- 1Department of Pediatrics, University of Wisconsin–Madison, Madison, WI, United States
- 2Department of Internal Medicine, University of Texas Southwestern Medical Center, Dallas, TX, United States
- 3Medical Oxygen Outpatient Clinic, The American Center, Madison, WI, United States
Introduction: Hyperbaric air (HBA) was first used pharmaceutically in 1662 to treat lung disease. Extensive use in Europe and North America followed throughout the 19th century to treat pulmonary and neurological disorders. HBA reached its zenith in the early 20th century when cyanotic, moribund “Spanish flu pandemic” patients turned normal color and regained consciousness within minutes after HBA treatment. Since that time the 78% Nitrogen fraction in HBA has been completely displaced by 100% oxygen to create the modern pharmaceutical hyperbaric oxygen therapy (HBOT), a powerful treatment that is FDA approved for multiple indications. Current belief purports oxygen as the active element mobilizing stem progenitor cells (SPCs) in HBOT, but hyperbaric air, which increases tensions of both oxygen and nitrogen, has been untested until now. In this study we test HBA for SPC mobilization, cytokine and chemokine expression, and complete blood count.
Methods: Ten 34–35-year-old healthy volunteers were exposed to 1.27ATA (4 psig/965 mmHg) room air for 90 min, M-F, for 10 exposures over 2-weeks. Venous blood samples were taken: (1) prior to the first exposure (served as the control for each subject), (2) directly after the first exposure (to measure the acute effect), (3) immediately prior to the ninth exposure (to measure the chronic effect), and (4) 3 days after the completion of tenth/final exposure (to assess durability). SPCs were gated by blinded scientists using Flow Cytometry.
Results: SPCs (CD45dim/CD34+/CD133-) were mobilized by nearly two-fold following 9 exposures (p = 0.02) increasing to three-fold 72-h post completion of the final (10th) exposure (p = 0.008) confirming durability.
Discussion: This research demonstrates that SPCs are mobilized, and cytokines are modulated by hyperbaric air. HBA likely is a therapeutic treatment. Previously published research using HBA placebos should be re-evaluated to reflect a dose treatment finding rather than finding a placebo effect. Our findings of SPC mobilization by HBA support further investigation into hyperbaric air as a pharmaceutical/therapy.
Introduction
Presently, hyperbaric air (HBA) is not thought of as a medication. Its singular approved medicinal use is for Acute Mountain Sickness. Historically it has been used medicinally, initially reported by Henshaw in 1662 to treat “afflictions of the lungs” (1) and additional reports from Europe and America followed (1). In 1857 Simpson published a paper using HBA to treat lung pathologies including tuberculosis (2). Interest in hyperbaric air as a medication surged following successful treatments of “Spanish flu” patients by Cunningham in 1918 (3–5). Unfortunately, Cunningham produced a paucity of papers supporting his work and when he died in 1937, interest in hyperbaric air abated.
HBA began to be employed again in the modern era with controversial results. It was used as a placebo by Collet while investigating the use of hyperbaric oxygen in Cerebral Palsy (6) and also as a placebo in brain injury by Miller, Wolf and Cifu (7–9). In each of these experiments, both the treatment group and the placebo group improved revealing an apparent placebo effect. The use of hyperbaric air as a placebo is energetically debated and controversial because it increases the oxygen tension. In contrast to the placebo findings, similar published studies not using the hyperbaric air placebo, found significant improvements in brain injury (10–20).
Considering the aforementioned, we asked the question, “is hyperbaric air an appropriate placebo?”. We searched the literature and found no evidence that hyperbaric air has been tested. We designed a test of hyperbaric air using a gold standard endpoint of oxygen therapy, stem cell mobilization.
Our specific aims were (1) to characterize stem cell mobilization in healthy adults following daily exposures to hyperbaric air, (2) determine if other biomarkers were modulated, (3) determine if there were acute changes, and (4) if so, were the changes durable. Based on previous research done in our lab (21) and calculating a 27% increase in oxygen partial pressures in the inhaled gases, we hypothesized that (1) stem cells would be mobilized, (2) biomarkers would be modulated (3) there would be acute changes and (4) the stem cell mobilization would be durable.
Materials and methods
Design and subjects
This prospective hyperbaric air study was a randomized, single-blind study conducted at the University of Wisconsin – Madison Clinical Sciences Center between May 1st, 2021, and August 31st, 2021. This study is approved by the Institutional Review Board of the University of Wisconsin – Madison. UW IRB ID: 2020-0293-CR001. All participants provided written informed consent. Healthy adults were recruited for participation in this study (Table 1).
Hyperbaric air exposure
All exposures were at 1.27 ATA (4 psig) of room air for 90 min in a Gamow style Mountain Sickness Chamber (Hyperbaric Technologies Inc. Amsterdam, NY, United States). To minimize circadian cell cycle variations including acrophase and circannual cycle, all subjects were diurnally active and all hyperbaric exposures and sample collections occurred at the same time of day over the contiguous 15-day experiment.
Sample collection
Each peripheral venous blood sample was collected during the subject’s exposure time slot using either a 21- or 23-gauge BD Vacutainer Safety-Lok Blood Collection Set (Becton, Dickinson and Company, Franklin Lakes, NJ, United States) into a Cyto-Chex BCT tube (Streck Inc. NE USA), BD Vacutainer Plastic Blood Collection Tubes with K2EDTA, BD Vacutainer Plastic Blood Collection Tubes – PST Plasma Separation Tubes, and Greiner Bio-One K2EDTA GelTubes. All samples were stored according to manufacturer’s directions. Study protocol in graphic format is included in Figure 1.
Flow cytometry
Fluorescence minus one (FMO) tubes, rainbow bead tubes, and single antibody tubes were prepared as gating references. Antibodies were pipetted into flow cytometry tubes according to manufacturer’s instructions. Antibodies used include CD34 = Brilliant Violet 421 (BioLegend, San Diego, CA, United States), CD45 = Alexa Fluor 488 (BioLegend, San Diego, CA, United States), CD133 = PE (Miltenyi Biotec, North Rhine-Westphalia, Germany) CD31 = Brilliant Violet 605 (BioLegend, San Diego, CA, United States), CD105 = PE-Cy7 (BioLegend, San Diego, CA, United States), Ghost Dye Red 780 = Tonbo Biosciences, San Diego, CA.
Flow cytometry was performed by blinded scientists on a ThermoFisher Attune NxT (Waltham, MA, United States). Samples were analyzed by blinded scientists using FlowJo software (FlowJo, Ashland, OR, United States).
Enzyme-linked immunosorbent assay
The Invitrogen ProcartaPlex™ Human Immune Monitoring Panel 65-Plex (Invitrogen, Waltham, MA, United States) was used to assess changes in cytokines, chemokines and growth factors. All tests were performed by blinded scientists at the University of Wisconsin Non-Human Primate Research Center (Madison, WI, United States).
