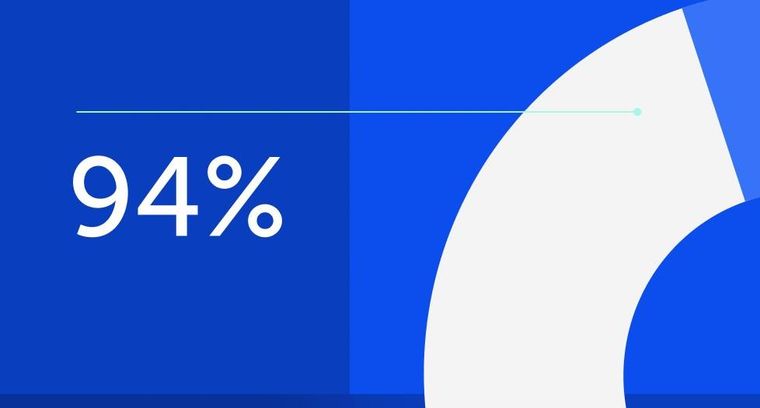
94% of researchers rate our articles as excellent or good
Learn more about the work of our research integrity team to safeguard the quality of each article we publish.
Find out more
MINI REVIEW article
Front. Neurol., 23 February 2023
Sec. Movement Disorders
Volume 14 - 2023 | https://doi.org/10.3389/fneur.2023.1128092
This article is part of the Research TopicBalance-Controlling Mechanism and Fall-Prevention StrategyView all 10 articles
Falls are the main contributor to both fatal and nonfatal injuries in elderly individuals as well as significant sources of morbidity and mortality, which are mostly induced by impaired balance control. The ability to keep balance is a remarkably complex process that allows for rapid and precise changes to prevent falls with multiple systems involved, such as musculoskeletal system, the central nervous system and sensory system. However, the exact pathogenesis of falls caused by balance disorders in the elderly has eluded researchers to date. In consideration of aging phenomenon aggravation and fall risks in the elderly, there is an urgent need to explore the pathogenesis and treatments of falls caused by balance disorders in the elderly. The present review discusses the epidemiology of falls in the elderly, potential pathogenic mechanisms underlying multiple systems involved in falls caused by balance disorders, including musculoskeletal system, the central nervous system and sensory system. Meanwhile, some common treatment strategies, such as physical exercise, new equipment based on artificial intelligence, pharmacologic treatments and fall prevention education are also reviewed. To fully understand the pathogenesis and treatment of falls caused by balance disorders, a need remains for future large-scale multi-center randomized controlled trials and in-depth mechanism studies.
Falls are the leading cause of injury-related mortality among the elderly globally, trailing only traffic accidents in prominence (1), and increasing the mortality rate and disability rate in the elderly. Approximately 27,000 older adults died due to falls in a year (2). Elderly fall victims may endure severe physical harm, including fractures, especially under the circumstance of prior hip surgery or osteoporosis. They may also experience loss of independence and are forced into nursing home admittance (3). Thus, fearfulness of falling might cause social withdrawal and disengagement (4). Psychological and physical injuries resulting from falls impose a heavy social and financial strain on patients' family, community health services, and economy. Consequently, preventing falls in the elderly is a pressing public health issue.
One of the leading causes of falls in the elderly is balance disorders, which frequently result in harm, disabling conditions, loss of independence, and lowered quality of life (5). With several systems cooperating together to prevent falls, good balance is likely the result of a quick synergistic interplay between diverse physiologic and cognitive factors that enables a speedy and precise reaction to the perturbation (6). Elderly people are more inclined to fall due to balance disorders resulting from the steady reduction of several systems' functions, including musculoskeletal system, the central nervous system and sensory system (7–10). The present review discusses the epidemiology of falls in the elderly, potential pathogenic mechanisms underlying multiple systems involved in falls caused by balance disorders, including musculoskeletal system, the central nervous system and sensory system, as well as some common treatment strategies for balance-disorder-induced falls by regulating different systems.
Approximately 28 to 35% of individuals over 65 years of age fall each year, and 32–42% of individuals over 70 years of age fall, according to the WHO's (world health organization) survey. This demonstrates that the risk of falling increases with age (11). 20–30% of mild–severe injuries are the results of falls (12), and more than 50% of such injuries require hospitalization for treatment (13). Among them, about 35% of individuals over 70 years of age and 61% of individuals over 80 years of age have suffered from balance disorders (14). With increasing age, greater mobility difficulty, decreased cognitive function, living alone, more concomitant conditions, and the likelihood of experiencing multiple falls increased dramatically (15). A cross-sectional study on the prevalence and risk factors for falls among the elderly indicates that the associated factors of falls among older adults includes impaired balance ability, less physical activity, cognition impairment, mild and moderate depression (16). In conclusion, falls are common in the elderly, which is the result of a complicated pathological process involving a multitude of factors.
Balance is the ability to maintain the projected center of mass of the body within the stability limits of support, which has three fundamental properties, such as steadiness, symmetry, and dynamic stability (17). In addition to the aging of physiological functions, balance disorders in the elderly also represents the aggregation of pathology in multiple systems, all of which can result in falls (18). Identifying the pathogenic factors of falls caused by balance disorders in the elderly are the premise and basis for the identification, assessment, and control of dysfunction and loss of independence, which are shown briefly in Figure 1.
