- 1College of Health, The University of Montana, Missoula, MT, United States
- 2Universidad del Valle de México, Mexico City, Mexico
- 3Instituto de Ciencias de la Atmósfera y Cambio Climático, Universidad Nacional Autónoma de México, Mexico City, Mexico
- 4Department of Neurology, Geisel School of Medicine at Dartmouth, Hanover, NH, United States
- 5Department of Anatomy, Lake Erie College of Osteopathic Medicine, Erie, PA, United States
- 6Instituto Nacional de Pediatría, Mexico City, Mexico
- 7Instituto Potosino de Investigación Científica y Tecnológica A.C., San Luis Potosi, Mexico
- 8Sacramento Metropolitan Air Quality Management District, Sacramento, CA, United States
- 9Department of Mechanical and Aerospace Engineering, West Virginia University, Morgantown, WV, United States
- 10Interdisciplinary Statistical Research Unit, Indian Statistical Institute, Kolkata, India
Sustained exposures to ubiquitous outdoor/indoor fine particulate matter (PM2.5), including combustion and friction ultrafine PM (UFPM) and industrial nanoparticles (NPs) starting in utero, are linked to early pediatric and young adulthood aberrant neural protein accumulation, including hyperphosphorylated tau (p-tau), beta-amyloid (Aβ1 − 42), α-synuclein (α syn) and TAR DNA-binding protein 43 (TDP-43), hallmarks of Alzheimer's (AD), Parkinson's disease (PD), frontotemporal lobar degeneration (FTLD), and amyotrophic lateral sclerosis (ALS). UFPM from anthropogenic and natural sources and NPs enter the brain through the nasal/olfactory pathway, lung, gastrointestinal (GI) tract, skin, and placental barriers. On a global scale, the most important sources of outdoor UFPM are motor traffic emissions. This study focuses on the neuropathology heterogeneity and overlap of AD, PD, FTLD, and ALS in older adults, their similarities with the neuropathology of young, highly exposed urbanites, and their strong link with sleep disorders. Critical information includes how this UFPM and NPs cross all biological barriers, interact with brain soluble proteins and key organelles, and result in the oxidative, endoplasmic reticulum, and mitochondrial stress, neuroinflammation, DNA damage, protein aggregation and misfolding, and faulty complex protein quality control. The brain toxicity of UFPM and NPs makes them powerful candidates for early development and progression of fatal common neurodegenerative diseases, all having sleep disturbances. A detailed residential history, proximity to high-traffic roads, occupational histories, exposures to high-emission sources (i.e., factories, burning pits, forest fires, and airports), indoor PM sources (tobacco, wood burning in winter, cooking fumes, and microplastics in house dust), and consumption of industrial NPs, along with neurocognitive and neuropsychiatric histories, are critical. Environmental pollution is a ubiquitous, early, and cumulative risk factor for neurodegeneration and sleep disorders. Prevention of deadly neurological diseases associated with air pollution should be a public health priority.
1. Introduction
Chronic exposures to outdoor concentrations of PM2.5 above WHO air quality guidelines (annual 5 μg/m3) caused 6.4 million premature deaths and 93 billion days lived with illness in residents worldwide in 2019 (1). Exposures to traffic-generated pollutants, residency close to high-traffic roads, incomplete combustion emissions, firepit emissions, and in vitro experimental PM exposures of neural tissues, among other sources, have all been associated with extensive neural damage and increases in neurodegenerative diseases, including AD, PD, and ALS for the last two decades (2–17). Millions of US residents are exposed to wild forest fires and live near high-volume traffic roads and traffic-related air pollution (TRAP) (18, 19). Disadvantage populations, including minorities and low-income individuals, are exposed to high TRAP pollution (19, 20).
This study focuses on how incomplete combustion species and friction-derived and industrial-sourced nanoparticles reach neural tissues and damage target organelles in the nervous system; how these UFPMs and NPs travel in the brain and affect brain hubs with extensive communications and key roles in the integration of critical information, including sleep (21–23). For this study, we would be using either UFPMs and/or NPs, since our focus is on particle size, i.e., ≤ 100 nm.
The identification of the initial neuropathological stages of Alzheimer's disease (hyperphosphorylated tau and amyloid beta) (24) in 202/203 Metropolitan Mexico City forensic autopsies, with an average age of 25.4 ± 9.2 years, including 44 children with an average age of 12.89 ± 4.9 years, and the progression of the disease by the second and third decades of life, along with the concomitant development of PD and TDP-43 pathology in young urbanites, are at the core of our research efforts and our deep interest in comparing sleep disorders in patients with AD, PD, FLTD, and ALS, the involvement of aberrant neural proteins, and the presence of UFPM and NPs in sleep hubs in young highly exposed to air pollution cohorts (9–11, 24–34).
Populations that are exposed chronically to high concentrations of outdoor and indoor PM2.5 are at higher risk of developing early diagnostic and neurodegenerative hallmarks, and the fact that they overlap from the earliest ages strongly suggests that there is a common denominator affecting the protein neural structures. UFPM and NPs could be the causative agents in association with genetic, epigenetic, and other environmental variables, and damaged sleep hubs, and resulting sleep disorders could be early findings (35–43).
Millions of people worldwide are exposed to outdoor and indoor environmental fine particulate matter (PM2.5) and nanosize PM ≤ 100 nm [ultrafine particulate matter (UFPM) and industrial nanoparticles (NPs)]. Metal combustion and friction-derived UFPM and NPs are identified in brain organelles starting in utero and are directly responsible for intense oxidative stress, protein misfolding, protein aggregation, and fibrillation. AD, PD, and ALS are associated with exposure to air pollutants. Sleep disorders are strong predictors of fatal neurodegenerative disorders.
2. Particulate matter pollution, what is it? How do we measure it? Why nanosize PM is key?
Particulate matter (PM) consists of a mixture of microscopic solids and aerosols (liquid droplets) of different sizes and compositions found in the air. Different sizes of PM are based on their aerodynamic diameters: PM10 (mass of PM with an aerodynamic diameter <10 μm); fine or PM2.5 (particles <2.5 μm), and ultrafine particles (UFPM, with an aerodynamic diameter <0.1 μm). PM differs in chemical composition, size, shape, morphology, and air lifetime, depending mainly on their origin, which in turn can be primary or secondary. Particles emitted directly into the atmosphere are primary PM, while those formed within the atmosphere from a number of processes such as nucleation, condensation, and/or chemical reactions of gas-phase species are secondary PM, mainly gaseous air pollutants (44). PM10 and PM2.5 are our current indicators for PM pollution worldwide, particularly in highly polluted urban areas (i.e., Metropolitan Mexico City, Figure 1). Routine measurements of UFPM are neither common nor enforced, despite it being well-recognized that they can reach alveoli, circumvent primary airway defenses, and carry numerous toxic organic and inorganic compounds (44, 45).
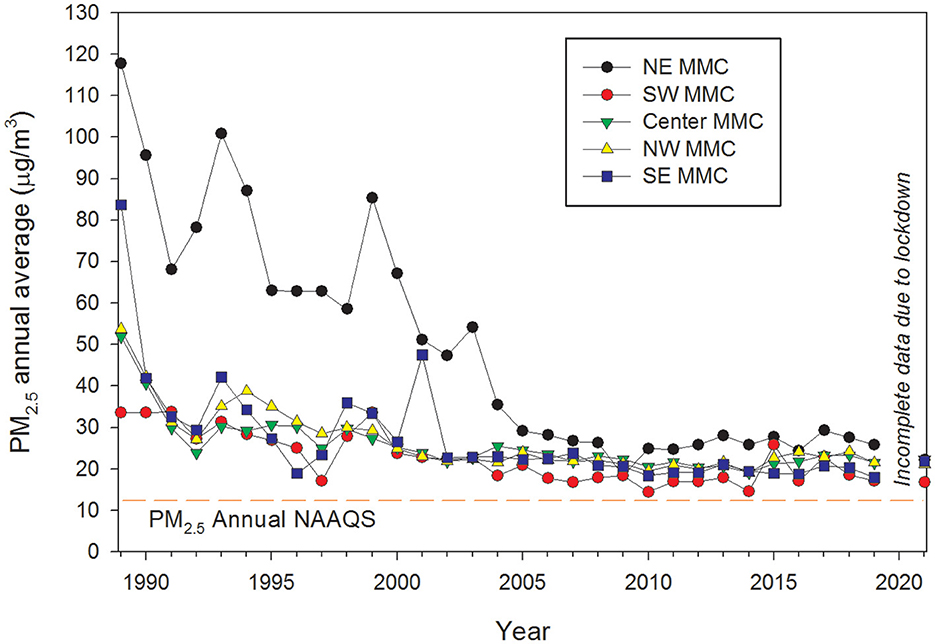
Figure 1. Time series trend of annual mean 24-h PM2.5 concentrations, averaged over 3 years, for five representative monitoring stations in MMC from 1990 to April 2020 and their comparison with the respective annual USEPA NAAQS. Data were processed and evaluated from measurements reported by the manual PM network of the Secretaría del Medio Ambiente del Gobierno de la Ciudad de México (SEDEMA) under a 6-day sampling schedule. Annual means from the years before 2004 were estimated from available information on PM10 since 1990 and the mean slope of the correlation PM10 vs. PM2.5 between 2004 and 2007. Source of data: http://www.aire.cdmx.gob.mx/default.php#.
Notably, while PM10 and PM2.5 ambient concentrations and their regulatory compliance with air quality standards are determined by mass-based methods, UFPMs have negligible mass, making them very difficult to measure. UFPMs are quantified by number concentration, which in many cases do not correlate with the mass concentrations reported as PM10 or PM2.5 (46–48). We currently do not have worldwide ultrafine particle matter regulations (21, 22). Although some countries have guidelines for UFPMs in terms of particulate number concentrations, their focus has been on short-term exposures in occupational environments and for specific materials, thus they do not apply to outdoor or indoor environments. Available measurement systems for UFPMs include condensation particle counters, electro-mobility spectrometers, diffusion battery counters, and photoelectric nucleus counters (44–49).
As road traffic and uncontrolled small combustion sources generate a significant number of nanoparticles, heavily polluted urban areas are suffering from strong UFPM problems (48). Metropolitan Mexico City (MMC) has experienced a dramatic increase in the number of vehicles in the last 20 years. Before 2000, CO and PM2.5 levels in MMC were among the highest levels registered in North America. However, due to actions to reduce traffic pollution, UFPM particle number concentrations (PNC) from the mid-2000s on, have been reduced to around 30,000 cm−3 (50). Using a non-linear correlation model between PNC, CO, and PM2.5 concentrations obtained from short-term monitoring studies, we have estimated that in the 1990s, PNC in MMC was around 300,000 cm−3 (50–54). Figure 2 shows the estimated annual average UFPMs number trend coupled with the CO annual median for MMC from 1989 to 2021 (50). We assumed that PM2.5 and CO could be reasonable proxies of vehicular emissions and incomplete combustion processes in the urban area. Typical particle number concentrations measured in 44 urban areas worldwide are in the order of ~5 × 103 to ~8 × 104 cm−3 with extremes above 1 × 105 cm−3 in China and India (48).
