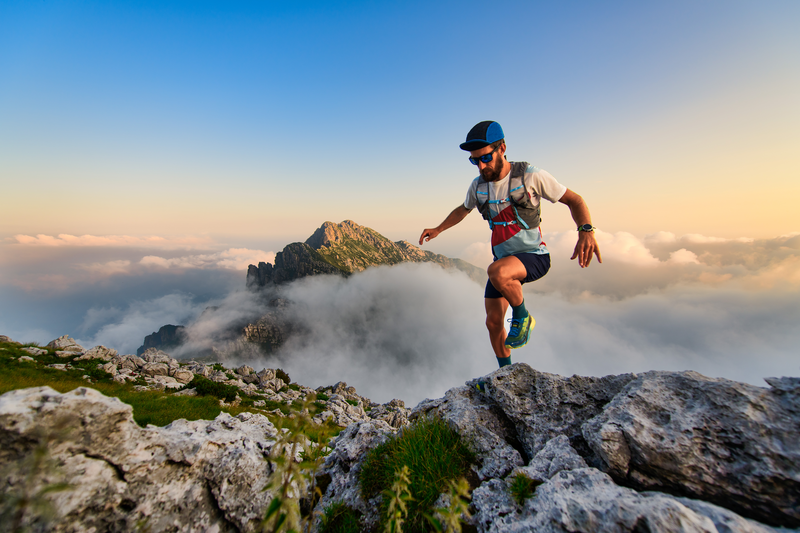
94% of researchers rate our articles as excellent or good
Learn more about the work of our research integrity team to safeguard the quality of each article we publish.
Find out more
REVIEW article
Front. Neurol. , 20 June 2023
Sec. Neuro-Otology
Volume 14 - 2023 | https://doi.org/10.3389/fneur.2023.1122420
This article is part of the Research Topic Hearing Loss Rehabilitation and Higher-Order Auditory and Cognitive Processing View all 16 articles
Parts of this article's content have been modified or rectified in:
Erratum: A review of auditory processing and cognitive change during normal ageing, and the implications for setting hearing aids for older adults
Throughout our adult lives there is a decline in peripheral hearing, auditory processing and elements of cognition that support listening ability. Audiometry provides no information about the status of auditory processing and cognition, and older adults often struggle with complex listening situations, such as speech in noise perception, even if their peripheral hearing appears normal. Hearing aids can address some aspects of peripheral hearing impairment and improve signal-to-noise ratios. However, they cannot directly enhance central processes and may introduce distortion to sound that might act to undermine listening ability. This review paper highlights the need to consider the distortion introduced by hearing aids, specifically when considering normally-ageing older adults. We focus on patients with age-related hearing loss because they represent the vast majority of the population attending audiology clinics. We believe that it is important to recognize that the combination of peripheral and central, auditory and cognitive decline make older adults some of the most complex patients seen in audiology services, so they should not be treated as “standard” despite the high prevalence of age-related hearing loss. We argue that a primary concern should be to avoid hearing aid settings that introduce distortion to speech envelope cues, which is not a new concept. The primary cause of distortion is the speed and range of change to hearing aid amplification (i.e., compression). We argue that slow-acting compression should be considered as a default for some users and that other advanced features should be reconsidered as they may also introduce distortion that some users may not be able to tolerate. We discuss how this can be incorporated into a pragmatic approach to hearing aid fitting that does not require increased loading on audiology services.
In this review paper, we highlight the importance of recognizing the effects of normal ageing on hearing aid fitting because it has a considerable impact on an individual’s understanding of speech and ability to benefit from hearing aids. Auditory processing and cognition decline throughout early, middle and late adult life and undermine our ability to hear in all types of listening situations. “Normal ageing” refers to non-pathological changes in cognition and auditory ability with age (1). We do not discuss pathological changes with age such as mild cognitive impairment or dementia. Whilst considerable research efforts continue to be deployed to understand the association between hearing loss and dementia, we believe it is particularly important to address normally-ageing older adults because they represent the vast majority of patients attending audiology clinics. In the UK National Health Service (NHS), older adults (over 55 years of age) represent 78% of adult audiology attendances and 87% of those fitted with hearing aids (2); and reported hearing problems appear more strongly associated with age than the degree of peripheral hearing loss, which suggests that processes other than peripheral hearing play a role (2, 3). For the purposes of this review we define “peripheral” changes as those restricted to the outer, middle and inner ear and auditory nerve. We define “central” changes as those within the central auditory nervous system, from the level of the cochlear nucleus up to the cortex, which includes elements of cognition. The aim of this review is to determine the relevant factors in auditory processing and cognition that might have implications for the way in which we set hearing aids, and to define the best approach to setting hearing aids for normally-ageing older adults based on the evidence in the literature. Whilst we do not discuss the relationship between hearing and dementia, it is likely that any recommendations regarding setting hearing aids for those with reduced degrees of cognition due to normal ageing will be particularly applicable to those with pathological cognitive decline.
We first provide a brief review of age-related change to central auditory processing and how this alters dependence on different elements of speech (Section 2). We then offer a short review of the basic concepts of cognitive change with age (Section 3), and how a decline in some elements of cognition also alters speech perception, particularly undermining listening in more challenging circumstances (Section 4). This understanding then enables us to determine how hearing aid parameters and fitting procedures might affect older adults’ listening ability. In Section 5, we derive a set of evidence-based principles for fitting hearing aids to older adults, and assess specific hearing aid parameters against these principles. We review evidence for an association between some elements of cognition and the benefit, or increased impairment, that may be caused by hearing aid processing strategies. We particularly focus on evidence that addresses the benefit, or harm, caused by fast or slow-acting compression speeds for those with different degrees of cognition. We also assess why the evidence may not always appear consistent. We then offer a discussion of how one might determine practical fitting guidelines for audiologists who predominantly see older adults in clinic (Section 6). Finally, we discuss gaps in the current evidence-base and what further research might be needed (Section 7).
The central auditory nervous system (referred to simply as the “auditory system” in subsequent text) is a uniquely complex sensory network, engaging peripheral sensory organs, multiple processing layers in the brainstem, the auditory cortex and wider cortex, culminating in conscious perception and understanding, as well as extensive “top-down” control. The processing of different aspects of speech is distributed across the auditory system. For example, some neurons are tuned to overall amplitude modulation from lower to higher levels of the system (4) and others are phase-locked to periodic patterns in the acoustic detail of speech (5, 6). Segregating and grouping sounds depends on multiple binaural processes from the cochlear nucleus up to the cortex, dependent on effective neural synchrony (7). Multiple pathways in the cortex, largely between temporal and frontal lobes, support a hierarchical process of word recognition, integration into phrases and sentences, syntax (grammar) and semantics (meaning) (8–10). Higher-level processes also depend on the relevance of sounds and lower-level processes can be enhanced via top-down control, enabling selective processing (11).
Age-related changes to the underlying neural infrastructure cause progressive “central” auditory deficits with increasing age (1, 12–17). As “lower” and “higher” level processes are interrelated, the effects of peripheral and central decline can be difficult to dissociate. Age-related cochlear damage generates a degraded input to the auditory system. The degraded nature of the input is exacerbated by the loss of synapses and auditory nerve fibers, often referred to as “hidden hearing loss.” This synaptic loss particularly affects fibers that are sensitive to louder sounds and may particularly impair speech-in-noise (SIN) perception (18–20). With increasing age there is a general reduction in the density of connections in the brainstem and cortical structures involved in auditory processing (14, 21). A deterioration in brainstem structures and the cortex impairs timing information, gap detection, localization, spectral processing and efferent control of the hair cells (22–25). These combined changes can undermine auditory scene analysis, impair an individual’s ability to attend to a target speaker (26) and reduce the ability to distinguish elements of speech in quiet (SIQ), non-familiar accents and SIN (27, 28).
Temporal information in speech cues can be differentiated across a number of frequency bands (29): information about the speech envelope (ENV) is carried primarily in the frequency band below 50Hz; information about voicing and periodicity primarily in the frequency band between 50 and 500Hz; and information about temporal fine structure (TFS) in the frequency band above 500Hz. ENV and TFS are the most important cues forming the basis of speech perception, frequency perception and localization (30). However, for those with sufficient hearing, ENV and TFS provide redundant cues that support processing of the speech signal (31). A difference in the pitch of voicing helps differentiate individuals in multi-talker situations (32). Vocoder experiments that replaced TFS with a carrier signal or noise, which was modulated by ENV, demonstrated that only a small number of frequency bands are required for successful speech perception in quiet even when TFS was lacking (33). However, the same was not true when speech was presented in a noisy background. Adding back TFS to a signal progressively improved SIN performance (34, 35). The ability to make use of ENV with little TFS can also be evidenced by the success of cochlear implants, which provide a grossly reduced representation of spectral detail (29, 36). Besides supporting speech perception in noise (36–38), TFS also supports binaural localization and separation of competing sounds (39), perception of pitch and music (29, 30) and perception of motion (40). Individuals with peripheral hearing impairment cannot make good use of TFS which can lead to difficulties hearing in noisy situations (41).
Comparisons between young and old listeners with matched normal hearing, intelligence and education suggest that speech perception declines with age, demonstrating a greater effect than can be predicted by hearing thresholds (3, 42–44). Monaural TFS sensitivity declines constantly throughout early-to-late adulthood (45), whereas monaural processing of ENV is less affected (46, 47). Binaural processing of both TFS and ENV declines with age, although declining TFS sensitivity has some association with the degree of hearing loss whereas the association between ENV sensitivity and hearing loss is weak (46, 47). Besides TFS, cognitive performance has also been found to predict speech perception. Although both TFS sensitivity and some cognitive domains decline with age, they do not appear to be directly related to each other once the effect of age is removed (48). TFS sensitivity was found to be a stronger factor than cognitive ability and ENV sensitivity in predicting speech perception in quiet for normal hearing listeners, and cognition the stronger factor for predicting performance in noise (3). Reduced SIN perception with increasing age can be more generally associated with reduced cognitive abilities (49), reduced coding accuracy and TFS sensitivity (50, 51), poorer binaural processing (52) and sound segregation (26). Sensitivity to periodicity perception also declines with age, affecting the perception of intonation (53), discrimination of voicing (54) and the emotional content of speech (55, 56). Some elements of speech perception are therefore specific to auditory processing, whereas others are dependent on domains of cognition not specific to auditory processing.
“Cognition” refers to the processes of acquiring, retaining and using knowledge (57) and has many constituent elements, including reasoning, memory, speed, knowledge, reading, writing, maths, sensory, and motor abilities (58). One can differentiate cognition from auditory processing because the latter is specific to the analysis of sound, whereas the former is not. Whilst the auditory system processes sound, cognitive processes develop meaning and understanding from the sound. However, it should be recognized that this is a simplistic description because of the inter-related nature of the systems which together deploy “bottom-up” and “top-down” processes. Some elements of cognition decline with age, but some remain stable or improve (59). Cognitive abilities can be separated into two basic domains (60, 61): “fluid intelligence,” characterized as the speed and ability to resolve problems in novel situations; and “crystallized intelligence” that is an accumulation and use of skills, knowledge and experience. Fluid intelligence generally peaks at about 20 years of age and declines at a consistent rate throughout adult life, whereas crystallized intelligence increases with age. As a result, an individual’s overall performance in psychometric tests of intelligence remains largely stable through most of adult life (60, 62). It should be noted that the range of ability between individuals also increases with age (21, 60, 63) so age alone is not a good measure of specific elements of cognitive function. Individuals of different ages may therefore perform equally well at a complex task, but may be using a different blend of cognitive abilities to complete the task based on the strength of different cognitive domains (64). Accordingly, an overall measure of “general cognition” is not a useful concept when considering a specific complex task, such as listening. Cognitive reserve, developed through education and other lifetime experiences, appears to provide some individuals with greater resilience against cognitive decline (65). This, and other genetic, environmental, health and lifestyle factors, will mediate any cognitive change with age (63) and an individual might further modify function via cognitive training and physical exercise (66). In short, it is important to determine the specific elements of cognition that relate to different tasks rather than make any assumption of homogeneity amongst an age group.
Several theories have been proposed to describe the process of decline in some cognitive abilities. The processing-speed theory (67) suggests that a decline in speed results in processes failing to complete in time to be useful, and that slower processes reduce the amount of available processing capacity. A reduction in processing capacity, caused either by a reduction in processing speed or by other processes of decline, could undermine episodic memory (recollection of personal experiences) and the initiation of more complex processes (68). Note that episodic memory declines more with age compared to semantic memory (recollection of facts) and procedural memory (unconscious performance of tasks) (59). A second theory suggests that there is a limit on the amount of processing resources (69). Based on this idea, Baddeley & Hitch (70) introduced the concept of “working memory” (WM) as a system that stores and processes information relevant to a current task. A third theory postulates that an age-related failure to filter out irrelevant information is the cause for WM to become “cluttered,” thus reducing available capacity (71). Each of the individual theories cannot explain all of the features of cognitive decline and it is likely that there are multiple processes with cumulative effects (59).
One way to categorize the basic concepts of fluid and crystallized intelligence into further subtypes of cognition, particularly useful in relating specific processes to speech perception, is provided by the Cattell-Horn-Carroll model (72, 73) (Figure 1). The model describes a three-level hierarchy of cognitive abilities: general ability, broad domains and narrow domains. Broad domains are basic characteristics that manage a range of behaviors. Narrow domains are highly specialized and may be specific to certain types of task. Auditory processing (Ga) is included as a broad domain in the CHC model (Figure 1). Some elements of overall sound processing and perception are specific to Ga, whereas some elements rely on other narrow domains. Note that the generalized concept of fluid intelligence is not specifically shown in the model, but includes most of the broad domains of the model, i.e., those other than crystallized intelligence (Gc). Nevertheless, in general terms, abilities in the wide domain of fluid intelligence are those that tend to decline with increasing age.
