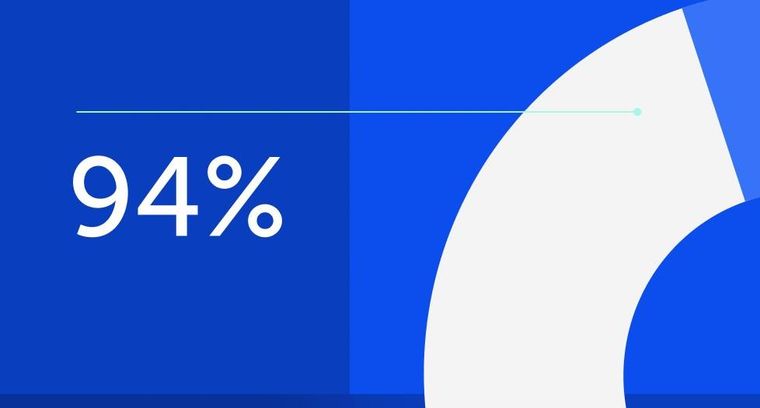
94% of researchers rate our articles as excellent or good
Learn more about the work of our research integrity team to safeguard the quality of each article we publish.
Find out more
REVIEW article
Front. Neurol., 12 October 2023
Sec. Dementia and Neurodegenerative Diseases
Volume 14 - 2023 | https://doi.org/10.3389/fneur.2023.1198262
This article is part of the Research TopicThe Neurobiology of ValuesView all 18 articles
Making good economic and social decisions is essential for individual and social welfare. Decades of research have provided compelling evidence that damage to the ventromedial prefrontal cortex (vmPFC) is associated with dramatic personality changes and impairments in economic and social decision-making. However, whether the vmPFC subserves a unified mechanism in the social and non-social domains remains unclear. When choosing between economic options, the vmPFC is thought to guide decision by encoding value signals that reflect the motivational relevance of the options on a common scale. A recent framework, the “extended common neural currency” hypothesis, suggests that the vmPFC may also assign values to social factors and principles, thereby guiding social decision-making. Although neural value signals have been observed in the vmPFC in both social and non-social studies, it is yet to be determined whether they have a causal influence on behavior or merely correlate with decision-making. In this review, we assess whether lesion studies of patients with vmPFC damage offer evidence for such a causal role of the vmPFC in shaping economic and social behavior.
Throughout the history of neurology, individual patient cases have played a major role in deepening our understanding of brain-behavior relationships. Among these cases, Phineas Gage and patient EVR, have generated enduring interest in the role of ventral areas of the prefrontal cortex, including the ventromedial prefrontal cortex (vmPFC, Figure 1). These two prototypical cases triggered a paradigm shift in neurology, from an era where the vmPFC was considered as a silent or less prominent cortex, to the view that the vmPFC plays a pivotal role in shaping human behavior. Following brain damage in ventromedial and orbital prefrontal areas, both patients displayed striking changes in personality and manner, despite seemingly preserved basic intellectual abilities (1–4). The story of these famous cases and their behavioral impairments have been documented in great details over the years. Here, we just briefly outline two aspects of the behavioral changes of these patients that appear relevant for the purpose of this review. First, both patients seemed impaired in value-based decision-making. Gage’s initial description stated that he “does not estimate size or money accurately, though he has memory as perfect as ever. He would not take $1,000 for a few pebbles which he took from an ancient river bed where he was at work” (4). Similarly, for EVR, “deciding where to dine might take hours, as he discussed each restaurant’s seating plan, particulars of menu, atmosphere, and management. He would drive to each restaurant to see how busy it was, but even then, he could not finally decide which to choose. Purchasing small items required in-depth consideration of brands, prices, and the best method of purchase” (2). Second, Gage and EVR also demonstrated impaired abilities in social decision-making. While Gage showed suspected impairments in social norm compliance and empathy, being described as “irreverent, indulging at times in the grossest profanity (which was not previously his custom) … manifesting but little deference for his fellows” (3), EVR showed poor judgment in choosing valuable social partners. For example, he began a short-lived relationship with a disreputable woman, and became “involved in a home-building partnership with a former coworker, a man of questionable reputation who had been fired from the company. Despite warnings by family and friends, EVR invested all his savings in the partnership. The business failed and he had to declare bankruptcy, losing his entire investment” (2).
Figure 1. Brain regions involved in the construction of economic and social value. The vmPFC is defined here as the medial orbitofrontal cortex and lower portions of the medial prefrontal cortex and anterior cingulate cortex, below the genu of the corpus callosum. mOFC, medial orbitofrontal cortex; lOFC, lateral orbitofrontal cortex; vmPFC, ventromedial prefrontal cortex; vlPFC, ventrolateral prefrontal cortex; dmPFC, dorsomedial prefrontal cortex; dlPFC, dorsolateral prefrontal cortex; dACC, dorsal anterior cingulate cortex; VS, ventral striatum; TPJ, temporo-parietal junction.
Although Gage and EVR cases pointed to a fundamental role of the vmPFC in social and non-social decision-making, the precise nature of the function(s) supported by this region has been long debated. Several theories have been put forward to propose a unified function of the vmPFC, including the somatic marker (5) and the affective meaning hypotheses (6). More recently the “cognitive map” hypothesis postulated a role for the vmPFC in learning the structure of the world by representing the relationships between its different states (7–10). Another theoretical framework, the “extended common neural currency” hypothesis, suggests that identical neural processes in the vmPFC assign values, or the motivational relevance, to social and non-social factors (11). This framework builds on theories proposed in the field of non-social decision-making. Empirical studies in that field have identified a set of brain regions, including the vmPFC and the ventral striatum, as part of a brain valuation system. Neural activity in these regions is thought to encode a “common neural currency,” allowing the value of different rewards or actions to be compared on a common scale, which is essential for guiding decision-making across different contexts (11). Recent observations showing the involvement of the brain valuation system during social decision-making have suggested extending the “common neural currency” hypothesis to social factors (11). In that schema, identical neurons in the vmPFC would process social and non-social value signals, although they may incorporate inputs from different brain regions that process information relevant for social or non-social contexts (11). By contrast, a “social valuation specific schema” assumes the existence of a distinct population of neurons, that may or may not be located in the vmPFC, dedicated specifically to computing values in social contexts (11).
Note that functional imaging studies implicating the vmPFC in reward valuation (12, 13) provide correlational, not causal, evidence for its role in value processing. Demonstrating the causal role of the vmPFC with non-invasive brain stimulation techniques is difficult as current methods mostly target brain areas at the cortical surface. Human lesion studies provide an alternative method to demonstrate the critical role of damaged regions.
Here, we aimed at assessing whether human vmPFC lesion studies support a causal role for the vmPFC in both social and non-social decision-making. We define social decisions as choice situations involving more than one person. We chose to focus this review on published works that could address the “extended common neural currency” hypothesis and establish whether the vmPFC represents the values of both social and non-social factors. We searched for vmPFC lesion studies spanning from 1990 to 2023 by querying the MEDLINE database with the following terms: (‘vmPFC’ OR ‘ventromedial prefrontal’ OR ‘orbitofrontal’ OR “medial prefrontal’) AND (‘patients’ OR ‘lesion’ OR ‘damage’). We only included group studies that used social or value-based decision-making tasks in patients with focal lesions in the vmPFC. We excluded clinical case studies, studies involving only patients with non-focal lesions such as neurodegenerative diseases or non-penetrating traumatic brain injuries, and studies that did not employ behavioral tasks addressing either social or non-social decision-making (for reviews on the effect of vmPFC damage on all cognitive domains, (see 14, 15)).
This review is structured as follows. First, we provide an overview of lesion studies implicating the vmPFC in representing the economic value of various goods during non-social decision-making. We then review lesion studies that have examined the role of the vmPFC in assigning values to the diverse contexts and interactions during social decision-making. For both economic and social values, we start off by summarizing key results from functional imaging studies identifying value signals in the vmPFC, and then outline the impairments in decision-making that result from vmPFC damage. Note that the functional imaging studies presented here are intended to provide a summary of the main findings related to each decision-making task, not to provide a comprehensive review on brain activations in social and non-social decision-making, which would fall beyond the scope of this review. We group the presentation of lesion studies by the type of valuation process or social situation they address. Note that in this review, we refer to the vmPFC as the medial orbitofrontal cortex (OFC) (Figure 1, area 14) and lower portions of the medial prefrontal (mPFC) and anterior cingulate cortex (ACC) below the genu of the corpus callosum (Figure 1, areas 24, 25, 32) (16), since imaging and lesion studies hardly dissociated these regions. Finally, we discuss open questions that could contribute to a more comprehensive understanding of the function of the vmPFC in shaping social and non-social behavior.
