- 1Research and Early Development, REGENXBIO Inc., Rockville, MD, United States
- 2Brain Development and Disorders Lab, Istituto Italiano di Tecnologia, Genoa, Italy
- 3Research Department, The Children's Memorial Health Institute (IPCZD), Warsaw, Poland
Editorial on the Research Topic
Viral vector-based gene therapy in neurological disease: The future is now
In this special issue of Frontiers in Neurology, we have focused on highlighting the advances being made using viral vectors as a medium for gene therapies to address diseases of the central nervous system (CNS). This emerging area of medicine, particularly in the domain of adeno-associated virus (AAV) vectors, has seen a dramatic increase in research, clinical trials, and more recently approved therapeutics over the last decade. However, this technology is still nascent, with more research necessary to fully understand both its potential and its pitfalls. This is particularly true for its use in the CNS, where AAVs have the potential to be truly revolutionary medicines for diseases with significant unmet medical needs that have not been addressable via earlier medical technology. The use of AAVs can be achieved via numerous routes of administration in order to target focused regions of the brain or broad expression throughout the CNS to produce a diverse set of transgene payloads, including proteins for gene replacement, micro-RNAs for gene knockdown, or antibody expression, as reviewed by Marino and Holt in this special issue. Across the remaining articles in this issue, two major themes emerge: a focus on promoters in controlling expression to occur selectively in particular cell types within the CNS; and insights into ongoing clinical uses of AAVs in spinal muscular atrophy.
Promoters for cell-type-specific expression
A significant advantage of AAV approaches to gene therapy is the ability to restrict transgene expression to specific cellular subtypes of interest. A wealth of recent papers has revealed novel promoter/enhancer sequences that can be used to target subtypes of neurons in the CNS, with a particular focus on GABAergic neurons (1–4). As discussed in the review by Duba-Kiss et al. in this special issue, this focus on GABAergic subtypes is largely motivated by a plethora of evidence linking these cells to various neurological and neuropsychiatric disorders. Importantly, the authors also point out that the effectiveness of these promoters can vary widely across brain regions, across ages (neonatal vs. adult), and across species (see their Table 1 for a concise summary of these factors across recent studies).
Also in this issue, Finneran et al. deftly demonstrate that following intravenous administration of PHP.eB, a vector that crosses the blood–brain barrier, the vector achieves widespread CNS transduction. Importantly, the use of neuronal-specific promoters (hSyn1 and CaMKIIa) largely blocked expression in peripheral organs (the liver, heart, etc.), as the authors observed when employing CAG, a ubiquitous promoter (see Figure 1A). Hollidge et al. also compared these same promoters (CAG, hSyn, and CaMKIIa) following direct intraparenchymal delivery to the striatum; they confirmed that hSyn and CaMKIIa restrict expression to neurons only, whereas CAG elicits expression in neurons, astrocytes, and oligodendrocytes (Figure 1B). However, an important result from Hollidge et al. is that these different promoters also exhibited very different expression profiles over a 6-month period (Figure 1C). Interestingly, RNA and protein expression were found to diverge at later time points: specifically, a time point was identified at which RNA was still increasing, but protein expression levels decreased. These findings raise the need for further research to characterize the mechanisms affecting AAV transcription and translation over time and warrant consideration in the implementation of long-term studies. Finally, these authors also demonstrate that vector DNA undergoes continuous processing over the first 3 months after injection (Figure 1D): in particular, over the first 3 weeks after injection, the total amount of vector DNA diminishes as the remaining vector DNA assembles into stable circular episomes that concatenate with up to 5 copies per circular episome.
