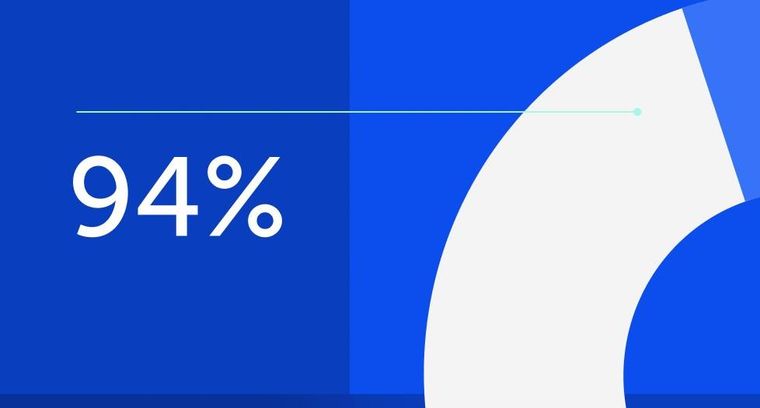
94% of researchers rate our articles as excellent or good
Learn more about the work of our research integrity team to safeguard the quality of each article we publish.
Find out more
SYSTEMATIC REVIEW article
Front. Neurol., 21 June 2023
Sec. Neurorehabilitation
Volume 14 - 2023 | https://doi.org/10.3389/fneur.2023.1146164
This article is part of the Research TopicReviews in NeurorehabilitationView all 17 articles
Background: Randomized controlled trials (RCTs) have shown conflicting results regarding the effects of perioperative cognitive training (CT) on the incidence of postoperative cognitive dysfunction (POCD) and postoperative delirium (POD). We, therefore, performed a meta-analysis to assess the overall effects of studies on this topic.
Methods: We searched PubMed, Embase, the Cochrane Library, and Web of Science for all RCTs and cohort studies that investigated the effects of perioperative CT on the incidence of POCD and POD. Data extraction and quality assessment were conducted independently by two researchers.
Results: This study included nine clinical trials with a total of 975 patients. The results showed that perioperative CT significantly reduced the incidence of POCD compared with the control group [risk ratio (RR) = 0.5, 95% CI (confidence interval): 0.28–0.89, P = 0.02]. Nevertheless, for the incidence of POD, the difference between the two groups was not statistically significant (RR = 0.64; 95% CI: 0.29–1.43, P = 0.28). In addition, the CT group had less postoperative decline in the cognitive function scores compared with the control group [mean differences (MD): 1.58, 95% CI: 0.57–2.59, P = 0.002]. In addition, there were no statistically differences in length of hospital stay between the two groups (MD: −0.18, 95% CI: −0.93–0.57, P = 0.64). Regarding CT adherence, the proportion of patients in the cognitive training group who completed the planned duration of CT was 10% (95% CI: 0.05–0.14, P = 0.258).
Conclusion: Our meta-analysis revealed that perioperative cognitive training is possibly an effective measure to reduce the incidence of POCD, but not for the incidence of POD.
Systematic review registration: https://www.crd.york.ac.uk/prospero/display_record.php?ID=CRD42022371306, identifier: CRD42022371306.
Alongside an aging population, the number of older adults undergoing surgical procedures is also increasing (1). Postoperative cognitive dysfunction (POCD) and postoperative delirium (POD) are common and serious postoperative complications in older people that can prolong hospital stay, reduce the quality of life, increase healthcare costs, and even increase mortality (2, 3). POCD is defined as a significant reduction in the cognitive performance from baseline following surgery (4). The incidence of POCD reportedly varies from 1.5 to 28% (5). POD is a postoperative acute and reversible cerebral dysfunction, mainly manifested as confusion and altered consciousness (6). Studies have reported that the incidence of POD after cardiovascular surgery is as high as 15.3–23.4% (7). The specific mechanisms of POCD and POD are still unclear, but studies have revealed that POCD and POD are the result of the interaction between multiple risk factors, including the patient's cognitive function level, coexisting chronic diseases, nutritional status, use of anesthetic drugs, surgery, and pain (8–12). Because of the difficulty in the prevention and treatment of POCD and POD, it is important to find an effective method to reduce the incidence of POCD and POD.