Dysfunction of the vmPFC has been associated early with abnormal goal-directed behaviors, including apathy, lack of initiative, poor judgment, indecisiveness and defective decision-making in particular in the economic domain. Later work led to the hypothesis that the vmPFC might represent the economic value of different goods in a common neural currency. In this section, we refer to economic values as the motivational and hedonic relevance of non-social options, such as material goods, monetary rewards, food etc. Economic value signals have been examined in the brain at three distinct stages of the decision-making process: during the receipt and consumption of rewards, when learning from obtained rewards, and when choosing rewards. These different stages involve distinct types of value signals: experienced value, anticipated value and decision value. We therefore group the presentation of studies on economic values according to these three types of neural value processes.
Experienced values signals correspond to the neural activity associated with the immediate hedonic aspects of receiving or consuming a reward, for example the outcome of a choice. Such value signals have been found in a set of brain regions that constitute the brain valuation system, including the ventral striatum (VS) and the vmPFC. In the vmPFC, neural activity has been associated, for example, with the subjective pleasantness of receiving monetary rewards, different types of food (e.g., snacks, juices, milkshakes, wine, etc.), or various goods (e.g., trinkets, pieces of music, movies, etc.) (13, 17–20). A causal role of the vmPFC in hedonic responses would therefore predict reduced expression of pleasure, i.e., anhedonia, after vmPFC damage. Few studies, however, have examined this prediction. Damage to the vmPFC was found to reduce self-reported happiness compared to prefrontal lesions outside of the vmPFC (21). However, in a larger population of combat veterans with penetrating brain injuries, bilateral lesion to the vmPFC was not associated with higher anhedonia in self-reported scales and clinical interviews (22). Moreover, in a behavioral gambling task, patients with vmPFC damage showed preserved pleasantness ratings and emotional autonomic responses when experiencing monetary gains (23, 24). Although these patients experienced weaker disappointment and no regret in the task (i.e., the effect on hedonic experience of unobtained outcomes and unchosen gambles, respectively), further studies suggested that those impairments reflected ventrolateral rather that ventromedial damage (24). Lesion studies therefore provide little evidence for a causal role of the vmPFC in hedonic experience. This is consistent with the idea that the vmPFC is involved in “coding” but not in “causing” pleasure (25), in the sense that it encodes a signal that correlates with hedonic experience without underlying this mental experience. However, such a signal that scales with the hedonic experience of reward might constitute a prerequisite to learn to anticipate future rewards and later guide decision-making.
Anticipated values signals reflect the prediction of the experienced value associated with the different options under consideration. These signals have been observed in functional imaging studies in the VS, the OFC and the vmPFC (26–29). During value learning, anticipated value is thought to be dynamically updated based on “reward prediction error” (RPE) signals. Prediction errors measure deviations from individuals’ reward expectancies: they are positive when experienced value is greater than anticipated, and negative otherwise. Such RPE signals have been identified in midbrain dopaminergic neurons and have also been consistently measured in the VS, to which these neurons project (30–32). Hence, dopaminergic projections could facilitate value learning by gating plasticity between sensory information and anticipated value representations in the VS and the vmPFC. To establish the causal role of the vmPFC in supporting value learning and anticipation, lesion studies have used different learning paradigms.
The critical role of the vmPFC in learning the rewarding outcome associated with a stimulus was first suggested after seminal work that employed the Iowa gambling task [IGT, Box 1; (33)]. Poor performance in the IGT, however, is not specific to vmPFC damage (34–36). Performance in the IGT is also difficult to interpret, in part because the task involves both deterministic and probabilistic aspects, meaning that stimuli may predict rewarding outcomes either with certainty or a certain degree of probability, as well as apparent reversals in stimulus-value contingencies (37). In tasks involving only deterministic associations between stimuli and reward values, damage to the vmPFC was not found to significantly impair reward learning (38). Tasks involving probabilistic contingencies demonstrated inconsistent impairments and suggested that bilateral vmPFC lesions may be required to reliably affect reward learning (39–42). Furthermore, the vmPFC was shown to be specifically critical for learning the value of stimuli but not for learning the value of actions (39, 43). Instead, successful action-value learning was found to depend on the integrity of the dmPFC, although the vmPFC may be necessary for awareness about action-value relationships (39, 43). In a changing environment, stimulus-value associations can evolve over time, for example when discovering that a previously liked food is toxic, requiring individuals to update the learned value. Deficits in reversal learning tasks have been demonstrated consistently after vmPFC injury (21, 38–40, 42, 44) [but (see 41)]. Moreover, reward value can also change as a result of a change in individuals’ internal states, for example when satiated with a particular food. In devaluation tasks, patients with focal damage to the vmPFC demonstrated impaired devaluation, persisting in selecting conditioned stimuli associated with food that had been devaluated through selective satiation (45). Consistent evidence therefore supports the causal role of the vmPFC in the flexible updating of anticipated or learned reward values, which can then be used to guide behavior and decision-making.
Decision values signals are thought to measure the difference between the considered option value and another option value, and are used to guide decisions toward the option with the largest benefit. They rely on the net value of options, integrating the anticipated values and costs of each option. Functional imaging studies have identified such value signals at the time of decision in a network of regions, including the vmPFC, during simple binary decisions, for example when choosing between two different food items, drinks, monetary rewards, products, artworks, etc. (12, 13). Decision value signals in the vmPFC have also been shown to integrate the different costs, such as the delay, the effort, or the uncertainty associated with the options of a choice (27, 46–48). The vmPFC has, therefore, been hypothesized to be critical for economic rationality and utility maximization. Damage to the vmPFC has been shown to induce choice inconsistency and choice intransitivity. Choice inconsistency consists in not choosing the same option during repeated choices, or not choosing the option that was given the best rating. Choice intransitivity consists in choosing A over B, B over C, but C over A (39, 49, 50) although not always (51). Yet, when present (but (see 51)), these effects are small and vmPFC patients can still make decisions readily. This led to the hypothesis that the vmPFC may not be necessary for rational decision-making per se, but rather may promote preference stability by reducing variability in valuation across time (52). In repeated choices, individuals with vmPFC damage express preferences that are indeed more variable, but fundamentally transitive (52). Therefore, vmPFC may not be the only critical structure supporting rational value-based choices, which may rely instead on distributed areas, including the VS, that can compensate for damage in the vmPFC (52). Increased choice variability may also participate to explain inconsistent results observed in tasks that assess cost-related preferences. In intertemporal choices that involve choosing between smaller immediate rewards and larger delayed rewards, some studies reported greater temporal discounting of delayed rewards after vmPFC damage (53–57), while others reported no difference compared to controls (58, 59). Similarly, lesions to the vmPFC have been suggested to affect decision-making under uncertainty. Yet, although increased risk-taking has been reported in vmPFC patients (54, 56, 60–62), some studies found no effect (24, 59), one study reported higher risk-taking in the loss-domain but lower risk-taking in the gain-domain (63), and another found increased risk-taking only while receiving dynamic feedback (64). Little data exist on the impact of vmPFC damage in humans on effort- and reward-based decision-making, that involve deciding to make an effort to obtain a reward, but preliminary results suggests that vmPFC may not be critical for such decisions (65). In closely related incentive motivation tasks, that involve effectively producing effort to obtain a reward, damage to the vmPFC reduced the vigor of effort (saccade velocity) produced in response to different reward levels (66). However, here again, the magnitude of this deficit was moderate, in particular in comparison to that observed after lesion to the ventral striato-pallidal complex which completely abolishes effort modulation (67, 68). This series of lesion studies therefore suggest that, at the time of decision or action, the vmPFC may not be critical, but may play a modulating role in shaping value-based behavior. Interestingly, recent findings on valuation about multidimensional options could shed light on the specific role of the vmPFC in decision-making. When value has to be inferred from the multiple attributes of a stimulus, vmPFC patients differ from prefrontal lesioned and healthy control individuals in how they weight the different attributes in certain choices, for example when choosing artworks based on their perceptual, conceptual and affective characteristics (69), but not in other, for example when choosing potential houses based on their features (70). Recent work suggested that vmPFC damage might specifically affect decision-making when value must be inferred from the unique combination of attributes, in other words their interaction, and not when value can be inferred from the sum of independent attributes (71). Such a role could potentially account for why vmPFC patients are not incapable of making choices, but systematically deviate from healthy individuals in their decisions.