Statistical analysis
The first blood draw taken prior to the first exposure served as the control. To determine whether there was an overall effect across exposures, we utilized the Friedman test (nonparametric alternative to one-way ANVOA with repeated measures) and if significant, comparisons between all-time points were performed using the Wilcoxon signed rank test. Significance level was determined a priori at the 0.05 level and all tests were two-tailed. Statistical analyses were calculated using Graph Pad Prism (GraphPad Prism 9.0.0 Software, San Diego, CA, United States).
Results
CD45dim
Increased frequency of CD45dim/CD34+/CD133− following nominal exposure to hyperbaric room air
As previously described 10 humans were exposed to 1.27 ATA of room air 10 times over the course of a 12-day period. Results revealed a significant increase in the frequency of CD45dim/CD34+/CD133− stem progenitor cells in venous blood resulting in an approximate two-fold increase directly prior to the 10th exposure (p = 0.02). CD45dim/CD34+/CD133− SPCs continued to increase during the 3 days following the end of exposures and increased to three-fold, 72 h after the 10th exposure (p = 0.008); (Figure 2A).
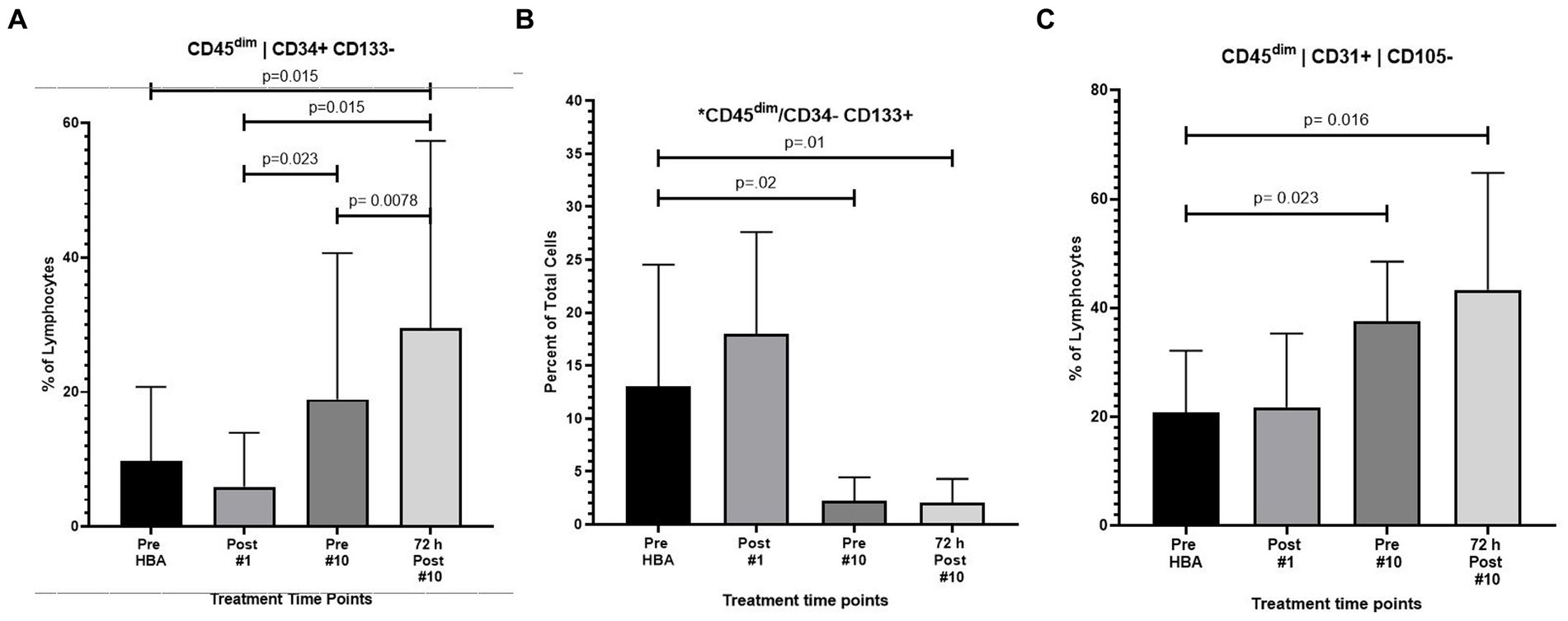
Figure 2. Frequency of CD45dim/CD34+/CD133-, CD45dim/CD34-/CD133+ and CD45dim/CD31+/CD105-after intermittent hyperbaric air exposure detected by flow cytometry. (A) CD34+ and CD133- stem progenitor cells. (B) CD34- and CD133+ stem progenitor cells. (C) CD31+ and CD105- stem progenitor cells.
Decreased frequency of CD45dim/CD34−/CD133+ after hyperbaric exposure
While CD45dim/CD34+/CD133− SPCs increased, the frequency of CD45dim/CD34−/CD133+ primitive pro-angiogenic stem progenitor cells significantly decreased after exposure to intermittent hyperbaric air. CD45dim/CD34−/CD133+ decreased by nearly five-fold prior to the 10th exposure (p = 0.02) and mobilization decreased by six-fold 72 h after the 10th exposure (p = 0.01) (Figure 2B).
Increased frequency of CD45dim/CD31+/CD105− after hyperbaric exposure
The frequency of CD45dim/CD31+/CD105- was also significantly increased after hyperbaric exposure by nearly two-fold prior to the 10th exposure (p = 0.023) and increased to over two-fold 3 days after the 10th exposure (p = 0.016) (Figure 2C).
Mobilization of CD45+/CD34+/CD133−
The expression of CD45+/CD34+/CD133- also increased by nearly 3.5 fold after 9 full exposures (p = 0.012) and increased to over four fold 72 h following the end of the 10th exposure (p = 0.002) (Figure 3A).