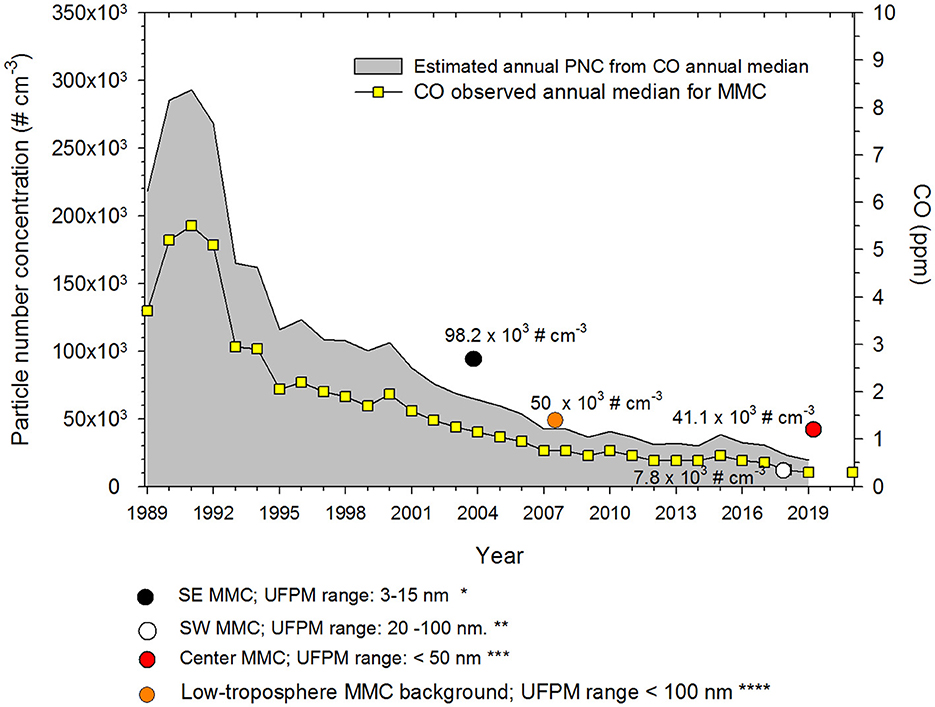
Figure 2. Trends of estimated PNCs and the associated annual medians of 1-h average CO for five representative monitoring stations of the MMC from 1989 to 2021. The colored circles in the figure correspond to the medians of PNCs measured by the authors referenced in (51*), (52**), (53***), and (54****). CO data source: http://www.aire.cdmx.gob.mx/default.php.
3. Nanoparticles, metals, metalloids, and plastics. How harmful? How early? Where do they go in the brain? How relevant are systemic inflammation and neuroinflammation in neurodegeneration and their association with air pollution?
Nanoparticles, regardless of composition or shape, go everywhere in the body, cross all biological barriers, and go through paracellular pathways, including tight junctions, adherens junctions, and cytoskeletons (55–62). Their small size facilitates their absorption capabilities and their passage through membranes (5, 63–71); red blood cells (RBCs) and white blood cells (WBCs) are very efficient transporters of UFPM and NPs because they can reach any place, including the brain (12, 71). Their portals of entry (55) are key to understanding the importance of inhalation and ingestion of NPs and their direct brain entrance through the olfactory region and access to the trigeminal nerve. The inhalation entry starts in the nasal mucosa and continues to the alveolar space and the enormous lung capillary bed with the transport of UFPM and NPs through RBCs and WBCs and their free systemic circulation transportation. The massive amount of NPs we ingest every day have direct access to the intestinal epithelium and submucosa, causing significant damage to the paracellular structures and allowing direct entry of NPs to the enteric nervous system (ENS) (72).
The neurovascular unit (NVU) (73), defined as a complex functional and anatomical structure integrated by endothelial cells, capillaries, arterioles, a basal lamina covered by pericytes, smooth muscle cells, and neural cells including neurons, interneurons, astrocytes, and an extracellular matrix, is a direct UFPM/NP target, a critical observation explaining the extensive capillary and small arteriole vascular damage starting in utero and in childhood upon PM air pollution exposures (9, 10, 12, 55). As described by Schaeffer and Iadecola (73), NVU damage, regardless of the source, has serious effects on neurovascular regulation and coordination of vascular responses to central and peripheral signals, which are critical to maintaining brain homeostasis. NVU damage predicts neurodegeneration (21, 55, 72–74).
The detrimental impact of NPs on the brain includes high production of reactive oxygen species, neural inflammation, depletion of anti-oxidative enzymes, DNA damage, apoptosis, structural cell damage, including organelles, nuclei, tight junctions, adherens junctions, endothelial damage, and dysfunction (5, 55, 59, 62, 64, 70, 75–78).
Particularly relevant to this study is the fact that UFPM/NPs are very effective in their capacity to aggregate, conglomerate, and produce protein folding, destabilization, and fibrillation (5, 61, 63, 65, 67, 69, 70, 79–82). John et al. (81) referred to large nanostructures of ≥20 nm affecting the kinetic peptide aggregation, thus size and shape matter. They also discussed how NPs serve as a surface for the adsorption of peptide monomers and facilitate nucleation to oligomers and fibril formation (81). Mohammad-Beigi et al. (82) discussed how α-synuclein undergoes interactions with NPs and how these interactions can be prevented by the characteristics of the protein corona acquired during the exposure of NPs to serum proteins. When α-synuclein and polyethylenimine-coated carboxyl-modified polystyrene NPs (PsNPs-PEI) interact, the NP surface promotes the primary nucleation step of amyloid fibril formation, thus key to pathological fibrillation, serum proteins modulate the complex interplay between NPs and amyloid proteins (82).
NP/UFPM interactions with brain cells are complex, and variables such as the nature of the protein corona, bioavailability, biodistribution, size, shape, charge, composition, cell and organelle targets, and certainly portal of entry are all impacting the extent and type of brain damage.
An interesting and concerning factor in UFPM composed of iron (magnetite and maghemite) is precisely their magnetic properties (5, 83–85). In the study by Shu et al. (85), the superparamagnetic NPs could respond to an external magnetic field, and magnetic NPs could be seen setting down in the magnetic pole regions (see Figure 4d of that study). This magnetic cell settling does, in fact, occur in MMC residents, as we have documented the phenomenon in electron micrographs [(55), Figure 3B]. The issue is more than a sporadic finding; MMC brains contain significant concentrations of magnetic NPs measured as saturation remanent magnetization (SIRM), being highest in the cerebellum (10). The cerebellum in young MMC residents shows extensive vascular pathology and cerebellar endothelial erythrophagocytosis (50) and significant atrophy by volumetric brain MRI in young MMC residents (39).
Systemic inflammation and endothelial dysfunction are very relevant to air pollution exposures, as shown by our laboratory in Mexico City children (86), along with nasal inflammation, DNA nasal epithelial damage (87–90), and CSF inflammation (43). Systemic inflammation and endothelial dysfunction have been described in 295 pregnant women (91) with strong associations between increases in soluble vascular adhesion molecule-1 (sVCAM-1) levels for each 10 μg/m3 increase in PM10 concentration, strongly suggesting that inflammation and endothelial dysfunction have a key role in modulating the detrimental effects of air pollution exposure during pregnancy, as shown recently in our laboratory with the extensive presence of nanoparticles of industrial origin in placentas of all ages and brain fetal tissues (12).
Oxidative stress and inflammation are common denominators of particulate matter (PM) air pollution exposures (92), including PM containing polycyclic aromatic hydrocarbons (PAHs) at low exposure settings. Occupational exposures are equally important for both systemic and neural inflammation and neurodegeneration (93, 94). Orysiak et al. (93) described a significant increase in proinflammatory cytokines in firefighters, along with respiratory inflammation, a piece of information that is very significant given the massive exposure of the US population to forest fires (18) and traffic air pollution (19). Thus, the report of Huang et al. (94) on neuroinflammation in the 2001 World Trade Center (WTC) responders is not a surprise, nor is the increment in suicides among the same responder population (95–97). The expected responses of the highly PM-exposed WTC responders were precisely what researchers are publishing 22 years later and what we commented within hours of the tragic event: acceleration of neuroinflammation, neurodegeneration, and suicides, as we see in Mexico City residents, more pronounced in APOE4 carriers, and associated with dose and routes of exposure key for both WTC responders and MMC residents (9–12).
Monitoring systemic inflammation in children should be a health priority since ambient air pollution impacts inflammatory responses from childhood (86, 98). Certainly, UFPM/NPs play a key role in both systemic and neural inflammation (12, 14, 16, 17, 21, 45, 50, 55, 62, 64), and diesel and Fe-NPs cause significant damage to neural cells under experimental conditions (62, 64). The issue also applies to industrial NPs consumed worldwide in massive amounts, i.e., titanium oxide NPs (99). Rolo and coworkers (99) have an excellent review of the TiO2-NPs in foods causing oxidative stress, cytotoxicity/apoptosis/cell death, inflammation, cellular and systemic uptake, genotoxicity, and carcinogenicity, and although the authors made a plea to support limiting the use of TiO2-NPs in food, we are aware as toxicologists that the food industry will be reluctant to follow-up on the recommendations. Thus, although the literature supports the multiple pathways UFPM/NPs are capable of causing systemic and neural damage through oxidative stress, neuroinflammation, mitochondrial function, neurodegeneration, via excessive activation of cellular prion protein signaling, hippocampal-impaired neurogenesis and synaptic plasticity, abnormal peptidomic responses, apoptosis, and necrosis (100–105), we still do not have NPs and UFPM regulations in the United States, and we need to establish clear correlations between PM exposures, neurodegeneration, and inflammation (106–109).
4. Development of Alzheimer's and Parkinson's diseases and TDP-43 pathology in children and young adult MMC residents. The diagnostic neural abnormal proteins are present and overlap from childhood and are key for the diagnosis of early sleep disturbances
In 2002, we described the association between the neuropathological hallmarks of Alzheimer's disease and air pollution exposures in our laboratory, stating: Neurodegenerative disorders such as Alzheimer's may begin early in life with air pollutants playing a crucial role (37). Two decades later, we have robust evidence to support this statement in populations exposed to high levels of PM2.5 and UFPM/NPs. Our studies demonstrate the development of AD, PD, and TDP-43 pathology starting in childhood, and it is corroborated clinically with the progressive cognitive deterioration, abnormal gait and equilibrium, brainstem-evoked auditory potentials, olfactory deficits, sleep abnormalities, and brain MRI cortical, subcortical, and cerebellar atrophy in seemingly healthy individuals (36–42). Low CSF concentrations of amyloid β 1 − 42 and BDNF differentiate children exposed to MMC air pollution from low pollution controls (43).