Beyond its role in economic valuation, the vmPFC has been implicated early in shaping social behavior. In the 1970s and 1980s, the terms “pseudopsychopathy” (72) and “acquired sociopathy” (2) were coined to describe the dramatic personality and behavioral changes in the social realm observed in patients with vmPFC damage: blunted affect, lack of empathy, poor tolerance to frustration and irritability, social inappropriateness and antisocial behaviors (73–78). However, the precise cognitive mechanisms underlying these social deficits have remained elusive. In the following sections, we review the lesion studies that have examined the role of the vmPFC in supporting social value signals and which may support the “extended common neural currency” model. To simplify the wide range of contexts and decision types encountered in these studies, we group them into three classes of social values: the value that one assigns to other individuals, the value of outcomes that benefit others, and the value of outcomes that conform to social norms (11).
Most people tend to pursue social interactions that offer some form of gratification or benefit. The first class of social valuation therefore concerns situations in which individuals assess the personal value of another person, for example when judging the attractiveness or trustworthiness of a person, or situations in which they assess the value of another person’s actions to themselves, for example when being applauded by someone or when having trust reciprocated by someone. Functional imaging studies have found neural activation in the vmPFC when receiving, or anticipating rewarding social outcomes, such as viewing faces with positive affects (79), attractive faces (80), erotic photos (81), or when receiving social approval or romantic interest from others (82–84). Neural activity in the vmPFC also predicts subsequent decisions about liked others, such as the willingness to pay to view attractive faces (85) or to donate to preferred charities (86). BOLD activity in the vmPFC is also associated with learning about moral values of others, such as their honesty and trustworthiness (87, 88) and with the willingness to reciprocate trust (89). Consistent with imaging studies, lesion studies support the causal involvement of the vmPFC in the valuation of others. First, vmPFC damage reduces the tendency to approach positive and avoid negative emotional faces, particularly for negative affect, while preserving the ability to recognize facial expressions (90–92). Similarly, patients with vmPFC lesions have lower inter-personal disgust, showing less reluctance to interact with unsavory others or with individuals described as socially deviant (93). They also show lower consistency when choosing between potential spouses, based on non-physical attributes (94). Additionally, vmPFC damage affects social judgment about others, decreasing for example the perceived trustworthiness of unknown individuals (95, 96), and impairing the ability to revise these judgments based on the individuals’ observed social and moral conducts (97). These findings demonstrate consistent impairment in behaving according to the value attached to other individuals after damage to the vmPFC.
People are not always driven by their own self-interest and frequently take into account the well-being of others. Thus, a second class of social value signals correspond to the vicarious valuation of outcomes that are rewarding for others, adopting their perspective, for example when rejoicing in someone’s victory, or when choosing to cook for someone his or her favorite food. Empirical studies have found that experiencing reward when directly receiving positive outcomes, or when observing others receiving such outcomes, activated the same regions of the brain valuation system, in particular the vmPFC (98). Experiencing reward for others also consistently recruit the VS (99, 100), although this structure may be preferentially activated in response to personal as compared to vicarious reward (98). Importantly, vicarious value signals in the vmPFC have been shown to predict choices during decisions that result in benefits for others, such as purchasing DVD movies or selecting monetary rewards for others (101–103). Additionally, the vmPFC encodes reward prediction error signals that support learning about another person’s preferences (104, 105). By contrast, bilateral damage to the vmPFC reduces empathic tendencies toward others in clinical questionnaires (76). In economic games, such as the dictator and the ultimatum game (Box 1), patients with focal vmPFC lesions showed impaired concern about payoff to others, giving less money to anonymous strangers (95), even to individuals who are suffering (106). In the trust game (Box 1), when they are endowed from an investor with a sum of money that is later tripled, patients with vmPFC damage make lower back transfer to the investor than healthy controls (96). Taken together, these results therefore provide support for a critical role of the vmPFC in other-regarding preferences. Empathy, the ability to share another person’s feelings, has been conceived as an initial step that can motivate such other-regarding motivation, or sympathy, and is considered one of the fundamental motives driving altruistic acts, which entail personal costs for the benefit of others (107).
People’s behavior is not solely determined by their own interest or by the interest of specific others, but is also shaped by the collective welfare. A third class of social value signals therefore consists in the valuation of options or outcomes according to their conformity with normative social principles, for example when rejoicing in a fair distribution, or when turning down a bribe. Functional imaging studies have consistently reported neural value signals in reward-related brain regions in relation to social principles, such as fairness, cooperation or morality. For example, in economic exchange tasks such as the ultimatum game (Box 1), a fair distribution of money among players is perceived as rewarding and is associated with neural activation in the ventral striatum and vmPFC (108, 109). By contrast, inequality has been associated with activation of neural networks involved in conflict and aversive outcomes, including dorsal ACC and anterior insula (110). When given the opportunity, people tend to punish norm violators who propose unfair distributions, even when this is costly for them (111). Punishing defectors is thought to promote social norms enforcement (112), and is perceived as rewarding and elicits activation in the vmPFC (111). Another example of social principle eliciting neural value signals is cooperation. Research using the Prisoner’s Dilemma game (Box 1) has shown that mutual cooperation is associated with increased activation in reward-related brain regions, including the vmPFC (113). These results have been proposed as evidence of the intrinsic value of cooperation, which can motivate individuals to engage in prosocial behavior and collective action. Finally, value signals have also been reported in the vmPFC during moral dilemmas (Box 1), for example when judging the moral acceptability of sacrificing a single life to save a larger group of dying (114). These findings suggest that normative social principles have inherent values that are encoded in the vmPFC. Damage to this region may therefore impact on how such principles shape human behavior. Lesion studies have, however, provided conflicting results on the antisocial or prosocial effects of vmPFC damage. In the ultimatum game (Box 1), vmPFC patients were initially reported to reject unfair offers at a higher rate than healthy controls, although showing normal levels of anger following unfair offers (95, 115, 116). These patients would also demand, as responders, the same amount that they offered as proposers, whereas controls generally offer more than they demand (95). This result has been interpreted as reflecting an insensitivity to guilt in vmPFC patients, defined as the aversion for advantageous inequity (95). This finding was replicated in another study, but only when monetary gains were presented as abstract amounts to be received later (117). When offers were readily available in cash, vmPFC patients showed normal rejection rate of unfair offers. Inconsistently, a recent study found that vmPFC patients showed diminished sensitivity to unfairness and were more willing to accept unfair offers than control participants (118). Research on the impact of vmPFC damage on cooperation is more limited, but one study reported that vmPFC patients were more likely to cooperate in a public good game (Box 1), with the opposite being true for dlPFC patients who cooperated less than control patients (94). These preliminary results challenge the view that vmPFC is a necessary component for cooperative behavior. By contrast, the impact of vmPFC damage on moral judgments is well-established. In moral dilemmas that involve causing the death of one person to save several lives, patients with vmPFC damage are more likely to choose the utilitarian option, that consists in sacrificing one person, than control individuals (116, 119–122). Although early works suggested that vmPFC patients might be especially impaired in personal versus impersonal moral dilemmas, when directly versus indirectly causing harm, further work showed that they endorsed utilitarian actions more often than healthy individuals, regardless of the situation (122). This utilitarian behavior has been interpreted as reflecting a lack of automatic affective response to moral transgressions in vmPFC patients, which was supported by the absence of autonomic skin response prior to such violations (120). Consistently, when judging the morality of actions made by others, vmPFC patients relied more on the outcome of the actions (i.e., whether they were harmful or not) than on the intention with which the actions were pursued (i.e., whether they were intentional or accidental), suggesting that vmPFC is necessary for integrating both intention and outcome into a moral value (123, 124). Although the impact of damage to the vmPFC on decision-making based on social principles, such as fairness, cooperation and morality, is often reported, it is not yet fully understood why such damage can result in both prosocial and antisocial behaviors.