Cognitive training (CT) refers to training programs that involve structured practice of specific cognitive tasks with the goal of improving performance in one or more cognitive domains, such as memory, attention, or executive function (13). Playing video games, reading books, practicing writing, remembering spatial locations, remembering objects or words, and communicating more with the patient are some common ways of CT (14–17). Many studies have shown that CT can improve cognitive function (18). Ball et al. (19) found that cognitive function training with three different cognitive functions (memory, reasoning, and processing speed) was effective in improving the cognitive performance in older adults over the age of 65 years, which was maintained for 2 years. Walton et al. (20), Xuefang et al. (21), Hu et al. (22), and Woolf et al. (23) found that CT can improve the cognitive function of patients with Parkinson's disease, stroke, mild cognitive impairment, and major depression, respectively. However, the effects of CT on POCD and POD are controversial. Saleh et al. (14) showed that preoperative CT significantly reduced the incidence of POCD after gastrointestinal surgery. However, other studies have found no significant difference in the incidence of POCD and POD between patients receiving perioperative CT and the control group, and CT had limitations in terms of the feasibility and patient adherence (24, 25). Therefore, in this meta-analysis, we aimed to investigate the effects of CT on POCD and POD.
The meta-analysis was conducted in accordance with the Preferred Reporting Items for Systematic Reviews and Meta-analysis (PRISMA) checklist (26). Ethics approval was not necessary because this study was a systematic review and meta-analysis. We registered this study in PROSPERO under number CRD42022371306 (https://www.crd.york.ac.uk/prospero/display_record.php?ID=CRD42022371306).
Two reviewers (Li Zhao and Hongyu Zhu) independently searched PubMed, EMBASE, the Cochrane library, and Web of Science from the inception of the databases to 31 August 2022. The search terms used were as follows: “cognitive training or cognitive intervention or memory training” and “perioperative neurocognitive disorders or postoperative cognitive dysfunction or POCD or postoperative delirium or POD”. No limitation was imposed. In addition, we searched the reference lists of the identified articles for relevant studies and manually screened the additional eligible studies.
The inclusion criteria of this study were as follows: (1) Patients in the intervention group received either preoperative CT, postoperative CT, or both. (2) Patients in the control group were treated only for the disease itself, without CT. (3) The diagnostic criteria of POCD and POD were clearly stated in the study. (4) Primary or secondary outcomes must include the incidence of POCD or POD. (5) There was no statistical difference in the cognitive function between the CT and control groups at the time of enrollment. (6) The included studies should be randomized controlled studies or cohort studies. We excluded studies where the data could not be extracted and used for analysis.
The primary outcomes were the incidence of POD and POCD. Secondary outcomes were CT adherence, length of hospital stay, and scores of cognitive function.
Data extraction and quality assessment were carried out by two independent authors (Li Zhao and Hongyu Zhu). If disagreements arose, they were discussed with the corresponding author (Linji Li). The following information was extracted: first author's name, year of publication, country, the average age of the participants, sample size, types of surgery, type of anesthesia, intervention measures, and results of POCD and POD assessment. Study quality was assessed using the Cochrane risk of bias tool. Some data conversion tools were used to convert interquartile ranges to means and standard deviation in some studies (27).
Data analysis was performed by Review Manager (version 5.3) and Stata (version 14) software. Dichotomous and continuous data were analyzed using risk ratio (RR) and mean differences (MD) with 95% confidence interval (CI), with a P-value of <0.05 considered statistically significant. Statistical heterogeneity was used to identify the differences among the included studies. I2 statistic was used to assess statistical heterogeneity, with I2 > 50% considered to be high heterogeneity and I2 < 50% considered to be low heterogeneity (28). The random effects model was used if there was high heterogeneity, while the fixed effects model was used if low heterogeneity was detected (29). Sensitivity analyses and subgroup analyses were used for studies with high heterogeneity. Publication bias was measured by Egger's test (30).
We initially identified a total of 106 studies through database search. Nine studies were eventually included, with a sample size of 975 cases, including 500 cases in the CT group and 475 cases in the control group (14–17, 24, 25, 31–33). The flow chart of study selection is shown in Figure 1.
The characteristics of the studies are shown in Figure 2. A total of five studies assessed the effects of CT on POCD, and five studies assessed the effects of CT on POD.
Figure 2. Characteristics of the included studies. Plus-minus values are mean ± SD. IQR, interquartile range.
