- 1Rehabilitation Medicine, The Third Affiliated Hospital of Southern Medical University, Guangzhou, China
- 2Department of Tuina, First Teaching Hospital of Tianjin University of Traditional Chinese Medicine, Tianjin, China
- 3National Clinical Research Center for Chinese Medicine Acupuncture and Moxibustion, Tianjin, China
- 4School of Traditional Chinese Medicine, Southern Medical University, Guangzhou, China
Background: Repetitive transcranial magnetic stimulation, a non-invasive brain stimulation technique, can manage cerebellar ataxia (CA) by suppressing cerebral cortical excitability. Hence, this study aimed to summarize the efficacy and safety of rTMS for CA patients by meta-analysis.
Methods: The PubMed, Embase, Web of Science, and Cochrane Library databases were searched for eligible studies published till 20 May 2023. Weighted mean difference (MD) and 95% confidence intervals (CIs) were used to assess the effect of rTMS treatment. Additionally, the quality of the included studies and the risk of bias were evaluated using the Physiotherapy Evidence Database (PEDro) scale.
Results: Overall, eight studies involving 278 CA patients were included in this meta-analysis. rTMS could significantly improve the Scale for the Assessment and Rating of Ataxia (SARA) (MD: −2.00; 95% CI: −3.97 to −0.02, p = 0.05), International Cooperative Ataxia Rating Scale (ICARS) (MD: −3.96; 95% CI: −5.51 to −2.40, p < 0.00001), Timed Up-and-Go test (TUG) (MD: −1.54; 95% CI: −2.24 to −0.84, p < 0.0001), 10-m walk test (10 MWT) (MD10−m steps: −2.44; 95% CI: −4.14 to −0.73, p = 0.005), and Berg Balance Scale (BBS) (MD: 2.59; 95% CI: 1.15–4.03, p = 0.0004) as compared to sham stimulation. Active rTMS was not significantly different from sham rTMS in changing the duration (MD10−m time: −1.29; 95% CI: −7.98 to 5.41, p = 0.71). No severe adverse events were observed in both sham stimulation and active rTMS groups.
Conclusion: This meta-analysis provides limited evidence that rTMS may be beneficial in treating CA patients. However, these findings should be treated with caution due to the limitations of the smaller sample size and the inconsistent approach and target of rTMS treatment. Therefore, more large-scale RCTs are required to further validate our analytical findings.
Systematic review registration: https://www.crd.york.ac.uk/PROSPERO/display_record.php?RecordID=295726, identifier: CRD42022295726.
1. Introduction
Cerebellar ataxia (CA) is a disease marked by impaired motor function that can have congenital or acquired etiologies (1). The characteristic symptoms include postural imbalance, gait disturbances, limb movement disorders, eyeball movement abnormalities, and speech impairment (2). Gait instability and poor balance contribute to the high incidence of injurious falls in CA patients (3). The prevalence of CA varies based on the etiology. Moreover, the estimated international prevalence of CA ranges between 0.3 and −3 per 100,000 (4, 5). CA may cause severe physical disabilities that impair the patient's daily living capability and burden the family and society (6, 7). The treatment of CA aims to improve the patient's motor-related abilities and quality of life. Targeted etiological treatment is the optimal treatment method for cerebellar disorders. However, the current clinical drug treatment lacks sufficient evidence of effect (8, 9). In this context, new neuromodulation therapies are urgently needed to improve the motor functions of CA patients (10).
Repetitive transcranial magnetic stimulation (rTMS) is an electrophysiological technique with neurostimulating and modulating effects (11). Due to its advantages, including high safety, non-invasiveness, and long-term neuroplasticity (12), rTMS is an alternative to the pharmacological treatment of various neuropsychiatric disorders. Through the magnetic field generated by the energized coil placed on the cranial surface, rTMS acts on cortical nerves to produce induced currents and alter the action potentials, affecting cortical excitability and promoting the neural remodeling of the targeted brain regions (13, 14). Additionally, patients are not expected to actively engage in the rTMS treatment, eliminating concerns about patient compliance and comprehension of instructions (13).
Several meta-analysis studies have demonstrated the positive effects of rTMS in the treatment of neurological diseases, including Parkinson's disease (PD) (15), Alzheimer's disease (AD) (16), epilepsy (17), migraine (18, 19), and multiple sclerosis (20). Additionally, according to several studies over the past two decades, rTMS plays an effective role in improving symptoms and facilitating recovery in CA patients (21). However, constrained by the small sample size (n = 1–20) of individual trials (22), it is challenging to obtain compelling evidence to affirmatively support the positive efficacy of rTMS in treating CA.
Two recent meta-analyses (23, 24) evaluated the positive effects of rTMS in CA patients. Despite these encouraging findings, certain problems were found in their study process. The previous two meta-analyses included studies published in non-peer-reviewed gray literature, such as graduation thesis, which may produce low-quality evidence. In addition, we updated the literature search to include more accumulating randomized controlled trials (RCTs). We performed a meta-analysis of published RCTs on rTMS for CA to obtain more comprehensive conclusions.