We have identified p-tau, the presence of Aβ and α-synuclein, and abnormal TDP-43 expression in 202 MMC forensic autopsies from residents who died in accidents, homicides, and suicides aged 25.3 ± 9.2 years (9, 10). Extensive, early, and progressive neurovascular unit damage and key organelle ultrastructural pathology were associated with metal- and metalloid-rich UFPM/NPs, making solid UFPM/NPs an agent for brain pathology in MMC subjects (9–12).
Figure 3 illustrates the two key AD neuropathology markers, namely, hyperphosphorylated tau and beta-amyloid, in MMC residents per decade, including 44 children (9). We thoroughly studied the extra neural tissues and confirmed there were no gross and/or light microscopy abnormalities.
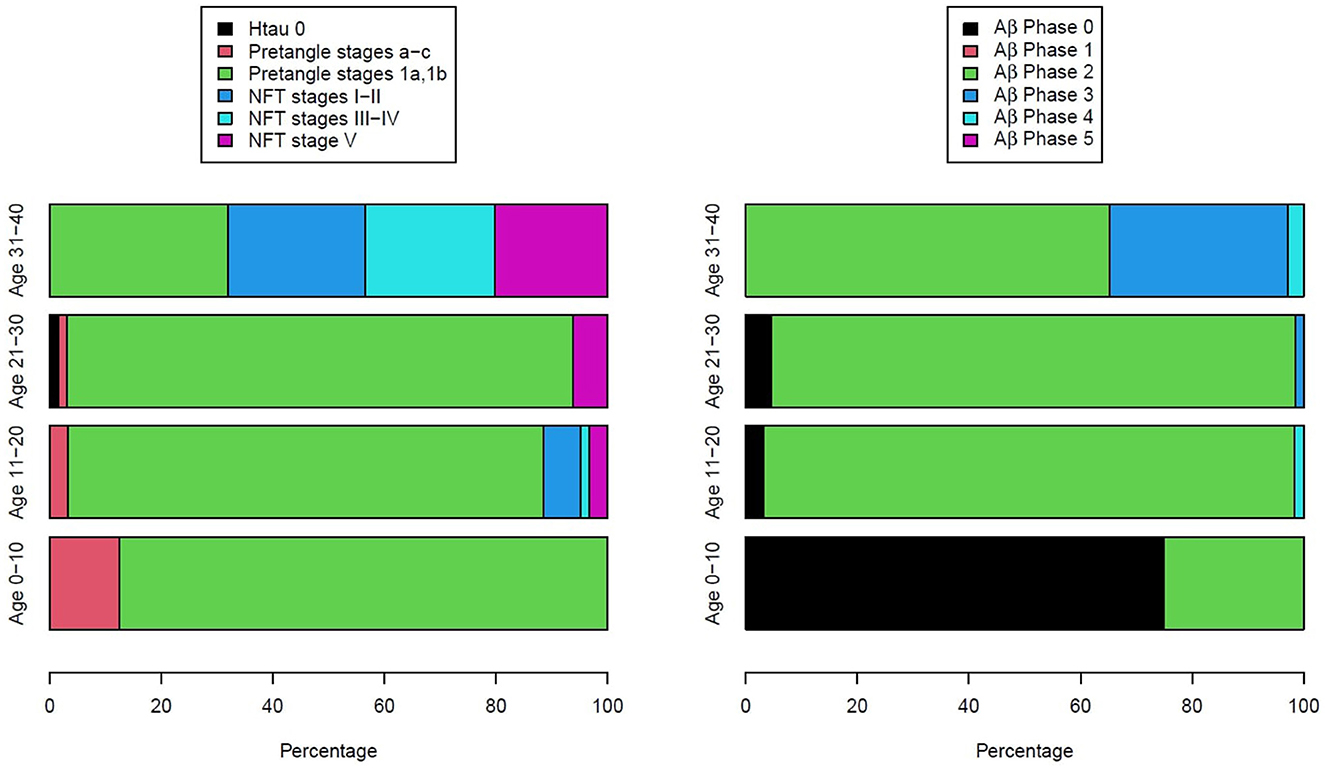
Figure 3. Two hundred and three forensic autopsies were staged for Alzheimer's disease using p-tau and Aβ1−42 (9). Subjects were 25.4 ± 9.2 years old and causes of death were related to car accidents, homicides, and suicides; 202/203 had AD pathology, including the youngest subject, an 11-month-old baby.
As seen in Figure 3, every child had h-tau pre-tangle stages in the 1st decade of life, and by the 2nd decade, we documented neurofibrillary (NFT) tangles I–V (24). Subjects in the 4th decade were clearly in NFT I–V stages, and pre-tangle stages could no longer be identified. In contrast, Aβ progressed slowly and remained in the early phases. Interestingly, in our autopsy studies (9, 10), apolipoprotein E allele 4 (APOE4) carriers of the strongest Alzheimer's disease genetic risk factor (110–113) had higher AD Braak stages and the highest risk for suicide associated with lower cumulative exposures to PM2.5 vs. APOE3 carriers. A finding in keeping with the literature regarding the higher risk of carrying two copies of ε4 allele increasing the AD risk up to 15-fold versus an APOE3 carrier in European ancestry subjects (114). APOE is a key protein in the equation of AD risk, neuroinflammation, oxidative stress, and metals (35, 112–114). The study by Tcw et al. (115), relating local APOE haplotype and the ε4-specific amino acid changes to important deficits in lipid metabolism dysregulation, glial activation, and inflammation, is of considerable interest in the setting of air pollution (9).
The overlap of AD, PD, and TDP-43 pathology is remarkable in young MMC residents as it is extraordinarily similar to the mixed protein pathologies described in elderly demented patients diagnosed with AD, FTLD, LBD, PD, ALS, and cerebral amyloid angiopathy (CAA), white matter rarefaction (WMR) pathology, and in the younger than 60-year patients who are AD demented (25–34, 116–119). Metha and Schneider (118) illustrated the overlapping neuropathology in a Venn diagram, which was further discussed by Jellinger (119, 120). It is clear that in elderly populations, AD is a heterogeneous disease, and co-pathologies (119), including LBD and TDP-43 pathology, and cerebrovascular lesions, are critical for the clinical picture, imaging, and laboratory results (25, 119–128). Figure 4 illustrates the aberrant neural protein overlap in MMC young without extra neural pathology (10).
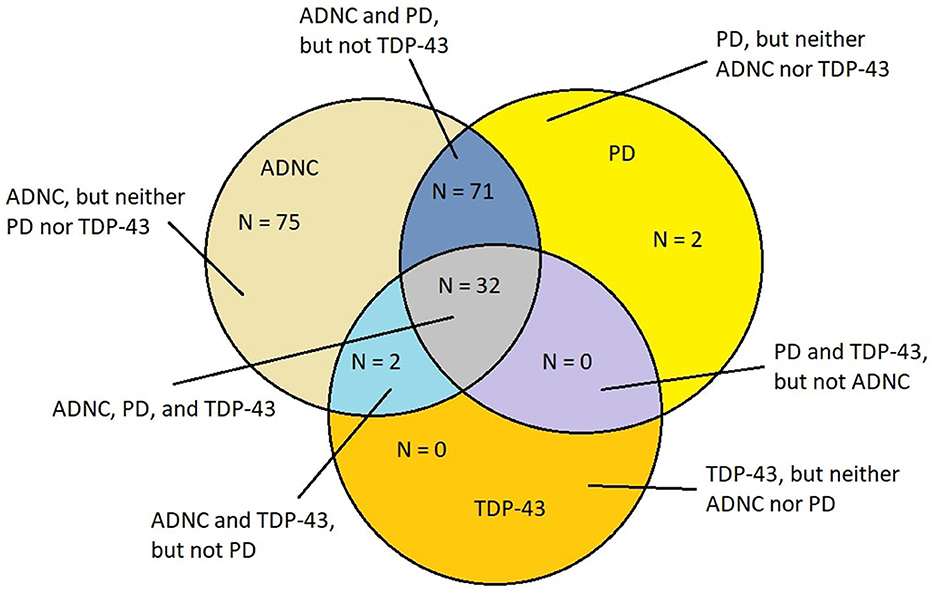
Figure 4. Venn diagram showing the overlap of neurodegenerative fatal diseases in a cohort of 186 MMC residents with an average age of 27.3 ± 11.8 years (10). Alzheimer's disease neuropathology changes (ADNC) (h-tau and Aβ) were present in each case.
All major neuropathological hallmarks of AD, PD, FTLD, and ALS are identified in young urbanites, from brainstem p-tau and diffuse amyloid plaques in an 11-month-old baby to extensive cortical p-tau in carriers of APOE4 alleles in 15-year olds. Common findings in MMC residents include p-tau in substantia nigrae and lack of nuclear TDP-43 in cortical motor neurons, lower motor neurons for cranial nerves III, V, and XII, and cervical motor neurons in teens and young adults (9–11). Hyperphosphorylated tau is definitely the major aberrant protein in highly exposed air pollution young urbanites (36). Figure 5 shows the overlap of aberrant neural protein in MMC young residents (10), compared to elderly subjects in the key work of Karanth et al. (25).