The results of assessing the risk of bias for the included studies are shown in the Figure 3. Two studies were considered to have high risk of random sequence generation and allocation concealment (31, 33). Three studies were considered to have unclear risk in allocation concealment (14, 24, 31). None of the included studies were blinded to patients probably because CT requires patient cooperation and takes a long time. Blinding for outcome assessment was unclear in two studies (32, 33). Clinical registrations for three studies were not found, and therefore, the risk was unclear for selective reporting (16, 31, 33). The total sample size of a study was only 50 people, which may have led to partial bias (16).
Five studies assessed the incidence of POCD (14, 16, 17, 24, 32). Two studies (14, 32) reported the incidence of POCD at 7 days postoperatively, and three studies (16, 17, 24) reported the incidence of POCD at hospital discharge. Due to the high heterogeneity (I2 = 61%), the random effects model was chosen and showed that the CT group had a significantly reduced incidence of POCD compared to the control group (RR = 0.5, 95% CI: 0.28–0.89, P = 0.02, Figure 4). No significant publication bias was found using Egger's test (P = 0.718). The Galbraith plots (Figure 5) show a clear heterogeneity between the study by O'Gara et al. and other studies. Sensitivity analysis revealed a significant decrease in heterogeneity (I2 = 0) when the study by O'Gara et al. was removed, but the result was unchanged (RR = 0.38, 95% CI: 0.14–0.49, P < 0.00001). Subgroup analysis (Figure 4) showed significant differences between the CT group and control group for non-cardiac surgery (I2 = 0%, RR = 0.39, 95%: 0.24–0.62, P = 0.0001) but not for cardiac surgery (I2 = 82%, RR = 0.74, 95% CI: 0.19–2.79, P = 0.65). Subgroup analysis of the timing of intervention (Figure 6) revealed that preoperative CT (RR = 0.44, 95% CI: 0.24–0.82, P = 0.01) or postoperative CT (RR = 0.41, 95%: 0.26–0.66, P = 0.0003) significantly reduced the incidence of POCD, but CT during both preoperative and postoperative periods showed no statistically significant difference compared to the control group (RR = 0.72, 95% CI: 0.17–3.03, P = 0.65).
Five studies assessed the incidence of POD (15, 24, 25, 31, 33). One study (25) reported POD within 3 days postoperatively, three studies (15, 24, 33) reported POD within 7 days postoperatively, and one study (31) reported POD at discharge. Due to the high heterogeneity (I2 = 67%), we chose the random effects model and the results showed no statistically significant difference between the two groups (RR = 0.64; 95% CI: 0.29–1.43, P = 0.28, Figure 7). No significant publication bias was found according to Egger's test (P = 0.810). On sensitivity analysis, the results did not change when any of the studies were removed. The Galbraith plots (Figure 8) show a clear heterogeneity between the study by Chen et al. and other studies. The results of the subgroup analysis (Figure 7) showed that there was no statistically significant difference between the CT group and control group for both cardiac surgery (RR = 0.71, 95% CI: 0.16–3.22, P = 0.65) and non-cardiac surgery (RR = 0.54, 95% CI: 0.14–2.11, P = 0.38). Subgroup analysis of the timing of intervention revealed (Figure 9) that preoperative CT (RR = 0.86, 95% CI: 0.37–1.98, P = 0.73) and CT during both the preoperative and postoperative periods (RR = 0.37, 95% CI: 0.06–2.15, P = 0.27) were not statistically different compared to the control group.
Two studies reported CT adherence in the intervention group (15, 25). We defined CT adherence as the proportion of patients in the studies who completed the planned duration of CT. Due to high heterogeneity (I2 = 21.9%), the fixed effects model was chosen and the result showed that the proportion of patients in the CT group who completed the planned duration of CT was 10% (95% CI: 0.05–0.14, P < 0.001, Figure 10).
Two studies (16, 31) used the Mini-mental State Examination (MMSE) scores to assess cognitive function, and one study (24) used the Montreal Cognitive Assessment (MOCA) scores. We extracted the difference by subtracting the baseline measurement from the post-intervention assessment scores of cognitive function in the studies. Due to high heterogeneity (I2 = 85%), we selected the random effects model. The results showed less decline in MMSE scores in the CT group compared to the control group (MD = 1.58, 95% CI: 0.57–2.59, P = 0.002, Figure 11). Another study used MOCA scores, and the difference between the two groups was not statistically significant (P = 0.74).