Figure 1. A partial representation of the Cattell-Horn-Carroll (CHC) theory of cognitive abilities showing general intelligence (g) consisting of broad domains of cognitive function, under which there are narrow domains that can be assessed with specific tests. Figure developed from (72, 73). Note that there are a greater number of broad domains and the narrow domains shown are only selected examples of those in each broad domain (24).
Note that ongoing refinements to the model introduce further broad and narrow domains beyond those shown here (72). Episodic memory is contained within the definition of learning/encoding efficiency (Gl) in the CHC model (Figure 1) (73). Executive function is not commonly included in the Cattell-Horn-Carroll model and there is no clear consensus on its definition (73). It may be thought of as a cognitive “control” function that mediates narrow domain abilities, although its three core elements may be considered as separate domains that: enable “shifting” between tasks; monitoring tasks and “updating” WM as necessary; and “inhibiting” other tasks or automated responses in order to complete a task (74).
In this section we focus on the elements of cognition that may underlie impaired listening ability. Not enough is currently known about how specific narrow domains of cognitive ability (Figure 1) relate to auditory performance in specific listening situations. However we do know that WM often plays a role and is frequently associated with SIN performance (75, 76), understanding fast speech (77) or with general auditory performance across a range of tasks (78, 79). WM is more predictive of SIN performance in older age groups compared to younger groups (80) or in more challenging conditions, such as a lower signal-to-noise ratio (81). Older adults with greater WM resources may be better able to adapt to difficult listening situations (78, 82). WM is often used as a broad term and encompasses both storage and processing-dependent WM tasks. These different types of tasks can have different associations to different types of listening situations (75, 83–85). Executive function is another cognitive factor that is often associated with listening and the degree of listening effort (86). Inhibition, which a sub-domain of executive function (Figure 1), and processing speed are also cognitive functions associated with reduced auditory performance (87–89). Different domains of cognition appear to be more strongly predictive of listening ability for more difficult listening tasks (90, 91). A meta-analysis (92) provided an overview over various cognitive functions and their association with different SIN tasks. It showed that SIN performance correlated with measures of processing speed (r = 0.39), inhibition (r = 0.34), WM (r = 0.28), and episodic memory (r = 0.26). These are all narrow cognitive domains that deteriorate throughout adulthood (62, 67, 68, 71, 93) and their effects may be, in part, additive.
One consequence of the changes to hearing and cognition may be an increase in effort when listening (94, 95). Listening effort is defined in the Framework for Understanding Effortful Listening (FUEL) (96) as an allocation of cognitive resources to overcome listening obstacles. It is a finite resource and its capacity will be expended at different rates dependent on the inherent difficulty of a task and an individual’s motivation to overcome obstacles in listening. Listening effort likely engages multiple neurological systems (97) and is an important factor in speech perception. It should be considered in the context of age-related cognitive decline because it may also affect the motivation of individuals to comply with treatment or engage socially (98, 99). In addition, hearing aids have the potential to reduce, or increase, listening effort (100–104).
The relative importance of hearing loss, auditory processing and cognitive function for predicting overall auditory performance will depend on the specific listening situations encountered (76), which will vary in real-life (105). Different forms of SIN test engage different narrow cognitive domains (92). SIN tasks can be distinguished according to the target and masker signal, and both of these affect the type of processing needed for successful listening (92). The type of masking noise has a considerable effect; individuals perform better in steady-state noise compared to multi-talker babble and the latter yields stronger associations with cognitive function (85, 92, 106–108). An intelligible masking signal provides both energetic and informational masking, and is most likely to divide the attention of a listener, offering the greatest cognitive challenge (75), although other cues such as the different gender or fundamental frequency of the signal and noise source can overcome some effects (109).
A number of speech models have attempted to conceptualize the role of different cognitive functions within the pathway of speech understanding. Gordon-Salant et al. (21) adapted a theoretical model (110) to describe a bottom-up process of hierarchical signal processing. The “sensory system” undertakes spectral and temporal processing to discriminate between sound sources. This is further refined by the “perceptual system,” employing inhibition to direct attention, and the “cognitive system” that analyses and identifies words, and develops meaning, feeding back to lower levels of the system, requiring processing speed, WM capacity and semantic knowledge, also engaging long-term memory (Glr). The model by Bronkhorst (11) is broadly similar in principle and highlights the role of attention control, an element of executive function, in which attention is triggered by certain signal characteristics, that then engenders selective processing at lower levels, or “pre-attentive” stages, of the auditory system. The Ease of Language Understanding (ELU) model (111, 112) largely focusses on situations in which there is conflict between an input signal and lexical information stored in memory, e.g., due to an unusual accent, which then requires engagement of a feedback loop to resolve it, particularly requiring WM, executive function and learning/encoding efficiency. Taken as a whole, these models highlight the critical dependence of listening on certain specific narrow cognitive domains. However, given that research studies cannot assess all real-life listening situations, one cannot conclude that these are the only cognitive domains of relevance, but that they represent those most commonly measured in the context of the limited listening tasks employed in studies, and there may well be other confounding factors such as alternative cognitive strategies that individuals use to compensate (64). The models also highlight that poor fidelity of the input signal (via hearing loss or inappropriate hearing aid processing) can cause greater conflict in resolving speech and, ultimately, a failure to do so. Overall, the models support the finding that speech intelligibility is associated with processing speed, inhibition, WM and long-term storage and retrieval (92) and will consequently decline with age as these narrow cognitive domains deteriorate. It should also be pointed out that a decline in some cognitive domains will not only undermine speech intelligibility, but may also impair the perception of speech quality (113), even where intelligibility is unaffected (114), and undermine an individual’s perception of aided speech.
In summary, certain narrow cognitive domains (Figure 1) will be associated with different types of auditory task. A wide array of cognitive tasks are employed across different studies (92), so the presence and strength of associations between cognition and auditory performance will depend on the narrow cognition domains assessed and the task used to assess them, as well as the type of auditory task, its level of difficulty, the type of stimulus, noise and other cues used (64, 115), the degree of context, vocabulary and visual cues (116–118). Furthermore, there is an association between hearing loss and cognitive decline (119) that may act as a confound in studies. Sensory impairment can affect an individual’s performance on cognitive tests, often requiring recall of spoken words or text-based tests, dependent on hearing and vision, so that it is possible that this causes falsely enhanced associations (61, 120, 121). It may therefore be considered unsurprising that the outcomes of research studies are not wholly consistent because they do not employ consistent paradigms.
The preceding sections have summarized the effects of ageing on peripheral hearing, auditory processing and cognition. It is now important to determine which of these factors are relevant to hearing aid fitting and how some signal processing strategies may create benefit or impediment for individuals with differing degrees of cognition and auditory processing. The “optimum” hearing aid fitting should not solely consider peripheral hearing loss, but should aim to deliver maximum benefit over time, considering hearing loss, auditory processing, cognition and non-auditory factors that affect an individual, their perception of treatment and ability or intention to comply with it. For example, no amount of fine-tuning of a hearing aid’s gain will deliver benefit if there is a perception of unacceptable distortion or if individuals feel unable or unwilling to use their hearing aids. As a result, too many hearing aids remain under-utilized or unused (122). Selection of hearing aid parameters must take all of these factors into account.
Hearing loss degrades the fidelity of the input to the auditory system, undermining its ability to take advantage of temporal and spectral cues and requiring additional effort to resolve mismatches. It is unreasonable to expect that hearing aids can fully restore this input (123). Hearing aids can only address some of the loss in peripheral sensitivity. They cannot directly improve elements of auditory processing or cognition, but they may enhance the signal-to-noise ratio or suppress noise that might distract from the signal or increase listening effort. However, some hearing aid settings may significantly degrade the input signal in ways that hamper auditory processing, undermine speech perception or listening comfort, and increase listening effort (124, 125). The primary role of audiologists should be to address the concerns of, and provide benefit to, the individual patient, so it is important that the patient is given the opportunity for input and that these signal enhancement principles are understood and alternative settings considered (126). In general, hearing aid users with greater cognitive ability appear to benefit more from hearing aids (127). It is therefore important to derive some clear evidence-based principles for hearing aid settings for older adults. Accordingly, for those older adults with reduced auditory processing and cognitive abilities in relevant narrow domains, we can state the following principles based on the preceding review:
1. Older adults will generally be more dependent than younger adults on ENV for speech perception, which is less impaired than TFS with increasing age for monaural listening (46, 47, 128). Older adults will therefore be more susceptible to ENV distortion (124, 129). A primary aim of hearing aid fitting should therefore be to ensure that speech is audible but with minimal distortion to ENV (130).
2. Given that a reduced temporal accuracy with age undermines gap detection (23, 131), quiet gaps between elements of speech should be maintained.
3. Binaural processing of TFS and ENV is degraded with age (46, 47, 52) and impairs interaural level difference (ILD) and interaural time difference (ITD) cues (23, 132). Use of bilateral hearing aids should not further disrupt theses cues, e.g., via fast gain changes that particularly diminish the aided level difference.
4. Reduced binaural processing makes older adults more susceptible to binaural interference (132, 133). There is evidence that some individuals may perform better in noisy situations with a unilateral aid (134) although this is not consistent across studies (135). Unilateral aiding should be considered where there is any indication of binaural interference (136). There should not be a presumption that bilateral aiding is best in every case.
5. General impairment of SIN performance with age (21, 27, 49) indicates a need to improve the signal-to-noise ratio through the use of directionality.
6. Introducing distortion to ENV may increase listening effort and diminish the capacity for listening (100–103). Hearing aid processing should aim to minimize cognitive load and listening effort (86, 96) by avoiding unnecessary distortion to the speech signal, loudness discomfort or other perceptual sound difficulties. Noise reduction settings should be considered (104).
These principles should not be regarded as novel in any way. Directionality has been employed for many years in analogue and digital hearing aids. However, since the inception of digital hearing aids it has been suggested that audiologists should pay greater attention to the distortional aspects of hearing aid processing, rather than solely considering the amplification needed to correct peripheral hearing loss (137, 138). This has also previously been applied specifically to hearing aid fittings for older adults (130). It has long been recognized that hearing aid settings, the associated aided speech recognition and overall satisfaction will be, in part, dependent on cognitive ability (139–141) and that different hearing aid processing strategies will have different advantages and disadvantages for individuals with varying degrees of cognitive function (96). The following sections will consider various hearing aid parameters, how they affect the principles above, and the effect of cognitive change on the fitting process.
Before doing so, it is worth considering more carefully what is meant by “distortion.” In simple terms, distortion can be defined as any non-linear change from the original signal, although the inherent purpose of hearing aids is to modify sound. Multiple signal processing strategies alter speech signals in many ways and it is not a straightforward exercise to measure distortion across its different forms (142), and even less so to equate any measure of disruptive distortion to the perception of a hearing aid user. For example, if one were to measure the distortion introduced by compression, one is immediately faced with the issue of how to combine a measure of change to ENV and a measure of change to TFS, and whether these have detrimental effects after processing in the auditory system. Korhonen et al. (143) suggested a method of assessing modulation in frequency bands, but this only evaluates changes to ENV. Hearing aids introduce multiple sources of distortion and widely-available test box measures, such as total harmonic distortion, are unrepresentative of the overall distorting effects of a hearing aid on the speech signal. The loss of differentiation between the modulation of the speech signal and that of a competing talker or background noise when they are compressed together in a hearing aid is known as “cross-modulation.” This undermines the auditory system’s ability to separate sounds because shared modulation will be interpreted as originating from a single source (144), and can be considered as a loss of information from the original signal. There is more recent evidence that, for complex speech signals, the auditory system uses the interaction between modulations in the frequency and time domains, known as “spectrotemporal modulation,” which is an important determinant in speech intelligibility. Spectrotemporal modulation is undermined by a combination of reduced frequency-tuning and TFS sensitivity (145, 146), although it is unclear how it is distorted by hearing aid processing (147).
There have been numerous initiatives that have attempted to quantify overall distortion and relate it to speech intelligibility and perceived quality; see Kates and Arehart (148) for a summary. Perhaps most promising are the Hearing Aid Speech Perception Index (HASPI) and the Hearing Aid Speech Quality Index (HASQI) (148). These are neural-network models incorporating assumptions of peripheral and central auditory processes, then fit to measurement data for hearing impaired and normal-hearing listeners. The models offer clear differentiation in quality and intelligibility between hearing aids from different manufacturers and between different hearing aid settings (149). It is therefore possible that HASPI and HASQI could become clinical tools that can be used to evaluate distortion. However, to be most useful they would need to be validated against different patient groups because optimal settings are likely dependent on an individual’s cognition or other factors. Moreover, even after validation, there may remain considerable variation between individual preferences. Likewise, it is unclear what trade-off there should be between intelligibility and quality scores, or how this would be determined on an individual basis.