Figure 1. Summary of pathogenic factors with multiple systems involved in falls caused by balance disorders in the elderly. Musculoskeletal system: In the elderly, decreased thigh and core muscle thickness is favorably connected with dynamic balance and correlated with fall risk. Functional degradation of tendons and joints occurs with aging, which is characterized by decreased muscle mass and reduced contractility, and thus leads to falls. Degenerative spinal deformity, which develops in the elderly due to accumulated degenerative changes brought on by aging, such as asymmetrical disc degeneration, dehydration, and collapse, combined with facet degeneration and ligamentous laxity, can significantly alter the body's center of gravity and cause falls. Central nervous system (CNS): Aging brings about the spinal networks' structural changes that impair their functionality and motor commanding. Unmyelinated fiber density decreases by 37% in the elderly, while myelinated fiber density decreases by 38%, which is the main culprit of elderly's diminished nerve conduction velocity. Physical balance disorders and an elevated risk of falling are caused by the CNS's degeneration, which makes it more difficult for the body to integrate motor signals. Sensory System: The visual system and vestibular system are like GPS and sentry mod. Older people with visual impairment have a higher incidence of falls than those without visual impairment. The decline in vestibular function with increasing age has been confirmed by many studies and thus can be classified as one of the causes of falls.
From a biomechanical point of view, when a body is stationary on a plane, its center of mass and pressure are in a vertical projection that touches the support surface (8). While, when the upright balance is lost, with the shifting center on the wrong supporting surface, falls happen (8, 19). The coordination of skeletal muscles throughout the body helps to maintain the forementioned balance dynamically and statically by impacting biomechanical parameters such as body sway, stride length, stride frequency and symmetry (20–23). Thigh and core muscle thickness is favorably connected with dynamic balance and negatively correlated with fall risks (24). However, functional degradation of tendons and joints occurs with aging, which is characterized by decreased muscle mass and reduced contractility, thus leading to falls (25). Elderly people alter the joint torque of their ankle, knee, and hip joints, as well as their body balance, via coronal and sagittal adjustments (26). Degenerative spinal deformity, which develops in the elderly due to accumulated degenerative changes, such as asymmetrical disc degeneration, dehydration, and collapse, combined with facet degeneration and ligamentous laxity, can significantly alter the body's center of gravity and cause falls (27).
A study indicates that balance disorders in the severe stage of myasthenia are considerably greater than those in non-myasthenia and pre-myopathy, and the chance of falling is higher, demonstrating a strong correlation between the severity of myasthenia and the risk of balance disturbance (28). Sarcopenia, or the loss of muscle mass with aging, is mostly caused by a decrease in the size of the fast muscle fibers (type II), which results in a higher proportion of slow muscle fibers (type I) in elderly patients (18). Therefore, in older people, the muscles' ability to generate force and their contractile characteristics are more uniform. Aged muscles do, in fact, exhibit a decreased functional working range and are still unable to contract quickly. A study indicates that falls are associated with high levels of muscle activation, which are traits of age-related losses in postural stability (29).
The intake of vitamin D also plays an indispensable role in the balance disorders induced by skeletal muscle. Conventionally, vitamin D plays a major role in regulating calcium and phosphorus metabolism. When vitamin D is deficient, bone density and hardness are reduced, making fractures and falls more likely to happen (30). For the link between muscle and vitamin D, many people have focused on the vitamin D receptor (VDR) although VDR cannot be detected in skeletal muscle (31). However, recent studies have shown that muscles can be regulated by VDR, including atrophy, regeneration, and repairment (32–34). Most studies have shown that vitamin D can predict skeletal muscle health in senior adults and improve lower limb muscle mass and function (35–37). However, it is still unclear how vitamin D functions in muscle, even though it has been documented that muscle function improves after supplementation of vitamin D.
Besides the muscular and skeletal control in falls, the presence of the central nervous system (CNS) is often not negligible. When the CNS including afferent and efferent pathways are damaged, such as stroke, spinal cord injury or long-term bedridden, significant changes in the muscle mass will cause myasthenia and eventually lead to falls (38–40). At the same time, the spinal networks' structural changes that impair their functionality and motor command are also brought on by aging. Unmyelinated fiber density decreases by 37% in the elderly, while myelinated fiber density decreases by 38% (41). This is the main culprit of elderly's diminished nerve conduction velocity (42). Physical balance disorders and an elevated risk of falling are caused by the CNS's degeneration, which makes it more difficult for the body to integrate motor signals.
The anterior tibialis muscle, a dorsal foot flexor, is thought to have more efferent pathway degradation than other foot flexors in the elderly, but isometric muscular strength does not appear to be affected until 80 years of age. This is possible because the muscles are collaterally re-innervated by intact nerve endings, which expands the size of the functional remaining motor units (43). The maximum isometric torque (MIT) of the ankle muscles correlates adversely with center of foot pressure (CoP) displacements (43). It is considered that neuropathy-related injury in proprioceptive sensation of ankle and excessive burden of torque development rate in the elderly lead to the decrease of balance function, and thus eventually cause falls (44).
It is now widely known that aging affects the sensory systems involved in the body's orientation and stabilization in space (45). The visual system and vestibular system are like GPS (global positioning system) and sentry mod. As the “GPS” of the human body, the visual system can let us know whether there is danger around us through the input of environmental information, ensure our safe movement, and also predict and give feedback in different directions and spaces (10). A survey of older people in communities found that older people with visual impairment had a higher incidence of falls than those without visual impairment (46). There is also a large proportion of vision loss due to other causes besides aging. Glaucoma, cataract and other diseases that lead to vision loss plague the elderly, which also leads to an increase in falls (47, 48). As the “sentry mod” of the body, the vestibular system is responsible for standing, movement, balance and control of navigation (10). The vestibular system consists of three parts, namely the homogeneous and bony labyrinths, the motion sensors of the vestibular system, and the hair cells (49). The cooperation of extraocular and vestibular functions through the vestibulo-ocular reflex (VOR) helps the body compensate the head and stabilize the image (49, 50). In vestibular-loss patients, when the eyes are closed, it is difficult for the human body to compensate and they are more inclined to fall (51). The decline in vestibular function with increasing age has been confirmed by many studies and thus can be classified as one of the causes of falls (52, 53).