2. Methods
2.1. Search strategy
The Preferred Reporting Items for Systematic Reviews and Meta-Analyses statement was used to conduct this meta-analysis (25). The protocol was registered on the International prospective register of systematic reviews (PROSPERO) (CRD 42022295726). The PubMed, Embase, Web of Science, and Cochrane Library databases were searched for studies published in English from the inception to 20 May 2023, using the following keywords as the search terms: “Ataxia,” “Cerebellar ataxia,” “Repetitive transcranial magnetic stimulation,” and “Randomized controlled trial.” The references of included studies were also searched for potential clinical trials. Table 1 represents the search strategy for PubMed. More search strategies are available in Appendix 1.
2.2. Inclusion and exclusion criteria
Inclusion criteria were formulated based on the Population, Intervention, Comparison, Outcomes and Study (PICOS) framework.
(1) Participants: CA patients based on the clinical history and neurological examination, regardless of age, social status, or region.
(2) Intervention: utilizes rTMS interventions (frequency, target location, intensity, and duration are not limited).
(3) Control: control groups should be sham rTMS.
(4) Outcome: changes in values of motor function scales post-therapy and adverse events.
(5) Study: a prospective (randomized) controlled intervention study with pre- and post-testing.
Exclusion criteria are listed as follows:
(1) The participants had other concurrent neurological conditions.
(2) Repeated published study.
(3) Study with insufficient data.
(4) rTMS in combination with other interventions.
(5) Trials with fewer than five treatment sessions, which would not be considered as a treatment course of brain stimulation.
2.3. Data extraction and quality assessment
The studies were examined by two reviewers, who individually retrieved the following information: (1) study characteristics (the first author, publication date, region, and diagnosis); (2) patient characteristics (age and gender); (3) study design (sample size, randomization, allocation, blinding, control, and intervention); (4) rTMS protocol (target position, intensity, frequency, number, and duration of sessions); (5) measures evaluating the motor function, including Scale for the Assessment and Rating of Ataxia (SARA), International Cooperative Ataxia Rating Scale (ICARS), Timed Up-and-Go test (TUG), 10-m walk test (10 MWT), and the Berg Balance Scale (BBS). This study was particularly concerned with the severity of ataxia symptoms using the SARA, which includes eight dimensions of gait, stance, sitting, speech, finger chase, nose-finger test, fast alternating hand movements, and heel-shin slide, with scores ranging between 0 and 40 (26). Higher scores indicate more severe patient dysfunction. SARA has been reported to have good reliability, validity, and responsiveness (27). Additionally, the ICARS scale was utilized to evaluate cerebellar deficits (28); the TUG test was used to assess functional mobility (29); the 10 MWT was used to measure walking ability in CA patients (30); the BBS was used to assess balance during the performance of functional activities (31); and (6) adverse effects. Moreover, two reviewers independently evaluated the risk of bias and study quality using the Physiotherapy Evidence Database (PEDro) scale (http://www.pedro.org.au/english/downloads/pedro-scale). The PEDro scale consists of 11 dichotomous items (either yes or no); the first item (eligibility criteria) is not scored; thus, the total score ranges from 0 to 10. Those scoring ≥6 are considered high-quality studies (32). Discussion with a third reviewer helped resolve the disagreement.
2.4. Statistical analysis
The statistical analyses were performed using the RevMan 5.4 software. Continuous variables (SARA, ICARS, TUG, 10 WMT, and BBS) were expressed by weighted mean difference (MD) together with 95% confidence intervals (CIs). Changes in mean and SDs were calculated using the formulas provided in the Cochrane Handbook (33). If the data were presented in a form other than mean and SDs, such as interquartile range and SEM, the corresponding formula was used for conversion (33–35). The statistically significant differences were set at p < 0.05. Study heterogeneity was assessed using the I2 values based on the Cochrane Handbook. The random-effects model was utilized if heterogeneity was found (I2 ≥ 50%). A further subgroup or sensitivity analysis was conducted to investigate possible causes of the heterogeneity. However, the fixed-effects model was used when the I2 values were <50%.
3. Results
3.1. Study selection
A total of 342 studies were obtained through the initial literature search, and 127 duplicates were removed. After reading the title and abstract, 187 irrelevant studies were excluded. The remaining 28 studies were assessed by full-text reading. A total of 20 studies were further excluded as these were reviews/meta-analyses (n = 9) or outcomes that were not reported using clinical ataxia rating scales (n = 11). Finally, eight studies (36–43) were included in this meta-analysis. The flow diagram of selected studies is summarized in Figure 1.