A total of five studies evaluated the length of hospital stay in the CT and control groups (14, 16, 24, 31, 32). Due to high heterogeneity (I2 = 73%), we used the random effects model and the results showed that the difference in the length of hospital stay between the CT and control groups was not statistically significant (MD: −0.18, 95% CI: −0.93–0.57, P = 0.64, Figure 12). On sensitivity analysis, there was a significant decrease in heterogeneity (I2 = 1%) when the study by Saleh et al. was removed, but the result was unchanged (MD: 0.08, 95% CI: −0.30–0.46, P = 0.68).
With the increasing demand for comfortable perioperative care, more studies have begun to focus on postoperative complications (34). We, therefore, carried out this meta-analysis to evaluate the effect of perioperative CT on POCD and POD. In this meta-analysis, we found that perioperative CT is potentially an effective measure to reduce the incidence of POCD but not the incidence of POD. In addition, our study showed less decline in the cognitive function scores in the CT group compared to the control group. In addition, there was no significant difference in the length of hospital stay. Regarding CT adherence, we found that the proportion of patients in the CT group who completed the planned duration of CT was 10%.
The new 2018 guidelines defined neurocognitive disorders occurring in the perioperative period, including preoperative cognitive impairment, POD, cognitive decline diagnosed within 30 days postoperatively (delayed neurocognitive recovery), and cognitive decline diagnosed within 2–12 months postoperatively (35). As most previous studies have used POD and POCD as the outcome indicators of postoperative cognitive function, we also used POCD and POD to assess the postoperative cognitive function (4).
This meta-analysis showed that perioperative CT significantly reduced the incidence of POCD (P = 0.02). Possible mechanisms underlying the effects of CT in improving cognitive function are as follows. First, CT may increase the density of cortical dopamine D1 receptors, which play a key role in human cognition as it is dependent on adequate dopamine neurotransmission (36). Second, Feinkohl et al. found that patients with more cognitive reserve had a lower incidence of POCD (36). In addition, Mondini et al. have found that CT enhances patients' cognitive reserve (37); thus, CT may improve patients' cognitive function by enhancing their cognitive reserve. Third, studies have found that cognitive function, perception, and memory function decline progressively with age, but the brain retains lifelong plasticity and adaptive reorganization; therefore, some cognitive functions of the brain can be improved by using appropriately designed training programs (38–40).
Furthermore, the results of this meta-analysis showed no statistically significant difference in the incidence of POD between the CT and control groups (P = 0.28). The reason for this outcome is unclear, and it is speculated that it may be due to significant heterogeneity (I2 = 67%) and inadequate sample size of the study.
Of note, three studies assessed CT adherence in the CT group (15, 24, 25). The proportion of patients in the CT group who completed the planned duration of CT was 10% (15, 25). One study reported that the main reasons for low CT adherence were lack of computer access, time constraints, and feeling overwhelmed (25). Another study reported that the main reasons were “I did not have enough energy, I forget, the frequency of game was too often (24).” Therefore, simplifying the training methods and providing computer assistance are necessary to avoid low adherence. O'Gara et al. found that a low proportion of people completed the total scheduled training duration (10 h); however, most were able to complete a longer duration of the cognitive training (>4 h).
Statistical heterogeneity was high in our meta-analysis. This heterogeneity may be due to differences in the types of surgery, diagnostic tools for cognitive function, mean age, duration of CT, timing of CT, and methods of CT. However, we performed subgroup analyses for the type of surgery and timing of CT. Only the non-cardiac surgery subgroup for the POCD outcome showed low heterogeneity (I2 = 0). Subgroup analyses for other categories were not performed due to the variety of diagnostic tools for cognitive function and CT methods, lack of detailed intervention duration, and the small difference in mean age.
There are some limitations of this study. First, the sample size was relatively small. Second, many factors including the methods of CT, duration of CT, and diagnostic methods for POCD and POD differed among the studies, which led to high clinical heterogeneity. Third, as the incidence rates of POCD and POD were our primary outcomes, we excluded studies that did not include data on POCD and POD; therefore, the evidence for secondary outcomes may be insufficient.
Our meta-analysis revealed that perioperative cognitive training is possibly an effective measure to reduce the incidence of POCD but not for the incidence of POD.