Reducing the dynamic range of sound to match that of a hearing-impaired person, i.e., compression, is a fundamental feature of digital hearing aids. Hearing aids filter sound into frequency bands, or frequency channels, such that different degrees of compression, and other types of processing, can be differently applied within each channel. Wide-dynamic range compression (WDRC) with short time constants, or fast-acting compression (FAC), was employed to enable exaggeration of quieter syllables or phonemes within words by quickly applying greater gain, so is often referred to as “syllabic compression.” This type of compression is based on the fact that some elements of speech may be inaccessible to a hearing-impaired listener, typically high-pitched fricatives for those with a high-frequency hearing loss. FAC aims to restore normal loudness perception across the frequency range (150). Hearing aids with slow-acting compression (SAC) enhance high-frequency sounds solely via the application of different amounts of gain in each frequency channel. In practice, most systems employ similar attack times (1–10 ms), which was to protect patients from sudden loud sounds (150), so “fast” and “slow” hearing aids are largely differentiated by the release times (151). FAC is usually characterized by attack times of 0.5–20 ms and release times of 5–200 ms, whereas the release times for SAC are typically between 500 ms and 2 s (150). The instantaneous gain for any input level and frequency will accordingly be determined by both the gain settings for quiet and loud input levels (i.e., that which should be applied for a long-duration sound) and the compression speed (i.e., whether the aid reacts fast enough to reach the gain setting). It should be recognized that simply comparing fast and slow compression speeds is a simplification. There are various approaches to the implementation of compression speeds in hearing aids that may be applied differently by frequency channel, by direction of change in sound intensity (150), or adaptive systems that change compression speed by acoustic situation (152–157). Furthermore, fast-acting impulse noise protection may be employed as a separate system to WDRC, although the details of these approaches are usually proprietary (158).
FAC can be interpreted as contravening many of the principles suggested above for individuals with reduced degrees of cognitive function. In general terms, FAC has the effect of “flattening” ENV, whereas ENV is preserved by SAC (Figure 2) (150, 159). FAC may therefore impair speech perception by changing the speech signal in a number of ways: distorting speech envelope cues; amplifying the noise in gaps, undermining gap detection; and reducing modulation detection that enables separation of individual speakers (150, 160–166). FAC might also impair sound localization based on interaural differences, although the evidence for this is weak (167). FAC also impairs localization of sounds in reverberant conditions (168). Even in quiet situations, increasing the number of frequency channels, as is typically deployed in almost all hearing aids, reduces the spectral contrast of vowels and associated speech intelligibility with FAC (159, 169). Proponents of FAC contended that listeners cannot be aware of contrasts in amplitude if a signal is presented below the threshold of the cochlea at a particular frequency (170). However, this argument was largely based on evidence from two-channel systems. In modern multi-channel hearing aids, increasing the number of channels increases the amount of distortion when using FAC (159). Hearing aid users prefer fewer channels when FAC is used, whereas the number of channels does not make a difference with SAC (159). In principle, FAC should only be employed to make weak phonemes audible in quiet conditions for those with mild-to-moderate degrees of hearing loss (171) and does not offer benefit in noisy situations (151, 172). Overall, those with greater degrees of hearing loss tend to benefit less from FAC (173). In truth, to make a proper comparison between FAC and SAC, one should consider the speech signal as presented to the auditory system after processing via the cochlea. As we are unable to do this in humans, we must seek to determine objective or subjective measures of performance from the patient population, such as performance in speech intelligibility tests or self-reported benefit and satisfaction. Other evidence can be sought from animal models in which neural responses can be measured with hearing aid-processed sound (174), suggesting that FAC acts to undermine spectral and temporal contrasts, leading to a failure to restore consonant identification in quiet. Computational models of the auditory system might also be used to assess the effects of different hearing aid processing strategies. These suggest that slow compression leads to a greater restoration of the neural representation of speech than fast compression (175). The HASPI-HASQI model, discussed above, can be employed to compare intelligibility and quality in different scenarios, although it has not been specifically used to compare compression speeds. However, it does suggest that increased processing complexity does not inherently provide better performance (149).
Figure 2. The effect of compression speed on speech envelope cues, reproduced with permission from Holube et al. (159). The original speech signal (top left) is processed through a 16-channel system with an exaggerated compression ratio of 8:1 using various release times (τrel) from long (1,400 ms, slow-acting compression) to short (15 ms, fast-acting compression).
There are a considerable number of studies that compare the outcomes of using hearing aids with FAC and SAC and relate it to individuals’ cognitive scores. A seminal set of studies by Gatehouse et al. (141, 172, 176) compared five linear and non-linear fitting strategies using FAC and SAC, with a crossover design, for older adults with mild-to-moderate sensorineural hearing loss. Three non-linear strategies were employed: slow-slow, using slow-acting release times across low and high frequency bands respectively; fast-slow, a hybrid strategy using fast release times for low frequencies (<1,500 Hz) and slow times for high frequencies; fast-fast, using fast release times in both bands. Speech intelligibility was measured in several conditions, altering the speech presentation level and signal-to-noise ratio. Non-linear hearing aid strategies provided better aided speech recognition than linear approaches, with FAC offering greater benefit than SAC, but the degree of benefit declined with increasing speech presentation level or reduced signal-to-noise ratio. The slow-slow paradigm offered significantly greater listening comfort, and slow-slow and fast-slow were significantly preferred by users. Conversely, fast-fast and fast-slow paradigms had significantly better speech intelligibility, both user-reported and measured. However, whilst the level of a subject’s cognition had no influence on speech tests scores when using SAC (slow-slow or fast-slow), fast-fast compression generated a significant negative correlation between cognition and speech perception, resulting in a wider range of benefit and impediment to patients. In summary, the benefits of FAC over SAC were only accessible to those with better cognitive scores, able to take advantage of increased audibility at the cost of reduced “temporal contrasts,” whereas SAC offered greater benefit than FAC for those with lower cognitive scores. It is notable that these studies used hearing aids with only two channels so, based on the discussion above, one might speculate that the difference observed may have been greater with multichannel hearing aids. The study was replicated with much the same result, further demonstrating that the association between SIN performance and cognitive test scores was stronger when more demanding listening tasks were used (106). Hearing loss was the stronger predictor of SIN performance, relative to WM, when SAC was employed; whereas WM was a stronger predictor than hearing loss when using FAC. Other studies have found WM to predict SIN performance in difficult situations when FAC was applied (177–179), concluding that FAC created a disadvantage relative to SAC for those with lower WM (125). Likewise, stronger preference for SAC relative to FAC, when listening to speech and music, can be associated with individuals with lower TFS sensitivity (180). Hearing aid users with poor TFS sensitivity are also affected more by ENV distortion (129). There is also some interaction between different elements of hearing aid processing. For example, adults may prefer SAC when mild noise reduction is employed, but FAC when strong noise reduction was applied, although the effect sizes were small (181). Conversely, a number of similar studies have found that the relationship between compression speed and SIN performance was not affected by the variation in cognitive scores (177, 182–188). One study suggested that FAC offers greater benefit than SAC in quiet and noisy situations for all users (189), irrespective of cognitive scores, although this showed linear amplification to be better than FAC or SAC, and significant differences were only seen at lower presentation levels. In any case, the researchers went on to suggest adaptive compression speeds that utilized SAC in noisy environments (155).
It is difficult to compare studies because of the different paradigms used for testing speech and cognition, as discussed in Section 4. This is further confounded by variable application of algorithms, the number of channels employed in commercial and research hearing aids, and situations that may not be representative of a user’s daily experience (190). Studies also vary widely in the amount of acclimatization allowed for research participants, if any, and results vary between new and experienced hearing aid users (107, 191). Complete consistency can therefore hardly be expected between studies and a systematic review found it difficult to draw clear lessons (192). On balance, the studies suggest that individuals with lower degrees of cognition will fare worse with FAC, compared to SAC, in some listening situations that are challenging. Those with high cognitive scores will derive benefit from FAC, compared to SAC, in quiet situations. Audiologists must consider audibility, distortion to ENV and listening comfort when setting a hearing aid, amongst other things. Furthermore, the preceding discussion largely addressed only objective benefit, i.e., aided speech intelligibility, in various situations, but not the perceptions of users that will affect outcomes, not least in mediating compliance with treatment. A number of studies show that users generally prefer SAC in noisy, or all, situations (107, 141, 184, 193) or that different individuals have different preferences, with a greater average preference towards SAC (180).
The compression ratio defines the range of gain applied within a hearing aid channel. A higher compression ratio increases the range of input levels that, after amplification, are audible without being uncomfortably loud and should improve speech intelligibility in quiet, but will reduce intelligibility in background noise (151, 171). The effective compression ratio is dependent on the compression speed. In simple terms, SAC reacts too slowly to reach the highest levels of gain determined by the compression ratio within a short timeframe, such as a word, so the distorting effect on ENV for that word is smaller than FAC (Figure 2), where gain may change within the full range determined by the compression ratio during each word spoken. Consequently, the same compression ratio cannot be set for FAC and SAC systems. A compression ratio of 3.0 or less will have little effect on speech envelope cues for SAC (194). However, the amount of distortion introduced by FAC will be broadly proportional to the compression ratio and it is generally recommended that it is not set greater than 3.0 (195) or 5.0 (151, 152). Distortion will be further exaggerated by increasing the number of frequency channels (159). Increasing the compression ratio can reduce consonant recognition (196) and overall speech recognition (197). When asked to subjectively rate the quality of speech, listeners tend to prefer lower compression ratios in quiet and even more so (CR ≤ 2.0) in noise (159, 197, 198). It should also be noted that that the compression ratio is measured with steady-state signals and the effective compression for a fluctuating signal like speech is lower (199); hence, the longer the release time, the lower the effective compression ratio (151, 152). A greater compression ratio can therefore be set for SAC without noticeable distortion, and the additional comfort availed can be interpreted as a benefit of SAC (150). In order to avoid distortion to ENV for older adults, compression ratio must be reduced significantly if FAC is used.
Noise reduction algorithms aim to reduce the level of non-speech sounds and increase comfort. A meta-analysis found that noise reduction does not consistently improve speech intelligibility, but is moderately beneficial for sound quality and comfort (200). Noise reduction can be fast or slow-acting and can cause disruption to the speech signal by alterations to gain in specific frequency channels, and this may be differently tolerated by those with different degrees of cognition. As noted above, the strength of noise reduction employed interacts with the preference for FAC or SAC (181). Nevertheless, those with relatively good cognitive scores benefit from stronger noise reduction, whereas it may be detrimental to those with lower scores (125). Background noise impairs WM function, making it harder to recall words, especially for more complex listening challenges. Noise reduction may overcome this problem by reducing the demands on WM, but may mainly apply to those with higher degrees of WM in more challenging tasks (201). Some studies suggest that it may apply to those with lower degrees of WM only in less challenging tasks (202, 203). Strong noise reduction likely impairs speech intelligibility for those with low WM or executive function (204, 205), although moderate noise reduction is preferred in most situations and some users prefer strong noise reduction despite the loss in intelligibility (206). Overall, moderate noise reduction likely improves listening comfort without undermining speech intelligibility in most cases, although there is some variability in individual preference.
Hearing aids, by their nature, alter a sound signal and inherently introduce some form of distortion relative to the original. Other features manipulate the sound, including frequency-dependent gain, directional microphones, feedback management, frequency lowering, expansion and wind-noise reduction. Any hearing aid processing that distorts ENV may impair speech perception and this may be especially detrimental to those with lower degrees of cognition (207). However, there is less evidence relating the benefits or drawbacks of these features in relation to cognition.
There is some evidence that frequency-lowering, or frequency compression, can benefit those with higher WM scores but it might act to undermine intelligibility in those with lower WM scores (124). A meta-analysis of frequency-lowering suggested that it has a small benefit in quiet situations, although results were inconsistent and situation-dependent (208), and it may impair speech perception in noise (209). However, frequency lowering might be beneficial in noise for younger adults or those with steeply-sloping hearing loss (103). Frequency-lowering might be trialed as an option, but may impair listening for those with lower cognitive scores.
One dimension of hearing aid setting that has well-established benefits and consistently positive outcomes is the use of directionality to improve signal-to-noise ratio (206) so it is not considered in any detail here. Whilst directionality applies gain preferentially to sounds based on direction, it does not inherently distort the sound in the same way as non-linear processing schemes. There is a similar benefit from directionality for any compression speed irrespective of the degree of WM function (210).
This review is focused on hearing aid parameters and their effect on aided outcomes for older adults with cognitive change related to normal ageing. Whilst not a subject of this review, we should be cognizant of the fact that hearing care for older adults must include a wider appreciation of their needs including physical and listening comfort, psychological, social, behavioral and environmental considerations, and these have implications on hearing aid settings, acceptance and outcomes (211). For example, despite a declining ability to make use of multi-sensory inputs (212), older adults still benefit from visual inputs for speech perception (118) so visual deficits should be corrected. Education of families and careers should incorporate some understanding of auditory decline and enable them to make adjustments, e.g., via avoidance of fast speech (212), use of clear speech, providing cues before speaking (109), maximizing context (213), familiarity (214) and environmental adjustments to reduce background noise. Other non-auditory factors associated with the comorbidities of age and reduced cognition that can inhibit successful use of hearing aids include dexterity, self-efficacy, attitude, motivation, family support and self-image (98, 99, 215, 216) as well as the “simple” or “complex” manner in which a hearing aid is configured (e.g., with or without programs or control buttons). The cognitive status of individuals will also affect clinical interactions because higher degrees of fluid intelligence enable them to engage more with life (217), handle problems such as acclimatizing to a hearing aid, and to be more proactive in taking action to address their health (218). In short, a hearing aid fitting must provide the patient with a solution that they find manageable and acceptable and, as seen in much of the research discussed above, patients’ preferences are not always aligned with the solution that gives maximum speech intelligibility. In summary, whilst we attempt to determine the best approach to setting hearing aid parameters in this review, we recognize that these recommendations should be interpreted within the broader scope of an individual’s needs.
The preceding sections of this paper have reviewed the literature to determine how age-related changes to cognitive domains and auditory processing alter speech perception and, in particular, an individual’s relative dependence on different speech cues that might be disrupted by different approaches to hearing aid processing. In this section, we aim to apply the evidence to the clinical hearing aid fitting process in order to provide pragmatic guidance to clinicians.