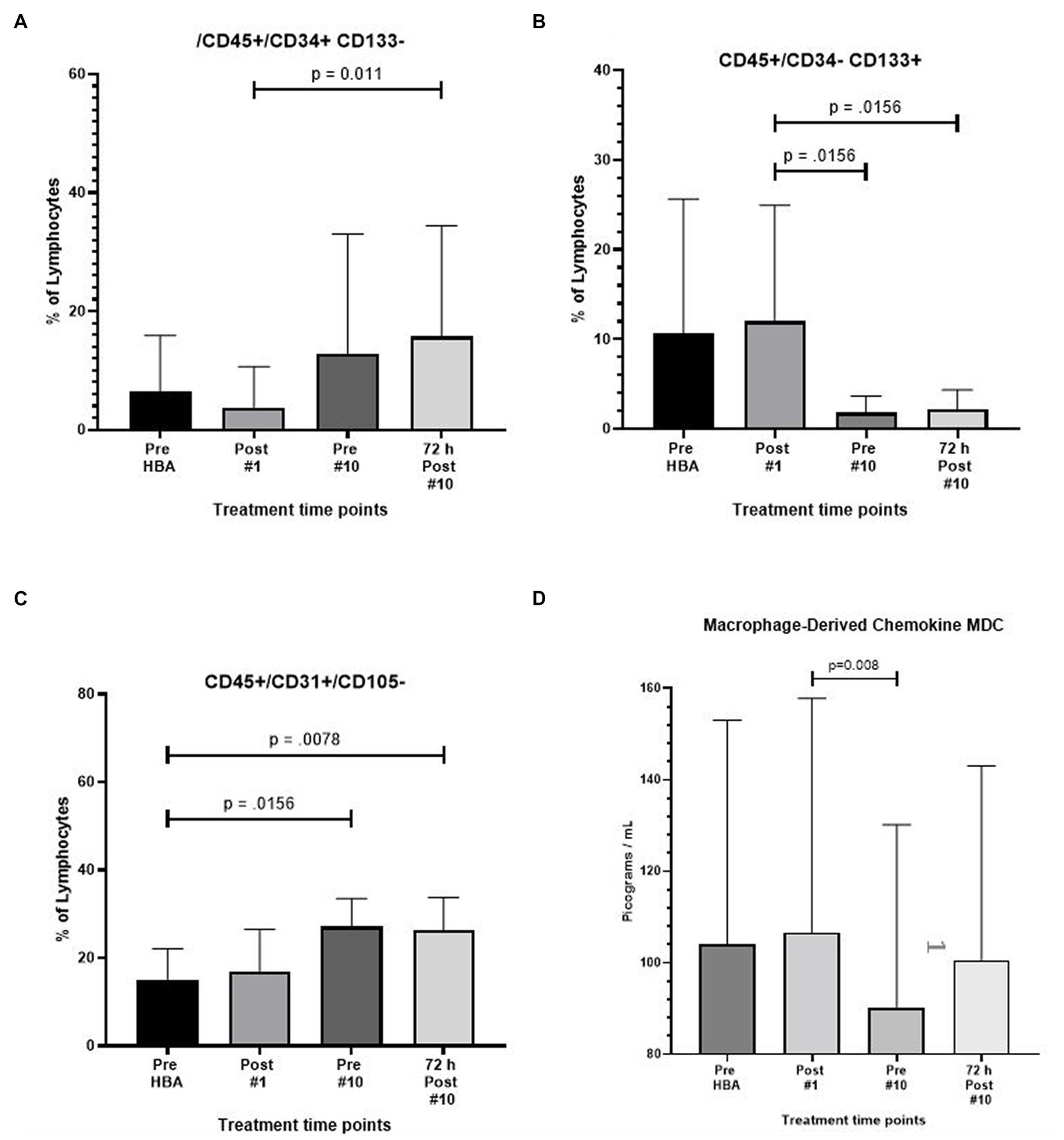
Figure 3. Frequency of CD45+/CD34+/CD133-, CD45+/CD34-/CD133+, CD45+/CD31+/CD105-, and macrophage derived chemokine after intermittent hyperbaric air exposure detected by flow cytometry and ELISA. (A) CD34+ and CD133- stem progenitor cells. (B) CD34- and CD133+ stem progenitor cells. (C) CD31+ and CD105- stem progenitor cells. (D) Macrophage derived chemokine.
Decreased frequency of CD45+/CD34−/CD133+
A significant reduction in the expression of CD45+/CD34−/CD133+ was noted resulting in a six-fold reduction prior to the 10th exposure (p = 0.016) and recovering to 5.4-fold 72 h after the 10th and final exposure (p = 0.016) (Figure 3B).
Mobilization of CD45+/CD31+/CD105−
The expression of CD45+/CD31+/105- increased by nearly two-fold after 9 full exposures (p = 0.02) and remained at the level through 72 h following the end of the 10th exposure (p = 0.008) (Figure 3C).
Complete results from flow cytometry
Complete results from flow cytometry testing, including non-significant findings are included in tabular form for reference. (Table 2).
Macrophage-derived chemokine (MDC) expression significantly decreased
Results from the Invitrogen ProcartaPlex™ Human Immune Monitoring Panel 65-Plex ELISA like analysis showed there was only one change to report. The expression of Macrophage-derived Chemokine (MDC) was significantly lower between the second and third time points (p = 0.008). All other tests revealed no change (Figure 3D).
Complete blood count with differential
There were no significant changes in CBC at any time points (Table 2).
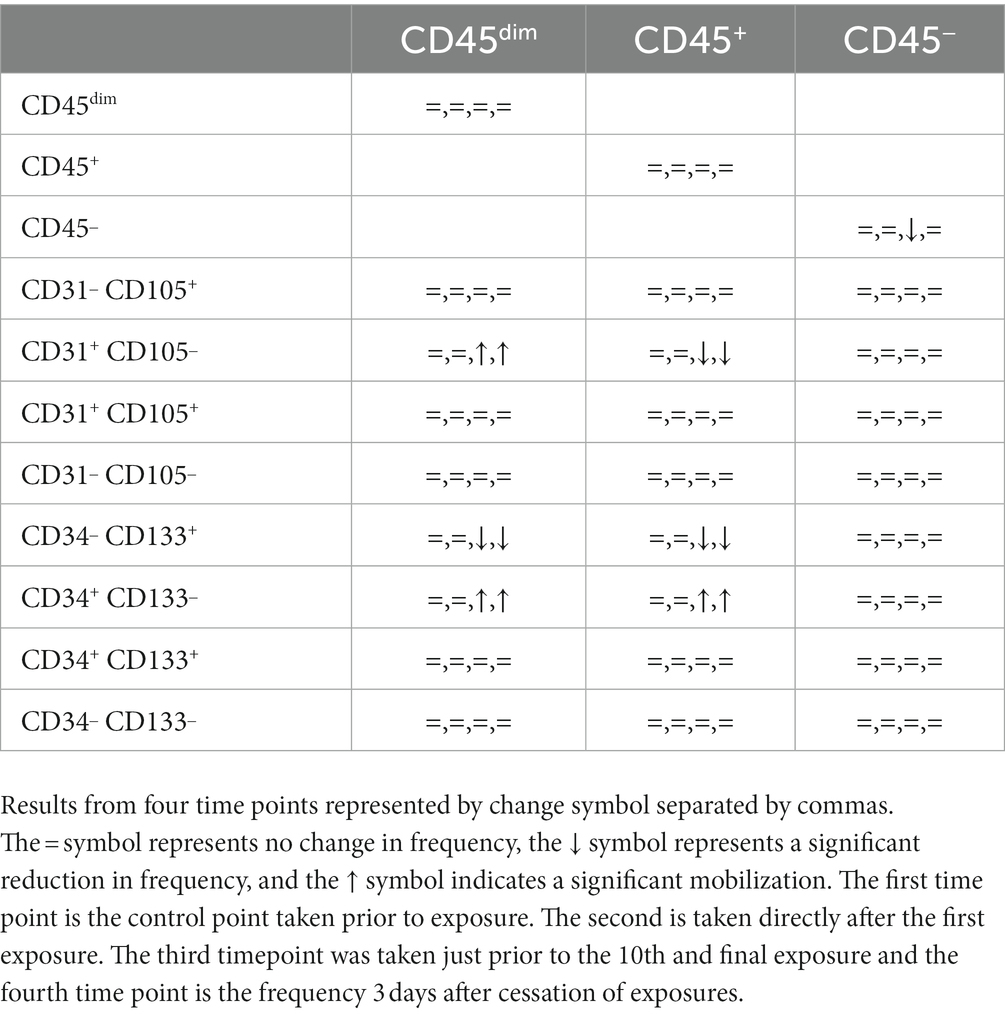
Table 2. Summary of cell type frequency after initial and intermittent exposure detected by flow cytometry.