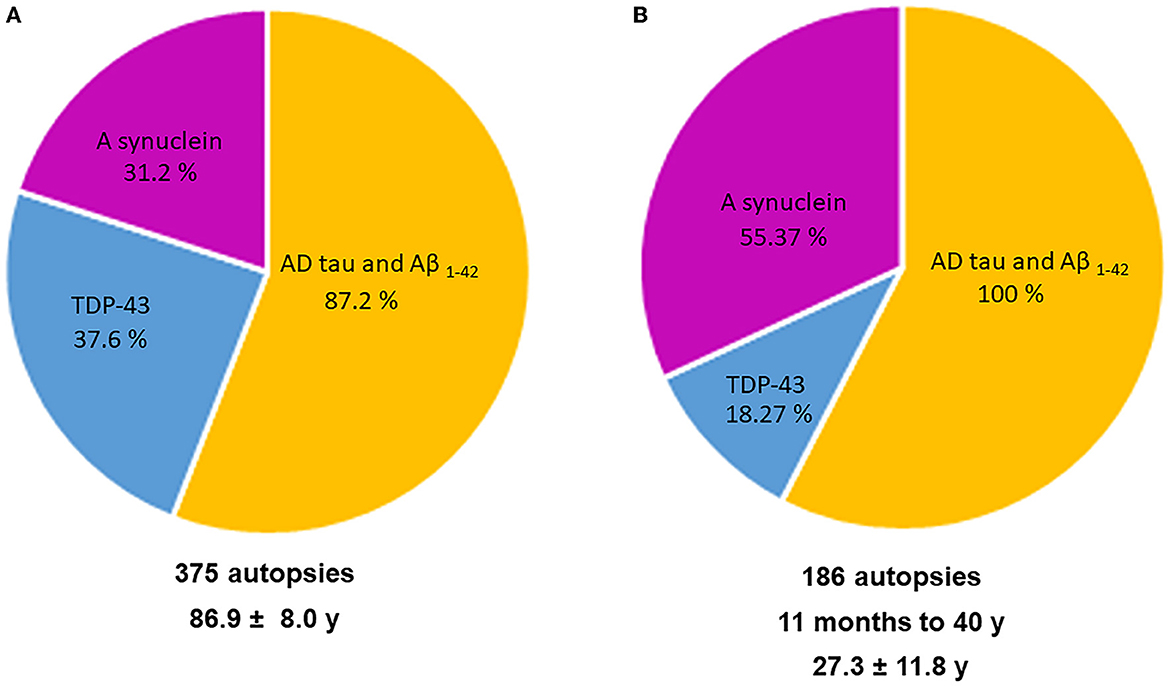
Figure 5. (A, B) A comparison in aberrant neural proteins between the young MMC 186 autopsy cohort (10) and Karanth et al. (25) 375 autopsies with an average age of 86.9 ± 8.04 years including subjects with normal cognition, mild cognitive impairment (MCI), impaired (but not MCI), and dementia.
Cerebrovascular pathology involving small and large cerebral vessels, with lesions ranging from gross and microscopic infarcts, atherosclerosis, and arteriolosclerosis, is commonly attributable to aging and independently associated with a higher risk of AD in elderly subjects (116, 118–120, 128). Strikingly, we have described extensive brain capillary and arteriole endothelial pathology and abnormal NVU in MMC dogs, children, and fetal brains in weeks 12–15 (12, 129). In dogs and children, capillaries displayed abnormal tight junctions—a critical component of the NVU (129), decorated with UFPM/NPs, and white matter extensive perivascular damage with leaking capillaries and arterioles displaying extravascular lipids and erythrocytes. The endothelial basement membranes are thickened and display beta-sheet structures, and the perivascular glial sheet is focally absent. NPs (10–48 nm) are localized in endothelial cells (EC), pericytes, and across the basement membranes. Endothelial damage associated with NPs is detected very early and worsens with age in children and teens with high PM exposures (Figure 6) (129).
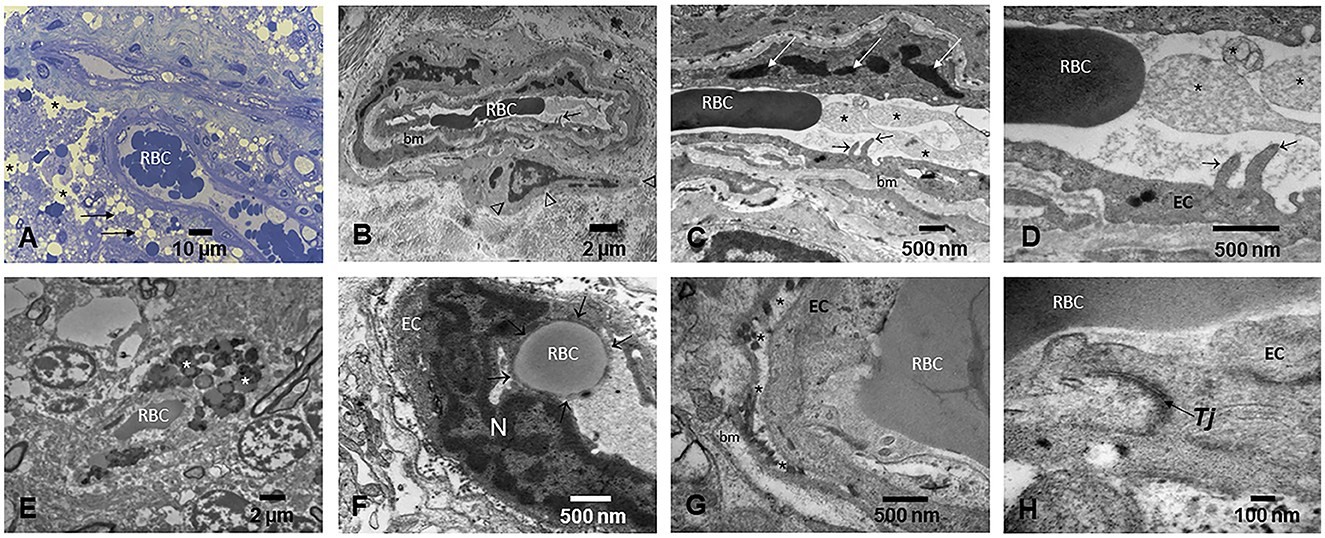
Figure 6. Light and electron microscopy of the brain in different anatomical locations in MMC young residents. (A) Thirteen-year-old girl's olfactory bulb showing small blood vessels with red blood cells (RBC) in the lumen and prominent endothelial cells. A significant number of perivascular vacuolated foamy cells (short arrows) and extensive areas of vacuolated neuropil (*) are observed. (B) A frontal blood vessel showing two luminal RBCs and prominent endothelial extensions into the lumen (short arrow). The basement membrane (bm) is thickened, and a pericyte (arrow heads) is identified. (C) The activated endothelial cell sends filopodia into the lumen (short arrows), while the lumen is occupied by ghost cell fragments (*), seen in higher magnification in (D). (E) Small frontal blood vessel containing one single luminal RBC and extravascular numerous lysosomal structures containing lipids and NPs (*). (F) Cerebellar blood vessel showing a typical RBC endothelial phagocytosis. The RBC is sequestered by the EC and surrounded by EC cytoplasm (short arrows). (G) Small blood cortical vessel with luminal RBCs closely in contact with the EC. The EC basement membrane is detached from the cell (*), and an accumulation of lysosome-like structures is seen. The basement membrane (bm) shows focal thickening. (H) A close-up of a tight junction Tj—a key structure in brain endothelial cells—showing poorly defined integrity (arrow).
We strongly suggest that neural abnormal protein overlap could be explained by the presence of UFPM/NPs in critical hubs with portals of entry, emission sources, cumulative exposures, size, shape, surface charge, chemical composition, biomolecular corona proteins, target organelles, cellular toxicity, axonal anterograde and retrograde transport, trans-synaptic movements, and a number of genetic (i.e., APOE4 carrier status) and environmental factors (in utero exposures), comorbidities, etc., accounting for the neural damage and the heterogeneity of neurodegenerative diseases (12–17, 25–34, 113, 116–129).
The nanosize PM is composed of metals including Fe, Ti, Hg, Cu, Al, and Bi; post-transition metals, i.e., Al and Pb; alkaline earth metals, i.e., Ba; and non-metallic chemical elements such as Si are identified in every organelle in neurons, glial cells, microglia, and endothelial cells in Mexico City residents (10). Mitochondria and endoplasmic reticulum (ER), as well as the mitochondria-ER membrane contacts (MERC), are key NPs targets, and abnormal MERCs are common in highly exposed subjects (55, 56, 129). UFPM/NPs are also localized in the nuclear matrix—in close contact with heterochromatin—and nuclear pores. The outstanding accumulation of NPs in endolysosomes and specific structures like neuromelanin has great relevance in targeted neurodegenerative processes, including PD (Figure 7) (10, 55, 56, 130).
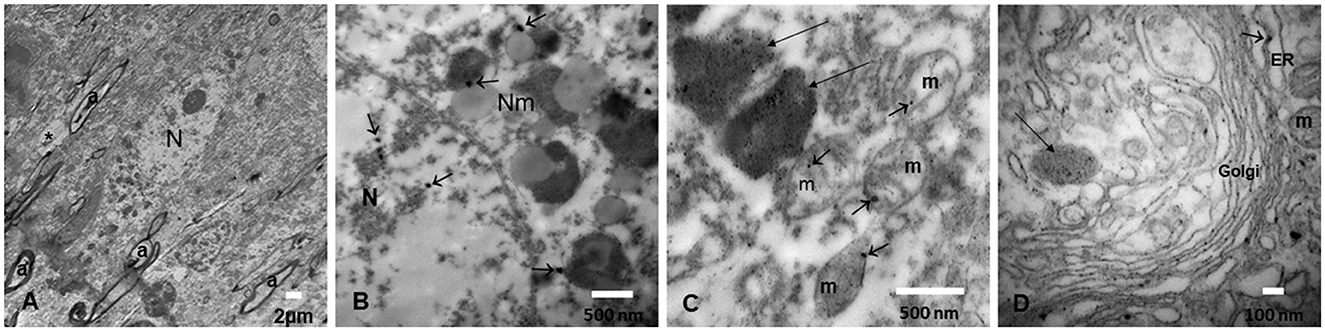
Figure 7. (A) Locus coeruleus (LC) neuron surrounded by loose neuropil (*) and myelinated axons (a). (B) An LC neuron with neuromelanin (Nm) structures containing nanoparticles (arrows) and similar NPs identified inside the nucleus (N). (C) Numerous NPs are identified both in large endolysosomal structures (long arrows) and in mitochondria (m) (short arrows). (D) The Golgi apparatus is a target of NPs, as well as the endoplasmic reticulum dilated structures (ER) (short arrows) and the lysosomal structures (long arrows).
The spectrum of metals and metalloids is critical for brain targets. We are identifying Fe-based, highly magnetic UFPM along with metals commonly associated with electronic waste, such as elongated TiO2 NPs (131). Shredding of e-waste is an extensive source of NPs in the United States (131), and very high concentrations of lead, for example, 2.9 μg-lead m3, are common 1.8 m away from the shredder operator, with extensive metal surface contamination reaching up to 250,000 particles cm3 with fine PM2.5 up to 171 μg m3, and both failing to return to background levels after 40 min of inactivity, as described by Ceballos et al. (131). As stated by Frazzoli et al. (132), the aggressively extractive advanced technology industry thrives on the intensive use of non-renewable resources and hyper-consumeristic culture and unfortunately, the health impact on the brain is detrimental. Figures 8, 9 show the metal and metalloid profiles in individual UFPM/NPs in neural and vascular cells analyzed by energy-dispersive X-ray spectrometry (EDX).