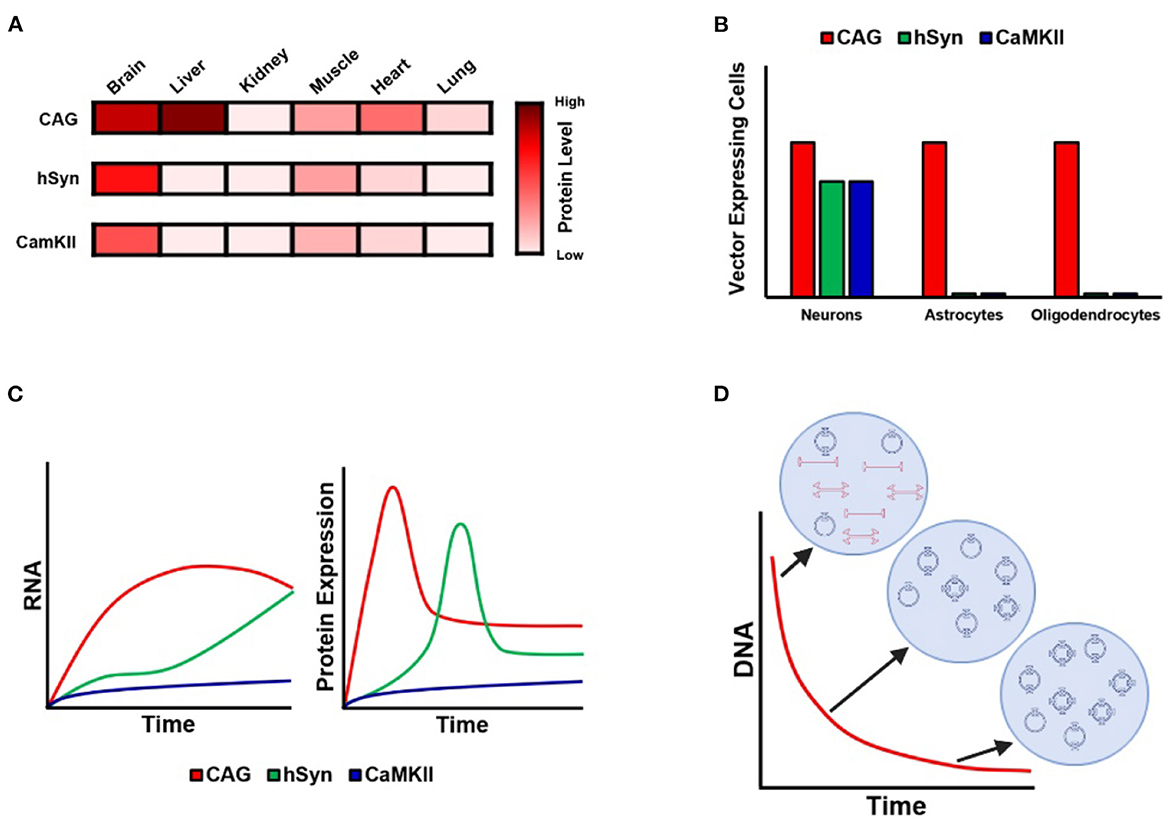
Figure 1. Kinetics of AAV expression with ubiquitous and neuron-specific promoters. (A) Illustration of GFP protein expression in different organs following administration of PHP.eB employing CAG, hSyn, and CamKII promoters. Note that CAG evinces strong expression in the liver and heart, whereas hSyn and CamKII are associated with almost no expression outside the brain, with the exception of weak expression in skeletal muscle. Data from Finneran et al.. (B) Cell-type-specific expression of GFP under CAG, hSyn, and CamKII following AAV9 intraparenchymal injection into the striatum. Note that CAG is associated with expression in neurons, astrocytes, and oligodendrocytes, whereas expression is restricted to neurons in the case of hSyn and CamKII. Data from Hollidge et al.. (C) Diagram of the 6-month time-course of RNA and protein expression under CAG, hSyn, and CamKII following AAV9 intraparenchymal injection into the striatum. Note the different kinetic profile of expression for each promoter. Data from Hollidge et al.. (D) Diagram of the 3-week time-course of vector DNA following AAV9 intraparenchymal injection into the striatum. Note that the total amount of vector DNA decreases continuously until stabilizing at 3 weeks, during which time the vector DNA forms stable circular episomes and concatemers of up to five vector copies per episome. Data from Hollidge et al..
Updates on clinical uses of AAV in the CNS
Beyond basic research into AAV biology and transgene cassette technology, this issue includes two updates on the challenges of AAV therapeutics from a clinical perspective. In Kotulska, Fattal-Valevski, et al. the authors review a currently available AAV therapeutic approved for clinical practice in patients with spinal muscular atrophy (SMA). Until now, most patients with acute forms of SMA have had a fatal prognosis before the end of the second year of life, due to general muscle hypotonia resulting in respiratory insufficiency. A major recent advance has occurred in the form of the introduction of SMN1 gene therapy, specifically via onasemnogene abeparvovec, which employs a self-complementary adeno-associated virus 9 (scAAV9) vector to deliver a healthy copy of the SMN1 gene (5). Phase 1 and phase 3 clinical trials have shown that a single administration of onasemnogene abeparvovec results in improvement of motor functions in the majority of infants with SMA. Kotulska, Fattal-Valevski, et al. review currently ongoing phase 3 clinical trials in SMA1 and SMA2 patients, as well as pre-symptomatic infants.
