- 1Unité de stimulation transcrânienne, Clinique Bretéché, Groupe Elsan, Nantes, France
- 2EA 4391, équipe ENT (Excitabilité Nerveuse et Thérapeutique), Université Paris-Est Créteil, Créteil, France
- 3Unité de Neurophysiologie Clinique, Hôpital Henri Mondor, Assistance Publique - Hôpitaux de Paris, Créteil, France
Purpose: Cognitive impairment secondary to traumatic brain injury (TBI) is difficult to treat and usually results in severe disability.
Method: A 48-year-old man presented with chronic refractory headaches and persistent disabling cognitive impairment after TBI. He was first treated with occipital nerve stimulation (ONS) implanted bilaterally to relieve headaches (8 years after the head trauma). Two years later, he was treated with a 6-week protocol combining repetitive transcranial magnetic stimulation (rTMS) delivered to multiple cortical sites (prefrontal cortex, language areas, and areas involved in visuo-spatial functions) and computerized cognitive training (CogT) (targeting memory, language, and visuo-spatial functions) to improve cognitive performance.
Results: Executive and cognitive functions (attention, ability to perform calculations, and verbal fluency) improved in association with pain relief after ONS (33–42% improvement) and then improved even more after the rTMS-CogT protocol with an additional improvement of 36–40% on apathy, depression, and anxiety, leading to a significant reduction in caregiver burden. The functional improvement persisted and even increased at 6 months after the end of the rTMS-CogT procedure (10 years after the onset of TBI and 2 years after ONS implantation).
Conclusion: This is the first observation describing sustained improvement in post-TBI refractory headache, depression, and cognitive impairment by the association of bilaterally implanted ONS and a combined procedure of multisite rTMS and CogT to target various brain functions.
Introduction
Cognitive impairment is one of the most common sequelae of traumatic brain injury (TBI) (1). Their number tends to increase with the increase in the number of survivors, linked to better management of severe head injuries in the acute phase (2). Cognitive sequelae are considered more disabling than motor sequelae (3–7). They play an important role in hindering the possibility of reintegration into working life. The most frequent disorders concern memory (8), attention (9), and executive functions (10). Classic treatment consists of cognitive rehabilitation combined with drug treatments used in the cognitive disorders of Alzheimer's disease, such as cholinesterase inhibitors (Donezepil, Rivastigmine) (11). However, these treatments have side effects and only act inconsistently (12).
The efficacy of cognitive rehabilitation depends above all on the patient's participation, which may be disrupted by stress, a depressive state or other symptoms encountered in the context of post-TBI syndrome, such as headaches. These different factors must be taken into account before starting cognitive rehabilitation (11).
Recovery from a cognitive deficit can also be hampered by other factors, such as arousal and attention disorders. These disorders are common when trauma causes shear damage to the white matter responsible for a disconnection between the thalamus and the neocortex (13–15). Thus, interventions that can stimulate the thalamus directly [thalamic stimulation (16)] or indirectly [stimulation of the vagus nerve or peripheral nerves (17)] have been proposed in this context.
Currently, it is considered that the goal of cognitive rehabilitation is to develop neuroplasticity that will make the neural circuits involved in cognition more efficient (18). The outcome could be optimized by combining cognitive rehabilitation with a technique of non-invasive stimulation of the cerebral cortex such as repetitive transcranial magnetic stimulation (rTMS) or transcranial direct current stimulation (tDCS) (19), which are known to promote synaptic plasticity.
We report the case of a patient who suffered a head injury responsible for a post-TBI syndrome with disabling headaches and cognitive disorders. A first treatment with surgically implanted occipital nerve stimulation (ONS) was effective on headaches and clearly led to a cognitive improvement. Subsequently, a treatment combining multisite rTMS with computerized cognitive training (CogT) (20–22) further improved cognitive performance and acted on depression, anxiety, and apathy.
Case report
This is a 48-year-old patient who in 2012 had severe head injury related to a serious quad bike accident. On the initial CT scan, there was a fracture of the temporal and petrous bones on the left side, a diffuse subarachnoid hemorrhage and an edematous parenchymal contusion predominating on the right side, accompanied by a right temporal subdural hematoma (3 mm thick). A follow-up CT scan performed 72 h later showed a small intracerebral hemorrhage localized in the right temporal region, with increased edema in the right temporoparietal region leading to a mass effect with displacement of midline structures and the effacement of some cortical sulci, but without signs of cerebral herniation. The patient initially presented with an intermediate disorder of consciousness (Glasgow score 12) which lasted 5 days. Afterwards, the patient rapidly complained of headaches, followed by memory and attention disturbances and verbal expression difficulties. He also became irritable and had sleep disorders with difficulty falling asleep and frequent waking up. Daily headaches affected the entire head, suggesting a diagnosis of tension headache. The patient was discharged from the intensive care unit after 10 days. Despite several drug trials, the headaches persisted. The same was true for cognitive impairment, despite cognitive rehabilitation with weekly speech therapy sessions. The patient was unable to resume his professional activity as a telephone network administrator at the national level. He was on occupational disability, living at home with a very good family environment.
He was referred to our center at the beginning of 2018 mainly due to permanent headaches that had become increasingly debilitating. A treatment with non-invasive transcutaneous electrical neurostimulation (TENS) applied to the both occipital nerves was initiated in February 2018 and proved to be remarkably effective. In July 2019, the neuropsychological assessment (initial evaluation) reported a total score of 19/30 on the Mini-Mental State Examination (MMSE) (23) (Table 1; Figure 1). Subsequently, the headaches gradually worsened as the patient used TENS less and less, which he considered too restrictive in daily life. At the same time, cognitive problems also increased, suggesting a link between the two symptoms. This led us to decide to implant an ONS device. The preoperative cognitive assessment performed at the end of May 2020 (pre-ONS baseline) confirmed the worsening of cognitive problems with a MMSE total score of 14/30. Cognitive disorders consisted of impaired executive functions, with a total score of 9/18 on the Frontal Assessment Battery (FAB) (24) (Table 1). In addition, psychomotor slowing was observed during visual attention and word reading tests. The patient also had great difficulty concentrating due to headaches.