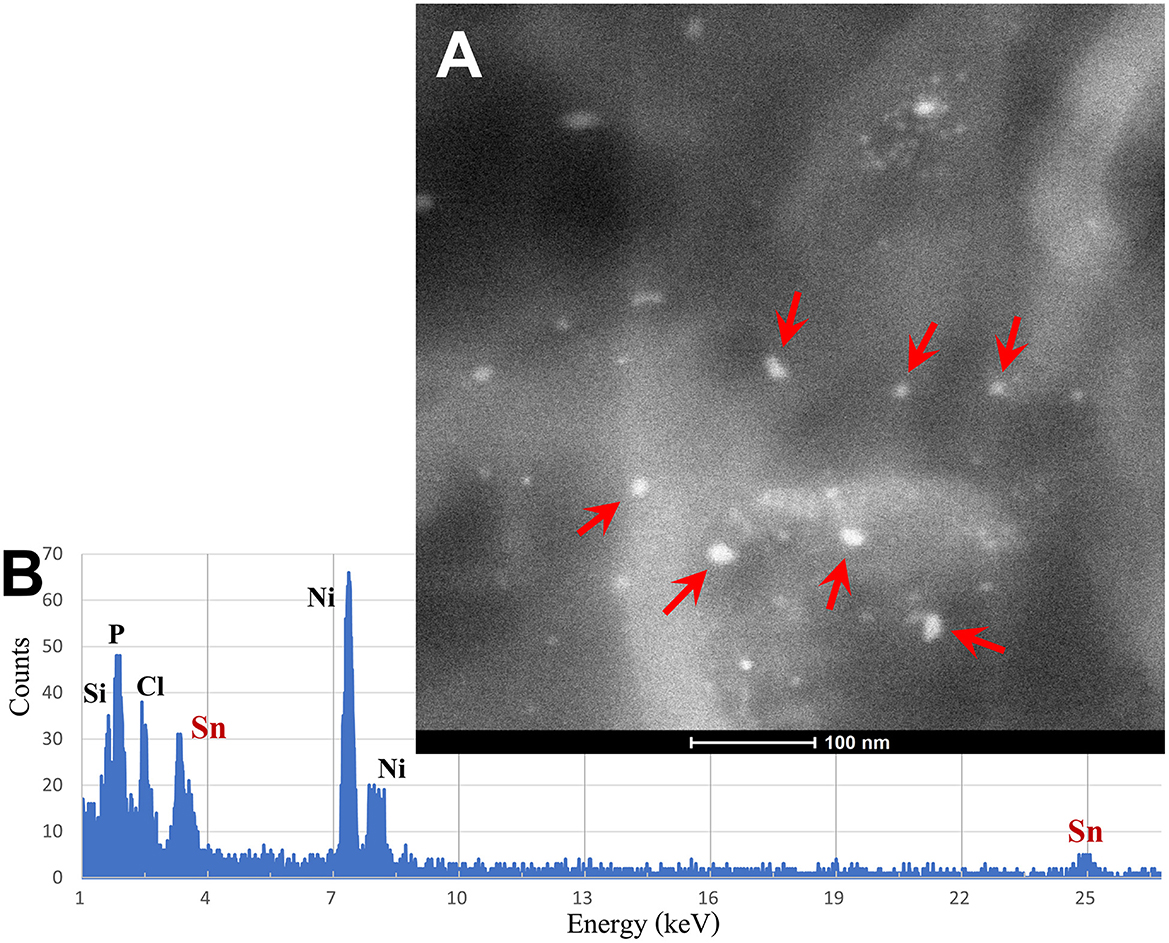
Figure 8. (A) Using the transmission electron microscopy (TEM) Z-contrast technique, metallic nanoparticles are documented in brain tissues. Only the nanoparticles marked with red arrows are Sn-NPs, while the rest are Fe-NPs. (B) The presence of Sn-NPs is verified through the acquired energy-dispersive X-ray spectrometry (EDX) that shows the tin metal (Sn) peak.
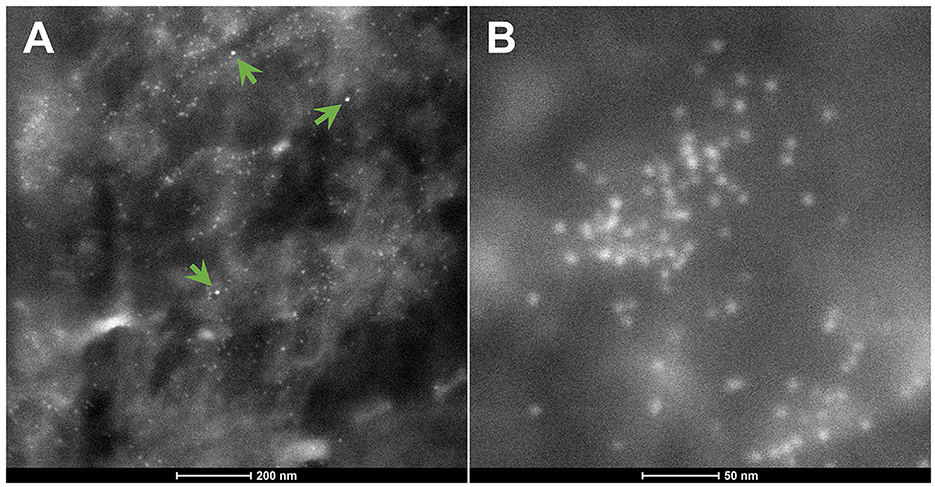
Figure 9. (A) The green arrows indicate three Hg-NPs that stand out from surrounding iron NPs. (B) An area of Fe-NPs. These NPs are magnetic.
UFPM/NPs in targeted organelles with critical functions, including the assembly of proteins, lipid synthesis, regulation, transportation, clearing of damaged organelles via lysosomal degradation, inter-organellar communication, Ca2+ storage, transport and signaling, apoptosis, autophagy, stress responses, and formation and activation of inflammasomes, are at the core of the nanoneuropathology, as shown by the myriad of interesting studies focusing on alterations of mitochondria, MERCS, ERs, mitochondria-lysosome connections, neuromelanin, and nuclear pores (133–142).
Neurodegenerative diseases are heterogeneous, multisystem disorders with multiple abnormal proteins frequently associated with cognitive impairment and sleep disorders. The heterogeneity includes clinical-brain imagen variants that complicate diagnoses and putting forward we still have limitations for clinical and neuropathology diagnostic criteria (25–34, 116–129). AD, PD, and TDP-43 pathology start in childhood in populations with high exposures to PM2.5 (for this review, concentrations above the USEPA annual standards of 12 μg/m3) and UFPM and NPs.
5. Neurodegeneration spectrum heterogeneity, quadruple neural abnormal proteins, and sleep disorders
At the core of this study are the neuropathology spectrum heterogeneity and the overlap in neuropsychiatric outcomes, including sleep disorders (25–34, 117–128, 143–166). There is consensus that for specific sleep disorders, i.e., rapid eye movement sleep behavior disorder (RBD), the association with synucleinopathies, i.e., PD, LBD, or multiple system atrophy (MSA), is supported (143–146, 152–160, 162–166). RBD is regarded clinically as preceding the appearance of motor symptoms and cognitive decline by several decades and the overlap of neurodegenerative diseases is certainly present as magnifically shown by Boeve and collaborators (26). LBD, LBD and AD, MSA, AD, and progressive supranuclear palsy (PSP) were diagnosed at autopsy in patients with a clinical diagnosis of PD, cognitive impairment, and autonomic dysfunction (29). Further support for evidence of dopaminergic and cholinergic system alterations in neuroimaging is present in the literature (143, 144).
There is a complex etiopathogenesis involved in the association of sleep disorders and diseases such as PD. As discussed by Mizrahi-Kliger et al. (147), patients with PD exhibited at least four distinct pathways to explain their sleep problems: (i). a path directly associated with their PD synucleinopathy with regional involvement, (ii). medical therapy, (iii). degeneration of non-dopaminergic cells altering the circadian rhythm, and (iv). damage to brainstem dopaminergic neurons and its effect on the basal ganglia (147). Thus, common sleep complaints are linked to complex etiopathogenesis in the context of synucleinopathies, along with poor sleep quality associated with depression, PTSD, mood disorders, and excessive daytime sleepiness (148, 149, 156).
How much should we be concerned about sleep complaints and neurodegeneration? The answer is that we should be concerned depending on the patient, age, gender, clinical history, and how many risk factors, including environmental factors, are impacting neurodegenerative processes. The issue is relatively easy when we deal with a diagnosed elderly patient with AD or FTLD (151, 156, 157, 161), but not if we have a young adult resident in a polluted city or with occupational exposures (36).
It is in the younger adult population that learning about the etiopathogenesis of sleep disorders in AD, FTLD, PD, and movement disorders is helpful (24–34, 161, 167–169). Standlee and Malkani (169) underlined the mechanisms by which movement disorders are associated with sleep and circadian rhythm disruption, sleep fragmentation, insomnia, and excessive daytime sleepiness. It is worth emphasizing, as these authors did (169), the extensive involvement of brainstem nuclei regulating sleep and wakefulness in neurodegenerative processes.
At this time, it is unclear if sleep disturbances precede the common clinical neurodegenerative symptoms (i.e., cognition deficits) or if the sleep problems are some of the initial, early manifestations of neurodegenerative processes. Sleep complaints and neurodegeneration may be bidirectional. The sleep literature has addressed the abovementioned concerns in many different ways. For example, Zamore and Veasey (170) addressed chronic sleep disruption and neural damage, focusing on key variables, including duration and type of sleep disruption, age at which sleep loss occurs, neuronal populations responding to the injury, and the presence of genes involved in neurodegenerative processes. Sleep disruption impacts cognitive targets, such as episodic memory and sustained vigilance, pre- and post-synaptic impairment, the release of inflammatory cytokines and chemokines from microglia, and in transgenic 3×Tg-AD mice models, daily sleep-wake rhythm chronic fragmentation, increases in brain amyloid-beta (Aβ) levels, and neuroinflammation (170, 171). Grigg-Dambererger and collaborators (172) discussed acceleration of mild cognitive impairment (MCI) and dementia in patients with sleep-wake disorders and the removal of Aβ in non-rapid eye movement stage 3 sleep and fragmented or insufficient sleep leading to accumulation of abnormal neural proteins in preclinical stages. Burke et al. (173) explored the association between sleep disturbance and brain volumes in 1,533 subjects (cognitively normal/cognitively impaired or demented) using a single question from the Neuropsychiatric Inventory Questionnaire (NPI-Q): “Does the patient awaken you during the night, rise too early in the morning, or take excessive naps during the day?” The sleep disturbance was rated in a binary fashion (yes/no). Subjects with a yes answer to the NPI-Q question had a lower total brain, hippocampal volume and frontal and temporal lobe gray matter volume. The authors concluded as follows: These findings suggest that disrupted sleep is associated with atrophy across multiple brain regions and ventricular hydrocephalus ex vacuo. We will add that since the brain MRI findings take years to evolve, it is possible that the atrophic brain changes preceded the sleep disturbances. The direct relationship between subcortical wake-promoting damaged neurons and sleep phenotypes has been described by Oh et al. (174), in patients with AD and progressive supranuclear palsy (PSP). In fact, in 19 subjects, aged 70 ± 7.7 years at their demise, neuronal counts in three wake-promoting nuclei, namely, noradrenergic locus coeruleus [LC], orexinergic lateral hypothalamic area [LHA], and histaminergic tuberomammillary nucleus [TMN], were correlated with decreased homeostatic sleep drive. The authors suggested subcortical wake neurons correlate with sleep phenotypes in a number of neurodegenerative diseases, an observation that has practical and immediate applications (174).