3.2. Study characteristics
The included eight studies involving 278 CA patients were conducted in China (n = 4) (36, 41–43), Japan (40), South Korea (38), the USA (39), and Brazil (37). These studies were published between 2002 and 2023 and were conducted as double-blind and sham rTMS-controlled parallel-group designs, except for one (37) that adopted the cross-over design. Among the 278 participants, more men (51.80%) were enrolled than women (48.20%). The sample size ranged from 16 to 74, and the treatment duration varied from 5 to 25 days. Moreover, sham stimulation was administered as the control. Motor function was assessed using SARA (n = 5) (37, 39, 41–43), ICARS (n = 4) (36, 37, 42, 43), TUG (n = 2) (37, 39), 10 MWT (n = 2) (38, 40), or BBS (n = 2) (38, 42). The rTMS interventions in the included studies are presented in Table 2.
3.3. Risk of bias
The results of the risk of bias assessment are summarized in Table 3. The PEDro scale scores for the included studies ranged from 6 to 10, with a mean score of 8.13, indicating that the included studies were of high methodological quality. Only one study (40) did not mention the application of a randomized grouping method. Moreover, only one study (37) performed concealed subject allocation.
3.4. Meta-analysis
3.4.1. Scale for the Assessment and Rating of Ataxia
Five trials (37, 39, 41–43) with 154 participants compared active rTMS with sham rTMS by SARA that assessed clinical disease severity. The random-effects model was adopted because of significant heterogeneity (I2 = 96%, p < 0.00001). The result indicated that compared with sham rTMS, active rTMS significantly improved the SARA in CA patients (MD: −2.00; 95% CI: −3.97 to −0.02, p = 0. 05) (Figure 2A). In the sensitivity analysis, removing this outlier study (39) will bring the model toward more statistical significance favoring active rTMS (MD: −2.65; 95% CI: −4.82 to −0.48, p = 0.02) (Figure 2B).
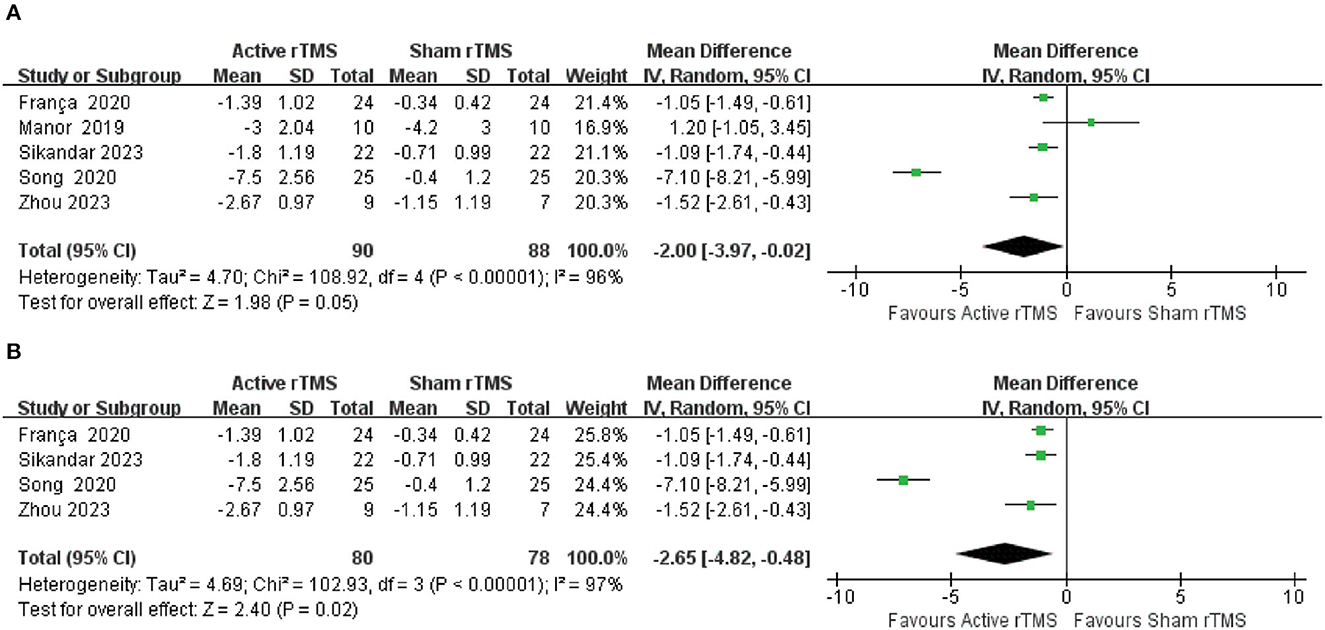
Figure 2. Forest plot and meta-analysis of Scale for the Assessment and Rating of Ataxia (SARA). (A) SARA. (B) Sensitivity analysis of SARA.
3.4.2. International Cooperative Ataxia Rating Scale
Four trials (36, 37, 42, 43) with 102 participants compared active rTMS with sham rTMS by ICARS that assessed cerebellar dysfunction. The random-effects model was adopted because of moderate heterogeneity (I2 = 68%, p = 0.02). The result indicated that compared with sham rTMS, active rTMS significantly improved the ICARS in CA patients (MD: −3.96; 95% CI: −5.51 to −2.40, p < 0.00001) (Figure 3).