As part of a patient-centered care model (219) the audiologist’s primary role is to offer support and provide benefit to each individual, where the total benefit can be considered as a combination of objective and perceived benefit, preference, and ability to comply with treatment. This perspective means that the “optimal hearing aid fitting” must therefore address all of these aspects of care. In consequence, audiologists require a broad skill set that includes counselling (219) and some level of technical understanding. However, some relevant technical factors, not least compression speed, are rarely provided by hearing aid manufacturers in specification sheets, nor is it easy in our experience for audiologists to acquire such information. This is a fundamental concern because compression speed and compression ratio are primary determinants for the amount of distortion introduced to ENV. Given that audiologists are, in part, dealing with the hearing aid as a “black box,” and that research findings are not always consistent, it is perhaps unsurprising that there are no clear technical guidelines for fitting hearing aids to older adults (220).
However, the research is broadly consistent in a number of ways. First, older adults are more likely dependent on ENV for understanding speech. FAC causes distortion to ENV that can undermine speech perception, whilst emphasizing elements of TFS that cannot be utilized by many older hearing aid users, and might disrupt binaural inputs. Second, hearing aid users tend to prefer SAC on average, although individuals have different preferences. FAC is likely better in quiet for those with good cognitive processes related to hearing, but likely degrades speech intelligibility and the acceptability of hearing aids for those with lower degrees of cognition. Third, the variation in cognitive processes between individuals increases with age, so we cannot know which specific settings offer the most benefit for an individual, nor whether objective benefit will be aligned with their preference.
The combination of peripheral and central auditory decline therefore means that older adults represent some of the most complex patients, as well as the most numerous. Consequently, age-related hearing loss should never be treated as “standard care” simply because of its high prevalence. Determining the correct hearing aid strategy for an individual hearing aid user is therefore a major challenge. One might think that it would be useful to undertake tests of cognition or TFS sensitivity in clinic. However, it is uncertain that a test score could usefully indicate a specific course of action, nor which cognitive tests should be used. Verbally-delivered cognitive tests are affected by hearing loss and there is no well-established test that has been shown to be unaffected by sensory impairment (61, 120, 221). It is unclear whether audiologists are able to conduct cognitive tests sufficiently well and their utility in clinical situations needs evidencing (222). Speech testing might give some indication of overall ability and it has been suggested that it be more widely used (223) because standard audiometry provides no useful evidence regarding auditory processing and cognition. However, it is equally unlikely that a speech test score could equate directly to a specific hearing aid setting and user preference. It is also unclear whether clinical speech-testing is sufficiently sensitive to determine any benefit derived from alternative hearing aid settings. Consequently, in addition to the current lack of applicable tools, it is debatable whether the additional loading on clinical time would yield sufficient benefit to be justified. In any case, many audiology services face demand and resource pressures, particularly in public sector systems with universal treatment, so extending the test battery significantly may not be implementable.
We therefore propose a pragmatic approach to hearing aid setting that could be implemented within typical current fitting appointments. The principles above can be employed to ensure that relevant factors are considered for older adults, which we define nominally as those over 55 years of age in line with the English NHS definition of age-related hearing loss. We believe the following statements concerning hearing aid settings for older adults follow directly from the evidence:
1. All factors that introduce distortion to ENV should be explicitly considered in hearing aid fittings. This should primarily include compression speed and compression ratio, but also noise reduction, frequency lowering and other advanced features.
2. Compression speed and compression ratio are primary determinants of the degree of distortion introduced to ENV. This indicates a need for hearing aid manufacturers to publish compression speeds as part of their standard product information, and provide products that allow clinicians to change compression speed, or offer adaptive compression speed algorithms that can be selected appropriately for each individual.
3. SAC should be considered as the default setting for older adults and should be employed in noisy environments for all hearing aid users. However, recognizing the variation in preference between individuals, both SAC and FAC approaches can be provided in separate hearing aid programs and the audiologist can employ validation techniques to assess which is best set as the default program. There is no good reason that hearing aids should default to FAC for older adults.
4. If FAC is used, the compression ratio should be reduced to levels that avoid ENV distortion. There is no clear evidence that suggests a specific value, so it is difficult to provide robust guidance. Our own clinical experience and discussions with hearing aid manufacturers suggests a value as low as 1.5. This is, admittedly, anecdotal but there is some evidence to suggest that linear aids are preferred over WDRC aids with FAC and a compression ratio of 2.0 when subjectively rating the quality of aided speech (159). Users also prefer CR ≤ 2.0 in noise (197, 198).
5. Directionality is always beneficial to improve the signal-to-noise ratio. This might also include a consideration of ear-molds in place of open-fittings, because the latter reduces directional benefit (224, 225). Alternatively, other assistive devices and accessories may be considered to improve directionality or enhance the signal-to-noise ratio.
6. Noise reduction is likely best set to moderate, although this depends on each hearing aid manufacturer’s approach and the evidence base is not strong. Strong noise reduction may well impair speech perception, especially for those with lower degrees of cognition. However, the user may be offered options or multiple programs because some may prefer stronger noise reduction in some situations.
7. Other advanced hearing aid features, such as frequency lowering, may also distort the signal in ways that further impair speech intelligibility for those with reduced abilities in some cognitive domains. The evidence is generally weak, so these should be considered as options for each individual.
8. Unilateral aiding should be considered where binaural interference is suspected. This is easily achieved in clinic by comparisons of speech intelligibility and listening comfort with bilateral hearing aids versus the left and right hearing aid working unilaterally. In some cases, clinicians may find that the individual’s speech intelligibility is significantly better when aiding only one of the ears, and intelligibility may be impaired or the sound distorted whenever gain is applied to the other ear.
One should recognize that any recommendation regarding hearing aid parameters cannot be prescriptive given the current state of knowledge and considering the wide variability in individual needs. This implies that there must be some process of ensuring that hearing aid settings are optimal, or at least acceptable, for each individual. Overall, we believe that a full consideration of hearing aid parameters needs to be combined with the over-riding principles of patient centered care. This engenders a need to balance the key elements of the hearing aid fitting process, including verification, validation and counselling. “Verification” is the process of matching hearing aid gain to a prescribed target using real ear measurements (REMs). “Validation” should aim to provide evidence that all hearing aid settings are suitable for an individual. This may include simple tests of loudness discomfort and speech intelligibility, more advanced techniques such as speech mapping, paired comparison approaches (226), or questionnaires and self-reporting tools (227). Finally, “counselling” addresses non-audiometric factors such as the patient’s expectations, self-image and self-efficacy (228). This approach will likely improve the rate of treatment compliance (229, 230). The following statements are not novel, but we believe they are worth re-stating in light of the principles discussed above for setting hearing aids for older adults:
9. Conducting verification and matching gain to a prescription target does not, in itself, make a good hearing aid fitting. The verification process is solely aimed at setting individualized hearing aid gain and does not encourage a consideration of the other parameters highlighted in this review. For example, setting the gain to a prescription target for 50, 65, and 80 dB input levels may lead to high compression ratios where FAC is used, causing inappropriate ENV distortion. Verification is an important step that may offer a large (231) or small (232) benefit, but the resulting gain settings must be considered in the light of the other parameters discussed above and adjusted appropriately.
10. The only clinical process that can evaluate overall hearing aid settings and their suitability for an individual is validation (233). Accordingly, we believe that it is important that verification is always followed by proper validation in clinic (234) and that clinicians should consider the balance of time given to each process during fitting appointments. The hearing aid user should not perceive distortion and loudness discomfort, and sufficient speech intelligibility and sound acceptability should be demonstrated. In short, clinicians should consider both the intelligibility and quality of speech (235). However, it is recognized that current validation techniques, e.g., using live voice or speech mapping, are subjective and more objective methods of assessing the likely perception of overall distortion would be helpful. Appropriate objective methods are currently lacking in clinical practice.
11. Hearing aids cannot overcome all of the peripheral and central auditory deficits discussed in this review, so wider considerations should be addressed to achieve the optimum outcome in the perception of the individual user. Audiologists must, of course, consider other non-auditory factors. Informational counselling regarding central auditory decline should aim to set reasonable expectations for the hearing aid user and their families or careers (236, 237) and can promote modification of the environment and behaviors related to the elements of decline in central auditory processing discussed above. This may include use of clear speech, visibility of mouth movements and reducing background noise.
Research studies use a wide array of both cognitive and listening tasks, so the associations observed between them are variable. Although WM tasks predominate in many studies, it is not the only domain of cognition that is relevant (92) and we are yet to fully understand which elements of cognition might relate to every listening condition (64). It would seem prudent to consolidate a range of cognitive tests in research studies. There is also a lack of validated cognitive tests for those with a sensory impairment, because many tasks are delivered verbally or visually, so hearing loss may be a confounding factor (61, 120, 121) and must be considered as part of research design. In large part, studies relate cognitive status to performance in listening tasks, although some studies relate it to user preference. It should be clear that individual preferences are not always aligned to objective performance and may be based more on listening comfort, effort or some other perception of quality, so it would seem sensible to measure both objective performance and subjective benefit in research studies.
Whilst there is variation in the evidence, it appears to us that the balance of evidence suggests that slow-acting compression may be the best default position for most older adults along with other cautious settings for compression ratios and noise reduction, although accepting there will be individual variations. However, further research should seek to confirm the size of the effect. Furthermore, most research on the effect of hearing aid settings considers one parameter in isolation whilst other parameters are held constant. This is unlikely to reflect the overall function of commercial hearing aids and assess the interaction between different hearing aid features. Recent work has demonstrated the interactions between compression speed and other settings, such as noise reduction and directionality (181, 210), so further research should establish whether combinations of hearing aid processing strategies negate the overall effect of individual settings in the user’s perception. It is also important to be able to evaluate balanced measures of speech intelligibility and quality, and relate them to the overall perception of distortion. This indicates a need to further develop clinical tools, such as HASQI and HASPI (148, 149), to determine an objective measure of distortion appropriate for individuals with varying degrees of hearing loss and cognitive ability, that also aligns with user perception. This further begs the question of whether some test of cognitive ability is useful or implementable within clinical practice.
In this paper we have proposed an approach to setting hearing aids for older adults. Whilst this may be derived from the balance of evidence, it is not specifically validated. We have therefore designed a randomized control trial based on the principles discussed in this paper, pre-registered on the Center for Open Science’s Open Science Framework (OSF), at https://osf.io/fdzeh. The SHAOA (Setting Hearing Aids for Older Adults) study has been approved by the UK Health Research Authority and National Health Service (NHS) Research Ethics Committee (IRAS ID 313159), and it has been adopted onto the NHS National Institute for Health Research (NIHR) portfolio. Finally, in suggesting this approach, we make an implicit assumption that audiologists may not consider all of the factors that have been highlighted here, which we have not evidenced. Accordingly, we will also complete an online survey of UK clinicians to evaluate this, under University of Manchester ethics approval.
RW conducted an initial long-form literature review which was reviewed with comments and changes from HD and AH. RW wrote the draft of this paper with comments and revisions from HD and AH. RW is Principal Investigator for ongoing research with HD and AH as academic supervisors. All authors contributed to the article and approved the submitted version.
RW is funded by the NHS National School of Healthcare Science (NSHCS) as part of the NHS Higher Scientific Specialist Training (HSST) scheme, supporting work at the Royal Berkshire NHS Foundation Trust. HD and AH are supported by the NHS National Institute for Health Research (NIHR) Manchester Biomedical Research Centre.
RW would like to thank: his colleagues at the Royal Berkshire NHS for the considerable support that enabled this work to be undertaken within a busy clinical domain; his father and father-in-law, Keith Windle and Peter Christie, for being the inspiration and unknowing subjects of the paper; and his family for doing family things whilst we were doing the research. Thanks also to Helen Henshaw for volunteering advice on cognitive domains and the paper.
The authors declare that the research was conducted in the absence of any commercial or financial relationships that could be construed as a potential conflict of interest.
All claims expressed in this article are solely those of the authors and do not necessarily represent those of their affiliated organizations, or those of the publisher, the editors and the reviewers. Any product that may be evaluated in this article, or claim that may be made by its manufacturer, is not guaranteed or endorsed by the publisher.
1. Helfer, KS, Bartlett, EL, Popper, AN, and Fay, RR. Aging and hearing: causes and consequences. Cham, Switzerland: Springer (2020).
2. Windle, R. Trends in COSI responses associated with age and degree of hearing loss. Int J Audiol. (2022) 61:416–27. doi: 10.1080/14992027.2021.1937347
3. Fullgrabe, C, Moore, BC, and Stone, MA. Age-group differences in speech identification despite matched audiometrically normal hearing: contributions from auditory temporal processing and cognition. Front Aging Neurosci. (2014) 6:347. doi: 10.3389/fnagi.2014.00347
4. Joris, PX, Schreiner, CE, and Rees, A. Neural processing of amplitude-modulated sounds. Physiol Rev. (2004) 84:541–77. doi: 10.1152/physrev.00029.2003
5. Steinmetzger, K, and Rosen, S. The role of periodicity in perceiving speech in quiet and in background noise. J Acoust Soc Am. (2015) 138:3586–99. doi: 10.1121/1.4936945
6. Xu, Y, Chen, M, LaFaire, P, Tan, X, and Richter, CP. Distorting temporal fine structure by phase shifting and its effects on speech intelligibility and neural phase locking. Sci Rep. (2017) 7:13387. doi: 10.1038/s41598-017-12975-3
7. Middlebrooks, JC, Simon, JZ, Popper, AN, and Fay, RR. The auditory system at the cocktail party. New York, NY: Springer Berlin Heidelberg (2017).