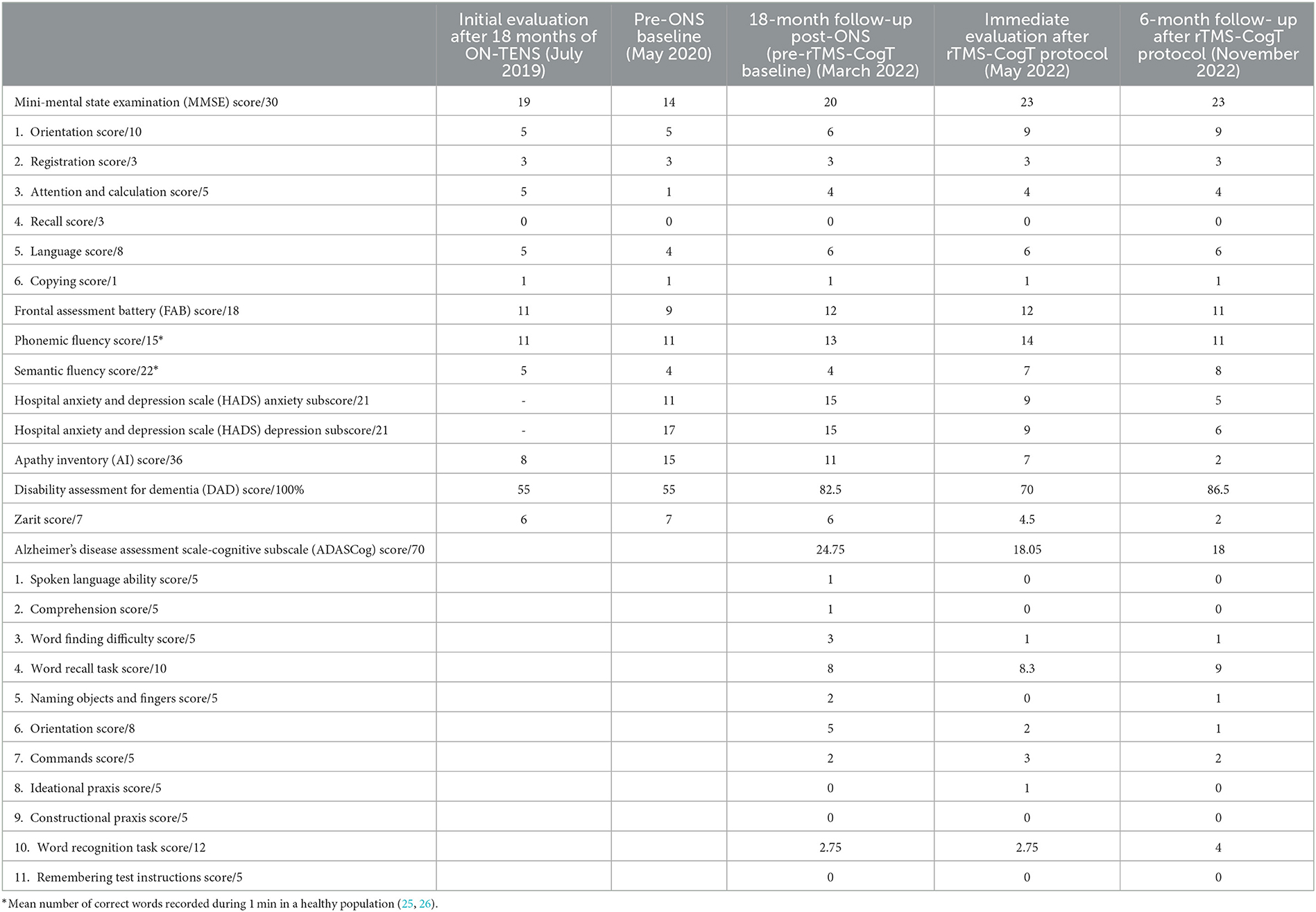
Table 1. Clinical assessment performed initially after 18 months of transcutaneous electrical neurostimulation of the occipital nerves (ON-TENS), before bilateral implantation of occipital nerve stimulation (ONS), 18 months after ONS implantation, at the end of a subsequent 6-week therapy combining multisite repetitive transcranial magnetic stimulation and computerized cognitive training (rTMS-CogT), and finally 6 months after the end of the rTMS-CogT protocol.
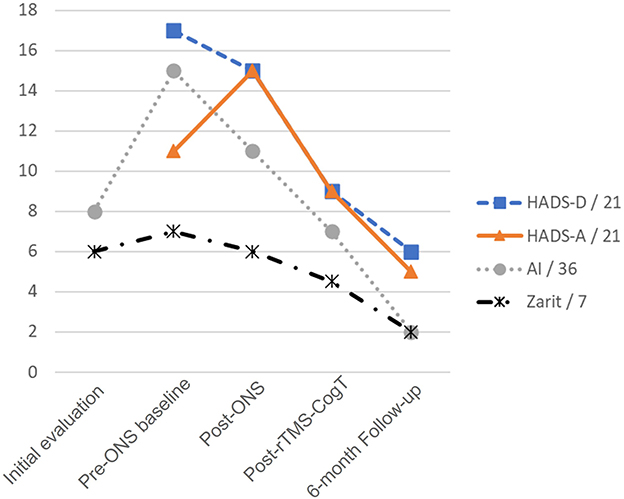
Figure 1. Evolution of clinical scores over time. Evolution of the Hospital Anxiety and Depression scale Depression (HADS-D) and Anxiety (HADS-A) subscores, the Apathy Inventory (AI) and the Zarit scores, at the following timepoints: (i) after 18 months of transcutaneous electrical neurostimulation of the occipital nerves: Initial evaluation; (ii) before bilateral implantation of occipital nerve stimulation (ONS): Pre-ONS baseline; (iii) 18 months after ONS implantation: Post-ONS; (iv) at the end of a subsequent 6-week therapy combining multisite repetitive transcranial magnetic stimulation and computerized cognitive training (rTMS-CogT): Post-rTMS-CogT; (v) 6 months after the end of the rTMS-CogT protocol: 6-month Follow-up.
The surgical implantation of the ONS device (electrodes and pulse generator) was performed in September 2020. The continuous stimulation of the occipital nerves allowed headaches to be relieved very efficaciously. Additionally, cognitive performance gradually improved over a period of time and then stabilized after 1 year. In March 2022 (18 months after ONS implantation), the MMSE total score was 20/30, corresponding to a 43% improvement from pre-ONS implantation baseline, including increased attention and ability to perform calculations as well as in language functions (Table 1). At the same time, the FAB score was 12/18, corresponding to a 33% improvement of executive functions from pre-ONS implantation baseline. This resulted in greater autonomy [Disability Assessment for Dementia (DAD) (27) score: 82.5 vs. 55% at baseline, 33% improvement], but with only a slightly lower load for caregivers [Zarit score (28): 6/7 vs. 7/7 at baseline].