In the other article by Kotulska, Jozwiak, et al., the authors identify an important issue regarding gene therapy in neonates with SMA. Recently introduced in some countries, newborn screening programs allow very early use of gene therapy. However, the necessity of steroid regimens is problematic with the administration of neonatal live vaccines, especially the tuberculosis vaccine. The timing of gene therapy in such patients has not yet been addressed in the existing international guidelines. In the article, the authors present the first recommendations from the Polish Vaccinology Association for gene therapy administration in newborns who have received live vaccination against tuberculosis, which is currently used in clinical practice in Poland.
Author contributions
All authors listed have made a substantial, direct, and intellectual contribution to the work and approved it for publication.
Funding
This work was funded by European Research Council (ERC) under the European Union's Horizon 2020 Research and Innovation Program (Grant Agreement No. 725563 to LC).
Acknowledgments
The authors would like to thank Haley Janowitz for making the figure, which was created using BioRender.com.
Conflict of interest
JS and AM are paid employees of REGENXBIO Inc, a for-profit AAV gene therapy company, REGENXBIO receives licensing royalties from the sale of Zolgensma to treat spinal muscular atrophy (SMA). LC has received research funding from REGENXBIO. SJ is a paid consultant of REGENXBIO.
Publisher's note
All claims expressed in this article are solely those of the authors and do not necessarily represent those of their affiliated organizations, or those of the publisher, the editors and the reviewers. Any product that may be evaluated in this article, or claim that may be made by its manufacturer, is not guaranteed or endorsed by the publisher.
References
1. Dimidschstein J, Chen Q, Tremblay R, Rogers S, Saldi G, Guo L, et al. A viral strategy for targeting and manipulating interneurons across vertebrate species. Nat Neurosci. (2016) 19:1743–9. doi: 10.1038/nn.4430
2. Mehta P, Kreeger L, Wylie DC, Pattadkal JJ, Lusignan T, Davis MJ, et al. Functional access to neuron subclasses in rodent and primate forebrain. Cell Rep. (2019) 26:2818–32.e8. doi: 10.1016/j.celrep.2019.02.011
3. Vormstein-Schneider D, Lin JD, Pelkey KA, Chittajallu R, Guo B, Arias-Garcia MA, et al. Viral manipulation of functionally distinct interneurons in mice, non-human primates and humans. Nat Neurosci. (2020) 23:1629–36. doi: 10.1038/s41593-020-0692-9
4. Mich JK, Graybuck LT, Hess EE, Mahoney JT, Kojima Y, Ding Y, et al. Functional enhancer elements drive subclass-selective expression from mouse to primate neocortex. Cell Rep. (2021) 34:108754. doi: 10.1016/j.celrep.2021.108754
Keywords: adeno-associated virus (AAV), gene therapy, promoter, spinal muscular atrophy (SMA), viral vector, antibody, gene replacement
Citation: Smith JB, Cancedda L, Jozwiak S and Mercer AC (2023) Editorial: Viral vector-based gene therapy in neurological disease: The future is now. Front. Neurol. 14:1153681. doi: 10.3389/fneur.2023.1153681
Received: 29 January 2023; Accepted: 31 January 2023;
Published: 14 February 2023.
Edited and reviewed by: Leonard Verhagen Metman, Rush University, United States
Copyright © 2023 Smith, Cancedda, Jozwiak and Mercer. This is an open-access article distributed under the terms of the Creative Commons Attribution License (CC BY). The use, distribution or reproduction in other forums is permitted, provided the original author(s) and the copyright owner(s) are credited and that the original publication in this journal is cited, in accordance with accepted academic practice. No use, distribution or reproduction is permitted which does not comply with these terms.
*Correspondence: Jared B. Smith, anNtaXRoMDFAcmVnZW54YmlvLmNvbQ==