8. Babajani-Feremi, A. Neural mechanism underling comprehension of narrative speech and its heritability: study in a large population. Brain Topogr. (2017) 30:592–609. doi: 10.1007/s10548-017-0550-6
9. Friederici, AD. The cortical language circuit: from auditory perception to sentence comprehension. Trends Cogn Sci. (2012) 16:262–8. doi: 10.1016/j.tics.2012.04.001
10. Musso, M, Weiller, C, Horn, A, Glauche, V, Umarova, R, Hennig, J, et al. A single dual-stream framework for syntactic computations in music and language. NeuroImage. (2015) 117:267–83. doi: 10.1016/j.neuroimage.2015.05.020
11. Bronkhorst, AW. The cocktail-party problem revisited: early processing and selection of multi-talker speech. Atten Percept Psychophysiol. (2015) 77:1465–87. doi: 10.3758/s13414-015-0882-9
12. Eggermont, JJ. The auditory brain and age-related hearing impairment. London, United Kingdom: Academic Press (2019).
13. Gordon-Salant, S, Frisina, RD, Popper, AN, and Fay, RR. The aging auditory system. New York: SpringerLink (2010).
14. Ouda, L, Profant, O, and Syka, J. Age-related changes in the central auditory system. Cell Tissue Res. (2015) 361:337–58. doi: 10.1007/s00441-014-2107-2
15. Roth, TN. Aging of the auditory system. Handb Clin Neurol. (2015) 129:357–73. doi: 10.1016/B978-0-444-62630-1.00020-2
16. Lee, JY. Aging and Speech Understanding. J Audiol Otol. (2015) 19:7–13. doi: 10.7874/jao.2015.19.1.7
17. Golding, M, Carter, N, Mitchell, P, and Hood, LJ. Prevalence of central auditory processing (cap) abnormality in an older Australian population: the Blue Mountains hearing study. J Am Acad Audiol. (2004) 15:633–42. doi: 10.3766/jaaa.15.9.4
18. Liberman, MC, and Kujawa, SG. Cochlear synaptopathy in acquired sensorineural hearing loss: manifestations and mechanisms. Hear Res. (2017) 349:138–47. doi: 10.1016/j.heares.2017.01.003
19. Oxenham, AJ. Predicting the perceptual consequences of hidden hearing loss. Trends Hear. (2016) 20:2331216516686768. doi: 10.1177/2331216516686768
20. Harris, KC, Ahlstrom, JB, Dias, JW, Kerouac, LB, McClaskey, CM, Dubno, JR, et al. Neural Presbyacusis in humans inferred from age-related differences in auditory nerve function and structure. J Neurosci. (2021) 41:10293–304. doi: 10.1523/JNEUROSCI.1747-21.2021
21. Gordon-Salant, S, Shader, MJ, and Wingfield, A. Age-related changes in speech understanding: peripheral versus cognitive influences In: KS Helfer, EL Bartlett, AN Popper, and RR Fay, editors. Aging and hearing: Causes and consequences. Cham: Springer International Publishing (2020). 199–230.
22. Chen, B, Zhong, Y, Peng, W, Sun, Y, and Kong, WJ. Age-related changes in the central auditory system: comparison of D-galactose-induced aging rats and naturally aging rats. Brain Res. (2010) 1344:43–53. doi: 10.1016/j.brainres.2010.04.082
23. Profant, O, Jilek, M, Bures, Z, Vencovsky, V, Kucharova, D, Svobodova, V, et al. Functional age-related changes within the human auditory system studied by audiometric examination. Front Aging Neurosci. (2019) 11:26. doi: 10.3389/fnagi.2019.00026
24. Syka, J. Age-related changes in the auditory brainstem and inferior colliculus In: KS Helfer, EL Bartlett, AN Popper, and RR Fay, editors. Aging and hearing: Causes and consequences. Cham: Springer International Publishing (2020). 67–96.
25. Walton, JP. Timing is everything: temporal processing deficits in the aged auditory brainstem. Hear Res. (2010) 264:63–9. doi: 10.1016/j.heares.2010.03.002
26. Gallun, FJ, and Best, V. Age-related changes in segregation of sound sources In: KS Helfer, EL Bartlett, AN Popper, and RR Fay, editors. Aging and hearing: Causes and consequences. Cham: Springer International Publishing (2020). 143–71.
27. Gordon-Salant, S, Yeni-Komshian, GH, Fitzgibbons, PJ, Cohen, JI, and Waldroup, C. Recognition of accented and unaccented speech in different maskers by younger and older listeners. J Acoust Soc Am. (2013) 134:618–27. doi: 10.1121/1.4807817
28. Weissgerber, T, Müller, C, Stöver, T, and Baumann, U. Age differences in speech perception in noise and sound localization in individuals with subjective Normal hearing. Front Psychol. (2022) 13:13. doi: 10.3389/fpsyg.2022.845285
29. Rosen, S. Temporal information in speech: acoustic, auditory and linguistic aspects. Philos Trans R Soc Lond Ser B Biol Sci. (1992) 336:367–73. doi: 10.1098/rstb.1992.0070
30. Smith, ZM, Delgutte, B, and Oxenham, AJ. Chimaeric sounds reveal dichotomies in auditory perception. Nature. (2002) 416:87–90. doi: 10.1038/416087a
31. Teng, X, Cogan, GB, and Poeppel, D. Speech fine structure contains critical temporal cues to support speech segmentation. NeuroImage. (2019) 202:116152. doi: 10.1016/j.neuroimage.2019.116152
32. Cameron, S, Dillon, H, and Newall, P. Development and evaluation of the listening in spatialized noise test. Ear Hear. (2006) 27:30–42. doi: 10.1097/01.aud.0000194510.57677.03
33. Shannon, RV, Zeng, FG, Kamath, V, Wygonski, J, and Ekelid, M. Speech recognition with primarily temporal cues. Science. (1995) 270:303–4. doi: 10.1126/science.270.5234.303
34. Hopkins, K, and Moore, BC. The importance of temporal fine structure information in speech at different spectral regions for Normal-hearing and hearing-impaired subjects. J Acoust Soc Am. (2010) 127:1595–608. doi: 10.1121/1.3293003
35. Hopkins, K, Moore, BC, and Stone, MA. Effects of moderate Cochlear hearing loss on the ability to benefit from temporal fine structure information in speech. J Acoust Soc Am. (2008) 123:1140–53. doi: 10.1121/1.2824018
36. Shamma, S, and Lorenzi, C. On the balance of envelope and temporal fine structure in the encoding of speech in the early auditory system. J Acoust Soc Am. (2013) 133:2818–33. doi: 10.1121/1.4795783
37. Ding, N, Chatterjee, M, and Simon, JZ. Robust cortical entrainment to the speech envelope relies on the spectro-temporal fine structure. NeuroImage. (2014) 88:41–6. doi: 10.1016/j.neuroimage.2013.10.054
38. Moon, IJ, Won, JH, Park, MH, Ives, DT, Nie, K, Heinz, MG, et al. Optimal combination of neural temporal envelope and fine structure cues to explain speech identification in background noise. J Neurosci. (2014) 34:12145–54. doi: 10.1523/JNEUROSCI.1025-14.2014
39. Swaminathan, J, Mason, CR, Streeter, TM, Best, V, Roverud, E, and Kidd, G Jr. Role of binaural temporal fine structure and envelope cues in cocktail-party listening. J Neurosci. (2016) 36:8250–7. doi: 10.1523/JNEUROSCI.4421-15.2016
40. Warnecke, M, Peng, ZE, and Litovsky, RY. The impact of temporal fine structure and signal envelope on auditory motion perception. PLoS One. (2020) 15:e0238125. doi: 10.1371/journal.pone.0238125
41. Lorenzi, C, Gilbert, G, Carn, H, Garnier, S, and Moore, BC. Speech perception problems of the hearing impaired reflect inability to use temporal fine structure. Proc Natl Acad Sci U S A. (2006) 103:18866–9. doi: 10.1073/pnas.0607364103
42. Besser, J, Festen, JM, Goverts, ST, Kramer, SE, and Pichora-Fuller, MK. Speech-in-speech listening on the Lisn-S test by older adults with good audiograms depends on cognition and hearing acuity at high frequencies. Ear Hear. (2015) 36:24–41. doi: 10.1097/AUD.0000000000000096
43. Ren, F, Luo, J, Ma, W, Xin, Q, Xu, L, Fan, Z, et al. Hearing loss and cognition among older adults in a Han Chinese cohort. Front Neurosci. (2019) 13:632. doi: 10.3389/fnins.2019.00632
44. Schurman, J, Brungart, D, and Gordon-Salant, S. Effects of masker type, sentence context, and listener age on speech recognition performance in 1-Back listening tasks. J Acoust Soc Am. (2014) 136:3337. doi: 10.1121/1.4901708
45. Moore, BC, Vickers, DA, and Mehta, A. The effects of age on temporal fine structure sensitivity in monaural and binaural conditions. Int J Audiol. (2012) 51:715–21. doi: 10.3109/14992027.2012.690079
46. Moore, BCJ. Effects of age and hearing loss on the processing of auditory temporal fine structure. Adv Exp Med Biol. (2016) 894:1–8. doi: 10.1007/978-3-319-25474-6_1
47. Moore, BCJ. Effects of hearing loss and age on the binaural processing of temporal envelope and temporal fine structure information. Hear Res. (2021) 402:107991. doi: 10.1016/j.heares.2020.107991
48. Ellis, RJ, and Ronnberg, J. Temporal fine structure: associations with cognition and speech-in-noise recognition in adults with Normal hearing or hearing impairment. Int J Audiol. (2022) 61:778–86. doi: 10.1080/14992027.2021.1948119
49. Moore, DR, Edmondson-Jones, M, Dawes, P, Fortnum, H, McCormack, A, Pierzycki, RH, et al. Relation between speech-in-noise threshold, hearing loss and cognition from 40-69 years of age. PLoS One. (2014) 9:e107720. doi: 10.1371/journal.pone.0107720
50. Innes-Brown, H, Tsongas, R, Marozeau, J, and McKay, C. Towards objective measures of functional hearing abilities. Adv Exp Med Biol. (2016) 894:315–25. doi: 10.1007/978-3-319-25474-6_33
51. Ronnberg, J, Lunner, T, Ng, EH, Lidestam, B, Zekveld, AA, Sorqvist, P, et al. Hearing impairment, cognition and speech understanding: exploratory factor analyses of a comprehensive test battery for a Group of Hearing aid Users, the N200 study. Int J Audiol. (2016) 55:623–42. doi: 10.1080/14992027.2016.1219775
52. Vercammen, C, Goossens, T, Undurraga, J, Wouters, J, and van Wieringen, A. Electrophysiological and Behavioral evidence of reduced binaural temporal processing in the aging and hearing impaired human auditory system. Trends Hear. (2018) 22:2331216518785733. doi: 10.1177/2331216518785733
53. Souza, P, Arehart, K, Miller, CW, and Muralimanohar, RK. Effects of age on F0 discrimination and intonation perception in simulated electric and electroacoustic hearing. Ear Hear. (2011) 32:75–83. doi: 10.1097/AUD.0b013e3181eccfe9
54. Zaltz, Y, and Kishon-Rabin, L. Difficulties experienced by older listeners in utilizing voice cues for speaker discrimination. Front Psychol. (2022) 13:13. doi: 10.3389/fpsyg.2022.797422
55. Paulmann, S, Pell, MD, and Kotz, SA. How aging affects the recognition of emotional speech. Brain Lang. (2008) 104:262–9. doi: 10.1016/j.bandl.2007.03.002
56. Dor, YI, Algom, D, Shakuf, V, and Ben-David, BM. Age-related changes in the perception of emotions in speech: assessing thresholds of prosody and semantics recognition in noise for young and older adults. Front Neurosci. (2022) 16:16. doi: 10.3389/fnins.2022.846117
57. Kihlstrom, JF. Unconscious cognition. Reference module in neuroscience and Biobehavioral psychology. Amsterdam: Elsevier (2018).
58. Flanagan, DP, McGrew, KS, and Ortiz, SO. The Wechsler intelligence scales and gf-Gc theory: a contemporary approach to interpretation, vol. xx. Boston: Allyn and Bacon (2000). 424 p.
59. Davidson, PSR, and Winocur, G. Aging and cognition. Reference module in neuroscience and Biobehavioral psychology. Amsterdam: Elsevier (2017).
60. Fisher, GG, Chacon, M, and Chaffee, DS. Chapter 2 - theories of cognitive aging and work In: BB Baltes, CW Rudolph, and H Zacher, editors. Work across the lifespan. London: Academic Press (2019). 17–45.
61. Shen, J, Anderson, MC, Arehart, KH, and Souza, PE. Using cognitive screening tests in audiology. Am J Audiol. (2016) 25:319–31. doi: 10.1044/2016_AJA-16-0032
62. Park, DC, and Bischof, GN. The aging mind: neuroplasticity in response to cognitive training. Dialogues Clin Neurosci. (2013) 15:109–19. doi: 10.31887/DCNS.2013.15.1/dpark
63. Sanchez-Izquierdo, M, and Fernandez-Ballesteros, R. Cognition in healthy aging. Int J Environ Res Public Health. (2021) 18:962. doi: 10.3390/ijerph18030962
64. Heinrich, A. The role of cognition for speech-in-noise perception: considering individual listening strategies related to aging and hearing loss. Int J Behav Dev. (2021) 45:382–8. doi: 10.1177/0165025420914984
65. Song, S, Stern, Y, and Gu, Y. Modifiable lifestyle factors and cognitive reserve: a systematic review of current evidence. Ageing Res Rev. (2022) 74:101551. doi: 10.1016/j.arr.2021.101551
66. Hertzog, C, Kramer, AF, Wilson, RS, and Lindenberger, U. Enrichment effects on adult cognitive development: can the functional capacity of older adults be preserved and enhanced? Psychol Sci Public Interest. (2008) 9:1–65. doi: 10.1111/j.1539-6053.2009.01034.x
67. Salthouse, TA. The processing-speed theory of adult age differences in cognition. Psychol Rev. (1996) 103:403–28. doi: 10.1037/0033-295X.103.3.403
68. Craik, FIM, and Byrd, M. Aging and cognitive deficits In: C FIM and S Trehub, editors. Aging and cognitive processes. Boston, MA: Springer US (1982). 191–211. doi: 10.1007/978-1-4684-4178-9_11
70. Baddeley, AD, and Hitch, G. Working memory In: GH Bower, editor. Psychology of learning and motivation. 8. London: Academic Press (1974). 47–89.