As the improvement produced by ONS did not seem sufficient and no longer progressing, we decided to perform an additional therapeutic approach, based on rTMS applied to multiple cortical sites combined with CogT. Such a combined protocol has been developed under the name NeuroAD® therapy for the treatment of cognitive disorders, mainly apathy in the context of Alzheimer's disease (AD) (20–22). The detailed protocol we applied, similar to that used for patients with AD, has been described elsewhere (22). Briefly, this consisted of a daily session of rTMS-CogT for 5 consecutive days per week for a period of 6 weeks (30 sessions in total). Regarding rTMS, six different cortical regions were targeted, identified by a neuronavigation system (NeuroAD, Neuronix Ltd., Yoqnea'm, Israel) on the patient's brain magnetic resonance imaging (MRI): the right and left dorsolateral prefrontal cortices (DLPFC), the Broca's and Wernicke's language areas, and the right and left posterior parietal areas. On each region, 20 trains of 20 rTMS pulses were delivered at 10 Hz (2-s train duration and 40-s intertrain interval) for a total of 400 pulses over a period of 14 min. The intensity of stimulation was set at 100% of the rest motor threshold. During each intertrain interval of 40 s of rTMS interruption, the patient was asked to perform a cognitive task corresponding to the function of the stimulated cortical area: (i) naming of actions or objects, word recall, or spatial memory tasks for the DLPFC; (ii) syntax or grammar tasks for language areas; (iii) visuospatial attention tasks for parietal areas. Each task had six levels of gradual difficulty and the patients were allowed to progress to the next level of difficulty based on their performance in the previous session. During each daily session, three different cortical regions were treated by combining rTMS and corresponding CogT and the three other regions were treated the following day. Overall, each daily rTMS-CogT session lasted ~1 h.
The rTMS-CogT protocol was initiated in March 2022 and was completed without any adverse event or side effect. An initial assessment was performed just at the end of the 6 weeks of treatment (Table 1; Figure 1), including the Alzheimer's Disease Assessment Scale-Cognitive Subscale (ADASCog) (29) score. Unlike the MMSE, a lower ADASCog score reveals a better cognitive level. Following rTMS-CogT, the ADASCog score decreased by almost 7 points (27% improvement from pre-rTMS-CogT assessment), mainly in the naming objects, word finding, and orientation subscores. The orientation subscore of the MMSE also improved (from 6/10 to 9/10), leading to a 3-point increase in total MMSE score, as well as in semantic verbal fluency score. The rTMS-CogT therapy also produced beneficial effects on apathy [Apathy Inventory (AI) (30) score: 7/36 vs. 11/36 before rTMS-CogT, 36% improvement] and on depression and anxiety [Hospital Anxiety and Depression scale (HADS) (31) total score: 18/42 vs. 30/42 before rTMS-CogT, 40% improvement]. Finally, the caregiver burden was reduced (Zarit score: 4.5/7 vs. 6/7 before rTMS-CogT).
A follow-up assessment was performed 6 months after the end of the rTMS-CogT protocol (Table 1). At this time point, apathy, as well as depression and anxiety, further improved compared to the assessment performed just after the rTMS-CogT protocol (AI score: 2/36 vs. 7/36, 71% improvement; HADS total score: 11/42 vs. 18/42, 39% improvement). The caregiver burden was also greatly reduced (Zarit score: 2/7 vs. 4.5/7).
Discussion
In this observation, a significant and lasting improvement in cognition and mood was achieved in a patient with a severe TBI after a sequence of two neuromodulation treatments including (1) bilateral implantation of ONS and (2) multisite rTMS performed in combination with CogT. The latter approach is generally dedicated to the treatment of cognitive disorders associated with AD (20–22). Our study is, to our knowledge, the first to have used this procedure to treat cognitive impairment secondary to head trauma. This therapeutic solution was justified in this patient who presented with memory, language and orientation disorders, such as those encountered in AD (32).
Cognitive disorders secondary to head trauma are related to complex biochemical processes, resulting in particular from damage to the blood-brain barrier in the white matter, later responsible for diffuse axonal damage (13–15). Depending on whether these lesions are located at the superficial cortical, subcortical or deep brain level close to the basal ganglia, quite different clinical sequelae can result. Superficial lesions are more likely to disrupt the functioning of different cortical areas and the connections between them, resulting in a picture of cognitive impairment similar to that observed in AD. Deep lesions are more responsible for disorders of consciousness, alertness and attention that are encountered in more or less severe vegetative states. In the case presented here, brain imaging showed that there was a left temporal impact, marked by the fracture of the temporal bone and its petrous part, and also lesions on the opposite side, marked by an acute subdural hematoma and a right temporoparietal contusion. These findings suggest traumatic lesions secondary to a rotation mechanism in the coronal plane (33) theoretically responsible for axonal lesions of moderate severity but affecting both the subcortical and deep brain regions (34).
However, cognitive impairment following head trauma is not solely determined by white matter lesions. The homeostatic balance between inhibition and excitation is also disrupted in the brain's neural networks following TBI (35). Gamma-aminobutyric acid (GABA) is the major inhibitory neurotransmitter and glutamate the major excitatory neurotransmitter in the central nervous system. There is evidence for the occurrence of an immediate rise in glutamate levels following severe TBI in humans (36). A disruption in GABAergic signaling may lead to a further increase in glutamate excitotoxicity, which can worsen the impact of neuronal damage (37). By being able to modulate the GABA/glutamate balance and producing long-lasting effects on synaptic transmission (38–40), non-invasive brain stimulation techniques, such as rTMS, have a certain interest in this clinical context (41). However, it is difficult to have preconceptions regarding the type of rTMS pattern to apply to modulate the GABA/glutamate balance after TBI, particularly according to the influence of metaplasticity processes (42). Indeed, while rTMS tonically applied at low frequency (≤ 1 Hz) is known to be able to depress long-term synaptic transmission and is therefore potentially neuroprotective, high-frequency rTMS (as applied in our patient), although considered excitatory, has also shown neuroprotective or pro-GABAergic effects in various experimental models (43–45) or clinical conditions (46–48). Furthermore, the situation is more complex than a dual mechanism of increased vs. decreased excitability, because cognitive recovery after TBI depends on various neural repair processes, including restoration and synchronization of neuronal network connectivity for cognitive performance, in which the modulation of tonic and phasic GABA levels plays a complex interaction role.
Our results show that combined rTMS-CogT therapy may be a well-suited approach to promote post-TBI cognitive recovery. However, performing such a protocol requires good attentional and psychomotor capacities to complete the cognitive tasks quickly within the time imposed by the rTMS protocol. Our patient initially presented with great difficulty concentrating, mainly due to disabling headaches, which could have initially prevented him from complying with the rTMS-CogT protocol. This is the reason why it appeared to us that the priority was to treat his headaches first, which led us to propose the treatment by ONS. High analgesic efficacy of this neuromodulation technique has been reported in various types of non-migrainous chronic headaches (49) and was therefore confirmed in our patient. To our knowledge, implanted ONS has never been proposed before to relieve refractory headaches secondary to TBI, but clearly appears to be an interesting therapeutic solution in this context.