On the other side of the relationship between sleep disorders and neurodegeneration, we find the literature on the brain impact of obstructive sleep apnea (OSA) and intermittent hypoxia as risk factors for preclinical AD and the incidence and progression of cognitive deficits (175–180). There is strong data on OSA producing intermittent hypoxia and sleep disruption and the observation that patients with OSA have higher serum levels of amyloid-beta and total tau and neuronal-derived Aβ and tau exosomes, going hand in hand with changes in sleep architecture (175, 176). There is strong evidence for the role of OSA as a risk factor for cognitive deficits (177) and the risk of developing or having OSA is significantly higher in patients with MCI or who are demented (178). It is also clear that several pathways are involved in neuropathological processes, including dysregulation of the orexinergic system and cerebral β-amyloid metabolism (179), major changes in CSF production, circulation, and glymphatic system abnormalities, which are crucial for the removal of metabolic waste (180). The American Thoracic Society workshop on the link between obstructive sleep apnea and neurocognitive impairment (181) concluded there is a strong biological plausibility but insufficient data to prove bidirectional causality of the associations between OSA and aging brain pathology. Thus, future research needs to address sleep disorders, oxidative stress, and accelerated brain aging (182, 183).
The WHO Mental Health Action Plan 2013–2030 emphasizes depression, affecting 4% of the population, ~280 million people, as an important cause of disability worldwide (184). Depression should be considered in the setting of sleep–wake disorders, anxiety, stress, burnout, and suicide (185, 186). Insomnia and mental health conditions coexist among US college students: depressed students (adjusted odds ratio, 9.54; 95% CI, 4.50–20.26) had significantly higher odds of insomnia, which were also significantly higher among employed students (odds ratio, 2.10; 95% CI, 1.05–4.18) (187). Sleep and mental disorders are related, and in the case of major depressive disorder (MDD), insomnia seems to be a comorbid disorder (188). The relationship between depression and sleep is highly concerning for sleep physicians given the association between depression and neuropathology (189). Villela Nunes and coworkers (189) examined the autopsies in 741 Brazilian non-demented individuals with an average age of 72.2 ± 11.7 years and major depressive disorder (MDD) (7.3%), late-life MDD (LLD) (10.8%), and depressive symptoms (DS) close to death (22.7%). Remarkably, all three correlated with small vessel disease: LLD and DS with brain infarcts and LBD, and DS with beta-amyloid plaques and amyloid angiopathy (189). Therefore, in fact, depression could be considered a premorbid neurodegeneration in elderly people (189), and it is associated with insomnia in young individuals (187).
The issues of sleep outcomes, sleep deprivation, sleep spindles, and neurodegeneration are critical to the bidirectionality of the relationship (190–194). The relationship between fast-frequency sleep spindles, aging, AD, and glial activation is very interesting and opens up the possibility of establishing an early marker associated with microglia dysfunction, synaptic loss, p-tau, and memory impairment. Sleep spindle deficits are a good example of the opportunity of using sleep variables as early AD biomarkers in aging and as trackers of AD progression (190–192). Furthermore, slow oscillations, sleep spindles, and their coupling during non-REM sleep are useful in experimental AD mouse models and could apply to patients with AD as key biomarkers and as guides to identify translationally relevant biomarkers and early intervention strategies to prevent or delay AD progression (193, 194).
Most of the associations between neurodegenerative processes have been done with particulate matter, especially UFPM and NPs, due to their capacity to travel to the brain and be localized in every organelle and cellular compartment (4, 5, 9–11, 16, 21, 22, 44, 137); however, the atmospheric chemistry is very complex and has to be seen as a continuum connecting emissions through chemistry and transport, as discussed by Finlayson-Pitts (195). Toxicologists and atmospheric chemistry researchers are working to understand sources, chemical characteristics, relationships between different pollutants, and transformations, as they are major challenges in air quality control and climate research (196, 197).
There has been a significant reduction in the solid fraction of PM in the United States and Europe; however, the generation of UFPM by nucleation of organic vapor during the dilution of the exhaust remains a serious issue (198), and the carbon UFPM from brakes, tires, and road wear will remain a problem even if we accomplish a fully electric vehicle fleet (198, 199). Furthermore, exposure to microplastics and nanoplastics is ubiquitous, and these nanoplastics can reach the brain and induce oxidative stress (57, 58).
Sleep is impacted in every neurodegenerative disease and has a robust link with depression. For a number of patients with sleep disorders, there is a close association between sleep complaints and the development and progression of well-characterized proteinopathies. It is important to determine if a sleep disorder is a consequence of the neurodegenerative process or if it plays a key role in the development of the neurodegenerative process itself. The bidirectionally/interplay between sleep and neurodegeneration makes sleep a critical physiological process subject to study in young populations with high risk for neurodegenerative pathologies.
6. Summary
1. Sleep disorders are common in neurodegenerative diseases, and the presence of targeted sleep problems associated with a high risk of development of common proteinopathies, along with significant associations between sleep deprivation, obstructive sleep apnea, intermittent hypoxia, cognitive deficits, pre-clinical AD, and other neurodegenerative pathologies, make sleep and neurodegeneration a focus for exploration in a number of patients sent to sleep laboratories (200).
2. Sustained and significant exposures to high concentrations of PM2.5 and UFPM/NPs are likely to play a significant role in the developing of neurodegenerative processes, dating back to in utero exposures. The presence of quadruple abnormal neural proteins starting in MMC infants and progressing as the subjects remain in the polluted environment should be of deep concern for health workers and has serious implications, including sleep disorders, for millions of people residing in such places.
3. We have shown the overlap of AD, PD, and TDP-43 pathology in highly exposed Mexico City children and young adults and the similarity of the overlap five decades later, when the patients are in terminal stages. We support that nanosized PM plays a key role in brain protein alterations and the complex subsequent cellular pathology.
4. Sleep disorders affect individuals of all ages with serious consequences across professions, including sleep deprivation in physicians (201). Our children are sleeping less, and there is a strong association between adverse childhood experiences and age-specific insufficient sleep duration in US youth, with serious repercussions in adulthood (202–208). There are also significant differences in sleep duration for US children depending on ethnicity and socioeconomic status (SES): among 9–13-year olds; black children sleep fewer hours compared to white, and poor children compared to higher-income children (204). Across the US, children sleep much less than what pediatricians recommend according to age, and minorities and disadvantaged children accumulate risk factors detrimental to their health (205). Moreover, lack of sleep increases the risk for addiction in adolescents based on chronic sleep loss and circadian misalignment (208). A potential association between inadequate sleep duration and changes in telomere length raises significant concerns related to cellular function (209).
5. The relationship between air pollution, sleep, neurodegeneration, depression, and suicide (210, 211) should encourage health workers to know about combustion and friction UFPM sources and engineered NPs (food products, cosmetics, toothpaste, sun protectors, surface disinfectants, paints, and e-waste). The presence of zinc, silver, copper, gold, selenium, and calcium NPs as potential food additives for animals (212), nanoplastics in drinking water (213), the massive presence of nanometric particle fraction of TiO2 in the food industry, and Fe3 O4 magnetic nanoparticles from food production, processing, storage, and detection, make constant exposures to NPs a serious health issue (76, 214).
6. The problem of human exposure to ultrafine particle pollution is solvable. We are knowledgeable of the cellular effects under experimental conditions and their intracellular and key organelle presence in the brains of urbanites (5, 10, 45, 48, 57–59, 62–71, 81, 82, 84). We also know the main emission sources and the technological options to control them (27, 215–217). The cost-benefit ratio is in favor of raising awareness (the role of our study) and taking action. We need a broader concern and awareness and the will to protect public health from deadly UFPM and industrial nanoparticles. We are also facing a lack of support for research from sleep medical societies. Denial is not an option.
Author contributions
LC-G, RT-J, and PM: conception and design of the study. LC-G, RT-J, PM, GG, RK, AG-M, RR-R, GG-A, DC-F, EG-R, RB-A, and HS-P: acquisition and analysis of data. LC-G, AA, RK, PM, ES, and GG: drafting of the text, writing, critical analysis, and preparing the figures. PM: statistical analysis. RT-J: air pollutant data. All authors contributed to the article and approved the submitted version.
Conflict of interest
AA works for the Sacramento Metropolitan Air Quality Management District.
The remaining authors declare that the research was conducted in the absence of any commercial or financial relationships that could be construed as a potential conflict of interest.
Publisher's note
All claims expressed in this article are solely those of the authors and do not necessarily represent those of their affiliated organizations, or those of the publisher, the editors and the reviewers. Any product that may be evaluated in this article, or claim that may be made by its manufacturer, is not guaranteed or endorsed by the publisher.