3.4.3. Timed Up-and-Go test
Two trials (37, 39) with 44 participants compared active rTMS with sham rTMS by TUG that assessed functional mobility. The fixed-effects model was adopted because of no statistically significant heterogeneity (I2 = 0%, p = 0.56). The result indicated that compared with sham rTMS, active rTMS significantly improved the TUG in CA patients (MD: −1.54; 95% CI: −2.24 to −0.84, p < 0.0001) (Figure 4).
3.4.4. 10-m walk test
Two trials (38, 40) with 106 participants compared active rTMS with sham rTMS by 10 MWT, which measured walking ability. The result indicated that compared with sham rTMS, active rTMS showed significant changes in the CA patients in terms of the number of steps in 10 MWT (MD10−m steps: −2.44; 95% CI: −4.14 to −0.73, p = 0.005) (Figure 5B). However, active rTMS was not significantly different from sham rTMS in changing the duration (MD10−m time: −1.29; 95% CI: −7.98 to 5.41, p = 0.71) (Figure 5A).
3.4.5. The Berg Balance Scale
Two trials (38, 42) with 76 participants compared active rTMS with sham rTMS by BBS that evaluated balance function. The fixed-effects model was adopted because of slight heterogeneity (I2 = 40%, p = 0.20). The result indicated that compared with sham rTMS, active rTMS significantly improved the BBS in CA patients (MD: 2.59; 95% CI: 1.15–4.03, p = 0.0004) (Figure 6).
3.4.6. Subgroup analysis
According to different frequencies, five studies were included for subgroup analysis. The low-frequency subgroup included (0.17 and 1 Hz), while the high-frequency subgroup included (10 and 50 Hz as iTBS). The results showed that compared with sham rTMS, low-frequency rTMS had a statistically significant improvement in SARA, with slight heterogeneity (MD: −0.92; 95% CI: −1.53 to −0.31, p = 0.003; I2 = 47%). In contrast, there was no difference between the high-frequency of rTMS and sham rTMS groups, with high heterogeneity (MD: −4.31, 95% CI −9.78 to 1.16, p = 0.12; I2 = 98%) (Figure 7).
3.4.7. Safety of rTMS
The fixed-effects model was used as there was no significant heterogeneity (I2 = 0%, p = 0.87) between the included studies. Two studies reported adverse events during or after treatment. There was no significant difference in the incidence of adverse events between the two groups [odds ratio (OR): 1.24; 95% CI: 0.34–4.54, p = 0.74] (Figure 8). Six of the included studies had no adverse events during or after rTMS treatment. A study by Franca et al. (37) reported that five patients had slight side effects during or after treatment (one felt discomfort during treatment, three had a mild headache during or after treatment, and one underwent transient worsening of the left leg pain). The study by Sikandar et al. (42) reported that nausea occurred in one patient in the rTMS group. However, all side effects resolved spontaneously after the treatment without further interventions.
4. Discussion
In contrast to the previous two meta-analyses (23, 24), this study included only RCTs published in peer-reviewed journals, which can yield high-quality evidence. Our study updated the literature search to include only eight articles published in English. In the previous meta-analyses, the outcome measures for motor function included SARA, ICARS, and BBS. While the current meta-analysis included further motor outcome measures, including TUG for functional mobility, 10 MWT10−m time, and 10 MWT10−m steps for walking ability. In addition, previous meta-analyses excluded special rTMS protocols, such as theta-burst stimulation (TBS) while this meta-analysis included all rTMS protocols including iTBS (41). Our findings are consistent with previous meta-analyses (23, 24) conclusions that rTMS had positively affected patients. In this study, we performed a meta-analysis of eight studies involving 278 CA patients. It proved the positive effect of active rTMS on the motor functions of CA patients. According to the study, active rTMS showed advantages in improving the clinical disease severity (SARA), cerebellar dysfunction (ICARS), functional mobility (TUG), walking ability (10 WMT10−m steps), and balance function (BBS) of CA patients. However, it had no evident effect on the 10 WMT10−m time of CA patients. Furthermore, our study proved that rTMS was safe and patients would not develop any severe adverse events other than mild pain and nausea. To summarize, rTMS has revealed inspiring potential in the clinical treatment of CA patients.