71. Hasher, L, Lustig, C, and Zacks, R. Inhibitory mechanisms and the control of attention In: ARA Conway, C Jarrold, MJ Kane, A Miyake, and JN Towse, editors. Variation in working memory : Oxford: University Press (2007). 227–49.
72. Flanagan, DP, and Dixon, SG. The Cattell-Horn-Carroll theory of cognitive abilities In: CR Reynolds, KJ Vannes, and E Fletcher-Janzen, editors. Encyclopedia of special education. New Jersey: Wiley (2014)
73. Webb, SL, Loh, V, Lampit, A, Bateman, JE, and Birney, DP. Meta-analysis of the effects of computerized cognitive training on executive functions: a cross-disciplinary taxonomy for classifying outcome cognitive factors. Neuropsychol Rev. (2018) 28:232–50. doi: 10.1007/s11065-018-9374-8
74. Miyake, A, and Friedman, NP. The nature and Organization of Individual Differences in executive functions:four general conclusions. Curr Dir Psychol Sci. (2012) 21:8–14. doi: 10.1177/0963721411429458
75. Heinrich, A, Henshaw, H, and Ferguson, MA. Only Behavioral but not self-report measures of speech perception correlate with cognitive abilities. Front Psychol. (2016) 7:576. doi: 10.3389/fpsyg.2016.00576
76. Heinrich, A, and Knight, S. The contribution of auditory and cognitive factors to intelligibility of words and sentences in noise. Adv Exp Med Biol. (2016) 894:37–45. doi: 10.1007/978-3-319-25474-6_5
77. Janse, E, and Andringa, SJ. The roles of cognitive abilities and hearing acuity in older adults’ recognition of words taken from fast and spectrally reduced speech. Appl Psycholinguist. (2021) 42:1–28. doi: 10.1017/S0142716421000047
78. Lentz, JJ, Humes, LE, and Kidd, GR. Differences in auditory perception between young and older adults when controlling for differences in hearing loss and cognition. Trends Hear. (2022) 26:23312165211066180. doi: 10.1177/23312165211066180
79. Humes, LE, Kidd, GR, and Lentz, JJ. Differences between young and older adults in working memory and performance on the test of basic auditory capabilities†. Front Psychol. (2022) 12:12. doi: 10.3389/fpsyg.2021.804891
80. Fullgrabe, C, and Rosen, S. Investigating the role of working memory in speech-in-noise identification for listeners with normal hearing. Adv Exp Med Biol. (2016) 894:29–36. doi: 10.1007/978-3-319-25474-6_4
81. Michalek, AMP, Ash, I, and Schwartz, K. The Independence of working memory capacity and audiovisual cues when listening in noise. Scand J Psychol. (2018) 59:578–85. doi: 10.1111/sjop.12480
82. Nitsan, G, Banai, K, and Ben-David, BM. One size does not fit all: examining the effects of working memory capacity on spoken word recognition in older adults using eye tracking. Front Psychol. (2022) 13:13. doi: 10.3389/fpsyg.2022.841466
83. Akeroyd, MA. Are individual differences in speech reception related to individual differences in cognitive ability? A survey of twenty experimental studies with Normal and hearing-impaired adults. Int J Audiol. (2008) 47:S53–71. doi: 10.1080/14992020802301142
84. Heinrich, A, Henshaw, H, and Ferguson, MA. The relationship of speech intelligibility with hearing sensitivity, cognition, and perceived hearing difficulties varies for different speech perception tests. Front Psychol. (2015) 6:782. doi: 10.3389/fpsyg.2015.00782
85. Zekveld, AA, Rudner, M, Johnsrude, IS, and Ronnberg, J. The effects of working memory capacity and semantic cues on the intelligibility of speech in noise. J Acoust Soc Am. (2013) 134:2225–34. doi: 10.1121/1.4817926
86. Rudner, M. Cognitive spare capacity as an index of listening effort. Ear Hear. (2016) 37:69S–76S. doi: 10.1097/AUD.0000000000000302
87. Pichora-Fuller, MK. Processing speed and timing in aging adults: psychoacoustics, speech perception, and comprehension. Int J Audiol. (2003) 42:S59–67. doi: 10.3109/14992020309074625
88. Janse, E. A non-auditory measure of interference predicts distraction by competing speech in older adults. Neuropsychol Dev Cogn B Aging Neuropsychol Cogn. (2012) 19:741–58. doi: 10.1080/13825585.2011.652590
89. Roque, L, Karawani, H, Gordon-Salant, S, and Anderson, S. Effects of age, cognition, and neural encoding on the perception of temporal speech cues. Front Neurosci. (2019) 13:749. doi: 10.3389/fnins.2019.00749
90. Torrente, MC, Vergara, R, Moreno-Gomez, FN, Leiva, A, San Martin, S, Belkhiria, C, et al. Speech perception and dichotic listening are associated with hearing thresholds and cognition, respectively, in unaided Presbycusis. Front Aging Neurosci. (2022) 14:786330. doi: 10.3389/fnagi.2022.786330
91. Shechter Shvartzman, L, Lavie, L, and Banai, K. Speech perception in older adults: an interplay of hearing, cognition, and learning? Front Psychol. (2022) 13:816864. doi: 10.3389/fpsyg.2022.816864
92. Dryden, A, Allen, HA, Henshaw, H, and Heinrich, A. The association between cognitive performance and speech-in-noise perception for adult listeners: a systematic literature review and Meta-analysis. Trends Hear. (2017) 21:23–31. doi: 10.1177/2331216517744675
93. Salthouse, TA. Influence of processing speed on adult age differences in working memory. Acta Psychol. (1992) 79:155–70. doi: 10.1016/0001-6918(92)90030-H
94. Ward, KM, Shen, J, Souza, PE, and Grieco-Calub, TM. Age-related differences in listening effort during degraded speech recognition. Ear Hear. (2017) 38:74–84. doi: 10.1097/AUD.0000000000000355
95. Zekveld, AA, Kramer, SE, and Festen, JM. Cognitive load during speech perception in noise: the influence of age, hearing loss, and cognition on the pupil response. Ear Hear. (2011) 32:498–510. doi: 10.1097/AUD.0b013e31820512bb
96. Pichora-Fuller, MK, Kramer, SE, Eckert, MA, Edwards, B, Hornsby, BW, Humes, LE, et al. Hearing impairment and cognitive energy: the framework for understanding effortful listening (Fuel). Ear Hear. (2016) 37:5S–27S. doi: 10.1097/AUD.0000000000000312
97. Francis, AL, and Love, J. Listening effort: are we measuring cognition or affect, or both? Wiley Interdiscip Rev Cogn Sci. (2020) 11:e1514. doi: 10.1002/wcs.1514
98. Hickson, L, Meyer, C, Lovelock, K, Lampert, M, and Khan, A. Factors associated with success with hearing aids in older adults. Int J Audiol. (2014) 53:S18–27. doi: 10.3109/14992027.2013.860488
99. Meyer, C, Hickson, L, and Fletcher, A. Identifying the barriers and facilitators to optimal hearing aid self-efficacy. Int J Audiol. (2014) 53:S28–37. doi: 10.3109/14992027.2013.832420
100. Brennan, MA, Lewis, D, McCreery, R, Kopun, J, and Alexander, JM. Listening effort and speech recognition with frequency compression amplification for children and adults with hearing loss. J Am Acad Audiol. (2017) 28:823–37. doi: 10.3766/jaaa.16158
101. Ohlenforst, B, Zekveld, AA, Jansma, EP, Wang, Y, Naylor, G, Lorens, A, et al. Effects of hearing impairment and hearing aid amplification on listening effort: a systematic review. Ear Hear. (2017) 38:267–81. doi: 10.1097/AUD.0000000000000396
102. Rovetti, J, Goy, H, Pichora-Fuller, MK, and Russo, FA. Functional near-infrared spectroscopy as a measure of listening effort in older adults who use hearing aids. Trends Hear. (2019) 23:2331216519886722. doi: 10.1177/2331216519886722
103. Shehorn, J, Marrone, N, and Muller, T. Speech perception in noise and listening effort of older adults with nonlinear frequency compression hearing aids. Ear Hear. (2018) 39:215–25. doi: 10.1097/AUD.0000000000000481
104. Desjardins, JL, and Doherty, KA. The effect of hearing aid noise reduction on listening effort in hearing-impaired adults. Ear Hear. (2014) 35:600–10. doi: 10.1097/AUD.0000000000000028
105. Mattys, SL, Davis, MH, Bradlow, AR, and Scott, SK. Speech recognition in adverse conditions: a review. Lang Cogn Process. (2012) 27:953–78. doi: 10.1080/01690965.2012.705006
106. Lunner, T, and Sundewall-Thoren, E. Interactions between cognition, compression, and listening conditions: effects on speech-in-noise performance in a Two-Channel hearing aid. J Am Acad Audiol. (2007) 18:604–17. doi: 10.3766/jaaa.18.7.7
107. Ng, EHN, and Ronnberg, J. Hearing aid experience and background noise affect the robust relationship between working memory and speech recognition in noise. Int J Audiol. (2020) 59:208–18. doi: 10.1080/14992027.2019.1677951
108. Nuesse, T, Steenken, R, Neher, T, and Holube, I. Exploring the link between cognitive abilities and speech recognition in the elderly under different listening conditions. Front Psychol. (2018) 9:678. doi: 10.3389/fpsyg.2018.00678
109. McAuley, JD, Shen, Y, Smith, T, and Kidd, GR. Effects of speech-rhythm disruption on selective listening with a single background talker. Atten Percept Psychophysiol. (2021) 83:2229–40. doi: 10.3758/s13414-021-02298-x
110. Wingfield, A, and Tun, PA. Cognitive supports and cognitive constraints on comprehension of spoken language. J Am Acad Audiol. (2007) 18:548–58. doi: 10.3766/jaaa.18.7.3
111. Rönnberg, J, Holmer, E, and Rudner, M. Cognitive hearing science and ease of language understanding. Int J Audiol. (2019) 58:247–61. doi: 10.1080/14992027.2018.1551631
112. Rönnberg, J, Holmer, E, and Rudner, M. Cognitive hearing science: three memory systems, two approaches, and the ease of language understanding model. J Speech Lang Hear Res. (2021) 64:359–70. doi: 10.1044/2020_JSLHR-20-00007
113. Kates, JM, Arehart, KH, and Souza, PE. Integrating cognitive and peripheral factors in predicting hearing-aid processing effectiveness. J Acoust Soc Am. (2013) 134:4458. doi: 10.1121/1.4824700
114. Huber, R, Rahlmann, S, Bisitz, T, Meis, M, Steinhauser, S, and Meister, H. Influence of working memory and attention on sound-quality ratings. J Acoust Soc Am. (2019) 145:1283. doi: 10.1121/1.5092808
115. Kurthen, I, Meyer, M, Schlesewsky, M, and Bornkessel-Schlesewsky, I. Individual differences in peripheral hearing and cognition reveal sentence processing differences in healthy older adults. Front Neurosci. (2020) 14:573513. doi: 10.3389/fnins.2020.573513
116. Fletcher, A, McAuliffe, M, Kerr, S, and Sinex, D. Effects of vocabulary and implicit linguistic knowledge on speech recognition in adverse listening conditions. Am J Audiol. (2019) 28:742–55. doi: 10.1044/2019_AJA-HEAL18-18-0169
117. Kim, S, Choi, I, Schwalje, AT, Kim, K, and Lee, JH. Auditory working memory explains variance in speech recognition in older listeners under adverse listening conditions. Clin Interv Aging. (2020) 15:395–406. doi: 10.2147/CIA.S241976
118. Schubotz, L, Holler, J, Drijvers, L, and Ozyurek, A. Aging and working memory modulate the ability to benefit from visible speech and iconic gestures during speech-in-noise comprehension. Psychol Res. (2020) 85:1997–2011. doi: 10.1007/s00426-020-01363-8
119. Taljaard, DS, Olaithe, M, Brennan-Jones, CG, Eikelboom, RH, and Bucks, RS. The relationship between hearing impairment and cognitive function: a meta-analysis in adults. Clin Otolaryngol. (2016) 41:718–29. doi: 10.1111/coa.12607
120. Chung, K. Theories on hearing-cognition functions. Hear J. (2018) 71:10–2. doi: 10.1097/01.HJ.0000550399.89895.51
121. Fullgrabe, C. On the possible overestimation of cognitive decline: the impact of age-related hearing loss on cognitive-test performance. Front Neurosci. (2020) 14:454. doi: 10.3389/fnins.2020.00454
122. Dillon, H, Day, J, Bant, S, and Munro, KJ. Adoption, use and non-use of hearing aids: a robust estimate based on welsh National Survey Statistics. Int J Audiol. (2020) 59:567–73. doi: 10.1080/14992027.2020.1773550
123. Lesica, NA. Why do hearing aids fail to restore Normal auditory perception? Trends Neurosci. (2018) 41:174–85. doi: 10.1016/j.tins.2018.01.008
124. Arehart, KH, Souza, P, Baca, R, and Kates, JM. Working memory, age, and hearing loss: susceptibility to hearing aid distortion. Ear Hear. (2013) 34:251–60. doi: 10.1097/AUD.0b013e318271aa5e
125. Lunner, T, Rudner, M, and RÖNnberg, J. Cognition and hearing aids. Scand J Psychol. (2009) 50:395–403. doi: 10.1111/j.1467-9450.2009.00742.x
126. Davidson, A, Marrone, N, and Souza, P. Hearing aid technology settings and speech-in-noise difficulties. Am J Audiol. (2022) 31:21–31. doi: 10.1044/2021_AJA-21-00176
127. Tognola, G, Mainardi, A, Vincenti, V, and Cuda, D. Benefit of hearing aid use in the elderly: the impact of age, cognition and hearing impairment. Acta Otorhinolaryngol Ital. (2019) 39:409–18. doi: 10.14639/0392-100X-2165
128. Farahani, ED, Wouters, J, and van Wieringen, A. Neural generators underlying temporal envelope processing show altered responses and hemispheric asymmetry across age. Front Aging Neurosci. (2020) 12:596551. doi: 10.3389/fnagi.2020.596551
129. Souza, PE, Ellis, G, Marks, K, Wright, R, and Gallun, F. Does the speech Cue profile affect response to amplitude envelope distortion? J Speech Lang Hear Res. (2021) 64:2053–69. doi: 10.1044/2021_JSLHR-20-00481
130. Jenstad, L. (2011). Considerations in hearing aid fitting for older adults & overcoming the hearing aid barrier. Audiology Online. December 6, 2011.