The proprioception and tactility can be complementary to vestibular perception and vision and can also serve as another source of information to the CNS to more accurately control the balance of the body (10, 54). Firstly, muscles, tendons and joints all provide different kinds of information for us to keep our balance and prevent from falling. Muscle spindle is the primary kinesthetic sensor (55). A study of patients with hereditary sensory and autonomic neuropathies (HSANs) found that the absence of functional muscle spindles afferents caused ataxia (56). In addition, the sense of touch is also a subtle but essential part of maintaining balance. Balance scores are also decreased in patients with reduced plantar sensation after stroke, which can be corrected via weight transfer between the legs, especially with the eyes closed (57). In patients with diabetes, researchers have found a significant relationship between sensory loss and falls due to peripheral nerve loss in the feet (58). One study of older adults over 60 years old showed that the risk of recurrent falls in patients with loss of sensation was 3.59 times than that of controls (59).
Recent research has shown that multisensory integration plays an important role in balance management as well. In general, the maintenance of balance does not depend on the above parts alone. Balance maintenance should be viewed as a collaborative process involving multiple systems, with different weights assigned to different components in different tasks (60). Multisensory integration is the process through which the nervous system combines data from several perceiving processes, including hearing, feeling, and other somatosensory events, into a single, unified, coherent, and stable multisensory process (61). Studies have shown that, with aging, the multisensory integration is progressively impaired and probably results in falls (62). Insufficient multisensory re-weighting, which is crucial for postural control in senior persons, has been linked to poor balance control in elderly people who are prone to falling (25, 63). A clinical study determining the association of multisensory integration with mobility outcomes in aging indicates that magnitude of multisensory integration is an incremental predictor of incident fall, over and above balance and other known fall risk factors (64). The prefrontal cortico-cortico facilitation, dedifferentiation, and prefronto-thalamo-cortical gatin have been linked to decreased information processing in an aged brain, which may be the cause of this condition (65). Collectively, balance maintenance should be viewed as a collaborative process involving multiple systems.
As previously mentioned, falls caused by balance disorders in the elderly are complicated and involve multiple systems. Thus, targeted considerations must be taken when treating and rehabilitating elderly patients with postural balance issues. Treatments of balance-disorder-induced falls by regulating different systems were summarized in this review and succinctly outlined in Table 1 (66–76).
Table 1. Summary of studies indicating treatment strategies of balance-disorder-induced falls by regulating different systems in the elderly.
The most effective method to decrease the rate of falls, enhance gait ability, keep balance, and strengthen performance in physically fragile older persons appears to be a multi-component exercise intervention that combines strength, endurance, and balance training (77). Multi-system physical exercise (MPE) is composed of four parts, including proprioceptive training, muscle strength training, reaction training and postural balance training, which can help to restore the function of musculoskeletal system, the CNS and sensory system. A study has shown that under MPE intervention training even without poorly supervised balance and endurance training (78), elderly people over 65 years old who are at risk of falling show significant improvement in all four aspects and muscle strength is significantly increased, and the fall risk reduced (79). At the same time, Tai Chi (TC) is also helpful for improving balance and can prevent falls in the elderly due to requiring more muscle strength of the lower limb joints. Therefore, the body can develop neuromuscular control strategies to maintain body balance and thus reduce the risk of falls (80). Also intense physical activity boosts levels of brain-derived neurotrophic factor (BDNF), slows down the loss of brain tissue, increases hippocampal capacity, boosts cerebral blood flow, and enhances CNS performance, including executive functions, restoring balance and lowering the risk of accidents (81).
New equipment based on artificial intelligence have been introduced to enrich the whole recovering process. Robot assisted training (RAGT) can bring many benefits to patients, including muscle strength, power, range of motion and so on. Patients with poststroke ankle spasms could significantly improve ankle spasms and increase balance after RAGT intervention (82). Virtual reality can be combined with RAGT to improve patients' gait (83). This combination enhances the function of musculoskeletal system, the CNS and sensory system in the elderly. Recently, a large number of fall detection system (FDS) have been developed, which can be divided into the following three categories: video-based (84), ambient sensor-based (85) and wearable sensor-based (86). A study invented the class-imbalanced deep learning fall detection (CDL-Fall) with a specificity of 91.86%, an F-Score of 98.44%, which is effective on class-imbalanced data and more suitable for real-life application algorithm (87). It has been demonstrated that a novel form of shoe insert called SoleSensor® (U.S. patent issued in 2001, licensed to Hart Mobility, Inc.), can improve the ability of sensory system in the elderly, which is effective in preventing falls (88). In developing novel, cost-effective interventions aimed at identifying specific balancing systems in the elderly, greater attention needs to be paid to target and implement artificial intelligence.
Beyond improved bone health, vitamin D helps to prevent falls and fractures. Strengthening muscles with vitamin D helps lowering the risk of falling. According to a meta-analysis, supplementing with vitamin D at a dose of 700 to 1000 IU per day lowers the risk of falling in older people by 19% (89). Before beginning supplementing, the doctor should also find out whether the elderly patient is using any over-the-counter medications that include vitamin D, as too much vitamin D might cause hypercalcemia. The practical strategy is to promote a vitamin D-rich, healthy, balanced diet (90). In the elderly, polypharmacy is quite common, and thus a lot of side effects from clinically drug-drug interaction emerges, including orthostatic hypotension, dizziness, and somnolence, all of which can lead to falls. According to a study, there was a 39% decrease in the rate of falling when psychotropic medicines, such as benzodiazepines, other sleep aids, neuroleptic agents, and antidepressants, were tapered and stopped over the course of a 14-week period (91). Besides, balance disorder induced by neurally mediated hypotension should get specialized treatments and prescriptions (92).