The original contributions presented in the study are included in the article/Supplementary material, further inquiries can be directed to the corresponding author.
LZ designed the study and wrote the manuscript. LZ, HZ, XZ, and WM participated in the extraction and analysis of the data. LL and YX critically supervised, evaluated, and validated the article. All of the authors worked on the article and agreed with the submitted version.
This study was supported by research funds from Nanchong Science and Technology Bureau (22SXQT0293).
The authors would like to thank all the authors who participated in this study for their contributions to this study.
The authors declare that the research was conducted in the absence of any commercial or financial relationships that could be construed as a potential conflict of interest.
All claims expressed in this article are solely those of the authors and do not necessarily represent those of their affiliated organizations, or those of the publisher, the editors and the reviewers. Any product that may be evaluated in this article, or claim that may be made by its manufacturer, is not guaranteed or endorsed by the publisher.
The Supplementary Material for this article can be found online at: https://www.frontiersin.org/articles/10.3389/fneur.2023.1146164/full#supplementary-material
1. Safavynia SA, Goldstein PA. The role of neuroinflammation in postoperative cognitive dysfunction: moving from hypothesis to treatment. Front Psychiatry. (2018) 9:752. doi: 10.3389/fpsyt.2018.00752
2. Steinmetz J, Christensen KB, Lund T, Lohse N, Rasmussen LS. Long-term consequences of postoperative cognitive dysfunction. Anesthesiology. (2009) 110:548–55. doi: 10.1097/ALN.0b013e318195b569
3. Rengel KF, Pandharipande PP, Hughes CG. Special considerations for the aging brain and perioperative neurocognitive dysfunction. Anesthesiol Clin. (2019) 37:521–36. doi: 10.1016/j.anclin.2019.04.010
4. Evered LA, Silbert BS. Postoperative cognitive dysfunction and noncardiac surgery. Anesth Analg. (2018) 127:496–505. doi: 10.1213/ANE.0000000000003514
5. Moller JT, Cluitmans P, Rasmussen LS, Houx P, Rasmussen H, Canet J, et al. Long-term postoperative cognitive dysfunction in the elderly ispocd1 study. Ispocd investigators International study of post-operative cognitive dysfunction. Lancet. (1998) 351:857–61. doi: 10.1016/S0140-6736(97)07382-0
7. Sockalingam S, Parekh N, Bogoch II, Sun J, Mahtani R, Beach C, et al. Delirium in the postoperative cardiac patient: a review. J Card Surg. (2005) 20:560–67. doi: 10.1111/j.1540-8191.2005.00134.x
8. Evered L, Atkins K, Silbert B, Scott DA. Acute peri-operative neurocognitive disorders: a narrative review. Anaesthesia. (2022) 77(Suppl. 1):34–42. doi: 10.1111/anae.15613
9. Kong H, Xu LM, Wang DX. Perioperative neurocognitive disorders: a narrative review focusing on diagnosis, prevention, and treatment. Cns Neurosci Ther. (2022) 28:1147–67. doi: 10.1111/cns.13873
10. Xu X, Hu Y, Yan E, Zhan G, Liu C, Yang C. Perioperative neurocognitive dysfunction: thinking from the gut? Aging. (2020) 12:15797–817. doi: 10.18632/aging.103738
11. Ding L, Chen DX Li Q. Effects of electroencephalography and regional cerebral oxygen saturation monitoring on perioperative neurocognitive disorders: a systematic review and meta-analysis. BMC Anesthesiol. (2020) 20:254. doi: 10.1186/s12871-020-01163-y
12. Feinkohl I, Winterer G, Pischon T. Obesity and post-operative cognitive dysfunction: a systematic review and meta-analysis. Diabetes Metab Res Rev. (2016) 32:643–51. doi: 10.1002/dmrr.2786
13. Guglietti B, Hobbs D, Collins-Praino LE. Optimizing cognitive training for the treatment of cognitive dysfunction in Parkinson's disease: current limitations and future directions. Front Aging Neurosci. (2021) 13:709484. doi: 10.3389/fnagi.2021.709484