References
1. World Bank. The Global Health Cost of PM2.5 Air Pollution: A Case for Action Beyond 2021. Geneva: World Bank (2022).
2. Jung CR, Lin YT, Hwang BF. Ozone, particulate matter, and newly diagnosed Alzheimer's disease: a population-based cohort study in Taiwan. J Alzheimers Dis. (2015) 44:573–84. doi: 10.3233/JAD-140855
3. Oudin A, Forsberg B, Adolfsson AN, Nordin Adolfsson A, Oudin Åström D, Adolfsson R, et al. Traffic-Related air pollution and dementia incidence in northern Sweden: a longitudinal study. Environ Health Perspect. (2016) 124:306–12. doi: 10.1289/ehp.1408322
4. Chen H, Kwong JC, Copes R, Tu K, Villeneuve PJ, van Donkelaar A, et al. Living near major roads and the incidence of dementia, Parkinson's disease, and multiple sclerosis: a population-based cohort study. Lancet. (2017) 389:718–26. doi: 10.1016/S0140-6736(16)32399-6
5. Yarjanli Z, Ghaedi K, Esmaeili A, Rahgozar S, Zarrabi A. Iron oxide nanoparticles may damage to the neural tissue through iron accumulation, oxidative stress, and protein aggregation. BMC Neurosci. (2017) 18:51. doi: 10.1186/s12868-017-0369-9
6. Shi L, Steenland K, Li H, Liu P, Zhang Y, Lyles RH, et al. A national cohort study (2000-2018) of long-term air pollution exposure and incident dementia in older adults in the United States. Nat Commun. (2021) 12:6754. doi: 10.1038/s41467-021-27049-2
7. Mortamais M, Gutierrez LA, de Hoogh K, Chen J, Vienneau D, Carrière I, et al. Long-term exposure to ambient air pollution and risk of dementia: results of the prospective three-city study. Environ Int. (2021) 148:106376. doi: 10.1016/j.envint.2020.106376
8. Russ TC, Cherrie MPC, Dibben C, Tomlinson S, Reis S, Dragosits U, et al. Life course air pollution exposure and cognitive decline: modelled historical air pollution data and the Lothian birth cohort 1936. J Alzheimers Dis. (2021) 79:1063–74. doi: 10.3233/JAD-200910
9. Calderón-Garcidueñas L, Gónzalez-Maciel A, Reynoso-Robles R, Delgado-Chávez R, Mukjerjee PS, Kulesza RJ, et al. Hallmarks of Alzheimer disease are evolving relentlessly in Metropolitan Mexico City infants, children and young adults. APOE4 carriers have higher suicide risk and higher odds of reaching NFT stage V at ≤ 40 years of age. Environ Res. (2018) 164:475–87. doi: 10.1016/j.envres.2018.03.023
10. Calderón-Garcidueñas L, González-Maciel A, Reynoso-Robles R, Hammond J, Kulesza R, Lachmann I, et al. Quadruple abnormal protein aggregates in brainstem pathology and exogenous metal-rich magnetic nanoparticles (and engineered Ti-rich nanorods). The substantia nigrae is a very early target in young urbanites and the gastrointestinal tract a key brainstem portal. Environ Res. (2020) 191:110139. doi: 10.1016/j.envres.2020.110139
11. Calderón-Garcidueñas L, Ayala A. Air pollution, ultrafine particles, and your brain: are combustion nanoparticle emissions and engineered nanoparticles causing preventable fatal neurodegenerative diseases and common neuropsychiatric outcomes? Environ Sci Technol. (2022) 56:6847–56. doi: 10.1021/acs.est.1c04706
12. Calderón-Garcidueñas L, Pérez-Calatayud ÁA, González-Maciel A, Reynoso-Robles R, Silva-Pereyra HG, Ramos-Morales A, et al. Environmental nanoparticles reach human fetal brains. Biomedicines. (2022) 10:410. doi: 10.3390/biomedicines10020410
13. Lee H, Kim OJ, Jung J, Myung W, Kim SY. Long-term exposure to particulate air pollution and incidence of Parkinson's disease: a nationwide population-based cohort study in South Korea. Environ Res. (2022) 212 (Pt A):113165. doi: 10.1016/j.envres.2022.113165
14. Choi D, Lee G, Kim KH, Bae H. Particulate matter exacerbates the death of dopaminergic neurons in Parkinson's disease through an inflammatory response. Int J Mol Sci. (2022) 23:6487. doi: 10.3390/ijms23126487
15. Parra KL, Alexander GE, Raichlen DA, Klimentidis YC, Furlong MA. Exposure to air pollution and risk of incident dementia in the UK Biobank. Environ Res. (2022) 209:112895. doi: 10.1016/j.envres.2022.112895
16. Navarro-Sempere A, Martínez-Peinado P, Rodrigues AS, Garcia PV, Camarinho R, Garcia M, Segovia Y. The health hazards of volcanoes: first evidence of neuroinflammation in the hippocampus of mice exposed to active volcanic surroundings. Mediators Inflamm. (2021) 2021:5891095. doi: 10.1155/2021/5891095
17. Andrew A, Zhou J, Gui J, Harrison A, Shi X, Li M, et al. Airborne lead and polychlorinated biphenyls (PCBs) are associated with amyotrophic lateral sclerosis (ALS) risk in the U.S. Sci Total Environ. (2022) 819:153096. doi: 10.1016/j.scitotenv.2022.153096
18. Filonchyk M, Peterson MP, Sun D. Deterioration of air quality associated with the 2020 US wildfires. Sci Total Environ. (2022) 826:154103. doi: 10.1016/j.scitotenv.2022.154103
19. Su JG, Apte JS, Lipsitt J, Garcia-Gonzalez DA, Beckerman BS, de Nazelle A, et al. Populations potentially exposed to traffic-related air pollution in seven world cities. Environ Int. (2015) 78:82–9. doi: 10.1016/j.envint.2014.12.007
20. Rowangould GM. A census of the US near-roadway population: public health and environmental justice considerations. Transp Res Part D Transp Environ. (2013) 25:59–67. doi: 10.1016/j.trd.2013.08.003
21. Yousef MI, Roychoudhury S, Jafaar KS, Slama P, Kesari KK, Kamle, et al. Aluminum oxide and zinc oxide induced nanotoxicity in rat brain, heart and lung. Physiol Res. (2022) 71:677–94. doi: 10.33549/physiolres.934831
22. van den Heuvel MP, Sporns O. Network hubs in the human brain. Trends Cogn Sci. (2013) 17:683–96. doi: 10.1016/j.tics.2013.09.012
23. García-Gomar MG, Videnovic A, Singh K, Wald LL, Rosen BR, Bianciardi M. Disruption of brainstem structural connectivity in REM sleep behavior disorder using 7 tesla magnetic resonance imaging. Mov Disord. (2022) 37:847–53. doi: 10.1002/mds.28895
24. Braak H, Del Tredeci K. The preclinical phase of the pathological process underlying sporadic Alzheimer's disease. Brain. (2015) 138:2814–33. doi: 10.1093/brain/awv236
25. Karanth S, Nelson PT, Katsumata Y, Krysci RJ, Schmitt FA, Fardo DW, et al. Prevalence and clinical phenotype of quadruple misfolded proteins in older adults. JAMA Neurol. (2020) 77:1299–307. doi: 10.1001/jamaneurol.2020.1741
26. Boeve BF, Silber MH, Ferman TJ, Lin SC, Benarroch EE, Schmeichel AM, et al. Clinicopathologic correlations in 172 cases of rapid eye movement sleep behavior disorder with or without a coexisting neurologic disorder. Sleep Med. (2013) 14:754–62. doi: 10.1016/j.sleep.2012.10.015
27. James BD, Wilson RS, Boyle PA, Trojanowski JQ, Bennett DA, Schneider JA. TDP-43 stage, mixed pathologies, and clinical Alzheimer's-type dementia. Brain. (2016) 139:2983–93. doi: 10.1093/brain/aww224
28. Scarioni M, Gami-Patel P, Peeters CFW, de Koning F, Seelaar H, Mol MO, et al. Psychiatric symptoms of frontotemporal dementia and subcortical (co-)pathology burden: new insights. Brain. (2022) 146:307–20. doi: 10.1093/brain/awac043
29. Bayram E, Shan G, Cummings JL. Associations between comorbid TDP-43, Lewy body pathology, and neuropsychiatric symptoms in Alzheimer's disease. J Alzheimers Dis. (2019) 69:953–61. doi: 10.3233/JAD-181285
30. Virgilio E, De Marchi F, Contaldi E, Dianzani U, Cantello R, Mazzini L, et al. The role of tau beyond Alzheimer's disease: a narrative review. Biomedicines. (2022) 10:760. doi: 10.3390/biomedicines10040760
31. Carlos AF, Tosakulwong N, Weigand SD, Boeve BF, Knopman DS, Petersen RC, et al. Frequency and distribution of TAR DNA-binding protein 43 (TDP-43) pathology increase linearly with age in a large cohort of older adults with and without dementia. Acta Neuropathol. (2022) 144:159–60. doi: 10.1007/s00401-022-02434-3
32. Buciuc M, Martin PR, Tosakulwong N, Murray ME, Petrucelli L, Senjem ML, et al. TDP-43-associated atrophy in brains with and without frontotemporal lobar degeneration. Neuroimage Clin. (2022) 34:102954. doi: 10.1016/j.nicl.2022.102954
33. Josephs KA, Martin PR, Weigand SD, Tosakulwong N, Buciuc M, Murray ME, et al. Protein contributions to brain atrophy acceleration in Alzheimer's disease and primary age-related tauopathy. Brain. (2020) 143:3463–76. doi: 10.1093/brain/awaa299
34. Ferman TJ, Aoki N, Boeve BF, Aakre JA, Kantarci K, Graff-Radford J, et al. Subtypes of dementia with lewy bodies are associated with α-synuclein and tau distribution. Neurology. (2020) 95:e155–65. doi: 10.1212/WNL.0000000000009763
35. Xu H, Finkelstein DI, Adlard PA. Interactions of metals and apolipoprotein E in Alzheimer's disease. Front Aging Neurosci. (2014) 6:121. doi: 10.3389/fnagi.2014.00121
36. Calderón-Garcidueñas L, Rajkumar RP, Stommel EW, Kulesza R, Mansour Y, Rico-Villanueva A, et al. Brainstem quadruple aberrant hyperphosphorylated tau, beta-amyloid, alpha-synuclein and TDP-43 pathology, stress and sleep behavior disorders. Int J Environ Res Public Health. (2021) 18:6689. doi: 10.3390/ijerph18136689
37. Calderón-Garcidueñas L, Azzarelli B, Acuna H, Garcia R, Gambling TM, Del Tizapantzi MR, et al. Air pollution and brain damage. Toxicol Pathol. (2002) 30:373–89. doi: 10.1080/01926230252929954
38. Calderón-Garcidueñas L, Mukherjee PS, Kulesza RJ, Torres-Jardón R, Hernández-Luna J, Avila-Cervantes R, et al. Mild cognitive impairment and dementia involving multiple cognitive domains in Mexican urbanites. J Alzheimers Dis. (2019) 68:1113–123. doi: 10.3233/JAD-181208
39. Calderón-Garcidueñas L, Hernández-Luna J, Mukherjee PS, Styner M, Chávez-Franco DA, Luèvano-Castro SC, et al. Hemispheric cortical, cerebellar and caudate atrophy associated to cognitive impairment in metropolitan Mexico City young adults exposed to fine particulate matter air pollution. Toxics. (2022) 10:156. doi: 10.3390/toxics10040156