The ONS probably made it possible to take a first step in cognitive improvement in our patient, but indirectly, thanks to the reduction of pain. However, a cognitive improvement more directly produced by ONS was also possible, as suggested by a previous study showing the increase in memory performance thanks to the application of tDCS to stimulate the greater occipital nerve (50), possibly via the activation of the locus coeruleus (51). Other authors have suggested that ONS may also improve attention by acting on the thalamus or basal ganglia (52, 53). Thus, our patient improved his attentional and executive functions following ONS therapy, but this proved insufficient to restore quality daily life and the rTMS-CogT protocol seemed to us to be a good approach to further improve the cognitive performance and reduce mood disorders to a clinically satisfactory level.
Various rTMS protocols have already been proposed to treat the clinical symptoms associated with concussion (mild TBI) or more severe TBI and this has been the subject of about 40 publications since 2006, including three recent reviews (54–56) and three meta-analyses (57–59). Symptoms intended to be treated by rTMS, primarily targeted to the left DLPFC at high frequency (or more rarely to the right DLPFC at low frequency), were disorders of consciousness, dizziness, auditory disorders, motor dysfunction, pain, headache, depression, or cognitive impairment, including post-concussion syndrome after mild TBI.
All recent reviews and meta-analyses (54–59) have concluded that there is significant evidence for the efficacy of rTMS of the DLPFC as a therapeutic intervention for depression, headache or pain associated with TBI. In contrast, the effects were more moderate and variable with respect to the improvement of cognitive performance, including executive functions, attentional abilities, and memory, except perhaps for visuospatial memory tasks, whereas the level of evidence was very low for disorders of consciousness.
For example, in a series of 21 patients with refractory post-TBI headache, 4 sessions of 10 Hz rTMS administered to the left DLPFC showed a small but significant improvement in depressive symptoms on the Hamilton Rating Scale for Depression score (3-point reduction, 15% from baseline) after active but not sham rTMS, beyond major analgesic effects on headaches (60). However, other studies have shown less significant effects of rTMS on depression associated with TBI. First, in a series of 30 patients, only small and very variable beneficial effects were observed on depression following a protocol of 20 sessions of 1 Hz rTMS delivered to the right DLPFC (61). Second, in a series of 21 patients, no differences were observed between active and sham protocols of 20 sessions of sequential bilateral rTMS to the right and left DLPFC (62). Furthermore, in these studies, rTMS therapy improved post-concussion subjective symptoms (61) or cognitive performance regarding executive functions and working memory (62).
Thus, given the results of these previous studies, one can question the relevance of delivering rTMS at low (1 Hz) or high (10–20 Hz) frequency of stimulation to the DLPFC target depending on its laterality (right or left) and also the method to determine the optimal location of this DLPFC target (using cranial landmarks or image-guided navigation). On the one hand, in our case, rTMS trains were applied at high frequency on the DLPFC, whatever the hemisphere. On the other hand, one group targeted the DLPFC with individualized resting-state network brain mapping of the functional connectivity between the subgenual anterior cingulate cortex (sgACC) and the default-mode network using functional magnetic resonance imaging (63, 64). After 20 sequential bilateral rTMS sessions on this individualized target (low-frequency stimulation on the right side and high-frequency stimulation on the left side), TBI-associated depression was improved twice by active stimulation than by sham stimulation on the Montgomery-Asberg Depression Rating Scale score.
However, beyond depression, our study mainly showed rTMS-induced improvement on various cognitive symptoms. In the literature, at least six studies have evaluated the effects of rTMS delivered at high frequency over the left DLPFC on cognitive impairment associated with TBI (65–70). First, in 12 patients with mild TBI, 20 sessions of 10 Hz-rTMS delivered to the left DLPFC improved post-concussion symptoms, including cognitive deficits (mainly memory disturbances), for <3 months (65). Second, in 26 patients with cognitive complaints and a history of mild-to-moderate TBI, 5 sessions of 10 Hz-rTMS delivered to the left DLPFC improved executive functions and subjective measures of cognitive dysfunction related to a post-concussion syndrome, up to 2-week follow-up (66). In contrast, no effect of treatment was observed on cognitive test performance assessing selective attention control and verbal learning or fluency. Third, in 18 patients with persistent post-concussion syndrome, 13 sessions of 20 Hz rTMS delivered to the left DLPFC produced significant cognitive improvement up to 2 months after the intervention, but only in patients with recent TBI (<12 months) (67). In contrast, our patient benefited from rTMS therapy 10 years after the initial trauma. Finally, in two studies of patients with TBI, the overall effect of 10 Hz rTMS delivered to the left DLPFC produced either cognitive improvement below clinically meaningful thresholds (69) or no significant changes in executive function evaluated using the Trail Making Test Part B or other neuropsychological tests for attention, learning and visuospatial memory (70).
Concerning low-frequency (1 Hz) rTMS delivered to the right DLPFC, a protocol of 30 sessions twice-daily applied to 15 patients with mild TBI showed significant improvement in different post-concussion symptoms, such as pain, depression and anxiety, as well as in cognitive tasks assessing verbal fluency, working memory, selective attention, and cognitive processing speed (71). In contrast, there were no significant changes in executive functioning, fatigue severity, or apathy.
In all these studies, rTMS was applied in isolation, mainly targeting the left DLPFC and not associated with CogT at the same time. Only one study has previously evaluated the benefit of treating TBI-associated cognitive impairment by combining rTMS and CogT (72). In this retrospective study of 166 patients, half received rTMS and CogT and the other half (control group) various usual methods of treatment (72). The protocol was not well described but was based on 1 Hz-rTMS delivered to the DLPFC (likely to the right hemisphere) once a day, 5 days a week for 3 months, in combination with CogT (but not performed during the rTMS protocol), including various tasks to improve concentration, visuospatial memory, visual perception, judgment and reasoning. Cognitive improvement was significantly better in the rTMS-CogT group than in the control group.
Our case highlights several original elements likely to improve the therapeutic management of patients with TBI.
First, the benefit of using ONS to treat chronic refractory headaches secondary to TBI, even several years after the head trauma, must be emphasized, as this is the first case reported here. Our case broadens the spectrum of indications for this technique. It is important to point out that it is possible to perform this neuromodulation strategy non-invasively, using a TENS technique. ONS-TENS can be a temporary solution, but if this technique is effective but insufficient to control headaches over time, then this efficacy can be predictive of a good outcome provided by implanted ONS (73, 74).
Second, our case shows that the implantation of ONS can be well tolerated in patients who have previously had a head trauma, and that this implanted neuromodulation technique does not prohibit the subsequent performance of rTMS sessions, even on different cortical sites such as the posterior parietal areas.
Third, our case also shows that the treatment of headaches associated with TBI is a therapeutic priority, making it possible to trigger a virtuous circle of management of other post-concussion symptoms. Perhaps also the ONS could have direct beneficial effects on certain central dysfunctions, for example in the cognitive domain.