Most of the findings discussed above support the notion that the vmPFC represents neural value signals both in social and non-social contexts, consistent with the “extended common neural currency” hypothesis. However, the extent to which the vmPFC is critical for value processing may depend on the specific stage of the decision-making process or the nature of the rewarding outcome. In the economic domain, while the vmPFC may not be strictly necessary for experiencing the hedonic value of rewards, its integrity seems essential for learning and flexibly updating reward value. By contrast, empirical studies have produced mixed results regarding the effects of vmPFC damage on choices: some studies demonstrated deficits or biases that were moderate or restricted to certain types of decisions, while others showed preserved abilities to make consistent choices. In the social domain, lesion studies support the critical role of the vmPFC in assigning a subjective value to other individuals. They also support its necessary role in vicariously representing the benefits obtained by others. Damage to the vmPFC also demonstrated its critical role in moral judgments but showed inconsistent deficits in decision-making based on other normative social principles such as cooperation and fairness. The inconsistent effects of vmPFC’s lesions on choices in certain social and non-social contexts suggest that the vmPFC may exert a modulatory rather than a necessary role at the time of decision. Alternatively, it is possible that the vmPFC is involved in certain types of valuation more specifically, for example when an option’s value can only be determined by the interaction between its attributes and not by attributes independently (71).
The common involvement of the vmPFC in both social and non-social contexts raises the question of whether valuation in these contexts relies on the same neurons, or on distinct sub-regions or populations of neurons within the vmPFC. Meta-analyses have suggested a possible posterior-to-anterior gradient of value representations corresponding to concrete-to-abstract rewards (13). Yet, only few imaging studies have directly compared social and non-social value signals using the same experimental design (81, 104, 125–127). One of these studies observed a ventral-to-dorsal gradient in the processing of self- vs. other-regarding value signals (127). While lesion studies do not provide the necessary anatomical specificity to dissociate the roles of distinct areas within the vmPFC, single-unit recordings studies have started to identified neurons that may selectively encode social vs. non-social aspects of rewards both in non-human primates (128, 129) and in humans (130). However, it is worth noting that a clear distinction between social and non-social decisions can be subject to debate. Many decisions in people’s lives, which may not involve another person at first glance, may, in fact, carry a social component. Consider, for example, the strong social influence that can impact the value people assign to material goods, such as when they decide to eat a vegetarian meal or buy a luxury car. Further works will help clarify whether the valuation of stimuli associated with social versus non-social contexts is implemented by common or overlapping but specialized neuronal populations.
The inconsistent effects observed after damage to the vmPFC, both in social and non-social decision-making, also question the influence of the heterogeneity of lesions across patients (Figure 2). A first factor that may contribute to these inconsistencies is the variability in the nature of the lesions across different studies (Figure 2). Ischemic, hemorrhagic, traumatic, or surgical causes can lead to different distributions of lesions and varying degrees of functional impairment. Samples consisting primarily of orbital meningioma cases involve more bilateral and anterior lesions, while vascular lesions often concern more posterior and unilateral lesions (14). Moreover, although surgical resection of brain tumors can lead to a greater degree of functional impairment compared to vascular lesions or traumatic brain injuries, the slow progression of tissue damage, as the tumor expands, may allow for brain plasticity and functional compensation over time (131). The age of lesion onset may also significantly influence the degree of functional impairment. Early-onset lesions that impact neurodevelopment tend to lead to more pronounced deficits (132–134). The age of lesion onset may also interact with changes in brain plasticity across the lifespan, and thus condition functional recovery. Thus, an important guideline for future studies would be to report the nature and the location of individual lesions, as well as the age of lesion onset when describing vmPFC patients (14). Finally, the variability in the impact of vmPFC damage raises the question of possible hemispheric specialization or functional redundancy between left and right vmPFC. Bilateral lesions in the vmPFC are rare and most of the patients included in the lesion studies mentioned above had unilateral lesions (Figure 2). Thus, it remains possible that compensation from the preserved vmPFC masked the effects of unilateral vmPFC damage. However, the limited sample size of vmPFC studies, which classically include only a tenth of patients or fewer, hinder the ability to compare the effects of bilateral and unilateral damage to the right or left vmPFC. Small samples also limit our ability to investigate deficits that arise from the interaction of lesions in the vmPFC and another region. Therefore, future research should aim to include larger samples of patients, possibly through multicenter collaboration, with a sufficient number of bilateral lesions, to investigate the potential effects of laterality, redundancy, and interactions following vmPFC damage.
Figure 2. Heterogeneity of vmPFC lesions in human studies. (A) Etiology of vmPFC lesions among cited studies with individual lesion data. (B) Location of lesions among cited studies with individual lesion data. Each column represents a patient. Green and red rectangles represent preserved and damaged areas, respectively. Brain areas are represented from top to bottom in the following order: right dmPFC/ACC, right vmPFC, right OFC, left OFC, left vmPFC, left dmPFC/ACC. SAH, subarachnoid hemorrhage. TBI, Traumatic brain injury. dmPFC, dorsomedial prefrontal cortex. ACC, anterior cingulate cortex. vmPFC, ventromedial prefrontal cortex. OFC, orbitofrontal cortex.
Another question that remains is how specific the role of the vmPFC is in social and non-social decision-making, as such complex behaviors are likely to involve a network of multiple brain regions. While lesion studies shed light on the contribution of the vmPFC, few of them directly compared the effects of damage to the vmPFC to damage in other parts of the prefrontal cortex. Therefore, including control patients with prefrontal lesions outside the vmPFC in future studies would be essential to gaining a more comprehensive understanding of the unique role of the vmPFC in decision-making. Moreover, there is also evidence that the vmPFC receives specialized inputs from specific brain regions when constructing values in social and non-social contexts. For example, structures outside the classical reward circuitry and typically associated with social cognition, such as the dmPFC, dlPFC and TPJ, have been shown to be preferentially engaged in response to vicarious as compared to personal reward (98), and are thought to provide information that are relevant for the construction of social values through their connectivity with the vmPFC (11, 86). Further research is needed to provide a more comprehensive understanding of how remote cortical areas provide inputs for the computation of values in both social and non-social contexts. Additionally, investigating the impact of lesions in these structures and in the subcortical and white matter pathways that convey specific information to the vmPFC could shed light on diverse biases in decision-making.
In this review, we hope to have summarized the evidence supporting the notion that the vmPFC encodes value signals at different stages of the decision-making process, when receiving, learning and deciding about valued outcomes, both in the economic domain and in various social contexts, for example when valuating other individuals, others’ benefit, or social normative principles. In the modern era, non-invasive brain stimulation techniques, such as Transcranial Magnetic Simulation (TMS) and Transcranial Direct Current Stimulation (tdCS), are classically used to demonstrate causality in brain functions. Yet, these methods can hardly modulate neural activity in subcortical structures or medial cortical areas as they primarily target brain areas at the cortical surface. Here, we have therefore also tried to emphasize the invaluable contribution of lesion studies in establishing or challenging causal brain-behavior relationships, particularly in reward-related brain areas, like the vmPFC or the ventral striatum. Overall, neuroimaging and lesion studies support an “extended common neural currency” schema, where the vmPFC serves as key motivational node that shapes both social and non-social human behaviors, but flexibly integrates inputs specific to the decision-making context.
RB wrote the first draft of the manuscript. RB and DM wrote sections of the manuscript. All authors contributed to manuscript revision, read, and approved the submitted version.
DM was supported by a research grant from the Fondation pour la Recherche Médicale.
The authors declare that the research was conducted in the absence of any commercial or financial relationships that could be construed as a potential conflict of interest.
All claims expressed in this article are solely those of the authors and do not necessarily represent those of their affiliated organizations, or those of the publisher, the editors and the reviewers. Any product that may be evaluated in this article, or claim that may be made by its manufacturer, is not guaranteed or endorsed by the publisher.
1. Damasio, H, Grabowski, T, Frank, R, Galaburda, AM, and Damasio, AR. The return of Phineas Gage: clues about the brain from the skull of a famous patient. Science. (1994) 264:1102–5. doi: 10.1126/science.8178168
2. Eslinger, PJ, and Damasio, AR. Severe disturbance of higher cognition after bilateral frontal lobe ablation: patient EVR. Neurology. (1985) 35:1731–41. doi: 10.1212/WNL.35.12.1731
3. Harlow, JM. Recovery from the passage of an iron bar through the head. Hist Psychiatry. (1993) 4:274–81. doi: 10.1177/0957154X9300401407
4. Harlow, JM. Passage of an iron rod through the head. J Neuropsychiatry Clin Neurosci. (1999) 11:281–3. doi: 10.1176/jnp.11.2.281
5. Damasio, A. Descartes’ error: Emotion, rationality and the human brain. New York: Putnam (1994). 352 p.
6. Roy, M, Shohamy, D, and Wager, TD. Ventromedial prefrontal-subcortical systems and the generation of affective meaning. Trends Cogn Sci. (2012) 16:147–56. doi: 10.1016/j.tics.2012.01.005
7. Niv, Y. Learning task-state representations. Nat Neurosci. (2019) 22:1544–53. doi: 10.1038/s41593-019-0470-8
8. Schoenbaum, G, Takahashi, Y, Liu, TL, and McDannald, MA. Does the orbitofrontal cortex signal value? Ann N Y Acad Sci. (2011) 1239:87–99. doi: 10.1111/j.1749-6632.2011.06210.x
9. Schuck, N. W., Wilson, R., and Niv, Y. (2018). A state representation for reinforcement learning and decision-making in the orbitofrontal cortex goal-directed decision making (pp. 259–278): Elsevier.