131. Gordon-Salant, S, Yeni-Komshian, G, and Fitzgibbons, P. The role of temporal cues in word identification by younger and older adults: effects of sentence context. J Acoust Soc Am. (2008) 124:3249–60. doi: 10.1121/1.2982409
132. Goupell, MJ. Age-related changes in Interaural-level-difference-based across-frequency binaural interference. Front Aging Neurosci. (2022) 14:887401. doi: 10.3389/fnagi.2022.887401
133. Mussoi, BSS, and Bentler, RA. Binaural interference and the effects of age and hearing loss. J Am Acad Audiol. (2017) 28:5–13. doi: 10.3766/jaaa.15011
134. Henkin, Y, Waldman, A, and Kishon-Rabin, L. The benefits of bilateral versus unilateral amplification for the elderly: are two always better than one? J Basic Clin Physiol Pharmacol. (2007) 18:201–16. doi: 10.1515/jbcpp.2007.18.3.201
135. Azevedo, MM, Neujahr dos Santos, S, and Costa, MJ. Performance of elderly adults with binaural vs. monaural fitting in speech tests in silence and in noise. Rev CEFAC. (2015) 17:431–8. doi: 10.1590/1982-021620152514
136. Holmes, AE. Bilateral amplification for the elderly: are two aids better than one? Int J Audiol. (2003) 42:63–7. Epub 2003/08/16. doi: 10.3109/14992020309074646
137. Plomp, R. A signal-to-noise ratio model for the speech-reception threshold of the hearing impaired. J Speech Hear Res. (1986) 29:146–54. doi: 10.1044/jshr.2902.146
138. Schum, DJ. (2013). The distortional aspect of sensorineural hearing loss: what can be done. Audiology Online.
139. Humes, LE. Modeling and predicting hearing aid outcome. Trends Amplif. (2003) 7:41–75. doi: 10.1177/108471380300700202
140. Humes, LE. The contributions of audibility and cognitive factors to the benefit provided by amplified speech to older adults. J Am Acad Audiol. (2007) 18:590–603. doi: 10.3766/jaaa.18.7.6
141. Gatehouse, S, Naylor, G, and Elberling, C. Linear and nonlinear hearing aid fittings--2. Patterns of candidature. Int J Audiol. (2006) 45:153–71. doi: 10.1080/14992020500429484
142. Dillon, H, Keidser, G, O'Brien, A, and Silberstein, H. Sound quality comparisons of advanced hearing aids. Hear J. (2003) 56:30. doi: 10.1097/01.HJ.0000293908.50552.34
143. Korhonen, P, Kuk, F, and Slugocki, C. A method to evaluate the effect of signal processing on the temporal envelope of speech. Hear Rev. (2019) 26:10–8.
144. Stone, MA, and Moore, BC. Quantifying the effects of fast-acting compression on the envelope of speech. J Acoust Soc Am. (2007) 121:1654–64. doi: 10.1121/1.2434754
145. Ponsot, E, Varnet, L, Wallaert, N, Daoud, E, Shamma, SA, Lorenzi, C, et al. Mechanisms of Spectrotemporal modulation detection for Normal- and hearing-impaired listeners. Trends Hear. (2021) 25:2331216520978029. doi: 10.1177/2331216520978029
146. Mehraei, G, Gallun, FJ, Leek, MR, and Bernstein, JG. Spectrotemporal modulation sensitivity for hearing-impaired listeners: dependence on carrier Center frequency and the relationship to speech intelligibility. J Acoust Soc Am. (2014) 136:301–16. doi: 10.1121/1.4881918
147. Bernstein, JG, Danielsson, H, Hallgren, M, Stenfelt, S, Ronnberg, J, and Lunner, T. Spectrotemporal modulation sensitivity as a predictor of speech-reception performance in noise with hearing aids. Trends Hear. (2016) 20:20. doi: 10.1177/2331216516670387
148. Kates, JM, and Arehart, KH. An overview of the Haspi and Hasqi metrics for predicting speech intelligibility and speech quality for Normal hearing, hearing loss, and hearing aids. Hear Res. (2022) 426:108608. doi: 10.1016/j.heares.2022.108608
149. Kates, JM, Arehart, KH, Anderson, MC, Kumar Muralimanohar, R, and Harvey, LO Jr. Using objective metrics to measure hearing aid performance. Ear Hear. (2018) 39:1165–75. doi: 10.1097/AUD.0000000000000574
150. Moore, BC. The choice of compression speed in hearing aids: theoretical and practical considerations and the role of individual differences. Trends Amplif. (2008) 12:103–12. doi: 10.1177/1084713808317819
151. Souza, PE. Effects of compression on speech acoustics, intelligibility, and sound quality. Trends Amplif. (2002) 6:131–65. doi: 10.1177/108471380200600402
153. Kuk, F, and Hau, O. (2017) Compression speed and cognition: a variable speed compressor for all. Hearing Review. March, 2017.
154. Kuk, F, Slugocki, C, Korhonen, P, Seper, E, and Hau, O. Evaluation of the efficacy of a dual variable speed compressor over a single fixed speed compressor. J Am Acad Audiol. (2019) 30:590–606. doi: 10.3766/jaaa.17127
155. May, T, Kowalewski, B, and Dau, T. Signal-to-noise-ratio-aware dynamic range compression in hearing aids. Trends Hear. (2018) 22:2331216518790903. doi: 10.1177/2331216518790903
156. May, T, Kowalewski, B, and Dau, T. Scene-aware dynamic-range compression in hearing aids In: J Blauert and J Braasch, editors. The Technology of Binaural Understanding, Modern Acoustics and Signal Processing. Cham: Springer International Publishing (2020). 763–99.
157. Pittman, AL, Pederson, AJ, and Rash, MA. Effects of fast, slow, and adaptive amplitude compression on Children's and Adults' perception of meaningful acoustic information. J Am Acad Audiol. (2014) 25:834–47. doi: 10.3766/jaaa.25.9.6
158. Sjolander, L, Quilter, M, and Groth, J. Hearing Aid Users Show Preference for Resound Impulse Noise Reduction. Ballerup, Denmark: ReSound GN (2019). White paper, Available at: https://pro.resound.com/en-gb/research/features-explained
159. Holube, I, Hamacher, V, and Killion, MC. Multi-Channel compression: concepts and (early but timeless) results: less can be more in the application of compression, time constants, and channels. Hear Rev. (2016) 23:20.
160. Brennan, MA, Gallun, FJ, Souza, PE, and Stecker, GC. Temporal resolution with a prescriptive fitting formula. Am J Audiol. (2013) 22:216–25. doi: 10.1044/1059-0889(2013/13-0001)
161. Brown, AD, Rodriguez, FA, Portnuff, CD, Goupell, MJ, and Tollin, DJ. Time-varying distortions of binaural information by bilateral hearing aids: effects of nonlinear frequency compression. Trends Hear. (2016) 20:20. doi: 10.1177/2331216516668303
162. Neher, T, Behrens, T, Carlile, S, Jin, C, Kragelund, L, Petersen, AS, et al. Benefit from spatial separation of multiple talkers in bilateral hearing-aid users: effects of hearing loss, age, and cognition. Int J Audiol. (2009) 48:758–74. doi: 10.3109/14992020903079332
163. Shen, Y, and Lentz, JJ. Effect of fast-acting compression on modulation detection interference for Normal hearing and hearing impaired listeners. J Acoust Soc Am. (2010) 127:3654–65. doi: 10.1121/1.3418684
164. Stone, MA, and Moore, BC. Side effects of fast-acting dynamic range compression that affect intelligibility in a competing speech task. J Acoust Soc Am. (2004) 116:2311–23. doi: 10.1121/1.1784447
165. Wiinberg, A, Jepsen, ML, Epp, B, and Dau, T. Effects of hearing loss and fast-acting compression on amplitude modulation perception and speech intelligibility. Ear Hear. (2019) 40:45–54. doi: 10.1097/AUD.0000000000000589
166. Ernst, SMA, Kortlang, S, Grimm, G, Bisitz, T, Kollmeier, B, and Ewert, SD. Binaural model-based dynamic-range compression. Int J Audiol. (2018) 57:S31–42. doi: 10.1080/14992027.2018.1425554
167. Keidser, G, Rohrseitz, K, Dillon, H, Hamacher, V, Carter, L, Rass, U, et al. The effect of Multi-Channel wide dynamic range compression, noise reduction, and the directional microphone on horizontal localization performance in hearing aid wearers. Int J Audiol. (2006) 45:563–79. doi: 10.1080/14992020600920804
168. Hassager, HG, Wiinberg, A, and Dau, T. Effects of hearing-aid dynamic range compression on spatial perception in a reverberant environment. J Acoust Soc Am. (2017) 141:2556. doi: 10.1121/1.4979783
169. Bor, S, Souza, P, and Wright, R. Multichannel compression: effects of reduced spectral contrast on vowel identification. J Speech Lang Hear Res. (2008) 51:1315–27. doi: 10.1044/1092-4388(2008/07-0009)
170. Villchur, E. Compression in hearing aids: why fast multichannel processing systems work well: Villchur on the controversies surrounding compression in regard to recruitment and speech intelligibility in noise. Hear Rev. (2008) 15:16.