Fourth, the clinical results obtained in this patient may also suggest that the prior ONS could have set the stage for a significant and lasting improvement in cognitive performance and mood produced by the subsequent rTMS-CogT protocol. Persistent cognitive disorders may be responsible for anxiety and depression (75), possibly by alteration of dopaminergic circuits in the context of TBI, mainly concerning the striatum and the frontal/prefrontal cortex (76–78). It is therefore conceivable that the combination of techniques capable of modulating deep brain structures such as the basal ganglia on the one hand (ONS) and the cortical brain networks on the other hand (rTMS) could have a synergistic interest in reactivating dopaminergic circuits in order to improve various post-concussion symptoms in the context of TBI. This hypothesis could be tested in the future, in particular by functional brain imaging techniques.
Finally, our results support a likely greater efficacy (in terms of magnitude and duration) on improving cognitive performance and mood by means of a combined treatment with multisite rTMS and CogT compared to the rTMS strategy usually applied in isolation in the context of TBI, which is the stimulation of the DLPFC only (usually at high frequency on the left hemisphere). However, it is difficult to distinguish between (i) a potential beneficial effect of multisite stimulation related to the total stimulation dose or the modulation of cerebral connectivity, (ii) the specific effect of CogT, or (iii) the possible synergy between both approaches.
Of course, a single case does not justify unqualified approval of the techniques used. Additionally, given that most clinical measures improved linearly over time, this may suggest that the entire treatment received regularly may have benefited the patient rather than a specific technique (ONS or rTMS-CogT). It is also clear as we have repeatedly pointed out that the remission of the headaches contributed greatly to the overall improvement of the patient. Finally, it is obvious that this case does not eliminate a placebo effect of the different neuromodulation techniques used, and that the simple fact of being included in an innovative therapeutic program could have improved his symptoms, regarding mood for example. It must, however, be emphasized that the beneficial therapeutic effects were obtained very far from the initial traumatic episode and over a prolonged period of several years, which supports the real efficacy of the neuromodulation techniques that were added. In any case, this original observation opens the prospect of a controlled study on a larger sample, evaluating the effects on the various post-concussion symptoms that can be produced by means of an active multisite rTMS protocol compared to a sham condition and associated or not with a CogT protocol.
Data availability statement
The original contributions presented in the study are included in the article/supplementary material, further inquiries can be directed to the corresponding authors.
Ethics statement
Written informed consent was obtained from the individual(s) for the publication of any potentially identifiable images or data included in this article.
Author contributions
TC and J-PN: conceived and designed the study. J-PL and AS: provided supervision. EL, SL, and SD: collected the data. All authors contributed to manuscript revision and read and approved the final version.
Conflict of interest
The authors declare that the research was conducted in the absence of any commercial or financial relationships that could be construed as a potential conflict of interest.
Publisher's note
All claims expressed in this article are solely those of the authors and do not necessarily represent those of their affiliated organizations, or those of the publisher, the editors and the reviewers. Any product that may be evaluated in this article, or claim that may be made by its manufacturer, is not guaranteed or endorsed by the publisher.
References
1. McCullagh S, Oucherlony D, Protzner A, Blair N, Feinstein A. Prediction of neuropsychiatric outcome following mild trauma brain injury: an examination of the Glasgow Coma Scale. Brain Inj. (2001) 15:489–97. doi: 10.1080/02699050010007353
2. Bigler ED. Neuropsychology and clinical neuroscience of persistent post-concussive syndrome. J Int Neuropsychol Soc. (2008) 14:1–22. doi: 10.1017/S135561770808017X
3. Brooks N, McKinlay W, Symington C, Beattie A, Campsie L. Return to work within the first seven years of severe head injury. Brain Inj. (1987) 1:5–19. doi: 10.3109/02699058709034439
4. Prigatano GP. Personality disturbances associated with traumatic brain injury. J Consult Clin Psychol. (1992) 60:360–8. doi: 10.1037/0022-006X.60.3.360
5. Riggio S. Traumatic brain injury and its neurobehavioral sequelae. Neurol Clin. (2011) 29:35–47. doi: 10.1016/j.ncl.2010.10.008
6. Arciniegas DB, Wortzel HS. Emotional and behavioral dyscontrol after traumatic brain injury. Psychiatr Clin North Am. (2014) 37:31–53. doi: 10.1016/j.psc.2013.12.001
7. Walker WC, Pickett TC. Motor impairment after severe traumatic brain injury: a longitudinal multicenter study. J Rehabil Res Dev. (2007) 44:975–82. doi: 10.1682/JRRD.2006.12.0158
8. Wiegner S, Donders J. Performance on the California Verbal Learning Test after traumatic brain injury. J Clin Exp Neuropsychol. (1999) 21:159–70. doi: 10.1076/jcen.21.2.159.925
9. Slovarp L, Azuma T, Lapointe L. The effect of traumatic brain injury on sustained attention and working memory. Brain Inj. (2012) 26:48–57. doi: 10.3109/02699052.2011.635355
10. Brooks J, Fos LA, Greve KW, Hammond JS. Assessment of executive function in patients with mild traumatic brain injury. J Trauma. (1999) 46:159–63. doi: 10.1097/00005373-199901000-00027
11. Wortzel HS, Arciniegas DB. Treatment of post-traumatic cognitive impairments. Curr Treat Options Neurol. (2012) 14:493–508. doi: 10.1007/s11940-012-0193-6