The education of fall prevention is a necessary part of the whole strategy and runs through the whole process. A meta-analysis of six fall prevention education (FPE) programs indicated that FPE intervention reduced the incidence of fall-related behaviors among community-dwelling residents (93). The content of the education generally includes: the definition of falls, the prevalence of falls, risk factors, and complications of falls. The final goal is to make patients aware of their current situation and how to adjust and change by themselves, and seek the help of care-giver (93). Especially for the elderly with fear of falling (FOF), the combination of FPE and other therapies has greatly reduced their FOF, thus reducing the occurrence of falls (94).
Multiple systems, including the musculoskeletal system, the CNS and sensory system, are all involved in the mechanism underlying the increased occurrence of falls caused by balance disorders in the elderly. Contemporary research, however, has mostly focused on the parallels and correlations between the various systems, rather than the basic processes of falls brought on by balance impairments in the elderly. Few randomized controlled trials and animal model experiments, in contrast, thoroughly examine the mechanism of the efficient treatment approach. Previous research has shown that physical exercise, new technology based on artificial intelligence, pharmacological treatments, and fall prevention education can effectively treat falls caused by balance disorders in the elderly. However, due to varied approaches and a lack of randomized controlled studies with a high sample size, there is currently a lack of useful data supporting the use of these strategies to particularly target certain systems. Future large-scale multicenter randomized controlled trials, in-depth mechanistic research, including big multicenter trials are still required to completely understand the underlying mechanism and management of falls brought on by balance disorders in the elderly.
All authors listed have made a substantial, direct, and intellectual contribution to the work, and approved it for publication.
This study was supported by the National Natural Science Foundation of China (31960178 and 82160923), Applied Basic Research Programs of Science and Technology Commission Foundation of Yunnan Province (2019FA007), Key Laboratory of Traditional Chinese Medicine for Prevention and Treatment of Neuropsychiatric Diseases, Yunnan Provincial Department of Education; Scientific Research Projects for High-level Talents of Yunnan University of Chinese Medicine (2019YZG01), Young Top-Notch Talent in 10,000 Talent Program of Yunnan Province (YNWR-QNBJ-2019-235), National Science and Technology Innovation 2030 Major Program (2021ZD0200900), Yunnan Key Research and Development Program (202103AC100005), and Yunnan Province Fabao Gao Expert Workstation Construction Project (202105AF150037).
The authors declare that the research was conducted in the absence of any commercial or financial relationships that could be construed as a potential conflict of interest.
All claims expressed in this article are solely those of the authors and do not necessarily represent those of their affiliated organizations, or those of the publisher, the editors and the reviewers. Any product that may be evaluated in this article, or claim that may be made by its manufacturer, is not guaranteed or endorsed by the publisher.
1. Bergen G, Stevens MR, Burns ER. Falls and fall injuries among adults aged ≥65 Years - United States, 2014. MMWR Morb Mortal Wkly Rep. (2016) 65:993–8. doi: 10.15585/mmwr.mm6537a2
2. Rubenstein LZ. Falls in older people: epidemiology, risk factors and strategies for prevention. Age Ageing. (2006) 35:ii37–41. doi: 10.1093/ageing/afl084
3. Conley RB, Adib G, Adler RA, Åkesson KE, Alexander IM, Amenta KC, et al. Secondary fracture prevention: consensus clinical recommendations from a multistakeholder coalition. J Bone Miner Res. (2020) 35:36–52. doi: 10.1002/jbmr.3877
4. Arfken CL, Lach HW, Birge SJ, Miller JP. The prevalence and correlates of fear of falling in elderly persons living in the community. Am J Public Health. (1994) 84:565–70. doi: 10.2105/AJPH.84.4.565
5. Cuevas-Trisan R. Balance problems and fall risks in the elderly. Clin Geriatr Med. (2019) 35:173–83. doi: 10.1016/j.cger.2019.01.008
6. Richardson JK. Imbalanced: the confusing circular nature of falls research…and a possible antidote. Am J Phys Med Rehabil. (2017) 96:55–9. doi: 10.1097/PHM.0000000000000591
7. Melzer I, Kurz I, Oddsson LI. A. Retrospective analysis of balance control parameters in elderly fallers and non-fallers. Clin Biomech. (2010) 25:984–8. doi: 10.1016/j.clinbiomech.2010.07.007
8. Yiou E, Caderby T, Delafontaine A, Fourcade P, Honeine JL. Balance control during gait initiation: state-of-the-art and research perspectives. World J Orthop. (2017) 8:815–28. doi: 10.5312/wjo.v8.i11.815
9. Annweiler C, Montero-Odasso M, Schott AM, Berrut G, Fantino B, Beauchet O. Fall prevention and vitamin D in the elderly: an overview of the key role of the non-bone effects. J Neuroeng Rehabil. (2010) 7:50. doi: 10.1186/1743-0003-7-50
10. Lord SR, Delbaere K, Sturnieks DL. Aging. Handb Clin Neurol. (2018) 159:157–71. doi: 10.1016/B978-0-444-63916-5.00010-0
11. Kalache A, Fu D, Yoshida S, Alfaisal W, Beattie L, Chodzkozajko W, et al. World Health Organisation Global Report on Falls Prevention in Older Age. Geneva: World Health Organisation. (2007).