Although this meta-analysis has shown that active rTMS have beneficial effects on CA, the mechanism of rTMS has not been fully understood. The cerebellum is functionally complicated with direct or indirect relations to almost the entire central nervous system. Moreover, the dysfunction of the cerebellum and its connected neural network is considered the proximate cause of dyskinesia in CA patients (44–46). In this context, the treatments that aim to control and improve cerebellar dysfunction may have a significant clinical impact. The positive effect of rTMS in treating CA patients may be due to the action on their cerebellum, which causes lasting changes in cerebellar–thalamo–cortical pathway excitability and increases the blood flow in the cerebellar hemisphere or suppresses oxidative stress (47). The increased cerebral blood flow can activate the cerebellar functions, which have been diminished. This is proved by the investigations of Shimizu et al. (48) and Shiga (40), in which patients presented increased blood flow in the cerebellum and pontine accompanied by increased exercise volume and improved gait ataxia after rTMS treatment. In the study by Ihara et al. (47), 20 patients with spinocerebellar degeneration (SCD) received rTMS at 0.2 Hz 3 days a week for 8 weeks. The severity of oxidative stress in the central nervous system was assessed by detecting the concentration of ascorbate free radicals (AFR). According to Ihara et al., AFR levels in SCD patients decreased considerably after receiving rTMS treatment compared to healthy controls. Moreover, the decline rate was positively associated with the pretreatment AFR levels. The cerebellar facilitation effect rarely influences the motor system among patients with CA. rTMS can inhibit the excitability of the cortical motor area by acting on the cerebellar cortex and activating Purkinje cells or supplementing the insufficient inhibitory effect of the cerebellar nucleus due to the impaired or absent functions of Purkinje cells. This results in the transient facilitation of inhibitory neurons (49). Similarly, symptoms, such as gait, can be improved and motor functions can be regulated. rTMS can generate long-lasting effects in treating various complicated neurological diseases such as Parkinson's (50), Alzheimer's (51), and ataxia (52). Therefore, more research is required to determine the precise mechanism of action of rTMS for CA.
However, there are certain limitations in the current meta-analysis. First, due to strict inclusion criteria, only eight studies published in English in peer-reviewed journals were included, which inevitably led to the issue of publication bias. Second, heterogeneity is inevitable due to different stimulus locations, intervention duration, stimulus intensity, and pulse number, and this inconsistency may affect the results' validity and the study's reproducibility. Therefore, there is a need for a stimulation protocol based on evidence-based rTMS. Third, given the lack of subgroup analysis based on the ataxia subtype, gender, and age, the efficacy of rTMS should be drawn with careful deliberation. Fourth, multiple outcome measures evaluated the motor functions in CA patients in the included studies; however, only a few studies were selected for pooled analyses. Furthermore, studies have confirmed that the cerebellum plays a critical role in many cognitive and affective functions, resulting in cognitive and social deficits among CA that may significantly impact their quality of life, which were not mentioned in any of the included studies. Developing a core outcome set (COS) (53) in clinical trials concerning CA is necessary. Finally, it is challenging to assess the long-term effects of rTMS in CA patients due to limited studies that provided follow-up results.
5. Conclusion
The meta-analysis preliminarily indicates that rTMS has a positive effect on alleviating the symptoms of CA patients. However, these findings should be treated with caution due to the limited number of research articles, the small number of case studies in the included articles, the short duration of treatment, and the inconsistent approach and target of rTMS treatment. Further large-scale studies are needed to explore the optimal stimulus parameters.
Data availability statement
The original contributions presented in the study are included in the article/Supplementary material, further inquiries can be directed to the corresponding author.
Author contributions
LY, XW, and JC: conceptualization. LY, XW, and DL: methodology, software, and writing—original draft preparation. HL and ZL: study selection and data extraction. LY and YH: quality assessment. All authors have read and agreed to the published version of the manuscript.
Funding
This study was financially supported by the National Science Foundation of China (No. 81403455).
Conflict of interest
The authors declare that the research was conducted in the absence of any commercial or financial relationships that could be construed as a potential conflict of interest.
Publisher's note
All claims expressed in this article are solely those of the authors and do not necessarily represent those of their affiliated organizations, or those of the publisher, the editors and the reviewers. Any product that may be evaluated in this article, or claim that may be made by its manufacturer, is not guaranteed or endorsed by the publisher.