10. Wilson, RC, Takahashi, YK, Schoenbaum, G, and Niv, Y. Orbitofrontal cortex as a cognitive map of task space. Neuron. (2014) 81:267–79. doi: 10.1016/j.neuron.2013.11.005
11. Ruff, CC, and Fehr, E. The neurobiology of rewards and values in social decision making. Nat Rev Neurosci. (2014) 15:549–62. doi: 10.1038/nrn3776
12. Bartra, O, McGuire, JT, and Kable, JW. The valuation system: a coordinate-based meta-analysis of BOLD fMRI experiments examining neural correlates of subjective value. NeuroImage. (2013) 76:412–27. doi: 10.1016/j.neuroimage.2013.02.063
13. Clithero, JA, and Rangel, A. Informatic parcellation of the network involved in the computation of subjective value. Soc Cogn Affect Neurosci. (2014) 9:1289–302. doi: 10.1093/scan/nst106
14. Schneider, B, and Koenigs, M. Human lesion studies of ventromedial prefrontal cortex. Neuropsychologia. (2017) 107:84–93. doi: 10.1016/j.neuropsychologia.2017.09.035
15. Yu, LQ, Kan, IP, and Kable, JW. Beyond a rod through the skull: a systematic review of lesion studies of the human ventromedial frontal lobe. Cogn Neuropsychol. (2020) 37:97–141. doi: 10.1080/02643294.2019.1690981
16. Mackey, S, and Petrides, M. Architecture and morphology of the human ventromedial prefrontal cortex. Eur J Neurosci. (2014) 40:2777–96. doi: 10.1111/ejn.12654
17. Chib, VS, Rangel, A, Shimojo, S, and O'Doherty, JP. Evidence for a common representation of decision values for dissimilar goods in human ventromedial prefrontal cortex. J Neurosci. (2009) 29:12315–20. doi: 10.1523/JNEUROSCI.2575-09.2009
18. Lebreton, M, Jorge, S, Michel, V, Thirion, B, and Pessiglione, M. An automatic valuation system in the human brain: evidence from functional neuroimaging. Neuron. (2009) 64:431–9. doi: 10.1016/j.neuron.2009.09.040
19. Lopez-Persem, A, Bastin, J, Petton, M, Abitbol, R, Lehongre, K, Adam, C, et al. Four core properties of the human brain valuation system demonstrated in intracranial signals. Nat Neurosci. (2020) 23:664–75. doi: 10.1038/s41593-020-0615-9
20. Sescousse, G, Caldu, X, Segura, B, and Dreher, JC. Processing of primary and secondary rewards: a quantitative meta-analysis and review of human functional neuroimaging studies. Neurosci Biobehav Rev. (2013) 37:681–96. doi: 10.1016/j.neubiorev.2013.02.002
21. Berlin, H, Rolls, ET, and Kischka, U. Impulsivity, time perception, emotion and reinforcement sensitivity in patients with orbitofrontal cortex lesions. Brain. (2004) 127:1108–26. doi: 10.1093/brain/awh135
22. Lewis, JD, Krueger, F, Raymont, V, Solomon, J, Knutson, KM, Barbey, AK, et al. Anhedonia in combat veterans with penetrating head injury. Brain Imaging Behav. (2015) 9:456–60. doi: 10.1007/s11682-015-9414-4
23. Camille, N, Coricelli, G, Sallet, J, Pradat-Diehl, P, Duhamel, J-R, and Sirigu, A. The involvement of the orbitofrontal cortex in the experience of regret. Science. (2004) 304:1167–70. doi: 10.1126/science.1094550
24. Levens, SM, Larsen, JT, Bruss, J, Tranel, D, Bechara, A, and Mellers, BA. What might have been? The role of the ventromedial prefrontal cortex and lateral orbitofrontal cortex in counterfactual emotions and choice. Neuropsychologia. (2014) 54:77–86. doi: 10.1016/j.neuropsychologia.2013.10.026
25. Berridge, KC, and Kringelbach, ML. Building a neuroscience of pleasure and well-being. Psychol Well-Being. (2011) 1:3–26. doi: 10.1186/2211-1522-1-3
26. Hare, TA, O'Doherty, J, Camerer, CF, Schultz, W, and Rangel, A. Dissociating the role of the orbitofrontal cortex and the striatum in the computation of goal values and prediction errors. J Neurosci. (2008) 28:5623–30. doi: 10.1523/JNEUROSCI.1309-08.2008
27. Kable, JW, and Glimcher, PW. The neural correlates of subjective value during intertemporal choice. Nat Neurosci. (2007) 10:1625–33. doi: 10.1038/nn2007
28. Knutson, B, Fong, GW, Adams, CM, Varner, JL, and Hommer, D. Dissociation of reward anticipation and outcome with event-related fMRI. Neuroreport. (2001) 12:3683–7. doi: 10.1097/00001756-200112040-00016
29. Plassmann, H, O'Doherty, J, and Rangel, A. Orbitofrontal cortex encodes willingness to pay in everyday economic transactions. J Neurosci. (2007) 27:9984–8. doi: 10.1523/JNEUROSCI.2131-07.2007
30. McClure, SM, Berns, GS, and Montague, PR. Temporal prediction errors in a passive learning task activate human striatum. Neuron. (2003) 38:339–46. doi: 10.1016/S0896-6273(03)00154-5
31. O'Doherty, JP, Dayan, P, Friston, K, Critchley, H, and Dolan, RJ. Temporal difference models and reward-related learning in the human brain. Neuron. (2003) 38:329–37. doi: 10.1016/S0896-6273(03)00169-7
32. Schultz, W, Dayan, P, and Montague, PR. A neural substrate of prediction and reward. Science. (1997) 275:1593–9. doi: 10.1126/science.275.5306.1593
33. Bechara, A, Damasio, AR, Damasio, H, and Anderson, SW. Insensitivity to future consequences following damage to human prefrontal cortex. Cognition. (1994) 50:7–15. doi: 10.1016/0010-0277(94)90018-3
34. Fellows, LK, and Farah, MJ. Different underlying impairments in decision-making following ventromedial and dorsolateral frontal lobe damage in humans. Cereb Cortex. (2005) 15:58–63. doi: 10.1093/cercor/bhh108
35. Gläscher, J, Adolphs, R, Damasio, H, Bechara, A, Rudrauf, D, Calamia, M, et al. Lesion mapping of cognitive control and value-based decision making in the prefrontal cortex. Proc Natl Acad Sci. (2012) 109:14681–6. doi: 10.1073/pnas.1206608109
36. Ouerchefani, R, Ouerchefani, N, Allain, P, Rejeb, MRB, and Le Gall, D. Contribution of different regions of the prefrontal cortex and lesion laterality to deficit of decision-making on the Iowa gambling task. Brain Cogn. (2017) 111:73–85. doi: 10.1016/j.bandc.2016.06.010
37. Dunn, BD, Dalgleish, T, and Lawrence, AD. The somatic marker hypothesis: a critical evaluation. Neurosci Biobehav Rev. (2006) 30:239–71. doi: 10.1016/j.neubiorev.2005.07.001
38. Fellows, LK, and Farah, MJ. Ventromedial frontal cortex mediates affective shifting in humans: evidence from a reversal learning paradigm. Brain. (2003) 126:1830–7. doi: 10.1093/brain/awg180
39. Camille, N, Tsuchida, A, and Fellows, LK. Double dissociation of stimulus-value and action-value learning in humans with orbitofrontal or anterior cingulate cortex damage. J Neurosci. (2011) 31:15048–52. doi: 10.1523/JNEUROSCI.3164-11.2011
40. Hornak, J, O'Doherty, J, Bramham, J, Rolls, ET, Morris, RG, Bullock, PR, et al. Reward-related reversal learning after surgical excisions in orbito-frontal or dorsolateral prefrontal cortex in humans. J Cogn Neurosci. (2004) 16:463–78. doi: 10.1162/089892904322926791
41. Kumaran, D, Warren, DE, and Tranel, D. Damage to the ventromedial prefrontal cortex impairs learning from observed outcomes. Cereb Cortex. (2015) 25:4504–18. doi: 10.1093/cercor/bhv080