12. Arciniegas DB, Silver JM. Pharmacotherapy of posttraumatic cognitive impairments. Behav Neurol. (2006) 17:25–42. doi: 10.1155/2006/460592
13. Felmingham KL, Baguley IJ, Green AM. Effects of diffuse axonal injury on speed of information processing following severe traumatic brain injury. Neuropsychology. (2004) 18:564–71. doi: 10.1037/0894-4105.18.3.564
14. Scheid R, Walther K, Guthke T, Preul C, von Cramon DY. Cognitive sequelae of diffuse axonal injury. Arch Neurol. (2006) 63:418–24. doi: 10.1001/archneur.63.3.418
15. Schiff ND, Giacino JT, Kalmar K, Victor JD, Baker K, Gerber M, et al. Behavioural improvements with thalamic stimulation after severe traumatic brain injury. Nature. (2007) 448:600–3. doi: 10.1038/nature06041
16. Munivenkatappa A, Devi BI, Shukla DP, Rajeswaran J. Role of the thalamus in natural recovery of cognitive impairment in patients with mild traumatic brain injury. Brain Inj. (2016) 30:388–92. doi: 10.3109/02699052.2015.1089599
17. Shirvalkar P, Seth M, Schiff ND, Herrera DG. Cognitive enhancement with central thalamic electrical stimulation. Proc Natl Acad Sci USA. (2006) 103:17007–12. doi: 10.1073/pnas.0604811103
18. Galetto V, Sacco K. Neuroplastic changes induced by cognitive rehabilitation in traumatic brain injury: a review. Neurorehabil Neural Repair. (2017) 31:800–13. doi: 10.1177/1545968317723748
19. Rochon E, Eskes GA, Skidmore ER, Winstein CJ. Editorial: combined therapeutic approaches to neurological rehabilitation. Front Rehabil Sci. (2022) 3:918005. doi: 10.3389/978-2-88976-469-3
20. Bentwich J, Dobronevsky E, Aichenbaum S, Shorer R, Peretz R, Khaigrekht M, et al. Beneficial effect of repetitive transcranial magnetic stimulation combined with cognitive training for the treatment of Alzheimer's disease: a proof of concept study. J Neural Transm. (2011) 118:463–71. doi: 10.1007/s00702-010-0578-1
21. Lee J, Choi BH, Oh E, Sohn EH, Lee AY. Treatment of Alzheimer's disease with repetitive transcranial magnetic stimulation combined with cognitive training: a prospective, randomized, double-blind, placebo-controlled study. J Clin Neurol. (2016) 12:57–64. doi: 10.3988/jcn.2016.12.1.57
22. Nguyen JP, Suarez A, Kemoun G, Meignier M, Le Saout E, Damier P, et al. Repetitive transcranial magnetic stimulation combined with cognitive training for the treatment of Alzheimer's disease. Neurophysiol Clin. (2017) 47:47–53. doi: 10.1016/j.neucli.2017.01.001
23. Folstein MF, Folstein SE, McHugh PR. “Mini-mental state”. A practical method for grading the cognitive state of patients for the clinician. J Psychiatr Res. (1975) 12:189–98. doi: 10.1016/0022-3956(75)90026-6
24. Dubois B, Slachevsky A, Litvan I, Pillon B. The FAB: a frontal assessment battery at bedside. Neurology. (2000) 55:1621–6. doi: 10.1212/WNL.55.11.1621
25. Obeso I, Casabona E, Bringas ML, Alvarez L, Jahanshahi M. Semantic and phonemic verbal fluency in Parkinson's disease: Influence of clinical and demographic variables. Behav Neurol. (2012) 25:111–8. doi: 10.3233/BEN-2011-0354
26. Shao Z, Janse E, Visser K, Meyer AS. What do verbal fluency tasks measure? Predictors of verbal fluency performance in older adults. Front Psychol. (2014) 5:772. doi: 10.3389/fpsyg.2014.00772
27. Gélinas I, Gauthier L, McIntyre M, Gauthier S. Development of a functional measure for persons with Alzheimer's disease: the disability assessment for dementia. Am J Occup Ther. (1999) 53:471–81. doi: 10.5014/ajot.53.5.471
28. Bédard M, Molloy DW, Squire L, Dubois S, Lever JA, O'Donnell M. The Zarit Burden Interview: a new short version and screening version. Gerontologist. (2001) 41:652–7. doi: 10.1093/geront/41.5.652
29. Cano SJ, Posner HB, Moline ML, Hurt SW, Swartz J, Hsu T, et al. The ADAS-cog in Alzheimer's disease clinical trials: psychometric evaluation of the sum and its parts. J Neurol Neurosurg Psychiatry. (2010) 81:1363–8. doi: 10.1136/jnnp.2009.204008
30. Robert PH, Clairet S, Benoit M, Koutaich J, Bertogliati C, Tible O, et al. The apathy inventory: assessment of apathy and awareness in Alzheimer's disease, Parkinson's disease and mild cognitive impairment. Int J Geriatr Psychiatry. (2002) 17:1099–105. doi: 10.1002/gps.755
31. Mykletun A, Stordal E, Dahl AA. Hospital anxiety and depression (HAD) scale: factor structure, item analyses and internal consistency in a large population. Br J Psychiatry. (2001) 179:540–4. doi: 10.1192/bjp.179.6.540
32. Morris JC. Revised criteria for mild cognitive impairment may compromise the diagnosis of Alzheimer disease dementia. Arch Neurol. (2012) 69:700–8. doi: 10.1001/archneurol.2011.3152
33. Browne KD, Chen XH, Meaney DF, Smith DH. Mild traumatic brain injury and diffuse axonal injury in swine. J Neurotrauma. (2011) 28:1747–55. doi: 10.1089/neu.2011.1913
34. Laskowski RA, Creed JA, Raghupathi R. Pathophysiology of mild TBI: implications for altered signaling pathways. In:Kobeissy FH, , editor. Brain Neurotrauma: Molecular, Neuropsychological, and Rehabilitation Aspects. Boca Raton, FL: CRC Press/Taylor & Francis (2015).
35. Guerriero RM, Giza CC, Rotenberg A. Glutamate and GABA imbalance following traumatic brain injury. Curr Neurol Neurosci Rep. (2015) 15:27. doi: 10.1007/s11910-015-0545-1
36. Chamoun R, Suki D, Gopinath SP, Goodman JC, Robertson C. Role of extracellular glutamate measured by cerebral microdialysis in severe traumatic brain injury. J Neurosurg. (2010) 113:564–70. doi: 10.3171/2009.12.JNS09689
37. Cantu D, Walker K, Andresen L, Taylor-Weiner A, Hampton D, Tesco G, et al. Traumatic brain injury increases cortical glutamate network activity by compromising GABAergic control. Cereb Cortex. (2015) 25:2306–20. doi: 10.1093/cercor/bhu041
38. Lefaucheur JP. Principles of therapeutic use of transcranial and epidural cortical stimulation. Clin Neurophysiol. (2008) 119:2179–84. doi: 10.1016/j.clinph.2008.07.007
39. Lefaucheur JP. Methods of therapeutic cortical stimulation. Neurophysiol Clin. (2009) 39:1–14. doi: 10.1016/j.neucli.2008.11.001