Supplementary material
The Supplementary Material for this article can be found online at: https://www.frontiersin.org/articles/10.3389/fneur.2023.1177746/full#supplementary-material
References
1. Bonni S, Ponzo V, Caltagirone C, Koch G. Cerebellar theta burst stimulation in stroke patients with ataxia. Funct Neurol. (2014) 29:41–5. doi: 10.11138/FNeur/2014.29.1.041
2. Klockgether T. Sporadic ataxia with adult onset: classification and diagnostic criteria. Lancet Neurol. (2010) 9:94–104. doi: 10.1016/S1474-4422(09)70305-9
3. Serrao M, Pierelli F, Ranavolo A, Draicchio F, Conte C, Don R, et al. Gait pattern in inherited cerebellar ataxias. Cerebellum. (2012) 11:194–211. doi: 10.1007/s12311-011-0296-8
4. Ruano L, Melo C, Silva MC, Coutinho P. The global epidemiology of hereditary ataxia and spastic paraplegia: a systematic review of prevalence studies. Neuroepidemiology. (2014) 42:174–83. doi: 10.1159/000358801
5. Marsden J, Harris C. Cerebellar ataxia: pathophysiology and rehabilitation. Clin Rehabil. (2011) 25:195–216. doi: 10.1177/0269215510382495
6. Marsden JF. Cerebellar ataxia. Handb Clin Neurol. (2018) 159:261–81. doi: 10.1016/B978-0-444-63916-5.00017-3
7. Pandolfo M, Manto M. Cerebellar and afferent ataxias. Continuum. (2013) 19:1312–43. doi: 10.1212/01.CON.0000436158.39285.22
8. Gandini J, Manto M, Bremova-Ertl T, Feil K, Strupp M. The neurological update: therapies for cerebellar ataxias in 2020. J Neurol. (2020) 267:1211–20. doi: 10.1007/s00415-020-09717-3
9. Wei G, Zhong D, Zhang W, Luo J. Comprehensive systematic review summary: treatment of cerebellar motor dysfunction and ataxia: report of the guideline development, dissemination, and implementation subcommittee of the American academy of neurology. Neurology. (2018) 90:464–71. doi: 10.1212/WNL.0000000000005055
10. Franca C, de Andrade DC, Teixeira MJ, Galhardoni R, Silva V, Barbosa ER, et al. Effects of cerebellar neuromodulation in movement disorders: a systematic review. Brain Stimul. (2018) 11:249–60. doi: 10.1016/j.brs.2017.11.015
11. Hoogendam JM, Ramakers G, Lazzaro VD. Physiology of repetitive transcranial magnetic stimulation of the human brain. Brain Stimul. (2010) 3:95–118. doi: 10.1016/j.brs.2009.10.005
12. Ikeda T, Kurosawa M, Morimoto C, Kitayama S, Nukina N. Multiple effects of repetitive transcranial magnetic stimulation on neuropsychiatric disorders. Biochem Biophys Res Commun. (2013) 436:121–7. doi: 10.1016/j.bbrc.2013.03.017
13. Robertson C, Mortimer A. Quantitative EEG (qEEG) guided transcranial magnetic stimulation (TMS) treatment for depression and anxiety disorders: an open, observational cohort study of 210 patients. J Affect Disord. (2022) 308:322–7. doi: 10.1016/j.jad.2022.04.076
14. Eldaief MC, Press DZ, Pascual-Leone A. Transcranial magnetic stimulation in neurology: a review of established and prospective applications. Neurol Clin Pract. (2013) 3:519–26. doi: 10.1212/01.CPJ.0000436213.11132.8e
15. Chou Y-H, Hickey PT, Sundman M, Song AW, Chen N-K. Effects of repetitive transcranial magnetic stimulation on motor symptoms in Parkinson disease: a systematic review and meta-analysis. JAMA Neurol. (2015) 72:432–40. doi: 10.1001/jamaneurol.2014.4380
16. Dong X, Yan L, Huang L, Guan X, Dong C, Tao H, et al. Repetitive transcranial magnetic stimulation for the treatment of Alzheimer's disease: a systematic review and meta-analysis of randomized controlled trials. PLoS One. (2018) 13:e0205704. doi: 10.1371/journal.pone.0205704
17. Chen R, Spencer DC, Weston J, Nolan SJ. Transcranial magnetic stimulation for the treatment of epilepsy. Cochr Database Syst Rev. (2016) 11:CD011025. doi: 10.1002/14651858.CD011025.pub2
18. Saltychev M, Juhola J. Effectiveness of high-frequency repetitive transcranial magnetic stimulation in migraine: a systematic review and meta-analysis. Am J Phys Med Rehabil. (2022) 101:1001–6. doi: 10.1097/PHM.0000000000001953
19. Mohamad Safiai NI, Mohamad NA, Basri H, Inche Mat LN, Hoo FK, Abdul Rashid AM, et al. High-frequency repetitive transcranial magnetic stimulation at dorsolateral prefrontal cortex for migraine prevention: a systematic review and meta-analysis. Cephalalgia. (2022) 42:1071–85. doi: 10.1177/03331024221092423
20. Chen X, Yin L, An Y, Yan H, Zhang T, Lu X, et al. Effects of repetitive transcranial magnetic stimulation in multiple sclerosis: a systematic review and meta-analysis. Mult Scler Relat Disord. (2022) 59:103564. doi: 10.1016/j.msard.2022.103564
21. Cha HG. The effect of low-frequency (1 hz) rtms on the cerebellar cortex in patients with ataxia after a posterior circulation stroke: randomized control trial. Journal of Magnetics. (2017) 22:625–9. doi: 10.4283/JMAG.2017.22.4.625
22. Cury RG, Teixeira MJ, Galhardoni R, Barboza VR, Alho E, Seixas CM, et al. Neuronavigation-guided transcranial magnetic stimulation of the dentate nucleus improves cerebellar ataxia: a sham-controlled, double-blind n = 1 study. Parkinson Relat Disord. (2015) 21:999–1001. doi: 10.1016/j.parkreldis.2015.05.010