42. Tsuchida, A, Doll, BB, and Fellows, LK. Beyond reversal: a critical role for human orbitofrontal cortex in flexible learning from probabilistic feedback. J Neurosci. (2010) 30:16868–75. doi: 10.1523/JNEUROSCI.1958-10.2010
43. O’Callaghan, C, Vaghi, MM, Brummerloh, B, Cardinal, RN, and Robbins, TW. Impaired awareness of action-outcome contingency and causality during healthy ageing and following ventromedial prefrontal cortex lesions. Neuropsychologia. (2019) 128:282–9. doi: 10.1016/j.neuropsychologia.2018.01.021
44. Berlin, HA, Rolls, ET, and Iversen, SD. Borderline personality disorder, impulsivity, and the orbitofrontal cortex. Am J Psychiatr. (2005) 162:2360–73. doi: 10.1176/appi.ajp.162.12.2360
45. Reber, J, Feinstein, JS, O’Doherty, JP, Liljeholm, M, Adolphs, R, and Tranel, D. Selective impairment of goal-directed decision-making following lesions to the human ventromedial prefrontal cortex. Brain. (2017) 140:1743–56. doi: 10.1093/brain/awx105
46. Le Bouc, R, and Pessiglione, M. A neuro-computational account of procrastination behavior. Nat Commun. (2022) 13:5639. doi: 10.1038/s41467-022-33119-w
47. Levy, DJ, and Glimcher, PW. The root of all value: a neural common currency for choice. Curr Opin Neurobiol. (2012) 22:1027–38. doi: 10.1016/j.conb.2012.06.001
48. Lopez-Gamundi, P, Yao, Y-W, Chong, TT, Heekeren, HR, Mas-Herrero, E, and Marco-Pallarés, J. The neural basis of effort valuation: a meta-analysis of functional magnetic resonance imaging studies. Neurosci Biobehav Rev. (2021) 131:1275–87. doi: 10.1016/j.neubiorev.2021.10.024
49. Fellows, LK, and Farah, MJ. The role of ventromedial prefrontal cortex in decision making: judgment under uncertainty or judgment per se? Cereb Cortex. (2007) 17:2669–74. doi: 10.1093/cercor/bhl176
50. Henri-Bhargava, A, Simioni, A, and Fellows, LK. Ventromedial frontal lobe damage disrupts the accuracy, but not the speed, of value-based preference judgments. Neuropsychologia. (2012) 50:1536–42. doi: 10.1016/j.neuropsychologia.2012.03.006
51. Vaidya, AR, and Fellows, LK. Testing necessary regional frontal contributions to value assessment and fixation-based updating. Nat Commun. (2015) 6:10120. doi: 10.1038/ncomms10120
52. Yu, LQ, Dana, J, and Kable, JW. Individuals with ventromedial frontal damage display unstable but transitive preferences during decision making. Nat Commun. (2022) 13:4758. doi: 10.1038/s41467-022-32511-w
53. Ciaramelli, E, De Luca, F, Kwan, D, Mok, J, Bianconi, F, Knyagnytska, V, et al. The role of ventromedial prefrontal cortex in reward valuation and future thinking during intertemporal choice. elife. (2021) 10:e67387. doi: 10.7554/eLife.67387
54. Mok, JN, Green, L, Myerson, J, Kwan, D, Kurczek, J, Ciaramelli, E, et al. Does ventromedial prefrontal cortex damage really increase impulsiveness? Delay and probability discounting in patients with focal lesions. J Cogn Neurosci. (2021) 33:1909–27. doi: 10.1162/jocn_a_01721
55. Peters, J, and D’Esposito, M. Effects of medial orbitofrontal cortex lesions on self-control in intertemporal choice. Curr Biol. (2016) 26:2625–8. doi: 10.1016/j.cub.2016.07.035
56. Peters, J, and D’Esposito, M. The drift diffusion model as the choice rule in inter-temporal and risky choice: a case study in medial orbitofrontal cortex lesion patients and controls. PLoS Comput Biol. (2020) 16:e1007615. doi: 10.1371/journal.pcbi.1007615
57. Sellitto, M, Ciaramelli, E, and di Pellegrino, G. Myopic discounting of future rewards after medial orbitofrontal damage in humans. J Neurosci. (2010) 30:16429–36. doi: 10.1523/JNEUROSCI.2516-10.2010
58. Fellows, LK, and Farah, MJ. Dissociable elements of human foresight: a role for the ventromedial frontal lobes in framing the future, but not in discounting future rewards. Neuropsychologia. (2005) 43:1214–21. doi: 10.1016/j.neuropsychologia.2004.07.018
59. Leland, JW, and Grafman, J. Experimental tests of the somatic marker hypothesis. Games Econ Behav. (2005) 52:386–409. doi: 10.1016/j.geb.2004.09.001
60. Hsu, M, Bhatt, M, Adolphs, R, Tranel, D, and Camerer, CF. Neural systems responding to degrees of uncertainty in human decision-making. Science. (2005) 310:1680–3. doi: 10.1126/science.1115327
61. Studer, B, Manes, F, Humphreys, G, Robbins, TW, and Clark, L. Risk-sensitive decision-making in patients with posterior parietal and ventromedial prefrontal cortex injury. Cereb Cortex. (2015) 25:1–9. doi: 10.1093/cercor/bht197
62. Weller, JA, Levin, IP, Shiv, B, and Bechara, A. Neural correlates of adaptive decision making for risky gains and losses. Psychol Sci. (2007) 18:958–64. doi: 10.1111/j.1467-9280.2007.02009.x
63. Pujara, MS, Wolf, RC, Baskaya, MK, and Koenigs, M. Ventromedial prefrontal cortex damage alters relative risk tolerance for prospective gains and losses. Neuropsychologia. (2015) 79:70–5. doi: 10.1016/j.neuropsychologia.2015.10.026
64. Spaniol, J, Di Muro, F, and Ciaramelli, E. Differential impact of ventromedial prefrontal cortex damage on “hot” and “cold” decisions under risk. Cogn Affect Behav Neurosci. (2019) 19:477–89. doi: 10.3758/s13415-018-00680-1
65. Löffler, A. Worth the effort? Measuring component processes of effort-based decision making in lesion patients. Canada: McGill University (2015).
66. Manohar, SG, and Husain, M. Human ventromedial prefrontal lesions alter incentivisation by reward. Cortex. (2016) 76:104–20. doi: 10.1016/j.cortex.2016.01.005
67. Adam, R, Leff, A, Sinha, N, Turner, C, Bays, P, Draganski, B, et al. Dopamine reverses reward insensitivity in apathy following globus pallidus lesions. Cortex. (2013) 49:1292–303. doi: 10.1016/j.cortex.2012.04.013
68. Schmidt, L, d'Arc, BF, Lafargue, G, Galanaud, D, Czernecki, V, Grabli, D, et al. Disconnecting force from money: effects of basal ganglia damage on incentive motivation. Brain. (2008) 131:1303–10. doi: 10.1093/brain/awn045
69. Vaidya, AR, Sefranek, M, and Fellows, LK. Ventromedial frontal lobe damage alters how specific attributes are weighed in subjective valuation. Cereb Cortex. (2018) 28:3857–67. doi: 10.1093/cercor/bhx246
70. Bowren, MD, Croft, KE, Reber, J, and Tranel, D. Choosing spouses and houses: impaired congruence between preference and choice following damage to the ventromedial prefrontal cortex. Neuropsychology. (2018) 32:280–303. doi: 10.1037/neu0000421
71. Pelletier, G, and Fellows, LK. A critical role for human ventromedial frontal lobe in value comparison of complex objects based on attribute configuration. J Neurosci. (2019) 39:4124–32. doi: 10.1523/JNEUROSCI.2969-18.2019
72. Blumer, D. (1975). Personality changes with frontal and temporal lobe lesions. Benson, DF, and Blumer, D Psychiatric aspects of neurologic disease, New York: Grune & Stratton 1, 151–170.