Discussion
The smallest dose of hyperbaric air that will result in a therapeutic effect is unknown and strongly debated among scientists and physicians. Pressures below 1.4 atmospheres absolute of hyperbaric air are accepted as a placebo but have not previously been tested in humans. Studies using hyperbaric air as a placebo have resulted in a “placebo” or “participation” effect (7–9). This placebo effect finding is vigorously disputed because hyperbaric air significantly increases the partial pressure of oxygen (and nitrogen) in the inspired air (22–27). The “placebo effect” findings have effectively restricted the use of HBOT for many conditions including Traumatic Brain Injury in soldiers returning from combat.
In this study we asked the question, will a small dose of hyperbaric air (1.27 ATA), that is below the accepted 1.4 ATA therapeutic threshold, mobilize stem cells similar to HBOT? We hypothesized that stem cells would be mobilized.
Indeed, stem cells were significantly mobilized, refuting previous “placebo effect” findings. We intend that the results will provide needed experimental data to medical societies and journal editors, and in turn provide guidance to FDA and physicians.
Critical analysis of major findings
Testing for stem cell mobilization using flow cytometry and gated by blinded scientists, the major finding of this study is that 1.27 ATA hyperbaric air mobilizes CD34+/CD133- SPCs and CD31+/CD105- stem cells in humans receiving 10 daily 90-min exposures (Figures 2A,C, respectively). Mobilization of CD34+ SPCs has also been observed in isobaric hyperoxic (21) and in hyperbaric hyperoxic conditions (28, 29).
The mechanism of hyperbaric air SPC mobilization in this experiment is beyond the scope of this study, but may be similar to SPC mobilization found in hyperbaric oxygen therapy, which activates nitric oxide synthase and plays a prime role in initiating CD34+ SPC mobilization (30–33). (CD34 background) CD34+ adult stem/progenitor cells are a group of specific cell types that possess the abilities of self-renewal and multipotent differentiation (34, 35).
CD34+ is expressed on hematopoietic and pro-angiogenic stem progenitor cells and on endothelium (36). Pro-angiogenic stem/progenitor cells contribute to neovascularization by a process of homing to ischemic tissue called vasculogenesis, and by budding endothelium from established blood vessels in a process called angiogenesis (37, 38). Further research is needed to understand the homing and function of the CD34+ SPCs mobilized by intermittent hyperbaric air exposures.
A second significant finding of this study, which we report for the first time, is hyperbaric air mobilizes CD31+/CD105- SPCs. This novel finding has many implications in the field of hyperbaric air and hyperbaric oxygen. (CD31+ background) CD31 is thought to have a protective role in experimental atherosclerosis (39). The therapeutic potential of CD31 agonists to manage atherosclerotic disease manifestations is a consistent finding in pre-clinical studies (40). Although this is the first time that CD31 has been reported to be mobilized by either hyperbaric air or hyperbaric oxygen, it is not unexpected, given that CD31 is associated with endothelial function much like CD34 and CD133. It was beyond the scope of this research project to determine if these mobilized CD31 stem cells participate in wound healing and angiogenesis. Future research testing the effect of hyperbaric air mobilized CD31 stem cells on healing, angiogenesis, and atherosclerotic disease states would be prudent.
Our results also revealed an interesting relationship between SPCs expressing CD45dim/CD34+/CD133− and those SPCs expressing CD45dim/CD34−/CD133+. CD133+ SPCs are hematopoietic precursors to CD34+ and almost all hematopoietic pluripotent and committed stem cells in colony-forming assays express CD34+ (36). In this study we found that CD34+ SPCs increased while at the same time CD133+ SPCs decreased. We hypothesize that the exposure to intermittent hyperbaric air may mobilize CD133+ from bone marrow, and also play a role in the differentiation of the CD133+ primitive hematopoietic precursor into the CD34+ SPC. However, it has also been shown that CD133+ have a high capacity to differentiate into other cell types including fibroblasts, hepatocytes, and neural cell-types (35). Unfolding the explanation for the significant reduction in the expression of CD45dim/CD34−/CD133+ expressing SPCs holds exciting potential.
Previous research has shown that breathing 100% oxygen at 2.4 atmospheres absolute mobilized stem/progenitor cells at a significantly greater rate than 2.0 Atmospheres Absolute suggesting a dose effect (41). HBOT at 2.0 ATA (PIO2 of 1426 mmHg and PIN2 of 0 mmHg) resulted in a two-fold mobilization of CD34+ SPCs after 1 exposure and an eight-fold increase after 20 exposures (28). In this study using 1.27 ATA of hyperbaric air (PIO2 of 189 mmHg and PIN2 of 706 mmHg) we saw a two-fold increase in SPC mobilization just prior to the 9th exposure. This supports the hypothetical relationship between oxygen dose and stem cell mobilization.
Another aim of this study was to determine if the stem progenitor cell mobilization was durable. Because the stem progenitor cell mobilization increased another fold following the end of exposures a durable effect is likely.
Interestingly, we also found changes in cells expressing CD45+ cell subtypes. These changes were remarkably similar to changes in the CD45dim population. CD45+ is expressed on all hematopoietic cells, including HSCs and osteoclasts, which are of hematopoietic origin (42), and is known as a pan-leukocyte marker.
Macrophage-derived chemokine (MDC) expressed in venous blood significantly decreased prior to the ninth exposure and returned to pre-exposure levels 72 h following the final exposure. The expression of MDC is increased in idiopathic pulmonary fibrosis (43) and elevated following resuscitation of patients after hemorrhage. Macrophage-derived chemokine may provide a therapeutic strategy to mitigate this inflammatory response (44). MDC is also thought to serve as a marker of pharmacological therapy response in Major Depressive Disorder (45).
Changes in barometric and hydrostatic pressure may be a mechanism of hyperbaric therapy. Small changes in atmospheric pressure elicit responses in many organisms (46). However the mechanism(s) is poorly understood. One possibility is that pressure is sensed through hydrostatic compression of heterogeneous structures. For instance, cells in suspension, including platelets (47, 48) and cartilage cells (49, 50), respond to small changes in pressure ostensibly through this mechanism. Another possibility is that cellular structures may produce shear and strain through differential compression. Microtubules, actin and other cytoskeletal proteins respond to this type of local mechanical stresses (51, 52). It should also be noted that certain animals appear to respond to changes in atmospheric (air) pressure. For example, pigeons exhibit changes in heart rate in response to changes of about 1 mbar, which is a typical daily fluctuation in barometric pressure. But in such cases, it is unclear how barometric pressure changes are sensed (53). It is probable that changes in pressure have effects on human physiology.