23. Qiu YT, Chen Y, Tan HX, Su W, Guo QF, Gao Q. Efficacy and safety of repetitive transcranial magnetic stimulation in cerebellar ataxia: a systematic review and meta-analysis. Cerebellum. (2023) 1–12. doi: 10.1007/s12311-022-01508-y
24. Wang Y, Zhang D, Wang J, Ma J, Lu L, Jin S. Effects of transcranial magnetic stimulation on cerebellar ataxia: a systematic review and meta-analysis. Front Neurol. (2023) 14:1049813. doi: 10.3389/fneur.2023.1049813
25. Moher D, Liberati A, Tetzlaff J, Altman DG, PRISMA Group* T. Preferred reporting items for systematic reviews and meta-analyses: the PRISMA statement. BMJ. (2009) 339:b2535. doi: 10.1136/bmj.b2535
26. Schmitz-Hübsch T, Montcel STD, Baliko L, Berciano J, Fancellu R. Scale for the assessment and rating of ataxia: development of a new clinical scale. Neurology. (2006) 66:1717–20. doi: 10.1212/01.wnl.0000219042.60538.92
27. Weyer A, Abele M, Schmitz-Hübsch T, Schoch B, Frings M, Timmann D, et al. Reliability and validity of the scale for the assessment and rating of ataxia: a study in 64 ataxia patients. Mov Disord. (2010) 22:1633–7. doi: 10.1002/mds.21544
28. Trouillas P, Takayanagi T, Hallett M, Currier RD, Subramony SH, Wessel K, et al. International Cooperative Ataxia Rating Scale for pharmacological assessment of the cerebellar syndrome. J Neurol Sci. (1997) 145:205–11. doi: 10.1016/S0022-510X(96)00231-6
29. Podsiadlo D, Richardson S. The timed “Up & Go”: a test of basic functional mobility for frail elderly persons. J Am Geriatr Soc. (1991) 39:142–8. doi: 10.1111/j.1532-5415.1991.tb01616.x
30. Van Bloemendaal M, Van DW, Alexander TM, Van DP, Ingrid GL. Walking tests for stroke survivors: a systematic review of their measurement properties. Disabil Rehabil. (2012) 34:2207–21. doi: 10.3109/09638288.2012.680649
31. Meseguer-Henarejos AB, Rubio-Aparicio M, López-Pina JA, Carles-Hernández R, Gómez-Conesa A. Characteristics that affect score reliability in the Berg Balance Scale: a meta-analytic reliability generalization study. Eur J Phys Rehabil Med. (2019) 55:570–84. doi: 10.23736/S1973-9087.19.05363-2
32. Maher CG, Catherine S, Herbert RD, Moseley AM, Mark E. Reliability of the PEDro scale for rating quality of randomized controlled trials. Phys Ther. (2003) 83:713–21. doi: 10.1093/ptj/83.8.713
33. Higgins JPT, Li T, Deeks JJ. Chapter 6: choosing effect measures and computing estimates of effect. In:Higgins JPT, Thomas J, Chandler J, Cumpston M, Li T, Page MJ, Welch VA, , editors. Cochrane Handbook for Systematic Reviews of Interventions Version 6.3 (updated February 2022) (Cochrane, AB) (2022). Available online at: https://training.cochrane.org/handbook/current/chapter-06#section-6-5-2-8
34. Luo D, Wan X, Liu J, Tong T. Optimally estimating the sample mean from the sample size, median, mid-range and/or mid-quartile range. Stat Methods Med Res. (2015) 27:1785–805. doi: 10.1177/0962280216669183
35. Wan X, Wang W, Liu J, Tong T. Estimating the sample mean and standard deviation from the sample size, median, range and/or interquartile range. BMC Med Res Methodol. (2014) 14:1–13. doi: 10.1186/1471-2288-14-135
36. Chen X-Y, Lian Y-H, Liu X-H, Sikandar A, Li M-C, Xu H-L, et al. Effects of repetitive transcranial magnetic stimulation on cerebellar metabolism in patients with spinocerebellar ataxia type 3. Front Aging Neurosci. (2022) 14:827993. doi: 10.3389/fnagi.2022.827993
37. Franca C, Andrade DD, Silva V, Galhardoni R, Barbosa ER, Teixeira MJ, et al. Effects of cerebellar transcranial magnetic stimulation on ataxias: a randomized trial. Parkinson Relat Disord. (2020) 80:1–6. doi: 10.1016/j.parkreldis.2020.09.001
38. Kim W, Jung S, Oh M, Min Y, Lim J, Paik N. Effect of repetitive transcranial magnetic stimulation over the cerebellum on patients with ataxia after posterior circulation stroke: a pilot study. J Rehabil Med. (2014) 46:418–23. doi: 10.2340/16501977-1802
39. Manor B, Greenstein PE, Davila-Perez P, Wakefield S, Zhou J, Pascual-Leone A. Repetitive transcranial magnetic stimulation in spinocerebellar ataxia: a pilot randomized controlled trial. Front Neurol. (2019) 10:73. doi: 10.3389/fneur.2019.00073
40. Shiga Y. Transcranial magnetic stimulation alleviates truncal ataxia in spinocerebellar degeneration. J Neurol Neurosurg Psychiatry. (2002) 72:124–6. doi: 10.1136/jnnp.72.1.124
41. Song P, Li S, Wang S, Wei H, Lin H, Wang Y. Repetitive transcranial magnetic stimulation of the cerebellum improves ataxia and cerebello-fronto plasticity in multiple system atrophy: a randomized, double-blind, sham-controlled and TMS-EEG study. Aging. (2020) 12:20611–22. doi: 10.18632/aging.103946
42. Sikandar A, Liu X-H, Xu H-L, Li Y, Lin Y-Q, Chen X-Y, et al. Short-term efficacy of repetitive transcranial magnetic stimulation in SCA3: a prospective, randomized, double-blind, sham-controlled study. Parkinson Relat Disord. (2023) 106:105236. doi: 10.1016/j.parkreldis.2022.105236
43. Zhou M, Qiu M, Jin Y, Li D, Tao C, Lou D, et al. effectiveness of high-frequency repetitive transcranial magnetic stimulation in patients with spinocerebellar ataxia type 3. J ECT. (2023). doi: 10.1097/YCT.0000000000000925. [Epub ahead of print].