40. Hoogendam JM, Ramakers GM, Di Lazzaro V. Physiology of repetitive transcranial magnetic stimulation of the human brain. Brain Stimul. (2010) 3:95–118. doi: 10.1016/j.brs.2009.10.005
41. Galimberti A, Tik M, Pellegrino G, Schuler AL. Effectiveness of rTMS and tDCS treatment for chronic TBI symptoms: a systematic review and meta-analysis. Prog Neuropsychopharmacol Biol Psychiatry. (2023) 128:110863. doi: 10.1016/j.pnpbp.2023.110863
42. Abraham WC, Bear MF. Metaplasticity: the plasticity of synaptic plasticity. Trends Neurosci. (1996) 19:126–30. doi: 10.1016/S0166-2236(96)80018-X
43. Wang F, Zhang Y, Wang L, Sun P, Luo X, Ishigaki Y, et al. Improvement of spatial learning by facilitating large-conductance calcium-activated potassium channel with transcranial magnetic stimulation in Alzheimer's disease model mice. Neuropharmacology. (2015) 97:210–9. doi: 10.1016/j.neuropharm.2015.05.027
44. Cao H, Zuo C, Gu Z, Huang Y, Yang Y, Zhu L, et al. High frequency repetitive transcranial magnetic stimulation alleviates cognitive deficits in 3xTg-AD mice by modulating the PI3K/Akt/GLT-1 axis. Redox Biol. (2022) 54:102354. doi: 10.1016/j.redox.2022.102354
45. Wang N, Jia Y, Zhou X, Wang X, Zhou H, Xiao N. Effects of repetitive transcranial magnetic stimulation on pallidum GABAergic neurons and motor function in rat models of kernicterus. Brain Sci. (2023) 13:1252. doi: 10.3390/brainsci13091252
46. Lefaucheur JP, Drouot X, Ménard-Lefaucheur I, Keravel Y, Nguyen JP. Motor cortex rTMS restores defective intracortical inhibition in chronic neuropathic pain. Neurology. (2006) 67:1568–74. doi: 10.1212/01.wnl.0000242731.10074.3c
47. Fierro B, Brighina F, D'Amelio M, Daniele O, Lupo I, Ragonese P, et al. Motor intracortical inhibition in PD: L-DOPA modulation of high-frequency rTMS effects. Exp Brain Res. (2008) 184:521–8. doi: 10.1007/s00221-007-1121-y
48. Sun H, Gan C, Wang L, Ji M, Cao X, Yuan Y, et al. Cortical disinhibition drives freezing of gait in Parkinson's disease and an exploratory repetitive transcranial magnetic stimulation study. Mov Disord. (2023). in press. doi: 10.1002/mds.29595
49. Carrondo Cottin S, Gallani N, Cantin L, Prud'Homme M. Occipital nerve stimulation for non-migrainous chronic headaches: a systematic review protocol. Syst Rev. (2019) 8:181. doi: 10.1186/s13643-019-1101-x
50. Vanneste S, Mohan A, Yoo HB, Huang Y, Luckey AM, McLeod SL, et al. The peripheral effect of direct current stimulation on brain circuits involving memory. Sci Adv. (2020) 6:eaax9538. doi: 10.1126/sciadv.aax9538
51. Luckey AM, McLeod SL, Robertson IH, To WT, Vanneste S. Greater occipital nerve stimulation boosts associative memory in older individuals: a randomized trial. Neurorehabil Neural Repair. (2020) 34:1020–9. doi: 10.1177/1545968320943573
52. Walling I, Smith H, Gee LE, Kaszuba B, Chockalingam A, Barborica A, et al. Occipital nerve stimulation attenuates neuronal firing response to mechanical stimuli in the ventral posteromedial thalamus of a rodent model of chronic migraine. Neurosurgery. (2017) 81:696–701. doi: 10.1093/neuros/nyx135
53. Kovacs S, Peeters R, De Ridder D, Plazier M, Menovsky T, Sunaert S. Central effects of occipital nerve electrical stimulation studied by functional magnetic resonance imaging. Neuromodulation. (2011) 14:46–55; discussion 56–7. doi: 10.1111/j.1525-1403.2010.00312.x
54. Nardone R, Sebastianelli L, Versace V, Brigo F, Golaszewski S, Manganotti P, et al. Repetitive transcranial magnetic stimulation in traumatic brain injury: evidence from animal and human studies. Brain Res Bull. (2020) 159:44–52. doi: 10.1016/j.brainresbull.2020.03.016
55. Pink AE, Williams C, Alderman N, Stoffels M. The use of repetitive transcranial magnetic stimulation (rTMS) following traumatic brain injury (TBI): a scoping review. Neuropsychol Rehabil. (2021) 31:479–505. doi: 10.1080/09602011.2019.1706585
56. Mollica A, Greben R, Oriuwa C, Siddiqi SH, Burke MJ. Neuromodulation treatments for mild traumatic brain injury and post-concussive symptoms. Curr Neurol Neurosci Rep. (2022) 22:171–81. doi: 10.1007/s11910-022-01183-w
57. Ahorsu DK, Adjaottor ES, Lam BYH. Intervention effect of non-invasive brain stimulation on cognitive functions among people with traumatic brain injury: a systematic review and meta-analysis. Brain Sci. (2021) 11:840. doi: 10.3390/brainsci11070840
58. Tsai PY, Chen YC, Wang JY, Chung KH, Lai CH. Effect of repetitive transcranial magnetic stimulation on depression and cognition in individuals with traumatic brain injury: a systematic review and meta-analysis. Sci Rep. (2021) 11:16940. doi: 10.1038/s41598-021-95838-2
59. Li X, Lu T, Yu H, Shen J, Chen Z, Yang X, et al. Repetitive transcranial magnetic stimulation for neuropathic pain and neuropsychiatric symptoms in traumatic brain injury: a systematic review and meta-analysis. Neural Plast. (2022) 2022:2036736. doi: 10.1155/2022/2036736
60. Leung A, Metzger-Smith V, He Y, Cordero J, Ehlert B, Song D, et al. Left dorsolateral prefrontal cortex rTMS in alleviating MTBI related headaches and depressive symptoms. Neuromodulation. (2018) 21:390–401. doi: 10.1111/ner.12615
61. Rao V, Bechtold K, McCann U, Roy D, Peters M, Vaishnavi S, et al. Low-frequency right repetitive transcranial magnetic stimulation for the treatment of depression after traumatic brain injury: a randomized sham-controlled pilot study. J Neuropsychiatry Clin Neurosci. (2019) 31:306–18. doi: 10.1176/appi.neuropsych.17110338
62. Hoy KE, McQueen S, Elliot D, Herring SE, Maller JJ, Fitzgerald PB, et al. Pilot investigation of repetitive transcranial magnetic stimulation for post-traumatic brain injury depression: safety, tolerability, and efficacy. J Neurotrauma. (2019) 36:2092–8. doi: 10.1089/neu.2018.6097
63. Siddiqi SH, Trapp NT, Hacker CD, Laumann TO, Kandala S, Hong X, et al. Repetitive transcranial magnetic stimulation with resting-state network targeting for treatment-resistant depression in traumatic brain injury: a randomized, controlled, double-blinded pilot study. J Neurotrauma. (2019) 36:1361–74. doi: 10.1089/neu.2018.5889