Another possible mechanism ultimately resulting in stem cell mobilization in this experiment is a progressive accumulation of endogenous anti-oxidants at the cellular level. These antioxidants accrue in response to repeated exposure to a hyperoxic environment. The result is an up-regulation of the Hypoxia-inducible factor 1α (HIF-1α) transcription factor activity (54–60). This hypothetical mechanism is best described as the Normoxic Hypoxic Paradox. This mechanism is best characterized by an increase in reactive oxygen species (ROS) due to the hyperoxic cellular environment. The increased cellular ROS creates an imbalance of ROS/scavenger antioxidants ratio. The increased ROS molecules initially hydroxylate most of the HIF-1α, facilitating ubiquitination and degradation of most of the HIF-1α subunits. However, it is postulated that an adaptive response to repeated hyperoxia, increases the production of scavengers in proportion to the increased ROS generation. The ROS/scavenger ratio gradually becomes balanced in the hyperoxic environment. However, when the hyperoxic exposure ends and the cell returns to a normoxic state, the ROS/ scavenger ratio is again imbalanced, but this time with more scavengers than ROS, which produces a reduced ROS environment. Less ROS leads to an increase in HIF-1α, initiating HIF transcription factor activity, resembling a hypoxic state, but in a normoxic environment. In this study CD34+/CD133- expression trended downward directly following the initial hyperbaric air exposure, but increased significantly prior to the tenth exposure. The opposite was true with CD34-/CD133+. These results support the hypothetical Normoxic Hypoxic Paradox mechanism.
While our study clearly showed that the hyperbaric air dose that was used as a placebo mobilizes proangiogenic and hematopoietic stem progenitor cells and likely has a therapeutic physiologic effect similar to hyperbaric oxygen therapy (29, 61–63), it was beyond the scope of this study to determine clinical significance. Hopefully our results will generate renewed interest in hyperbaric air and future studies will investigate both mechanism and clinical outcomes.
Limitations
The major limitation of our study is a relatively small sample size.
Future directions
It should not be overlooked that although we increased the oxygen partial pressure and presume that oxygen is the active element in hematopoietic and pro-angiogenic stem progenitor mobilization, the partial pressure of nitrogen and remaining trace gasses are also increased. Nitrogen is not an inert element and can form five potential oxidation states and three potential reduction states with various levels of reactivity (64). Recent evidence suggests that intracellular reactive oxygen and nitrogen species play an important role in intracellular signaling cascades (65). However, little is known about the effect of nitrogen and trace gasses on SPC mobilization or cytokine, chemokine and growth factor modulation. Increased nitrogen and trace gasses as a stimulator of SPC mobilization and chemokine modulation is a possible mechanism in this research project and an exciting idea to explore in future research.
It is very possible that stem cell mobilization by hyperbaric air provides an additional modality to heal injuries. Because of its reduced cost of delivery, it may prove beneficial in developing nations and in underserved populations. Hyperbaric air also increases safety because the oxygen partial pressure is relatively low. The low oxygen partial pressure in hyperbaric air may also provide increased therapeutic value by not overloading the cerebral energy metabolism balance (66, 67).
Finally, our data suggests that the therapeutic dose of oxygen begins at a much smaller partial pressure than previously thought and adds a new data point on the initial portion of the hormetic curve of oxygen dose.
Although we found that intermittent small increases in hyperbaric air pressure mobilized stem cells, this study should not be taken as an endorsement of the use of intermittent hyperbaric air for any purpose other than the indications approved by the FDA.
Why are these findings important? First, prior to this research it was not known that breathing a small increase in hyperbaric air would mobilize stem progenitor cells. This knowledge could be an important low-cost healthcare option when hyperbaric oxygen is not available, especially in underserved populations, remote areas, and developing nations. Second, because the technology is lightweight and portable, it is hypothetically possible to be used when transporting combatants and civilians out of a warzone to extend the viability of damaged tissue and to reduce exacerbating gas embolism when air-lifting by high altitude flights is required. Finally, this research refutes the findings of a placebo effect in the decades-old use of slightly pressurized room air as a placebo in hyperbaric oxygen research.
Conclusion and impact
In this study we demonstrate for the first time that intermittent exposure to ostensibly insignificant pressures of hyperbaric air mobilizes stem progenitor cells in a similar manner to that seen in isobaric hyperoxia and hyperbaric oxygen therapy (21, 28, 29, 41). We also establish that the stem progenitor cell mobilization is durable.
This research reveals that hyperbaric air, even at an ostensibly insignificant dose, has significant effects on human physiology, is not a placebo, and should be considered as an active physiologic intervention. Its use as a pharmaceutical should be investigated further.
Although this study did not test for clinical results, clinical outcomes of hyperbaric air have been reported in similar hyperbaric air studies (6–8). This study supports the data, but refutes the conclusions in those studies by revealing a mechanism of action for the clinical improvements reported in the hyperbaric air group in those studies. Much more work is needed to develop protocols of hyperbaric air dose that provide the maximum therapeutic benefit.
These findings substantiate the need for testing hyperbaric air doses prior to using hyperbaric air as a placebo in scientific investigations. These findings also substantiate the urgent need for reevaluation of findings in historical studies using hyperbaric air placebos. Our findings suggest that these historical placebo-controlled studies were not placebo-controlled studies. Paradoxically, our findings indicate that they were dose studies and the findings of a “placebo effect” or “participation effect” are inherently flawed. The “findings” and “conclusions” in studies using hyperbaric air as a placebo should be reevaluated from a dose study perspective. Finally, we hope the findings in this study will persuade the medical societies around the world to consider reevaluation of their definition of hyperbaric medicine to include nominal hyperbaric air.
Looking back at the “Hyperbaric Air” work of Henshaw, Simpson and Cunningham, our findings support their reports.
Data availability statement
The original contributions presented in the study are included in the article/supplementary material, further inquiries can be directed to the corresponding author.
Ethics statement
The studies involving human participants were reviewed and approved by the University of Wisconsin–Madison. The patients/participants provided their written informed consent to participate in this study.
Author contributions
KM and GB: ideas conception. KM, GB, RB, and ME: study and experiments design. KM, JM, RB, JL, and MM: experiments perform, data acquisition, and analysis. GB and RB: guidance and critical feedback on data acquisition and analysis. RB and ME: project supervision. KM: manuscript writing. All authors provided critical feedback and contributed to the final version of manuscript.
Funding
This work was supported by the Foundation for the Study of Inflammatory Disease and the International Hyperbaric Association.