171. Goedegebure, A, Hulshof, M, Maas, RJ, Dreschler, WA, and Verschuure, H. Effects of Single-Channel phonemic compression schemes on the understanding of speech by hearing-impaired listeners. Audiology. (2001) 40:10–25. doi: 10.3109/00206090109073096
172. Gatehouse, S, Naylor, G, and Elberling, C. Linear and nonlinear hearing aid fittings--1. Patterns of benefit. Int J Audiol. (2006) 45:130–52. doi: 10.1080/14992020500429518
173. Olsen, HL, Olofsson, Å, and Hagerman, B. The effect of presentation level and compression characteristics on sentence recognition in modulated noise. Int J Audiol. (2004) 43:283–94. doi: 10.1080/14992020400050038
174. Armstrong, A, Lam, CC, Sabesan, S, and Lesica, NA. The hearing aid dilemma: amplification, compression, and distortion of the neural code. bioRxiv. (2020):2020.10.02.323626). doi: 10.1101/2020.10.02.323626
175. Bruce, IC. Modeling of the auditory periphery for hearing aid evaluation and design. J Acoust Soc Am. (2010) 127:1846. doi: 10.1121/1.3384356
176. Gatehouse, S, Naylor, G, and Elberling, C. Benefits from hearing aids in relation to the interaction between the user and the environment. Int J Audiol. (2003) 42:S77–85. doi: 10.3109/14992020309074627
177. Rudner, M, Foo, C, Ronnberg, J, and Lunner, T. Cognition and aided speech recognition in noise: specific role for cognitive factors following nine-week experience with adjusted compression settings in hearing aids. Scand J Psychol. (2009) 50:405–18. doi: 10.1111/j.1467-9450.2009.00745.x
178. Souza, PE, and Sirow, L. Relating working memory to compression parameters in clinically fit hearing aids. Am J Audiol. (2014) 23:394–401. doi: 10.1044/2014_AJA-14-0006
179. Ohlenforst, B, Souza, PE, and MacDonald, EN. Exploring the relationship between working memory, compressor speed, and background noise characteristics. Ear Hear. (2016) 37:137–43. doi: 10.1097/AUD.0000000000000240
180. Moore, BC, and Sek, A. Preferred compression speed for speech and music and its relationship to sensitivity to temporal fine structure. Trends Hear. (2016) 20:233121651664048. doi: 10.1177/2331216516640486
181. Rallapalli, V, Schauer, J, and Souza, P. Preference for combinations of hearing aid signal processing. J Speech Lang Hear Res. (2022) 65:3100–16. doi: 10.1044/2022_JSLHR-22-00018
182. Rudner, M, Lunner, T, Behrens, T, Thoren, ES, and Ronnberg, J. Working memory capacity May influence perceived effort during aided speech recognition in noise. J Am Acad Audiol. (2012) 23:577–89. doi: 10.3766/jaaa.23.7.7
183. Rudner, M, Ronnberg, J, and Lunner, T. Working memory supports listening in noise for persons with hearing impairment. J Am Acad Audiol. (2011) 22:156–67. doi: 10.3766/jaaa.22.3.4
184. Chen, Y, Wong, LLN, Kuehnel, V, Qian, J, Voss, SC, and Shangqiguo, W. Can dual compression offer better mandarin speech intelligibility and sound quality than fast-acting compression? Trends Hear. (2021) 25:2331216521997610. doi: 10.1177/2331216521997610
185. Salorio-Corbetto, M, Baer, T, Stone, MA, and Moore, BCJ. Effect of the number of amplitude-compression channels and compression speed on speech recognition by listeners with mild to moderate sensorineural hearing loss. J Acoust Soc Am. (2020) 147:1344. doi: 10.1121/10.0000804
186. Jingjing, X, and Robyn, MC. Interactions between cognition and hearing aid compression release time: effects of linguistic context of speech test materials on speech-in-noise performance. Audiol Res. (2021) 11:129–49. doi: 10.3390/audiolres11020013
187. Brennan, M, McCreery, R, and Massey, J. Influence of audibility and distortion on the recognition of reverberant speech for children and adults with hearing aid amplification. J Am Acad Audiol. (2021) 60:849–57. doi: 10.1055/a-1678-3381
188. Xu, J, and Cox, RM. Interactions between cognition and hearing aid compression release time: effects of linguistic context of speech test materials on speech-in-noise performance. Audiol Res. (2021) 11:129–49. doi: 10.3390/audiolres11020013
189. Kowalewski, B, Zaar, J, Fereczkowski, M, MacDonald, EN, Strelcyk, O, May, T, et al. Effects of slow- and fast-acting compression on hearing-impaired Listeners' consonant-vowel identification in interrupted noise. Trends Hear. (2018) 22:2331216518800870. doi: 10.1177/2331216518800870
190. Leijon, A. Comment on exploring the relationship between working memory, compressor speed, and background noise characteristics, ear hear 37, 137-143. Ear Hear. (2017) 38:643–4. doi: 10.1097/AUD.0000000000000439
191. Rahlmann, S, Meis, M, Schulte, M, Kiessling, J, Walger, M, and Meister, H. Assessment of hearing aid algorithms using a master hearing aid: the influence of hearing aid experience on the relationship between speech recognition and cognitive capacity. Int J Audiol. (2018) 57:S105–11. doi: 10.1080/14992027.2017.1319079
192. Kestens, K, Degeest, S, and Keppler, H. The effect of cognition on the aided benefit in terms of speech understanding and listening effort obtained with digital hearing aids: a systematic review. Am J Audiol. (2021) 30:190–210. doi: 10.1044/2020_AJA-20-00019
193. Shetty, HN, and Raju, S. Effect of compression release time of a hearing aid on sentence recognition and the quality judgment of speech. Noise Health. (2019) 21:232–41. Epub 2020/09/27. doi: 10.4103/nah.NAH_54_19
194. Neuman, AC, Bakke, MH, Mackersie, CL, Hellman, S, and Levitt, H. The effect of compression ratio and release time on the categorical rating of sound quality. J Acoust Soc Am. (1998) 103:2273–81. doi: 10.1121/1.422745
196. Hornsby, BWY, and Ricketts, TA. The effects of compression ratio, signal-to-noise ratio, and level on speech recognition in Normal-hearing listeners. J Acoust Soc Am. (2001) 109:2964–73. doi: 10.1121/1.1369105
197. Boike, KT, and Souza, PE. Effect of compression ratio on speech recognition and speech-quality ratings with wide dynamic range compression amplification. J Speech Lang Hear Res. (2000) 43:456–68. doi: 10.1044/jslhr.4302.456
198. Neuman, AC, Bakke, MH, Hellman, S, and Levitt, H. Effect of compression ratio in a slow-acting compression hearing aid: paired-comparison judgments of quality. J Acoust Soc Am. (1994) 96:1471–8. doi: 10.1121/1.410289
199. Stone, MA, and Moore, BCJ. Syllabic compression: effective compression ratios for signals modulated at different rates. Br J Audiol. (1992) 26:351–61. doi: 10.3109/03005369209076659
200. Lakshmi, MSK, Rout, A, and O'Donoghue, CR. A systematic review and Meta-analysis of digital noise reduction hearing aids in adults. Disabil Rehabil Assist Technol. (2021) 16:120–9. doi: 10.1080/17483107.2019.1642394
201. Ng, EH, Rudner, M, Lunner, T, Pedersen, MS, and Ronnberg, J. Effects of noise and working memory capacity on memory processing of speech for hearing-aid users. Int J Audiol. (2013) 52:433–41. doi: 10.3109/14992027.2013.776181
202. Ng, EH, Rudner, M, Lunner, T, and Ronnberg, J. Noise reduction improves memory for target language speech in competing native but not foreign language speech. Ear Hear. (2015) 36:82–91. doi: 10.1097/AUD.0000000000000080
203. Micula, A, Ning Ng, EH, El-Azm, F, and Ronnberg, J. The effects of task difficulty, background noise and noise reduction on recall. Int J Audiol. (2020) 59:792–800. doi: 10.1080/14992027.2020.1771441
204. Neher, T, Grimm, G, Hohmann, V, and Kollmeier, B. Do hearing loss and cognitive function modulate benefit from different binaural noise-reduction settings? Ear Hear. (2014) 35:e52–62. doi: 10.1097/AUD.0000000000000003
205. Yumba, WK. Cognitive processing speed, working memory, and the intelligibility of hearing aid-processed speech in persons with hearing impairment. Front Psychol. (2017) 8:1308. doi: 10.3389/fpsyg.2017.01308
206. Neher, T. Relating hearing loss and executive functions to hearing aid Users' preference for, and speech recognition with, different combinations of binaural noise reduction and microphone directionality. Front Neurosci. (2014) 8:391. doi: 10.3389/fnins.2014.00391
207. Souza, P, Arehart, K, and Neher, T. Working memory and hearing aid processing: literature findings, future directions, and clinical applications. Front Psychol. (2015) 6:1894. doi: 10.3389/fpsyg.2015.01894
208. Simpson, A, Bond, A, Loeliger, M, and Clarke, S. Speech intelligibility benefits of frequency-lowering algorithms in adult hearing aid users: a systematic review and Meta-analysis. Int J Audiol. (2018) 57:249–61. doi: 10.1080/14992027.2017.1375163
209. Miller, CW, Bates, E, and Brennan, M. The effects of frequency lowering on speech perception in noise with adult hearing-aid users. Int J Audiol. (2016) 55:305–12. doi: 10.3109/14992027.2015.1137364
210. Rallapalli, V, Ellis, G, and Souza, P. Effects of directionality, compression, and working memory on speech recognition. Ear Hear. (2021) 42:492–505. doi: 10.1097/AUD.0000000000000970
211. Humes, LE, Pichora-Fuller, MK, and Hickson, L. Functional consequences of impaired hearing in older adults and implications for intervention In: KS Helfer, EL Bartlett, AN Popper, and RR Fay, editors. Aging and hearing: Causes and consequences. Cham: Springer International Publishing (2020). 257–91. doi: 10.1007/978-3-030-49367-7_11
212. Sommers, MS, Spehar, B, Tye-Murray, N, Myerson, J, and Hale, S. Age differences in the effects of speaking rate on auditory, visual, and auditory-visual speech perception. Ear Hear. (2020) 41:549–60. doi: 10.1097/AUD.0000000000000776
213. Benichov, J, Cox, LC, Tun, PA, and Wingfield, A. Word recognition within a linguistic context: effects of age, hearing acuity, verbal ability, and cognitive function. Ear Hear. (2012) 33:250–6. doi: 10.1097/AUD.0b013e31822f680f
214. Pichora-Fuller, MK, Alain, C, and Schneider, BA. Older adults at the cocktail party In: JC Middlebrooks, JZ Simon, AN Popper, and RR Fay, editors. The auditory system at the cocktail party. Cham: Springer International Publishing (2017). 227–59.
215. Nixon, G, Sarant, J, Tomlin, D, and Dowell, R. Hearing aid uptake, benefit, and use: the impact of hearing, cognition, and personal factors. J Speech Lang Hear Res. (2021) 64:651–63. doi: 10.1044/2020_JSLHR-20-00014
216. Pichora-Fuller, MK, Mick, P, and Reed, M. Hearing, cognition, and healthy aging: social and public health implications of the links between age-related declines in hearing and cognition. Semin Hear. (2015) 36:122–39. doi: 10.1055/s-0035-1555116
217. McCabe, DP, Roediger, HL, McDaniel, MA, Balota, DA, and Hambrick, DZ. The relationship between working memory capacity and executive functioning: evidence for a common executive attention construct. Neuropsychology. (2010) 24:222–43. doi: 10.1037/a0017619
218. Gottfredson, LS, and Deary, IJ. Intelligence predicts health and longevity, but why? Curr Dir Psychol Sci. (2004) 13:1–4. doi: 10.1111/j.0963-7214.2004.01301001.x
219. Grenness, C, Hickson, L, Laplante-Lévesque, A, and Davidson, B. Patient-centred care: a review for rehabilitative audiologists. Int J Audiol. (2014) 53:S60–7. doi: 10.3109/14992027.2013.847286
220. Kestens, K, Degeest, S, and Keppler, H. The views and experience of audiologists working in Flemish hearing aid Centers concerning cognition within audiological practice. Am J Audiol. (2022) 31:338–47. doi: 10.1044/2022_AJA-21-00186
221. Vita, L, and Bruno, D. The challenge of detecting cognitive impairment in hearing-impaired individuals. Eur J Neurol. (2021) 28:1795–6. doi: 10.1111/ene.14802
222. Edwards, B. A model of auditory-cognitive processing and relevance to clinical applicability. Ear Hear. (2016) 37:85S–91S. doi: 10.1097/AUD.0000000000000308
223. Parmar, BJ, Rajasingam, SL, Bizley, JK, and Vickers, DA. Factors affecting the use of speech testing in adult audiology. Am J Audiol. (2022) 31:528–40. doi: 10.1044/2022_AJA-21-00233
224. Magnusson, L, Claesson, A, Persson, M, and Tengstrand, T. Speech recognition in noise using bilateral open-fit hearing aids: the limited benefit of directional microphones and noise reduction. Int J Audiol. (2013) 52:29–36. doi: 10.3109/14992027.2012.707335
225. Winkler, A, Latzel, M, and Holube, I. Open versus closed hearing-aid fittings: a literature review of both fitting approaches. Trends Hear. (2016) 20:233121651663174. doi: 10.1177/2331216516631741
226. Amlani, AM, and Schafer, EC. Application of paired-comparison methods to hearing aids. Trends Amplif. (2009) 13:241–59. doi: 10.1177/1084713809352908
227. Humes, LE. Cover story: verification and validation: the chasm between protocol and practice. Hear J. (2012) 65:8–10. doi: 10.1097/01.HJ.0000412693.87680.7e
229. Roter, D, and Kinmonth, A-L. What Is the Evidence That Increasing Engagement of Individuals in Self-Management Improves the Processes and Outcomes of Care? Chapter 25. In: The Evidence Base for Diabetes Care. 2nd edition. eds. W.H. Herman, A.L. Kinmouth, N.J. Wareham and R. Williams (New Jersey: Wiley). (2002).
231. Kochkin, S. Marketrak viii: reducing patient visits through verification and validation. Hear Rev. (2011) 18:10–2.
232. Almufarrij, I, Dillon, H, and Munro, KJ. Does probe-tube verification of real-ear hearing aid amplification characteristics improve outcomes in adults? A systematic review and Meta-analysis. Trends Hear. (2021) 25:2331216521999563. doi: 10.1177/2331216521999563
233. Stender, T, Appleby, R, and Hallenbeck, S. V V (2011). Its impact on user satisfaction. Hearing Review. October 29, 2011.
234. Whitmer, WM, Wright-Whyte, KF, Holman, JA, and Akeroyd, MA. Hearing aid validation In: GR Popelka, BCJ Moore, RR Fay, and AN Popper, editors. Hearing aids. Cham: Springer International Publishing (2016). 291–321.
235. Arehart, KH, Chon, SH, Lundberg, EMH, Harvey, LO Jr, Kates, JM, Anderson, MC, et al. A comparison of speech intelligibility and subjective quality with hearing-aid processing in older adults with hearing loss. Int J Audiol. (2022) 61:46–58. doi: 10.1080/14992027.2021.1900609
236. Ekberg, K, Timmer, B, Schuetz, S, and Hickson, L. Use of the behaviour change wheel to design an intervention to improve the implementation of family-centred Care in Adult Audiology Services. Int J Audiol. (2021) 60:20–9. doi: 10.1080/14992027.2020.1844321
Keywords: ageing, cognitive performance, hearing aids, auditory processing, compression speed, compression ratio, noise reduction
Citation: Windle R, Dillon H and Heinrich A (2023) A review of auditory processing and cognitive change during normal ageing, and the implications for setting hearing aids for older adults. Front. Neurol. 14:1122420. doi: 10.3389/fneur.2023.1122420
Received: 12 December 2022; Accepted: 02 June 2023;
Published: 20 June 2023.
Edited by:
Aaron Moberly, Vanderbilt University Medical Center, United StatesReviewed by:
Kathryn Arehart, University of Colorado Boulder, United StatesCopyright © 2023 Windle, Dillon and Heinrich. This is an open-access article distributed under the terms of the Creative Commons Attribution License (CC BY). The use, distribution or reproduction in other forums is permitted, provided the original author(s) and the copyright owner(s) are credited and that the original publication in this journal is cited, in accordance with accepted academic practice. No use, distribution or reproduction is permitted which does not comply with these terms.
*Correspondence: Richard Windle, cmljaGFyZC53aW5kbGVAbmhzLm5ldA==
Disclaimer: All claims expressed in this article are solely those of the authors and do not necessarily represent those of their affiliated organizations, or those of the publisher, the editors and the reviewers. Any product that may be evaluated in this article or claim that may be made by its manufacturer is not guaranteed or endorsed by the publisher.
Research integrity at Frontiers
Learn more about the work of our research integrity team to safeguard the quality of each article we publish.