12. Scuffham P, Chaplin S, Legood R. Incidence and costs of unintentional falls in older people in the United Kingdom. J Epidemiol Community Health. (2003) 57:740–4. doi: 10.1136/jech.57.9.740
13. Stinchcombe A, Kuran N, Powell S. Report summary. seniors' falls in canada: second report: key highlights. Chronic Dis Inj Can. (2014) 34:171–4. doi: 10.24095/hpcdp.34.2/3.13
14. Auvinet B, Touzard C, Montestruc F, Delafond A, Goeb V. Gait disorders in the elderly and dual task gait analysis: a new approach for identifying motor phenotypes. J Neuroeng Rehabil. (2017) 14:7. doi: 10.1186/s12984-017-0218-1
15. Kuo FL, Yen CM, Chen HJ, Liao ZY, Lee Y. Trajectories of mobility difficulty and falls in community-dwelling adults aged 50 + in Taiwan from 2003 to 2015. BMC Geriatr. (2022) 22:902. doi: 10.1186/s12877-022-03613-3
16. Lin XZ, Meng RL, Peng DD Li C, Zheng XY, Xu HF, et al. Cross-sectional study on prevalence and risk factors for falls among the elderly in communities of Guangdong Province, China. BMJ Open. (2022) 12:e062257. doi: 10.1136/bmjopen-2022-062257
17. Leroux A, Pinet H, Nadeau S. Task-oriented intervention in chronic stroke: changes in clinical and laboratory measures of balance and mobility. Am J Phys Med Rehabil. (2006) 85:820–30. doi: 10.1097/01.phm.0000233179.64769.8c
18. Laurence BD, Michel L. The fall in older adults: physical and cognitive problems. Curr Aging Sci. (2017) 10:185–200. doi: 10.2174/1874609809666160630124552
19. Winter DA, Patla AE, Prince F, Ishac M, Gielo-Perczak K. Stiffness control of balance in quiet standing. J Neurophysiol. (1998) 80:1211–21. doi: 10.1152/jn.1998.80.3.1211
20. Allum JH, Carpenter MG. A speedy solution for balance and gait analysis: angular velocity measured at the centre of body mass. Curr Opin Neurol. (2005) 18:15–21. doi: 10.1097/00019052-200502000-00005
21. Kerkhoff YRA, van Boxtel W, Louwerens JWK, Keijsers NLW. Asymmetries in gait and balance control after ankle arthrodesis. J Foot Ankle Surg. (2018) 57:899–903. doi: 10.1053/j.jfas.2018.03.022
22. Pai YC, Patton J. Center of mass velocity-position predictions for balance control. J Biomech. (1997) 30:347–54. doi: 10.1016/S0021-9290(96)00165-0
23. Serrao M, Casali C, Ranavolo A, Mari S, Conte C, Chini G, et al. Use of dynamic movement orthoses to improve gait stability and trunk control in ataxic patients. Eur J Phys Rehabil Med. (2017) 53:735–43. doi: 10.23736/S1973-9087.17.04480-X
24. Özkal Ö, Kara M, Topuz S, Kaymak B, Baki A, Özçakar L. Assessment of core and lower limb muscles for static/dynamic balance in the older people: an ultrasonographic study. Age Ageing. (2019) 48:881–7. doi: 10.1093/ageing/afz079
25. Stel VS, Smit JH, Pluijm SM, Lips P. Balance and mobility performance as treatable risk factors for recurrent falling in older persons. J Clin Epidemiol. (2003) 56:659–68. doi: 10.1016/S0895-4356(03)00082-9
26. Lu J, Cai B, Fan S. Research progress of hip joint function in patients with chronic ankle instability. Chin J Rehab. (2019) 34:328–32. doi: 10.3870/zgkf.2019.06.013
27. Ailon T, Smith JS, Shaffrey CI, Lenke LG, Brodke D, Harrop JS, et al. Degenerative spinal deformity. Neurosurgery. (2015) 77:S75–91. doi: 10.1227/NEU.0000000000000938
28. Chen Y, Liu Y, Wu A, Liu X. Explore the correlation between sarcopenia at different stages and fall risk in elderly women in community. Zhejiang Medicine. (2019) 41:550–4. doi: 10.12056/j.issn.1006-2785.2019.41.6.2018-2351
29. Laughton CA, Slavin M, Katdare K, Nolan L, Bean JF, Kerrigan DC, et al. Aging, muscle activity, and balance control: physiologic changes associated with balance impairment. Gait Posture. (2003) 18:101–8. doi: 10.1016/S0966-6362(02)00200-X
30. Carmeliet G, Dermauw V, Bouillon R. Vitamin D signaling in calcium and bone homeostasis: a delicate balance. Best Pract Res Clin Endocrinol Metab. (2015) 29:621–31. doi: 10.1016/j.beem.2015.06.001
31. Wang Y, DeLuca HF. Is the vitamin D receptor found in muscle? Endocrinology. (2011) 152:354–63. doi: 10.1210/en.2010-1109
32. Mori R, Yokokawa T, Fujita S. Modified expression of vitamin D receptor and cyp27b1 in denervation-induced muscle atrophy. Biochem Biophys Res Commun. (2020) 529:733–9. doi: 10.1016/j.bbrc.2020.05.205
33. Latham CM, Brightwell CR, Keeble AR, Munson BD, Thomas NT, Zagzoog AM, et al. Vitamin D promotes skeletal muscle regeneration and mitochondrial health. Front Physiol. (2021) 12:660498. doi: 10.3389/fphys.2021.660498
34. Girgis CM. Vitamin D and skeletal muscle: emerging roles in development, anabolism and repair. Calcif Tissue Int. (2020) 106:47–57. doi: 10.1007/s00223-019-00583-4
35. Granic A, Hill TR, Davies K, Jagger C, Adamson A, Siervo M, et al. Vitamin D status, muscle strength and physical performance decline in very old adults: a prospective study. Nutrients. (2017) 9:4. doi: 10.3390/nu9040379