14. Saleh AJ, Tang GX, Hadi SM, Yan L, Chen MH, Duan KM, et al. Preoperative cognitive intervention reduces cognitive dysfunction in elderly patients after gastrointestinal surgery: a randomized controlled trial. Med Sci Monit. (2015) 21:798–805. doi: 10.12659/MSM.893359
15. Humeidan ML, Reyes JC, Mavarez-Martinez A, Roeth C, Nguyen CM, Sheridan E, et al. Effect of cognitive prehabilitation on the incidence of postoperative delirium among older adults undergoing major noncardiac surgery: the neurobics randomized clinical trial. JAMA Surg. (2021) 156:148–56. doi: 10.1001/jamasurg.2020.4371
16. Cheng CM, Chiu MJ, Wang JH, Liu HC, Shyu YI, Huang GH, et al. Cognitive stimulation during hospitalization improves global cognition of older taiwanese undergoing elective total knee and hip replacement surgery. J Adv Nurs. (2012) 68:1322–29. doi: 10.1111/j.1365-2648.2011.05842.x
17. Butz M, Gerriets T, Sammer G, El-Shazly J, Tschernatsch M, Huttner HB, et al. Effects of postoperative cognitive training on neurocognitive decline after heart surgery: a randomized clinical trial. Eur J Cardiothorac Surg. (2022) 62. doi: 10.1093/ejcts/ezac251
18. Scott JE, Mathias JL, Kneebone AC, Krishnan J. Postoperative cognitive dysfunction and its relationship to cognitive reserve in elderly total joint replacement patients. J Clin Exp Neuropsychol. (2017) 39:459–72. doi: 10.1080/13803395.2016.1233940
19. Ball K, Berch DB, Helmers KF, Jobe JB, Leveck MD, Marsiske M, et al. Effects of cognitive training interventions with older adults: a randomized controlled trial. JAMA. (2002) 288:2271–81. doi: 10.1001/jama.288.18.2271
20. Walton CC, Naismith SL, Lampit A, Mowszowski L, Lewis SJG. Cognitive training in parkinson's disease. Neurorehabil Neural Repair. (2017) 31:207–16. doi: 10.1177/1545968316680489
21. Xuefang L, Guihua W, Fengru M. The effect of early cognitive training and rehabilitation for patients with cognitive dysfunction in stroke. Int J Methods Psychiatr Res. (2021) 30:e1882. doi: 10.1002/mpr.1882
22. Hu M, Wu X, Shu X, Hu H, Chen Q, Peng L, et al. Effects of computerised cognitive training on cognitive impairment: a meta-analysis. J Neurol. (2021) 268:1680–88. doi: 10.1007/s00415-019-09522-7
23. Woolf C, Lampit A, Shahnawaz Z, Sabates J, Norrie LM, Burke D, et al. A systematic review and meta-analysis of cognitive training in adults with major depressive disorder. Neuropsychol Rev. (2022) 32:419–37. doi: 10.1007/s11065-021-09487-3
24. O'Gara BP, Mueller A, Gasangwa D, Patxot M, Shaefi S, Khabbaz K, et al. Prevention of early postoperative decline: a randomized, controlled feasibility trial of perioperative cognitive training. Anesth Analg. (2020) 130:586–95. doi: 10.1213/ANE.0000000000004469
25. Vlisides PE, Das AR, Thompson AM, Kunkler B, Zierau M, Cantley MJ, et al. Home-based cognitive prehabilitation in older surgical patients: a feasibility study. J Neurosurg Anesthesiol. (2019) 31:212–17. doi: 10.1097/ANA.0000000000000569
26. Moher D, Liberati A, Tetzlaff J, Altman DG. Preferred reporting items for systematic reviews and meta-analyses: the prisma statement. BMJ. (2009) 339:b2535. doi: 10.1136/bmj.b2535
27. Wan X, Wang W, Liu J, Tong T. Estimating the sample mean and standard deviation from the sample size, median, range and/or interquartile range. BMC Med Res Methodol. (2014) 14:135. doi: 10.1186/1471-2288-14-135
28. Higgins JP, Thompson SG, Deeks JJ, Altman DG. Measuring inconsistency in meta-analyses. BMJ. (2003) 327:557–60. doi: 10.1136/bmj.327.7414.557
29. Fletcher J. What is heterogeneity and is it important? BMJ. (2007) 334:94–96. doi: 10.1136/bmj.39057.406644.68
30. Egger M, Davey SG, Schneider M, Minder C. Bias in meta-analysis detected by a simple, graphical test. BMJ. (1997) 315:629–34. doi: 10.1136/bmj.315.7109.629