Acknowledgments
The authors would like to thank the study participants for their time and dedication to complete this study. Thanks also goes to Caleb and Sidney Gates, Dr. Rob Beckman, Dr. Vivek Balasubramanium, Dr. Barb Bendlin, Dr. Robert Stone, Dr. William Schrage, Dr. Paul Harch, Dr. Phillip James, Dr. Don Wollheim, Dr. Donata Oertel, Dr. Awni Al-Subu, Dr Kara Goss, Dr. Arij Beshish, Dr. Andrew Watson, Dr. Xavier Figueroa, Dr. Daphne Denham, Tom Fox, Freya Christensen, the University of Wisconsin CCC Flow Cytometry Lab, Dagna Sherrar, Lauren Nettenstrom, Kathryn Fox and Zach Sterneson, the Univeristy of Wisconsin-Madison Department of Pediatrics and Gradaute School, Dr. Ellen Wald, Dr. James Gern, and Kim Stevenson for the support.
Conflict of interest
The authors declare that the research was conducted in the absence of any commercial or financial relationships that could be construed as a potential conflict of interest.
Publisher’s note
All claims expressed in this article are solely those of the authors and do not necessarily represent those of their affiliated organizations, or those of the publisher, the editors and the reviewers. Any product that may be evaluated in this article, or claim that may be made by its manufacturer, is not guaranteed or endorsed by the publisher.
References
2. Simpson, A. Compressed Air as a Therapeutic Agent in the Treatment of Consumption, Asthma, Chronic Bronchitis, and Other Diseases. Glasgow Med J. (1857) 5:94–6.
3. Sellers, L.M. The fallibility of the FORRESTIAN principle. "semper primus PERVENIO maxima cum Vi". (ORVAL JAMES Cunningham). Transactions of the American Laryngological, Rhinological and Otological Society, Inc 23, 385–405 (1964).
4. Harch, P. New scientific definitions: hyperbaric therapy and hyperbaric oxygen therapy. Med Gas Res. (2023) 13:92–3. doi: 10.4103/2045-9912.356475
5. Trimble, VH. The uncertain miracle: hyperbaric oxygenation. Garden City, NY: Doubleday & Company, Inc (1974).
6. Collet, JP, Vanasse, M, Marois, P, Amar, M, Goldberg, J, Lambert, J, et al. Hyperbaric oxygen for children with cerebral palsy: a randomised multicentre trial. HBO-CP research group. Lancet. (2001) 357:582–6. doi: 10.1016/S0140-6736(00)04054-X
7. Miller, RS, Weaver, LK, Bahraini, N, Churchill, S, Price, RC, Skiba, V, et al. Effects of hyperbaric oxygen on symptoms and quality of life among service members with persistent postconcussion symptoms: a randomized clinical trial. JAMA Intern Med. (2015) 175:43–52. doi: 10.1001/jamainternmed.2014.5479
8. Wolf, G, Cifu, D, Baugh, L, Carne, W, and Profenna, L. The effect of hyperbaric oxygen on symptoms after mild traumatic brain injury. J Neurotrauma. (2012) 29:2606–12. doi: 10.1089/neu.2012.2549
9. Cifu, DX, Walker, WC, West, SL, Hart, BB, Franke, LM, Sima, A, et al. Hyperbaric oxygen for blast-related postconcussion syndrome: three-month outcomes. Ann Neurol. (2014) 75:277–86. doi: 10.1002/ana.24067
10. Harch, PG, and Fogarty, EF. Subacute normobaric oxygen and hyperbaric oxygen therapy in drowning, reversal of brain volume loss: a case report. Med Gas Res. (2017) 7:144–9. doi: 10.4103/2045-9912.208521
11. Harch, PG, Fogarty, EF, Staab, PK, and Van Meter, K. Low pressure hyperbaric oxygen therapy and SPECT brain imaging in the treatment of blast-induced chronic traumatic brain injury (post-concussion syndrome) and post traumatic stress disorder: a case report. Cases J. (2009) 2:6538. doi: 10.1186/1757-1626-0002-0000006538
12. Harch, PG, Andrews, SR, Fogarty, EF, Amen, D, Pezzullo, JC, Lucarini, J, et al. A phase I study of low-pressure hyperbaric oxygen therapy for blast-induced post-concussion syndrome and post-traumatic stress disorder. J Neurotrauma. (2012) 29:168–85. doi: 10.1089/neu.2011.1895
13. Hadanny, A, Catalogna, M, Yaniv, S, Stolar, O, Rothstein, L, Shabi, A, et al. Hyperbaric oxygen therapy in children with post-concussion syndrome improves cognitive and behavioral function: a randomized controlled trial. Sci Rep. (2022) 12:15233. doi: 10.1038/s41598-022-19395-y
14. Hadanny, A, Rittblat, M, Bitterman, M, May-Raz, I, Suzin, G, Boussi-Gross, R, et al. Hyperbaric oxygen therapy improves neurocognitive functions of post-stroke patients - a retrospective analysis. Restor Neurol Neurosci. (2020) 38:93–107. doi: 10.3233/RNN-190959
15. Doenyas-Barak, K, Catalogna, M, Kutz, I, Levi, G, Hadanny, A, Tal, S, et al. Hyperbaric oxygen therapy improves symptoms, brain's microstructure and functionality in veterans with treatment resistant post-traumatic stress disorder: a prospective, randomized, controlled trial. PLoS One. (2022) 17:e0264161. doi: 10.1371/journal.pone.0264161
16. Hadanny, A, Golan, H, Fishlev, G, Bechor, Y, Volkov, O, Suzin, G, et al. Hyperbaric oxygen can induce neuroplasticity and improve cognitive functions of patients suffering from anoxic brain damage. Restor Neurol Neurosci. (2015) 33:471–86. doi: 10.3233/RNN-150517
17. Boussi-Gross, R, Golan, H, Volkov, O, Bechor, Y, Hoofien, D, Schnaider Beeri, M, et al. Improvement of memory impairments in poststroke patients by hyperbaric oxygen therapy. Neuropsychology. (2015) 29:610–21. doi: 10.1037/neu0000149
18. Tal, S, Hadanny, A, Sasson, E, Suzin, G, and Efrati, S. Hyperbaric oxygen therapy can induce angiogenesis and regeneration of nerve fibers in traumatic brain injury patients. Front Hum Neurosci. (2017) 11:508. doi: 10.3389/fnhum.2017.00508
19. Boussi-Gross, R, Golan, H, Fishlev, G, Bechor, Y, Volkov, O, Bergan, J, et al. Hyperbaric oxygen therapy can improve post concussion syndrome years after mild traumatic brain injury - randomized prospective trial. PLoS One. (2013) 8:e79995. doi: 10.1371/journal.pone.0079995
20. Efrati, S, Fishlev, G, Bechor, Y, Volkov, O, Bergan, J, Kliakhandler, K, et al. Hyperbaric oxygen induces late neuroplasticity in post stroke patients--randomized, prospective trial. PLoS One. (2013) 8:e53716. doi: 10.1371/journal.pone.0053716
21. MacLaughlin, K, Barton, G, Braun, R, and Eldridge, M. Effect of intermittent hyperoxia on stem cell mobilization and cytokine expression. Med Gas Res. (2019) 9:139–44. doi: 10.4103/2045-9912.266989
