in humans. Brain Stimul. (2015) 8:624–36. doi: 10.1016/j.brs.2014.11.018
36. Badran BW, Dowdle LT, Mithoefer OJ, LaBate NT, Coatsworth J, Brown JC, et al. Neurophysiologic effects of transcutaneous auricular vagus nerve stimulation (taVNS) via electrical stimulation of the tragus: a concurrent taVNS/fMRI study and review. Brain Stimul. (2018) 11:492–500. doi: 10.1016/j.brs.2017.12.009
37. Yakunina N, Kim SS, Nam EC. Optimization of transcutaneous vagus nerve stimulation using functional MRI. Neuromodulation. (2017) 20:290–300. doi: 10.1111/ner.12541
38. Goetz CG, Fahn S, Martinez-Martin P, Poewe W, Sampaio C, Stebbins GT, et al. Movement Disorder Society-sponsored revision of the Unified Parkinson's Disease Rating Scale (MDS-UPDRS): process, format, and clinimetric testing plan. Mov Disord. (2007) 22:41–7. doi: 10.1002/mds.21198
39. Homack S, Lee D, Riccio CA. Test review: Delis-Kaplan executive function system. J Clin Exp Neuropsychol. (2005) 27:599–609. doi: 10.1080/13803390490918444
40. Young JC, Sawyer RJ, Roper BL, Baughman BC. Expansion and re-examination of Digit Span effort indices on the WAIS-IV. Clin Neuropsychol. (2012) 26:147–59. doi: 10.1080/13854046.2011.647083
41. Chaudhuri KR, Martinez-Martin P, Brown RG, Sethi K, Stocchi F, Odin P, et al. The metric properties of a novel non-motor symptoms scale for Parkinson's disease: results from an international pilot study. Mov Disord. (2007) 22:1901–11. doi: 10.1002/mds.21596
42. Giladi N, Shabtai H, Simon ES, Biran S, Tal J, Korczyn AD. Construction of freezing of gait questionnaire for patients with Parkinsonism. Parkinsonism Relat Disord. (2000) 6:165–70. doi: 10.1016/S1353-8020(99)00062-0
43. Conners CK. Rating scales in attention-deficit/hyperactivity disorder: use in assessment and treatment monitoring. J Clin Psychiatry. (1998) 59(Suppl.7):24–30.
44. Hinson VK, Delambo A, Elm J, Turner T. A randomized clinical trial of atomoxetine for mild cognitive impairment in Parkinson's disease. Mov Disord Clin Pract. (2017) 4:416–23. doi: 10.1002/mdc3.12455
45. Jones J, Nielson SA, Trout J, Swenson M, Reiley J, Tanner J, et al. A validation study of PROMIS sleep disturbance (PROMIS-SD) and sleep related impairment (PROMIS-SRI) item banks in individuals with idiopathic Parkinson's disease and matched controls. J Parkinsons Dis. (2021) 11:877–83. doi: 10.3233/JPD-202429
46. Posner K, Brown GK, Stanley B, Brent DA, Yershova KV, Oquendo MA, et al. The Columbia-Suicide Severity Rating Scale: initial validity and internal consistency findings from three multisite studies with adolescents and adults. Am J Psychiatry. (2011) 168:1266–77. doi: 10.1176/appi.ajp.2011.10111704
47. Kim JS, Lee SH, Oh YS, Park JW, An JY, Park SK, et al. Cardiovascular autonomic dysfunction in mild and advanced Parkinson's disease. J Mov Disord. (2016) 9:97–103. doi: 10.14802/jmd.16001
48. Kearney-Ramos TE, Lench DH, Hoffman M, Correia B, Dowdle LT, Hanlon CA. Gray and white matter integrity influence TMS signal propagation: a multimodal evaluation in cocaine-dependent individuals. Sci Rep. (2018) 8:3253. doi: 10.1038/s41598-018-21634-0
49. O'Reardon JP, Cristancho P, Peshek AD. Vagus nerve stimulation (VNS) and treatment of depression: to the brainstem and beyond. Psychiatry. (2006) 3:54–63.
50. Badran BW, Huffman SM, Dancy M, Austelle CW, Bikson M, Kautz SA, et al. A pilot randomized controlled trial of supervised, at-home, self-administered transcutaneous auricular vagus nerve stimulation (taVNS) to manage long COVID symptoms. Bioelectron Med. (2022) 8:13. doi: 10.1186/s42234-022-00094-y
51. Sackeim HA, Keilp JG, Rush AJ, George MS, Marangell LB, Dormer JS, et al. The effects of vagus nerve stimulation on cognitive performance in patients with treatment-resistant depression. Neuropsychiatry Neuropsychol Behav Neurol. (2001) 14:53–62.
52. Keatch C, Lambert E, Woods W, Kameneva T. Measuring brain response to transcutaneous vagus nerve stimulation (tVNS) using simultaneous magnetoencephalography (MEG). J Neural Eng. (2022) 19:ac620c. doi: 10.1088/1741-2552/ac620c
53. Capone F, Motolese F, Di Zazzo A, Antonini M, Magliozzi A, Rossi M, et al. The effects of transcutaneous auricular vagal nerve stimulation on pupil size. Clin Neurophysiol. (2021) 132:1859–65. doi: 10.1016/j.clinph.2021.05.014
Keywords: transcutaneous auricular vagus nerve stimulation, Parkinson's disease, vagus nerve, taVNS, non-invasive brain stimulation
Citation: Lench DH, Turner TH, McLeod C, Boger HA, Lovera L, Heidelberg L, Elm J, Phan A, Badran BW and Hinson VK (2023) Multi-session transcutaneous auricular vagus nerve stimulation for Parkinson's disease: evaluating feasibility, safety, and preliminary efficacy. Front. Neurol. 14:1210103. doi: 10.3389/fneur.2023.1210103
Received: 21 April 2023; Accepted: 03 July 2023;
Published: 18 July 2023.
Edited by:
Hae-Won Shin, Chung-Ang University, Republic of KoreaReviewed by:
Marcelo Mendonça, Champalimaud Foundation, PortugalSophie Cho, Clinical Center (NIH), United States
Tatiana Kameneva, Swinburne University of Technology, Australia
Charlotte Maria Keatch, Swinburne University of Technology, Australia
Copyright © 2023 Lench, Turner, McLeod, Boger, Lovera, Heidelberg, Elm, Phan, Badran and Hinson. This is an open-access article distributed under the terms of the Creative Commons Attribution License (CC BY). The use, distribution or reproduction in other forums is permitted, provided the original author(s) and the copyright owner(s) are credited and that the original publication in this journal is cited, in accordance with accepted academic practice. No use, distribution or reproduction is permitted which does not comply with these terms.
*Correspondence: Vanessa K. Hinson, aGluc29udmsmI3gwMDA0MDttdXNjLmVkdQ==