73. Anderson, SW, Barrash, J, Bechara, A, and Tranel, D. Impairments of emotion and real-world complex behavior following childhood-or adult-onset damage to ventromedial prefrontal cortex. J Int Neuropsychol Soc. (2006) 12:224–35. doi: 10.1017/S1355617706060346
74. Barrash, J, Asp, E, Markon, K, Manzel, K, Anderson, SW, and Tranel, D. Dimensions of personality disturbance after focal brain damage: investigation with the Iowa Scales of Personality Change. J Clin Exp Neuropsychol. (2011) 33:833–52. doi: 10.1080/13803395.2011.561300
75. Barrash, J, Tranel, D, and Anderson, SW. Acquired personality disturbances associated with bilateral damage to the ventromedial prefrontal region. Dev Neuropsychol. (2000) 18:355–81. doi: 10.1207/S1532694205Barrash
76. Bramham, J, Morris, R, Hornak, J, Bullock, P, and Polkey, C. Social and emotional functioning following bilateral and unilateral neurosurgical prefrontal cortex lesions. J Neuropsychol. (2009) 3:125–43. doi: 10.1348/174866408X293994
77. Robinson, H, Calamia, M, Gläscher, J, Bruss, J, and Tranel, D. Neuroanatomical correlates of executive functions: a neuropsychological approach using the EXAMINER battery. J Int Neuropsychol Soc. (2014) 20:52–63. doi: 10.1017/S135561771300060X
78. Trebuchon, A, Bartolomei, F, McGonigal, A, Laguitton, V, and Chauvel, P. Reversible antisocial behavior in ventromedial prefrontal lobe epilepsy. Epilepsy Behav. (2013) 29:367–73. doi: 10.1016/j.yebeh.2013.08.007
79. Lin, A, Adolphs, R, and Rangel, A. Social and monetary reward learning engage overlapping neural substrates. Soc Cogn Affect Neurosci. (2012) 7:274–81. doi: 10.1093/scan/nsr006
80. O’Doherty, J, Winston, J, Critchley, H, Perrett, D, Burt, DM, and Dolan, RJ. Beauty in a smile: the role of medial orbitofrontal cortex in facial attractiveness. Neuropsychologia. (2003) 41:147–55. doi: 10.1016/S0028-3932(02)00145-8
81. Sescousse, G, Redouté, J, and Dreher, J-C. The architecture of reward value coding in the human orbitofrontal cortex. J Neurosci. (2010) 30:13095–104. doi: 10.1523/JNEUROSCI.3501-10.2010
82. Cooper, JC, Dunne, S, Furey, T, and O'Doherty, JP. The role of the posterior temporal and medial prefrontal cortices in mediating learning from romantic interest and rejection. Cereb Cortex. (2014) 24:2502–11. doi: 10.1093/cercor/bht102
83. Davey, CG, Allen, NB, Harrison, BJ, Dwyer, DB, and Yücel, M. Being liked activates primary reward and midline self-related brain regions. Hum Brain Mapp. (2010) 31:660–8. doi: 10.1002/hbm.20895
84. Gunther Moor, B, van Leijenhorst, L, Rombouts, SA, Crone, EA, and Van der Molen, MW. Do you like me? Neural correlates of social evaluation and developmental trajectories. Soc Neurosci. (2010) 5:461–82. doi: 10.1080/17470910903526155
85. Smith, DV, Clithero, JA, Boltuck, SE, and Huettel, SA. Functional connectivity with ventromedial prefrontal cortex reflects subjective value for social rewards. Soc Cogn Affect Neurosci. (2014) 9:2017–25. doi: 10.1093/scan/nsu005
86. Hare, TA, Camerer, CF, Knoepfle, DT, O'Doherty, JP, and Rangel, A. Value computations in ventral medial prefrontal cortex during charitable decision making incorporate input from regions involved in social cognition. J Neurosci. (2010) 30:583–90. doi: 10.1523/JNEUROSCI.4089-09.2010
87. Bellucci, G, Hahn, T, Deshpande, G, and Krueger, F. Functional connectivity of specific resting-state networks predicts trust and reciprocity in the trust game. Cogn Affect Behav Neurosci. (2019) 19:165–76. doi: 10.3758/s13415-018-00654-3
88. Phan, KL, Sripada, CS, Angstadt, M, and McCabe, K. Reputation for reciprocity engages the brain reward center. Proc Natl Acad Sci. (2010) 107:13099–104. doi: 10.1073/pnas.1008137107
89. Li, J, Xiao, E, Houser, D, and Montague, PR. Neural responses to sanction threats in two-party economic exchange. Proc Natl Acad Sci. (2009) 106:16835–40. doi: 10.1073/pnas.0908855106
90. Buades-Rotger, M, Solbakk, A-K, Liebrand, M, Endestad, T, Funderud, I, Siegwardt, P, et al. Patients with ventromedial prefrontal lesions show an implicit approach bias to angry faces. J Cogn Neurosci. (2021) 33:1069–81. doi: 10.1162/jocn_a_01706
91. Willis, ML, Palermo, R, Burke, D, McGrillen, K, and Miller, L. Orbitofrontal cortex lesions result in abnormal social judgements to emotional faces. Neuropsychologia. (2010) 48:2182–7. doi: 10.1016/j.neuropsychologia.2010.04.010
92. Wolf, RC, Philippi, CL, Motzkin, JC, Baskaya, MK, and Koenigs, M. Ventromedial prefrontal cortex mediates visual attention during facial emotion recognition. Brain. (2014) 137:1772–80. doi: 10.1093/brain/awu063
93. Ciaramelli, E, Sperotto, RG, Mattioli, F, and di Pellegrino, G. Damage to the ventromedial prefrontal cortex reduces interpersonal disgust. Soc Cogn Affect Neurosci. (2013) 8:171–80. doi: 10.1093/scan/nss087
94. Wills, J, FeldmanHall, O, Meager, MR, and Van Bavel, JJ, NYU PROSPEC Collaboration. Dissociable contributions of the prefrontal cortex in group-based cooperation. Soc Cogn Affect Neurosci. (2018) 13:349–56. doi: 10.1093/scan/nsy023
95. Krajbich, I, Adolphs, R, Tranel, D, Denburg, NL, and Camerer, CF. Economic games quantify diminished sense of guilt in patients with damage to the prefrontal cortex. J Neurosci. (2009) 29:2188–92. doi: 10.1523/JNEUROSCI.5086-08.2009
96. Moretto, G, Sellitto, M, and di Pellegrino, G. Investment and repayment in a trust game after ventromedial prefrontal damage. Front Hum Neurosci. (2013) 7:593. doi: 10.3389/fnhum.2013.00593
97. Croft, KE, Duff, MC, Kovach, CK, Anderson, SW, Adolphs, R, and Tranel, D. Detestable or marvelous? Neuroanatomical correlates of character judgments. Neuropsychologia. (2010) 48:1789–801. doi: 10.1016/j.neuropsychologia.2010.03.001
98. Morelli, SA, Sacchet, MD, and Zaki, J. Common and distinct neural correlates of personal and vicarious reward: a quantitative meta-analysis. NeuroImage. (2015) 112:244–53. doi: 10.1016/j.neuroimage.2014.12.056
99. Braams, BR, Güroğlu, B, de Water, E, Meuwese, R, Koolschijn, PC, Peper, JS, et al. Reward-related neural responses are dependent on the beneficiary. Soc Cogn Affect Neurosci. (2014) 9:1030–7. doi: 10.1093/scan/nst077
100. Mobbs, D, Yu, R, Meyer, M, Passamonti, L, Seymour, B, Calder, AJ, et al. A key role for similarity in vicarious reward. Science. (2009) 324:900–11. doi: 10.1126/science.1170539
101. Hutcherson, CA, Bushong, B, and Rangel, A. A neurocomputational model of altruistic choice and its implications. Neuron. (2015) 87:451–62. doi: 10.1016/j.neuron.2015.06.031
102. Janowski, V, Camerer, C, and Rangel, A. Empathic choice involves vmPFC value signals that are modulated by social processing implemented in IPL. Soc Cogn Affect Neurosci. (2013) 8:201–8. doi: 10.1093/scan/nsr086
103. Nicolle, A, Klein-Flügge, MC, Hunt, LT, Vlaev, I, Dolan, RJ, and Behrens, TE. An agent independent axis for executed and modeled choice in medial prefrontal cortex. Neuron. (2012) 75:1114–21. doi: 10.1016/j.neuron.2012.07.023
104. Burke, CJ, Tobler, PN, Baddeley, M, and Schultz, W. Neural mechanisms of observational learning. Proc Natl Acad Sci. (2010) 107:14431–6. doi: 10.1073/pnas.1003111107
105. Suzuki, S, Harasawa, N, Ueno, K, Gardner, JL, Ichinohe, N, Haruno, M, et al. Learning to simulate others' decisions. Neuron. (2012) 74:1125–37. doi: 10.1016/j.neuron.2012.04.030
106. Beadle, JN, Paradiso, S, and Tranel, D. Ventromedial prefrontal cortex is critical for helping others who are suffering. Front Neurol. (2018) 9:288. doi: 10.3389/fneur.2018.00288
107. Singer, T., and Tusche, A. (2014). Understanding others: brain mechanisms of theory of mind and empathy neuroeconomics (pp. 513–532): Elsevier.