22. Harch, PG. Systematic review and dosage analysis: hyperbaric oxygen therapy efficacy in mild traumatic brain injury persistent Postconcussion syndrome. Front Neurol. (2022) 13:815056. doi: 10.3389/fneur.2022.815056
23. Hoge, CW, and Jonas, WB. The ritual of hyperbaric oxygen and lessons for the treatment of persistent postconcussion symptoms in military personnel. JAMA Intern Med. (2015) 175:53–4. doi: 10.1001/jamainternmed.2014.3375
24. Hoge, CW, and Jonas, WB. Hyperbaric oxygen treatment for persistent Postconcussion symptoms--reply. JAMA Intern Med. (2015) 175:1241. doi: 10.1001/jamainternmed.2015.1051
25. Hu, Q, Manaenko, A, Guo, Z, Huang, L, Tang, J, and Zhang, JH. Hyperbaric oxygen therapy for post concussion symptoms: issues may affect the results. Med Gas Res. (2015) 5:10. doi: 10.1186/s13618-015-0033-3
26. Marois, P, Mukherjee, A, and Ballaz, L. Hyperbaric oxygen treatment for persistent Postconcussion symptoms--a placebo effect? JAMA Intern Med. (2015) 175:1239–40. doi: 10.1001/jamainternmed.2015.1032
27. Miller, RS, Weaver, LK, and Brenner, LA. Hyperbaric oxygen treatment for persistent Postconcussion symptoms--reply. JAMA Intern Med. (2015) 175:1240–1. doi: 10.1001/jamainternmed.2015.1048
28. Thom, SR, Bhopale, VM, Velazquez, OC, Goldstein, LJ, Thom, LH, and Buerk, DG. Stem cell mobilization by hyperbaric oxygen. Am J Physiol Heart Circ Physiol. (2006) 290:H1378–86. doi: 10.1152/ajpheart.00888.2005
29. Thom, SR, Milovanova, TN, Yang, M, Bhopale, VM, Sorokina, EM, Uzun, G, et al. Vasculogenic stem cell mobilization and wound recruitment in diabetic patients: increased cell number and intracellular regulatory protein content associated with hyperbaric oxygen therapy. Wound Repair Regen. (2011) 19:149–61. doi: 10.1111/j.1524-475X.2010.00660.x
30. Aicher, A, Heeschen, C, Mildner-Rihm, C, Urbich, C, Ihling, C, Technau-Ihling, K, et al. Essential role of endothelial nitric oxide synthase for mobilization of stem and progenitor cells. Nat Med. (2003) 9:1370–6. doi: 10.1038/nm948
31. Thom, SR, Fisher, D, Zhang, J, Bhopale, VM, Ohnishi, ST, Kotake, Y, et al. Stimulation of perivascular nitric oxide synthesis by oxygen. Am J Physiol Heart Circ Physiol. (2003) 284:H1230–9. doi: 10.1152/ajpheart.01043.2002
32. Thom, SR, Bhopale, V, Fisher, D, Manevich, Y, Huang, PL, and Buerk, DG. Stimulation of nitric oxide synthase in cerebral cortex due to elevated partial pressures of oxygen: an oxidative stress response. J Neurobiol. (2002) 51:85–100. doi: 10.1002/neu.10044
33. Goldstein, LJ, Gallagher, KA, Bauer, SM, Bauer, RJ, Baireddy, V, Liu, ZJ, et al. Endothelial progenitor cell release into circulation is triggered by hyperoxia-induced increases in bone marrow nitric oxide. Stem Cells. (2006) 24:2309–18. doi: 10.1634/stemcells.2006-0010
34. Kitamura, S, Yamasaki, Y, Kinomura, M, Sugaya, T, Sugiyama, H, Maeshima, Y, et al. Establishment and characterization of renal progenitor like cells from S3 segment of nephron in rat adult kidney. FASEB J. (2005) 19:1789–97. doi: 10.1096/fj.05-3942com
35. Bachelier, K, Bergholz, C, and Friedrich, EB. Differentiation potential and functional properties of a CD34-CD133+ subpopulation of endothelial progenitor cells. Mol Med Rep. (2020) 21:501–7. doi: 10.3892/mmr.2019.10831
36. Faramarz, NP, Sophie, XS, and Ryan, TP. Chapter 2 – principles of immunophenotyping. Atlas Hematopathol. (2018):29–56. doi: 10.1016/B978-0-12-809843-1.00002-4
37. Rafii, S, and Lyden, D. Therapeutic stem and progenitor cell transplantation for organ vascularization and regeneration. Nat Med. (2003) 9:702–12. doi: 10.1038/nm0603-702
38. Pierro, M, Ciarmoli, E, and Thébaud, B. Stem cell therapy for neonatal lung diseases In: G Steinhoff, editor. Regenerative medicine - from protocol to patient: 4. Regenerative therapies I. Cham: Springer International Publishing (2016). 319–57.
39. Goel, R, Schrank, BR, Arora, S, Boylan, B, Fleming, B, Miura, H, et al. Site-specific effects of PECAM-1 on atherosclerosis in LDL receptor-deficient mice. Arterioscler Thromb Vasc Biol. (2008) 28:1996–2002. doi: 10.1161/ATVBAHA.108.172270
40. Caligiuri, G. CD31 as a therapeutic target in atherosclerosis. Circ Res. (2020) 126:1178–89. doi: 10.1161/CIRCRESAHA.120.315935
41. Heyboer, M 3rd, Milovanova, TN, Wojcik, S, Grant, W, Chin, M, Hardy, KR, et al. CD34+/CD45-dim stem cell mobilization by hyperbaric oxygen – changes with oxygen dosage. Stem Cell Res. (2014) 12:638–45. doi: 10.1016/j.scr.2014.02.005
42. Shivtiel, S, Kollet, O, Lapid, K, Schajnovitz, A, Goichberg, P, Kalinkovich, A, et al. CD45 regulates retention, motility, and numbers of hematopoietic progenitors, and affects osteoclast remodeling of metaphyseal trabecules. J Exp Med. (2008) 205:2381–95. doi: 10.1084/jem.20080072
43. Yogo, Y, Fujishima, S, Inoue, T, Saito, F, Shiomi, T, Yamaguchi, K, et al. Macrophage derived chemokine (CCL22), thymus and activation-regulated chemokine (CCL17), and CCR4 in idiopathic pulmonary fibrosis. Respir Res. (2009) 10:80. doi: 10.1186/1465-9921-10-80
44. Richter, JR, Sutton, JM, Belizaire, RM, Friend, LA, Schuster, RM, Johannigman, TA, et al. Macrophage-derived chemokine (CCL22) is a novel mediator of lung inflammation following hemorrhage and resuscitation. Shock. (2014) 42:525–31. doi: 10.1097/SHK.0000000000000253
45. Milenkovic, VM, Sarubin, N, Hilbert, S, Baghai, TC, Stöffler, F, Lima-Ojeda, JM, et al. Macrophage-derived chemokine: a putative marker of pharmacological therapy response in major depression? Neuroimmunomodulation. (2017) 24:106–12. doi: 10.1159/000479739
46. Macdonald, AG, and Fraser, PJ. The transduction of very small hydrostatic pressures. Comp Biochem Physiol A Mol Integr Physiol. (1999) 122:13–36. doi: 10.1016/S1095-6433(98)10173-3
47. Torsellini, A, Becucci, A, Citi, S, Cozzolino, F, Guidi, G, Lombardi, V, et al. Effects of pressure excursions on human platelets. In vitro studies on betathromboglobulin (beta-TG) and platelet factor 4 (PF4) release and on platelet sensitivity to ADP-aggregation. Haematologica. (1982) 67:860–6.