36. Bauer JM, Verlaan S, Bautmans I, Brandt K, Donini LM, Maggio M, et al. Effects of a vitamin D and leucine-enriched whey protein nutritional supplement on measures of sarcopenia in older adults, the provide study: a randomized, double-blind, placebo-controlled trial. J Am Med Dir Assoc. (2015) 16:740–7. doi: 10.1016/j.jamda.2015.05.021
37. Braga M, Simmons Z, Norris KC, Ferrini MG, Artaza JN. Vitamin D induces myogenic differentiation in skeletal muscle derived stem cells. Endocr Connect. (2017) 6:139–50. doi: 10.1530/EC-17-0008
38. Yoshimura Y, Bise T, Shimazu S, Tanoue M, Tomioka Y, Araki M, et al. Effects of a leucine-enriched amino acid supplement on muscle mass, muscle strength, and physical function in post-stroke patients with sarcopenia: a randomized controlled trial. Nutrition. (2019) 58:1–6. doi: 10.1016/j.nut.2018.05.028
39. Lester RM, Ghatas MP, Khan RM, Gorgey AS. Prediction of thigh skeletal muscle mass using dual energy x-ray absorptiometry compared to magnetic resonance imaging after spinal cord injury. J Spinal Cord Med. (2019) 42:622–30. doi: 10.1080/10790268.2019.1570438
40. Wade RC, Gorgey AS. Anthropometric prediction of skeletal muscle cross-sectional area in persons with spinal cord injury. J Appl Physiol. (1985) 122:1255–61. doi: 10.1152/japplphysiol.01042.2016
41. Jacobs JM, Love S. Qualitative and quantitative morphology of human sural nerve at different Ages. Brain. (1985) 108:897–924. doi: 10.1093/brain/108.4.897
42. Verdú E, Ceballos D, Vilches JJ, Navarro X. Influence of aging on peripheral nerve function and regeneration. J Peripher Nerv Syst. (2000) 5:191–208. doi: 10.1046/j.1529-8027.2000.00026.x
43. McNeil CJ, Doherty TJ, Stashuk DW, Rice CL. Motor unit number estimates in the tibialis anterior muscle of young, old, and very old men. Muscle Nerve. (2005) 31:461–7. doi: 10.1002/mus.20276
44. Brooks SV, Faulkner JA. Skeletal muscle weakness in old age: underlying mechanisms. Med Sci Sports Exerc. (1994) 26:432–9. doi: 10.1249/00005768-199404000-00006
45. Sleimen-Malkoun R, Temprado JJ, Hong SL. Aging induced loss of complexity and dedifferentiation: consequences for coordination dynamics within and between brain, muscular and behavioral levels. Front Aging Neurosci. (2014) 6:140. doi: 10.3389/fnagi.2014.00140
46. Zhou H, Peng K, Tiedemann A, Peng J, Sherrington C. Risk factors for falls among older community dwellers in Shenzhen, China. Inj Prev. (2019) 25:31–5. doi: 10.1136/injuryprev-2017-042597
47. Montana CL, Bhorade AM. Glaucoma and quality of life: fall and driving risk. Curr Opin Ophthalmol. (2018) 29:135–40. doi: 10.1097/ICU.0000000000000455
48. Keay L, Palagyi A, McCluskey P, Lamoureux E, Pesudovs K, Lo S, et al. Falls in older people with cataract, a longitudinal evaluation of impact and risk: the focus study protocol. Inj Prev. (2014) 20:e7. doi: 10.1136/injuryprev-2013-041124
50. Dieterich M, Brandt T. Vestibulo-ocular reflex. Curr Opin Neurol. (1995) 8:83–8. doi: 10.1097/00019052-199502000-00014
51. Mergner T, Schweigart G, Fennell L, Maurer C. Posture control in vestibular-loss patients. Ann N Y Acad Sci. (2009) 1164:206–15. doi: 10.1111/j.1749-6632.2008.03722.x
52. Johnson C, Hallemans A, Verbecque E, De Vestel C, Herssens N, Vereeck L. Aging and the relationship between balance performance, vestibular function and somatosensory thresholds. J Int Adv Otol. (2020) 16:328–37. doi: 10.5152/iao.2020.8287
53. Agrawal Y, Merfeld DM, Horak FB, Redfern MS, Manor B, Westlake KP, et al. Aging, Vestibular function, and balance: proceedings of a national institute on aging/national institute on deafness and other communication disorders workshop. J Gerontol A Biol Sci Med Sci. (2020) 75:2471–80. doi: 10.1093/gerona/glaa097
54. White O, Babič J, Trenado C, Johannsen L, Goswami N. The promise of stochastic resonance in falls prevention. Front Physiol. (2018) 9:1865. doi: 10.3389/fphys.2018.01865
55. Proske U, Gandevia SC. The proprioceptive senses: their roles in signaling body shape, body position and movement, and muscle force. Physiol Rev. (2012) 92:1651–97. doi: 10.1152/physrev.00048.2011
56. Macefield VG, Norcliffe-Kaufmann L, Gutiérrez J, Axelrod FB, Kaufmann H. Can loss of muscle spindle afferents explain the ataxic gait in riley-day syndrome? Brain. (2011) 134:3198–208. doi: 10.1093/brain/awr168
57. Parsons SL, Mansfield A, Inness EL, Patterson KK. The relationship of plantar cutaneous sensation and standing balance post-stroke. Top Stroke Rehabil. (2016) 23:326–32. doi: 10.1080/10749357.2016.1162396
58. Rinkel WD, van Nieuwkasteele S, Castro Cabezas M, van Neck JW, Birnie E, Coert JH. Balance, risk of falls, risk factors and fall-related costs in individuals with diabetes. Diabetes Res Clin Pract. (2019) 158:107930. doi: 10.1016/j.diabres.2019.107930