64. Siddiqi SH, Kandala S, Hacker CD, Trapp NT, Leuthardt EC, Carter AR, et al. Individualized precision targeting of dorsal attention and default mode networks with rTMS in traumatic brain injury-associated depression. Sci Rep. (2023) 13:4052. doi: 10.1038/s41598-022-21905-x
65. Koski L, Kolivakis T, Yu C, Chen JK, Delaney S, Ptito A. Noninvasive brainstimulation for persistent postconcussion symptoms in mild traumatic braininjury. J Neurotrauma. (2015) 32:38–44. doi: 10.1089/neu.2014.3449
66. Franke LM, Gitchel GT, Perera RA, Hadimani RL, Holloway KL, Walker WC. Randomized trial of rTMS in traumatic brain injury: improved subjective neurobehavioral symptoms and increases in EEG delta activity. Brain Inj. (2022) 36:683–92. doi: 10.1080/02699052.2022.2033845
67. Moussavi Z, Suleiman A, Rutherford G, Ranjbar Pouya O, Dastgheib Z, Zhang W, et al. A pilot randomised double-blind study of the tolerability and efficacy of repetitive transcranial magnetic stimulation on persistent post-concussion syndrome. Sci Rep. (2019) 9:5498. doi: 10.1038/s41598-019-41923-6
68. Stilling JM, Duszynski CC, Oni I, Paxman E, Dunn JF, Debert CT. Using functional near-infrared spectroscopy to study the effect of repetitive transcranial magnetic stimulation in concussion: a two-patient case study. Front Neurol. (2019) 10:476. doi: 10.3389/fneur.2019.00476
69. Stilling J, Paxman E, Mercier L, Gan LS, Wang M, Amoozegar F, et al. Treatment of persistent post-traumatic headache and post-concussion symptoms using repetitive transcranial magnetic stimulation: a pilot, double-blind, randomized controlled trial. J Neurotrauma. (2020) 37:312–23. doi: 10.1089/neu.2019.6692
70. Neville IS, Zaninotto AL, Hayashi CY, Rodrigues PA, Galhardoni R, Ciampi de Andrade D, et al. Repetitive TMS does not improve cognition in patients with TBI: a randomized double-blind trial. Neurology. (2019) 93:e190–9. doi: 10.1212/WNL.0000000000007748
71. Meek BP, Hill S, Modirrousta M. Accelerated repetitive transcranial magnetic stimulation in the treatment of post-concussion symptoms due to mild traumatic brain injury: a pilot study. Brain Inj. (2021) 35:48–58. doi: 10.1080/02699052.2020.1857837
72. Zhou L, Huang X, Li H, Guo R, Wang J, Zhang Y, et al. Rehabilitation effect of rTMS combined with cognitive training on cognitive impairment after traumatic brain injury. Am J Transl Res. (2021) 13:11711–7.
73. Nguyen JP, Nizard J, Kuhn E, Carduner F, Penverne F, Verleysen-Robin MC, et al. A good preoperative response to transcutaneous electrical nerve stimulation predicts a better therapeutic effect of implanted occipital nerve stimulation in pharmacologically intractable headaches. Neurophysiol Clin. (2016) 46:69–75. doi: 10.1016/j.neucli.2015.12.002
74. Nguyen JP, Raoul S, Desal H, Bourcier R, Kuhn E, Dixneuf V, et al. Treatment of refractory headache secondary to intracranial endovascular procedure by transcutaneous electrical nerve stimulation of the occipital nerve. Neurophysiol Clin. (2018) 48:309–12. doi: 10.1016/j.neucli.2018.05.041
75. Bales JW, Wagner AK, Kline AE, Dixon CE. Persistent cognitive dysfunction after traumatic brain injury: a dopamine hypothesis. Neurosci Biobehav Rev. (2009) 33:981–1003. doi: 10.1016/j.neubiorev.2009.03.011
76. McDonald BC, Flashman LA, Saykin AJ. Executive dysfunction following traumatic brain injury: neural substrates and treatment strategies. NeuroRehabilitation. (2002) 17:333–44. doi: 10.3233/NRE-2002-17407
77. Chudasama Y, Robbins TW. Functions of frontostriatal systems in cognition: comparative neuropsychopharmacological studies in rats, monkeys and humans. Biol Psychol. (2006) 73:19–38. doi: 10.1016/j.biopsycho.2006.01.005
78. Verduzco-Mendoza A, Carrillo-Mora P, Avila-Luna A, Gálvez-Rosas A, Olmos-Hernández A, Mota-Rojas D, et al. Role of the dopaminergic system in the striatum and its association with functional recovery or rehabilitation after brain injury. Front Neurosci. (2021) 15:693404. doi: 10.3389/fnins.2021.693404
Keywords: traumatic brain injury, refractory headache, occipital nerve stimulation, repetitive transcranial magnetic simulation, cognitive training, combined strategy
Citation: Caloc'h T, Le Saout E, Litaneur S, Suarez A, Durand S, Lefaucheur J-P and Nguyen J-P (2023) Treatment of cognitive and mood disorders secondary to traumatic brain injury by the association of bilateral occipital nerve stimulation and a combined protocol of multisite repetitive transcranial magnetic stimulation and cognitive training: A case report. Front. Neurol. 14:1195513. doi: 10.3389/fneur.2023.1195513
Received: 28 March 2023; Accepted: 23 October 2023;
Published: 07 November 2023.
Edited by:
Giorgio Sandrini, Fondazione Cirna Onlus, ItalyReviewed by:
Mustafa Q. Hameed, Boston Children's Hospital and Harvard Medical School, United StatesShankar Tumati, University of Toronto, Canada
Copyright © 2023 Caloc'h, Le Saout, Litaneur, Suarez, Durand, Lefaucheur and Nguyen. This is an open-access article distributed under the terms of the Creative Commons Attribution License (CC BY). The use, distribution or reproduction in other forums is permitted, provided the original author(s) and the copyright owner(s) are credited and that the original publication in this journal is cited, in accordance with accepted academic practice. No use, distribution or reproduction is permitted which does not comply with these terms.
*Correspondence: Jean-Paul Nguyen, amVhbnBhdWwubmd1eWUmI3gwMDA0MDtnbWFpbC5jb20=; Tiphanie Caloc'h, dGlwaGFuaWUuY2Fsb2NoJiN4MDAwNDA7bGFwb3N0ZS5uZXQ=; Estelle Le Saout, ZXN0ZWxsZS5sZXNhb3V0JiN4MDAwNDA7Y2xpbmlxdWUtYnJldGVjaGUuZnI=; Séverine Litaneur, c2V2ZXJpbmUubGl0YW5ldXImI3gwMDA0MDtjbGluaXF1ZS1icmV0ZWNoZS5mcg==; Alcira Suarez, YWxjaXJhc3VtJiN4MDAwNDA7eWFob28uZnI=; Sylvain Durand, bG9hbGRlc3kmI3gwMDA0MDtvcmFuZ2UuZnI=; Jean-Pascal Lefaucheur, amVhbi1wYXNjYWwubGVmYXVjaGV1ciYjeDAwMDQwO2FwaHAuZnI=