48. Torsellini, A, Doni, L, Palinski, W, Guidi, G, and Lombardi, V. Pressure-induced metabolic activation of platelets demonstrated in vitro by determination of malondialdehyde formation. Thromb Haemost. (1984) 52:4–6. doi: 10.1055/s-0038-1661123
49. Bourret, LA, and Rodan, GA. The role of calcium in the inhibition of cAMP accumulation in epiphyseal cartilage cells exposed to physiological pressure. J Cell Physiol. (1976) 88:353–61. doi: 10.1002/jcp.1040880311
50. Burger, EH, Klein-Nulend, J, and Veldhuijzen, JP. Mechanical stress and osteogenesis in vitro. J Bone Miner Res. (1992) 7:S397–401. doi: 10.1002/jbmr.5650071406
51. Buxbaum, RE, and Heidemann, SR. A thermodynamic model for force integration and microtubule assembly during axonal elongation. J Theor Biol. (1988) 134:379–90. doi: 10.1016/S0022-5193(88)80068-7
52. Wang, N, Butler, JP, and Ingber, DE. Mechanotransduction across the cell surface and through the cytoskeleton. Science. (1993) 260:1124–7. doi: 10.1126/science.7684161
53. Kreithen, ML, and Keeton, WT. Detection of changes in atmospheric pressure by the homing pigeon, Columba livia. J Compar. Physiol. (1974) 89:73–82. doi: 10.1007/BF00696164
54. Balestra, C, and Germonpre, P. Hypoxia, a multifaceted phenomenon: the example of the "normobaric oxygen paradox". Eur J Appl Physiol. (2012) 112:4173–5. doi: 10.1007/s00421-012-2392-y
55. Balestra, C, Germonpre, P, Poortmans, JR, and Marroni, A. Serum erythropoietin levels in healthy humans after a short period of normobaric and hyperbaric oxygen breathing: the “normobaric oxygen paradox”. J Appl Physiol. (2006) 100:512–8. doi: 10.1152/japplphysiol.00964.2005
56. de Bels, D, Theunissen, S, Devriendt, J, Germonpré, P, Lafere, P, Valsamis, J, et al. The ‘normobaric oxygen paradox’: does it increase haemoglobin? Diving Hyperb Med. (2012) 42:67–71.
57. Fratantonio, D, Virgili, F, Zucchi, A, Lambrechts, K, Latronico, T, Lafère, P, et al. Increasing oxygen partial pressures induce a distinct transcriptional response in human PBMC: a pilot study on the “normobaric oxygen paradox”. Int J Mol Sci. (2021) 22:458. doi: 10.3390/ijms22010458
58. Hadanny, A, and Efrati, S. The hyperoxic-hypoxic paradox. Biomol Ther. (2020) 10:958. doi: 10.3390/biom10060958
59. Lafere, P, Schubert, T, De Bels, D, Germonpre, P, and Balestra, C. Can the normobaric oxygen paradox (NOP) increase reticulocyte count after traumatic hip surgery? J Clin Anesth. (2013) 25:129–34. doi: 10.1016/j.jclinane.2012.06.021
60. Rocco, M, D'Itri, L, De Bels, D, Corazza, F, and Balestra, C. The “normobaric oxygen paradox”: a new tool for the anesthetist? Minerva Anestesiol. (2014) 80:366–72.
61. Fosen, KM, and Thom, SR. Hyperbaric oxygen, vasculogenic stem cells, and wound healing. Antioxid Redox Signal. (2014) 21:1634–47. doi: 10.1089/ars.2014.5940
62. Thom, SR, Hampton, M, Troiano, MA, Mirza, Z, Malay, DS, Shannon, S, et al. Measurements of CD34+/CD45-dim stem cells predict healing of diabetic neuropathic wounds. Diabetes. (2016) 65:486–97. doi: 10.2337/db15-0517
63. Gallagher, KA, Goldstein, LJ, Thom, SR, and Velazquez, OC. Hyperbaric oxygen and bone marrow-derived endothelial progenitor cells in diabetic wound healing. Vascular. (2006) 14:328–37. doi: 10.2310/6670.2006.00057
64. Marozkina, NV, and Gaston, B. Nitrogen chemistry and lung physiology. Annu Rev Physiol. (2015) 77:431–52. doi: 10.1146/annurev-physiol-021113-170352
65. Weidinger, A, and Kozlov, AV. Biological activities of reactive oxygen and nitrogen species: oxidative stress versus signal transduction. Biomol Ther. (2015) 5:472–84. doi: 10.3390/biom5020472
66. Holbach, KH, Schröder, FK, and Köster, S. Alterations of cerebral metabolism in cases with acute brain injuries during spontaneous respiration of air, oxygen and hyperbaric oxygen. Eur Neurol. (1972) 8:158–60. doi: 10.1159/000114570
Keywords: CD34+, Traumatic Brain Injury, CD133, war veteran self harm, war veterans’ psychological suffering, war veterans suicide, hyperbaric air placebo, HBOT
Citation: MacLaughlin KJ, Barton GP, Braun RK, MacLaughlin JE, Lamers JJ, Marcou MD and Eldridge MW (2023) Hyperbaric air mobilizes stem cells in humans; a new perspective on the hormetic dose curve. Front. Neurol. 14:1192793. doi: 10.3389/fneur.2023.1192793
Edited by:
George Mychaskiw, Ochsner LSU Health, United StatesReviewed by:
Ingrid Eftedal, Norwegian University of Science and Technology, NorwayShai Efrati, Tel Aviv University, Israel
Copyright © 2023 MacLaughlin, Barton, Braun, MacLaughlin, Lamers, Marcou and Eldridge. This is an open-access article distributed under the terms of the Creative Commons Attribution License (CC BY). The use, distribution or reproduction in other forums is permitted, provided the original author(s) and the copyright owner(s) are credited and that the original publication in this journal is cited, in accordance with accepted academic practice. No use, distribution or reproduction is permitted which does not comply with these terms.
*Correspondence: Kent J. MacLaughlin, kmaclaughlin@pediatrics.wisc.edu