108. Tabibnia, G, Satpute, AB, and Lieberman, MD. The sunny side of fairness: preference for fairness activates reward circuitry (and disregarding unfairness activates self-control circuitry). Psychol Sci. (2008) 19:339–47. doi: 10.1111/j.1467-9280.2008.02091.x
109. Tricomi, E, Rangel, A, Camerer, CF, and O’Doherty, JP. Neural evidence for inequality-averse social preferences. Nature. (2010) 463:1089–91. doi: 10.1038/nature08785
110. Sanfey, AG, Rilling, JK, Aronson, JA, Nystrom, LE, and Cohen, JD. The neural basis of economic decision-making in the ultimatum game. Science. (2003) 300:1755–8. doi: 10.1126/science.1082976
111. De Quervain, DJ-F, Fischbacher, U, Treyer, V, Schellhammer, M, Schnyder, U, Buck, A, et al. The neural basis of altruistic punishment. Science. (2004) 305:1254–8. doi: 10.1126/science.1100735
112. Fehr, E, and Gächter, S. Altruistic punishment in humans. Nature. (2002) 415:137–40. doi: 10.1038/415137a
113. Rilling, JK, Gutman, DA, Zeh, TR, Pagnoni, G, Berns, GS, and Kilts, CD. A neural basis for social cooperation. Neuron. (2002) 35:395–405. doi: 10.1016/S0896-6273(02)00755-9
114. Shenhav, A, and Greene, JD. Moral judgments recruit domain-general valuation mechanisms to integrate representations of probability and magnitude. Neuron. (2010) 67:667–77. doi: 10.1016/j.neuron.2010.07.020
115. Koenigs, M, Kruepke, M, and Newman, JP. Economic decision-making in psychopathy: a comparison with ventromedial prefrontal lesion patients. Neuropsychologia. (2010) 48:2198–204. doi: 10.1016/j.neuropsychologia.2010.04.012
116. Koenigs, M, and Tranel, D. Irrational economic decision-making after ventromedial prefrontal damage: evidence from the ultimatum game. J Neurosci. (2007) 27:951–6. doi: 10.1523/JNEUROSCI.4606-06.2007
117. Moretti, L, Dragone, D, and Di Pellegrino, G. Reward and social valuation deficits following ventromedial prefrontal damage. J Cogn Neurosci. (2009) 21:128–40. doi: 10.1162/jocn.2009.21011
118. Gu, X, Wang, X, Hula, A, Wang, S, Xu, S, Lohrenz, TM, et al. Necessary, yet dissociable contributions of the insular and ventromedial prefrontal cortices to norm adaptation: computational and lesion evidence in humans. J Neurosci. (2015) 35:467–73. doi: 10.1523/JNEUROSCI.2906-14.2015
119. Ciaramelli, E, Muccioli, M, Làdavas, E, and Di Pellegrino, G. Selective deficit in personal moral judgment following damage to ventromedial prefrontal cortex. Soc Cogn Affect Neurosci. (2007) 2:84–92. doi: 10.1093/scan/nsm001
120. Moretto, G, Làdavas, E, Mattioli, F, and Di Pellegrino, G. A psychophysiological investigation of moral judgment after ventromedial prefrontal damage. J Cogn Neurosci. (2010) 22:1888–99. doi: 10.1162/jocn.2009.21367
121. Taber-Thomas, BC, Asp, EW, Koenigs, M, Sutterer, M, Anderson, SW, and Tranel, D. Arrested development: early prefrontal lesions impair the maturation of moral judgement. Brain. (2014) 137:1254–61. doi: 10.1093/brain/awt377
122. Thomas, BC, Croft, KE, and Tranel, D. Harming kin to save strangers: further evidence for abnormally utilitarian moral judgments after ventromedial prefrontal damage. J Cogn Neurosci. (2011) 23:2186–96. doi: 10.1162/jocn.2010.21591
123. Ciaramelli, E, Braghittoni, D, and di Pellegrino, G. It is the outcome that counts! Damage to the ventromedial prefrontal cortex disrupts the integration of outcome and belief information for moral judgment. J Int Neuropsychol Soc. (2012) 18:962–71. doi: 10.1017/S1355617712000690
124. Young, L, Bechara, A, Tranel, D, Damasio, H, Hauser, M, and Damasio, A. Damage to ventromedial prefrontal cortex impairs judgment of harmful intent. Neuron. (2010) 65:845–51. doi: 10.1016/j.neuron.2010.03.003
125. Behrens, TE, Hunt, LT, Woolrich, MW, and Rushworth, MF. Associative learning of social value. Nature. (2008) 456:245–9. doi: 10.1038/nature07538
126. Smith, DV, Hayden, BY, Truong, T-K, Song, AW, Platt, ML, and Huettel, SA. Distinct value signals in anterior and posterior ventromedial prefrontal cortex. J Neurosci. (2010) 30:2490–5. doi: 10.1523/JNEUROSCI.3319-09.2010
127. Sul, S, Tobler, PN, Hein, G, Leiberg, S, Jung, D, Fehr, E, et al. Spatial gradient in value representation along the medial prefrontal cortex reflects individual differences in prosociality. Proc Natl Acad Sci. (2015) 112:7851–6. doi: 10.1073/pnas.1423895112
128. Chang, SW, Gariépy, J-F, and Platt, ML. Neuronal reference frames for social decisions in primate frontal cortex. Nat Neurosci. (2013) 16:243–50. doi: 10.1038/nn.3287
129. Watson, KK, and Platt, ML. Social signals in primate orbitofrontal cortex. Curr Biol. (2012) 22:2268–73. doi: 10.1016/j.cub.2012.10.016
130. Hill, MR, Boorman, ED, and Fried, I. Observational learning computations in neurons of the human anterior cingulate cortex. Nat Commun. (2016) 7:12722. doi: 10.1038/ncomms12722
131. Desmurget, M, Bonnetblanc, F, and Duffau, H. Contrasting acute and slow-growing lesions: a new door to brain plasticity. Brain. (2007) 130:898–914. doi: 10.1093/brain/awl300
132. Eslinger, PJ, Flaherty-Craig, CV, and Benton, AL. Developmental outcomes after early prefrontal cortex damage. Brain Cogn. (2004) 55:84–103.
133. Anderson, SW, Wisnowski, JL, Barrash, J, Damasio, H, and Tranel, D. Consistency of neuropsychological outcome following damage to prefrontal cortex in the first years of life. J. Clin. Exp. Neuropsychol, (2009) 31:170–179.
Keywords: reward value, social value, ventromedial prefrontal cortex, orbitofrontal cortex, lesion studies, decision-making
Citation: Messimeris D, Levy R and Le Bouc R (2023) Economic and social values in the brain: evidence from lesions to the human ventromedial prefrontal cortex. Front. Neurol. 14:1198262. doi: 10.3389/fneur.2023.1198262
Received: 31 March 2023; Accepted: 27 September 2023;
Published: 12 October 2023.
Edited by:
Bruce Miller, University of California, San Francisco, United StatesReviewed by:
Erica Ordali, IMT School for Advanced Studies Lucca, ItalyCopyright © 2023 Messimeris, Levy and Le Bouc. This is an open-access article distributed under the terms of the Creative Commons Attribution License (CC BY). The use, distribution or reproduction in other forums is permitted, provided the original author(s) and the copyright owner(s) are credited and that the original publication in this journal is cited, in accordance with accepted academic practice. No use, distribution or reproduction is permitted which does not comply with these terms.
*Correspondence: Raphaël Le Bouc, cmFwaGFlbC5sZWJvdWNAYXBocC5mcg==
Disclaimer: All claims expressed in this article are solely those of the authors and do not necessarily represent those of their affiliated organizations, or those of the publisher, the editors and the reviewers. Any product that may be evaluated in this article or claim that may be made by its manufacturer is not guaranteed or endorsed by the publisher.
Research integrity at Frontiers
Learn more about the work of our research integrity team to safeguard the quality of each article we publish.