59. Vu HM, Nguyen LH, Nguyen HLT, Vu GT, Nguyen CT, Hoang TN, et al. Individual and environmental factors associated with recurrent falls in elderly patients hospitalized after falls. Int J Environ Res Public Health. (2020) 17:7. doi: 10.3390/ijerph17072441
60. Li L, Zhang S, Dobson J. The contribution of small and large sensory afferents to postural control in patients with peripheral neuropathy. J Sport Health Sci. (2019) 8:218–27. doi: 10.1016/j.jshs.2018.09.010
61. Zhang S, Xu W, Zhu Y, Tian E, Kong W. Impaired multisensory integration predisposes the elderly people to fall: a systematic review. Front Neurosci. (2020) 14:411. doi: 10.3389/fnins.2020.00411
62. Teasdale N, Stelmach GE, Breunig A, Meeuwsen HJ. Age differences in visual sensory integration. Exp Brain Res. (1991) 85:691–6. doi: 10.1007/BF00231755
63. Stephen JM, Knoefel JE, Adair J, Hart B, Aine CJ. Aging-related changes in auditory and visual integration measured with meg. Neurosci Lett. (2010) 484:76–80. doi: 10.1016/j.neulet.2010.08.023
64. Mahoney JR, Cotton K, Verghese J. Multisensory integration predicts balance and falls in older adults. J Gerontol A Biol Sci Med Sci. (2019) 74:1429–35. doi: 10.1093/gerona/gly245
65. Zikopoulos B, Barbas H. Prefrontal projections to the thalamic reticular nucleus form a unique circuit for attentional mechanisms. J Neurosci. (2006) 26:7348–61. doi: 10.1523/JNEUROSCI.5511-05.2006
66. Moawd SA, Nambi G, Alrawaili SM, Abdelbasset WK, Aboelmagd F, Elsayed SH. Analyzing the influence of the combination of monochromatic infrared energy and tai chi exercise improve balance in community-dwelling older adults with lower-extremity disease: a double-blinded randomized controlled study. Eur Rev Med Pharmacol Sci. (2022) 26:7788–96. doi: 10.26355/eurrev_202211_30128
67. López-García A, Sánchez-Ruíz ME. Feasible intervention through simple exercise for risk of falls in dementia patients: a pilot study. Int J Environ Res Public Health. (2022) 19:19. doi: 10.3390/ijerph191911854
68. Feng Y, Liu J, Si J. Effects of chinese fitness dancing on lower limb strength and fall risk in middle-aged and older women: a cross-sectional study. Nurs Health Sci. (2022) 11:1–9. doi: 10.1111/nhs.12992
69. Benitez-Lugo ML, Suárez-Serrano C, Galvao-Carmona A, Vazquez-Marrufo M, Chamorro-Moriana G. Effectiveness of feedback-based technology on physical and cognitive abilities in the elderly. Front Aging Neurosci. (2022) 14:1050518. doi: 10.3389/fnagi.2022.1050518
70. Kim SH, Cho SH. Benefits of virtual reality program and motor imagery training on balance and fall efficacy in isolated older adults: a randomized controlled trial. Medicina. (2022) 58:11. doi: 10.3390/medicina58111545
71. Choi HS, Baek YS, In H. Ankle strategy assistance to improve gait stability using controllers based on in-shoe center of pressure in 2 degree-of-freedom powered ankle-foot orthoses: a clinical study. J Neuroeng Rehabil. (2022) 19:114. doi: 10.1186/s12984-022-01092-6
72. Ozsoy-Unubol T, Candan Z, Atar E, Ok NF, Ata E, Kilac H, et al. The effect of vitamin d and exercise on balance and fall risk in postmenopausal women: a randomised controlled study. Int J Clin Pract. (2021) 75:e14851. doi: 10.1111/ijcp.14851
73. Just KS, Dallmeier D, Böhme M, Steffens M, Braisch U, Denkinger MD, et al. Fall-associated drugs in community-dwelling older adults: results from the actife ulm study. J Am Med Dir Assoc. (2021) 22:2177–83. doi: 10.1016/j.jamda.2020.12.032
74. Hong C, Lee H, Lee M. Comparison of the effects of education only and exercise training combined with education on fall prevention in adults aged 70 years or older residing in elderly residential facilities. J Korean Acad Nurs. (2021) 51:173–87. doi: 10.4040/jkan.20203
75. Kuhirunyaratn P, Prasomrak P, Jindawong B. Effects of a health education program on fall risk prevention among the urban elderly: a quasi-experimental study. Iran J Public Health. (2019) 48:103–11. doi: 10.18502/ijph.v48i1.788
76. Lee C, Park H. Effects of a fall prevention program based on goal attainment theory for homebound older adults with osteoarthritis of the lower extremities. Orthop Nurs. (2022) 41:414–27. doi: 10.1097/NOR.0000000000000898
77. Cadore EL, Rodríguez-Mañas L, Sinclair A, Izquierdo M. Effects of different exercise interventions on risk of falls, gait ability, and balance in physically frail older adults: a systematic review. Rejuvenation Res. (2013) 16:105–14. doi: 10.1089/rej.2012.1397
78. Lacroix A, Kressig RW, Muehlbauer T, Gschwind YJ, Pfenninger B, Bruegger O, et al. Effects of a supervised versus an unsupervised combined balance and strength training program on balance and muscle power in healthy older adults: a randomized controlled trial. Gerontology. (2016) 62:275–88. doi: 10.1159/000442087
79. Chittrakul J, Siviroj P, Sungkarat S, Sapbamrer R. Multi-system physical exercise intervention for fall prevention and quality of life in pre-frail older adults: a randomized controlled trial. Int J Environ Res Public Health. (2020) 17:9. doi: 10.3390/ijerph17093102