44. Diener HC, Dichgans J. Pathophysiology of cerebellar ataxia. Mov Disord. (1992) 7:95–109. doi: 10.1002/mds.870070202
45. Koch G. Repetitive transcranial magnetic stimulation: a tool for human cerebellar plasticity. Funct Neurol. (2010) 25:159–63.
46. Restivo DA, Lanza S, Saponara R, Rapisarda G, Giuffrida S, Palmeri A. Changes of cortical excitability of human motor cortex in spinocerebellar ataxia type 2. A study with paired transcranial magnetic stimulation. J Neurol Sci. (2002) 198:87–92. doi: 10.1016/S0022-510X(02)00086-2
47. Ihara Y, Nobukuni K, Takata H, Sakai K, Takahashi K. Influence of repetitive transcranial magnetic stimulation on ataxia severity and biochemical parameters in cerebrospinal fluid of patients with spinocerebellar degeneration. Neurol Res. (2005) 27:310–3. doi: 10.1179/016164105X39897
48. Shimizu H, Tsuda T, Shiga Y, Miyazawa K, Onodera Y, Matsuzaki M, et al. Therapeutic efficacy of transcranial magnetic stimulation for hereditary spinocerebellar degeneration. Tohoku J Exp Med. (1999) 189:203–11. doi: 10.1620/tjem.189.203
49. Farzan F, Wu Y, Manor B, Anastasio EM, Lough M. Cerebellar TMS in treatment of a patient with cerebellar ataxia: evidence from clinical, biomechanics and neurophysiological assessments. Cerebellum. (2013) 12:707–12. doi: 10.1007/s12311-013-0485-8
50. Zhang W, Deng B, Xie F, Zhou H, Guo J-F, Jiang H, et al. Efficacy of repetitive transcranial magnetic stimulation in Parkinson's disease: a systematic review and meta-analysis of randomised controlled trials. EClinicalMedicine. (2022) 52:101589. doi: 10.1016/j.eclinm.2022.101589
51. Lin Y, Jiang WJ, Shan PY, Lu M, Wang T, Li RH, et al. The role of repetitive transcranial magnetic stimulation (rTMS) in the treatment of cognitive impairment in patients with Alzheimer's disease: a systematic review and meta-analysis. J Neurol Sci. (2019) 398:184–91. doi: 10.1016/j.jns.2019.01.038
52. Di Nuzzo C, Ruggiero F, Cortese F, Cova I, Priori A, Ferrucci R. Non-invasive cerebellar stimulation in cerebellar disorders. CNS Neurol Disord Drug Targets. (2018) 17:193–8. doi: 10.2174/1871527317666180404113444
Keywords: cerebellar ataxia, repetitive transcranial magnetic stimulation, motor functions, meta-analysis, systematic review
Citation: Yin L, Wang X, Chen L, Liu D, Li H, Liu Z, Huang Y and Chen J (2023) Repetitive transcranial magnetic stimulation for cerebellar ataxia: a systematic review and meta-analysis. Front. Neurol. 14:1177746. doi: 10.3389/fneur.2023.1177746
Received: 02 March 2023; Accepted: 19 June 2023;
Published: 07 July 2023.
Edited by:
Tomofumi Yamaguchi, Juntendo University, JapanReviewed by:
Honglin Hao, Peking Union Medical College Hospital (CAMS), ChinaAbdulhameed Tomeh, Universiti Putra Malaysia, Malaysia
Copyright © 2023 Yin, Wang, Chen, Liu, Li, Liu, Huang and Chen. This is an open-access article distributed under the terms of the Creative Commons Attribution License (CC BY). The use, distribution or reproduction in other forums is permitted, provided the original author(s) and the copyright owner(s) are credited and that the original publication in this journal is cited, in accordance with accepted academic practice. No use, distribution or reproduction is permitted which does not comply with these terms.
*Correspondence: Junqi Chen, meixibao@126.com
†ORCID: Junqi Chen orcid.org/0000-0001-6847-0891