31. Chen CC, Lin MT, Tien YW, Yen CJ, Huang GH, Inouye SK. Modified hospital elder life program: effects on abdominal surgery patients. J Am Coll Surg. (2011) 213:245–42. doi: 10.1016/j.jamcollsurg.2011.05.004
32. Duan S, Liao Y, Tang Y, Zhang B, Peng M, Tong J, et al. Short-term perioperative cognitive therapy combined with rehabilitation exercise reduces the incidence of neurocognitive disorder in elderly patients: a randomized controlled trial. Minerva Anestesiol. (2022) 88:145–55. doi: 10.23736/S0375-9393.21.15877-8
33. Lee J, Jung J, Noh JS, Yoo S, Hong YS. Perioperative psycho-educational intervention can reduce postoperative delirium in patients after cardiac surgery: a pilot study. Int J Psychiatry Med. (2013) 45:143–58. doi: 10.2190/PM.45.2.d
34. Kehlet H. Enhanced postoperative recovery: good from afar, but far from good? Anaesthesia. (2020) 75(Suppl. 1):e54–61. doi: 10.1111/anae.14860
35. Evered L, Silbert B, Knopman DS, Scott DA, DeKosky ST, Rasmussen LS, et al. Recommendations for the nomenclature of cognitive change associated with anaesthesia and surgery-2018. Br J Anaesth. (2018) 121:1005–12. doi: 10.1097/ALN.0000000000002334
36. Feinkohl I, Winterer G, Spies CD, Pischon T. Cognitive reserve and the risk of postoperative cognitive dysfunction. Dtsch Arztebl Int. (2017) 114:110–17. doi: 10.3238/arztebl.2017.0110
37. Mondini S, Madella I, Zangrossi A, Bigolin A, Tomasi C, Michieletto M, et al. Cognitive reserve in dementia: implications for cognitive training. Front Aging Neurosci. (2016) 8:84. doi: 10.3389/fnagi.2016.00084
38. Mahncke HW, Connor BB, Appelman J, Ahsanuddin ON, Hardy JL, Wood RA, et al. Memory enhancement in healthy older adults using a brain plasticity-based training program: a randomized, controlled study. Proc Natl Acad Sci U S A. (2006) 103:12523–28. doi: 10.1073/pnas.0605194103
39. Smith GE, Housen P, Yaffe K, Ruff R, Kennison RF, Mahncke HW, et al. A cognitive training program based on principles of brain plasticity: results from the improvement in memory with plasticity-based adaptive cognitive training (impact) study. J Am Geriatr Soc. (2009) 57:594–603. doi: 10.1111/j.1532-5415.2008.02167.x
Keywords: cognitive function training, cognitive intervention, perioperative cognitive disorders, postoperative cognitive dysfunction, postoperative delirium
Citation: Zhao L, Zhu H, Mao W, Zhou X, Xie Y and Li L (2023) Effects of perioperative cognitive function training on postoperative cognitive dysfunction and postoperative delirium: a systematic review and meta-analysis. Front. Neurol. 14:1146164. doi: 10.3389/fneur.2023.1146164
Received: 15 March 2023; Accepted: 25 May 2023;
Published: 21 June 2023.
Edited by:
Teresa Paolucci, University of Studies G. d'Annunzio Chieti and Pescara, ItalyReviewed by:
Sunil Swami, Blue Health Intelligence (BHI), United StatesCopyright © 2023 Zhao, Zhu, Mao, Zhou, Xie and Li. This is an open-access article distributed under the terms of the Creative Commons Attribution License (CC BY). The use, distribution or reproduction in other forums is permitted, provided the original author(s) and the copyright owner(s) are credited and that the original publication in this journal is cited, in accordance with accepted academic practice. No use, distribution or reproduction is permitted which does not comply with these terms.
*Correspondence: Linji Li, bGxqLXN0ZXBoZW5AMTYzLmNvbQ==
†These authors have contributed equally to this work
Disclaimer: All claims expressed in this article are solely those of the authors and do not necessarily represent those of their affiliated organizations, or those of the publisher, the editors and the reviewers. Any product that may be evaluated in this article or claim that may be made by its manufacturer is not guaranteed or endorsed by the publisher.
Research integrity at Frontiers
Learn more about the work of our research integrity team to safeguard the quality of each article we publish.