80. Yang F, Liu W. Biomechanical mechanism of tai-chi gait for preventing falls: a pilot study. J Biomech. (2020) 105:109769. doi: 10.1016/j.jbiomech.2020.109769
81. Deslandes A, Moraes H, Ferreira C, Veiga H, Silveira H, Mouta R, et al. Exercise and mental health: many reasons to move. Neuropsychobiology. (2009) 59:191–8. doi: 10.1159/000223730
82. Zhai X, Wu Q, Li X, Xu Q, Zhang Y, Fan S, et al. Effects of robot-aided rehabilitation on the ankle joint properties and balance function in stroke survivors: a randomized controlled trial. Front Neurol. (2021) 12:719305. doi: 10.3389/fneur.2021.719305
83. Kayabinar B, Alemdaroglu-Gürbüz I, Yilmaz Ö. The effects of virtual reality augmented robot-assisted gait training on dual-task performance and functional measures in chronic stroke: a randomized controlled single-blind trial. Eur J Phys Rehabil Med. (2021) 57:227–37. doi: 10.23736/S1973-9087.21.06441-8
84. Lu N, Wu Y, Feng L, Song J. Deep learning for fall detection: three-dimensional cnn combined with lstm on video kinematic data. IEEE J Biomed Health Inform. (2019) 23:314–23. doi: 10.1109/JBHI.2018.2808281
85. Ariani A, Redmond SJ, Chang D, Lovell NH. Software simulation of unobtrusive falls detection at night-time using passive infrared and pressure mat sensors. Annu Int Conf IEEE Eng Med Biol Soc. (2010) 2010:2115–8. doi: 10.1109/IEMBS.2010.5627202
86. Bourke AK, Lyons GM. A. Threshold-based fall-detection algorithm using a bi-axial gyroscope sensor. Med Eng Phys. (2008) 30:84–90. doi: 10.1016/j.medengphy.2006.12.001
87. Zhang J, Li J, Wang W, A. Class-imbalanced deep learning fall detection algorithm using wearable sensors. Sensors. (2021) 21:19. doi: 10.3390/s21196511
88. Maki BE, Sibley KM, Jaglal SB, Bayley M, Brooks D, Fernie GR, et al. Reducing fall risk by improving balance control: development, evaluation and knowledge-translation of new approaches. J Safety Res. (2011) 42:473–85. doi: 10.1016/j.jsr.2011.02.002
89. Bischoff-Ferrari HA, Dawson-Hughes B, Staehelin HB, Orav JE, Stuck AE, Theiler R, et al. Fall Prevention with supplemental and active forms of vitamin d: a meta-analysis of randomised controlled trials. BMJ. (2009) 339:b3692. doi: 10.1136/bmj.b3692
90. Luk JK, Chan TY, Chan DK. Falls prevention in the elderly: translating evidence into practice. Hong Kong Med J. (2015) 21:165–71. doi: 10.12809/hkmj144469
91. Campbell AJ, Robertson MC, Gardner MM, Norton RN, Buchner DM. Psychotropic medication withdrawal and a home-based exercise program to prevent falls: a randomized, controlled trial. J Am Geriatr Soc. (1999) 47:850–3. doi: 10.1111/j.1532-5415.1999.tb03843.x
92. Carey BJ, Potter JF. Cardiovascular causes of falls. Age Ageing. (2001) 30:19–24. doi: 10.1093/ageing/30.suppl_4.19
93. Ong MF, Soh KL, Saimon R, Wai MW, Mortell M, Soh KG. Fall prevention education to reduce fall risk among community-dwelling older persons: a systematic review. J Nurs Manag. (2021) 29:2674–88. doi: 10.1111/jonm.13434
Keywords: balance, fall, elderly, pathogenesis, treatments, mechanism
Citation: Xing L, Bao Y, Wang B, Shi M, Wei Y, Huang X, Dai Y, Shi H, Gai X, Luo Q, Yin Y and Qin D (2023) Falls caused by balance disorders in the elderly with multiple systems involved: Pathogenic mechanisms and treatment strategies. Front. Neurol. 14:1128092. doi: 10.3389/fneur.2023.1128092
Received: 20 December 2022; Accepted: 07 February 2023;
Published: 23 February 2023.
Edited by:
Christina Zong-Hao Ma, Hong Kong Polytechnic University, ChinaReviewed by:
Wenchao Xu, Huazhong University of Science and Technology, ChinaCopyright © 2023 Xing, Bao, Wang, Shi, Wei, Huang, Dai, Shi, Gai, Luo, Yin and Qin. This is an open-access article distributed under the terms of the Creative Commons Attribution License (CC BY). The use, distribution or reproduction in other forums is permitted, provided the original author(s) and the copyright owner(s) are credited and that the original publication in this journal is cited, in accordance with accepted academic practice. No use, distribution or reproduction is permitted which does not comply with these terms.
*Correspondence: Dongdong Qin, cWluZG9uZzEwOEAxNjMuY29t; Yong Yin,
eXlpbnBtckAxMjYuY29t; Qiu Luo,
bHVvcWl1LTIyMEAxNjMuY29t
†These authors have contributed equally to this work
Disclaimer: All claims expressed in this article are solely those of the authors and do not necessarily represent those of their affiliated organizations, or those of the publisher, the editors and the reviewers. Any product that may be evaluated in this article or claim that may be made by its manufacturer is not guaranteed or endorsed by the publisher.
Research integrity at Frontiers
Learn more about the work of our research integrity team to safeguard the quality of each article we publish.