40. Calderón-Garcidueñas L, Mora-Tiscareño A, Ontiveros E, Gómez-Garza G, Barragan-Mejia G, Broadway J, et al. Air pollution, cognitive deficits and brain abnormalities: a pilot study with children and dogs. Brain Cogn. (2008) 68:117–27. doi: 10.1016/j.bandc.2008.04.008
41. Calderón-Garcidueñas L, Torres-Solorio AK, Kulesza RJ, Torres-Jardon R, González-González LO, García-Arreola B, et al. Gait and balance disturbances are common in young urbanites and associated with cognitive impairment. Air pollution and the historical development of Alzheimer's disease in the young. Environ Res. (2020) 191:110087. doi: 10.1016/j.envres.2020.110087
42. Calderón-Garcidueñas L, Kulesza RJ, Mansour Y, Aiello-Mora M, Mukherjee PS, González-González LO. Increased gain in the auditory pathway, Alzheimer's disease continuum, and air pollution: peripheral and central auditory system dysfunction evolves across pediatric and adult urbanites. J Alzheimers Dis. (2019) 70:1275–86. doi: 10.3233/JAD-190405
43. Calderón-Garcidueñas L, Chao CK, Thompson C, Rodriguez-Diaz J, Franco-Lira M, Mukherjee PS, et al. CSF biomarkers: low amyloid β 1-42 and BDNF and high IFN⋎ differentiate children exposed to Mexico City high air pollution v controls. Alzheimer's disease uncertainties. Alzheimer Dis Park. (2015) 5:2. doi: 10.4172/2161-0460.1000189
44. Heal MR, Kumar P, Harrison RM. Particles, air quality, policy and health. Chem Soc Rev. (2012) 41:6606–30. doi: 10.1039/c2cs35076a
45. Kwon HS, Ryu MH, Carlsten C. Ultrafine particles: unique physicochemical properties relevant to health and disease. Exp Mol Med. (2020) 52:318–28. doi: 10.1038/s12276-020-0405-1
46. de Jesús AL, Rahman MM, Mazaheri M, Thompson H, Knibbs LD, Jeong C, et al. Ultrafine particles and PM2.5 in the air of cities around the world: are they representative of each other? Environ Int. (2019) 129:118–35. doi: 10.1016/j.envint.2019.05.021
47. Ankilov A, Baklanov A, Colhoun M, Enderle KH, Gras J, Julanox Y, et al. Intercomparison of number concentration measurements by various aerosol particle counters. Atmosph Res. (2002) 62:177–207. doi: 10.1016/S0169-8095(02)00010-8
48. Kumar P, Morawska L, Birmili W, Paasonen P, Hu M, Kulmala M, et al. Ultrafine particles in cities. Environ Int. (2014) 66:1–10. doi: 10.1016/j.envint.2014.01.013
49. Sardar SB, Fine PM, Yoon H, Sioutas C. Associations between particle number and gaseous co-pollutant concentrations in the Los Angeles Basin. J Air Waste Manag Assoc. (2004) 54:992–1005. doi: 10.1080/10473289.2004.10470970
50. Calderón-Garcidueñas L, González-Maciel A, Reynoso-Robles R, Silva-Pereyra HG, Torres-Jardon R, Brito-Aguilar R, et al. Environmentally toxic solid nanoparticles in noradrenergic and dopaminergic nuclei and cerebellum of metropolitan Mexico City children and young adults with neural quadruple misfolded protein pathologies and high exposures to nano particulate matter. Toxics. (2022) 10:164. doi: 10.3390/toxics10040164
51. Dunn MJ, Jiménez JL, Baumgardner D, Castro T, McMurry PH, Smith JN. Measurements of Mexico City nanoparticle size distributions: observations of new particle formation and growth. Geophys Res Lett. (2004) 31:1–4. doi: 10.1029/2004GL019483
52. Caudillo L, Salcedo D, Peralta O, Castro T, Alvarez-Ospina H. Nanoparticle size distributions in Mexico City. Atmosph Pollut Res. (2020) 11:78–84. doi: 10.1016/j.apr.2019.09.017
53. Velasco E, Retama A, Segovia E, Ramos R. Particle exposure and inhaled dose while commuting by public transport in Mexico City. Atmosph Environ. (2019) 219:117044. doi: 10.1016/j.atmosenv.2019.117044
54. Kleinman LI, Springston SR, Wang J, Daum PH, Lee YN, Nunnermacker LJ, et al. The time evolution of aerosol size distribution over the Mexico City plateau. Atmosph Chem Phys. (2009) 9:4261–78. doi: 10.5194/acp-9-4261-2009
55. Calderón-Garcidueñas L, Reynoso-Robles R, González-Maciel A. Combustion and friction-derived nanoparticles and industrial-sourced nanoparticles: the culprit of Alzheimer and Parkinson's diseases. Environ Res. (2019) 176:108574. doi: 10.1016/j.envres.2019.108574
56. Calderón-Garcidueñas L, González-Maciel A, Mukherjee PS, Reynoso-Robles R, Pérez-Guille B, Gayosso-Chávez C, et al. Combustion- and friction-derived magnetic air pollution nanoparticles in human hearts. Environ Res. (2019) 176:108567. doi: 10.1016/j.envres.2019.108567
57. Prüst M, Meijer J, Westerink RHS. The plastic brain: neurotoxicity of micro- and nanoplastics. Part Fibre Toxicol. (2020) 17:24. doi: 10.1186/s12989-020-00358-y
58. Lamichhane G, Acharya A, Marahatha R, Modi B, Paudel R, Adhikari A, et al. Microplastics in environment: global concern, challenges, and controlling measures. Int J Environ Sci Technol. (2022) 26:1–22. doi: 10.1007/s13762-022-04261-1
59. Abdelkhaliq A, van der Zande M, Punt A, Helsdingen R, Boeren S, Vervoort JJM, et al. Impact of nanoparticle surface functionalization on the protein corona and cellular adhesion, uptake and transport. J Nanobiotechnology. (2018) 16:70. doi: 10.1186/s12951-018-0394-6
60. Cedervall T, Lynch I, Lindman S, Berggard T, Thulin E, Nilsson H, et al. Understanding the nanoparticle-protein corona using methods to quantify exchange rates and affinities of proteins for nanoparticles. Proc Natl Acad Sci USA. (2007) 104:2050–5. doi: 10.1073/pnas.0608582104
61. Eftekharzadeh B, Hyman BT, Wegmann S. Structural studies on the mechanism of protein aggregation in age related neurodegenerative diseases. Mech Ageing Dev. (2016) 156:1–13. doi: 10.1016/j.mad.2016.03.001
62. Ehsanifar M, Tameh AA, Farzadkia M, Kalantari RR, Zavareh MS, Nikzaad H, et al. Exposure to nanoscale diesel exhaust particles: oxidative stress, neuroinflammation, anxiety and depression on adult male mice. Ecotoxicol Environ Saf. (2019) 168:338–47. doi: 10.1016/j.ecoenv.2018.10.090
63. Gutiérrez L, de la Cueva L, Moros M, Mazario E, de Bernardo S, de la Fuente JM, et al. Aggregation effects on the magnetic properties of iron oxide colloids. Nanotechnology. (2019) 30:112001. doi: 10.1088/1361-6528/aafbff
64. Imam SZ, Lantz-McPeak SM, Cuevas E, Rosas-Hernandez H, Liachenko S, Zhang Y, et al. Iron oxide nanoparticles induce dopaminergic damage: in vitro pathways and in vivo imaging reveals mechanism of neuronal damage. Mol Neurobiol. (2015) 52:913–26. doi: 10.1007/s12035-015-9259-2
65. Linse S, Cabaleiro-Lago C, Xue WF, Lynch I, Lindman S, Thulin E, et al. Nucleation of protein fibrillation by nanoparticles. Proc Natl Acad Sci USA. (2007) 104:8691–6. doi: 10.1073/pnas.0701250104
66. Shakeel M, Jabeen F, Shabbir S, Asghar MS, Khan MS, Chaudhry AS. Toxicity of nano-titanium dioxide (TiO2-NP) through various routes of exposure: a review. Biol Trace Elem Res. (2016) 172:1–36. doi: 10.1007/s12011-015-0550-x
67. Vitali M, Rigamonti V, Natalello A, Colzani B, Avvakumova S, Brocca S, et al. Conformational properties of intrinsically disordered proteins bound to the surface of silica nanoparticles. Biochim Biophys Acta Gen. (2018) 1862:1556–64. doi: 10.1016/j.bbagen.2018.03.026
68. Wu T, Tang M. The inflammatory response to silver and titanium dioxide nanoparticles in the central nervous system. Nanomedicine. (2018) 13:233–49. doi: 10.2217/nnm-2017-0270
69. Xie H, Wu J. Silica nanoparticles induce alpha-synuclein induction and aggregation in PC12-cells. Chem Biol Interact. (2016) 258:197–204. doi: 10.1016/j.cbi.2016.09.006
70. Frtús A, Smolková B, Uzhytchak M, Lunova M, Jirsa M, Kubinova S, et al. Analyzing the mechanisms of iron oxide nanoparticles interactions with cells: a road from failure to success in clinical applications. J Control Release. (2020) 328:59–77. doi: 10.1016/j.jconrel.2020.08.036
71. Brenner JS, Pan DC, Myerson JW, Marcos-Contreras OA, Villa CH, Patel P, et al. Red blood cell-hitchhiking boosts delivery of nanocarriers to chosen organs by orders of magnitude. Nat Commun. (2018) 9:2684. doi: 10.1038/s41467-018-05079-7
72. Calderón-Garcidueñas L, Reynoso-Robles R, Pérez-Guillé B, Mukherjee PS, Gónzalez-Maciel A. Combustion-derived nanoparticles, the neuroenteric system, cervical vagus, hyperphosphorylated alpha synuclein and tau in young Mexico City residents. Environ Res. (2017) 159:186–201. doi: 10.1016/j.envres.2017.08.008
73. Schaeffer S, Iadecola C. Revisiting the neurovascular unit. Nat Neurosci. (2021) 24:1198–209. doi: 10.1038/s41593-021-00904-7
74. de la Torre JC. Are major dementias triggered by poor blood flow to the brain? Theoretical considerations. J Alzheimers Dis. (2017) 57:353–71. doi: 10.3233/JAD-161266
75. Bassiony H, El-Ghor AA, Salaheldin TA, Sabet S, Mohamed MM. Tissue distribution, histopathological and genotoxic effects of magnetite nanoparticles on ehrlich solid carcinoma. Biol Trace Elem Res. (2022) 200:5145–58. doi: 10.1007/s12011-022-03102-z
76. Cornu R, Béduneau A, Martin H. Ingestion of titanium dioxide nanoparticles: a definite health risk for consumers and their progeny. Arch Toxicol. (2022) 96:2655–86. doi: 10.1007/s00204-022-03334-x
77. Yao Y, Chen Z, Zhang T, Tang M. Adverse reproductive and developmental consequences of quantum dots. Environ Res. (2022) 213:113666. doi: 10.1016/j.envres.2022.113666
78. Wu J, Zhu Z, Liu W, Zhang Y, Kang Y, Liu J, et al. How nanoparticles open the paracellular route of biological barriers: mechanisms, applications, and prospects. ACS Nano. (2022) 16:15627–52. doi: 10.1021/acsnano.2c05317
79. Hartl FU. Protein misfolding diseases. Annu Rev Biochem. (2017) 86:21–6. doi: 10.1146/annurev-biochem-061516-044518
80. Aliakbari F, Attar F, Movahedi M, Falahati M. Human tau fibrillization and neurotoxicity in the presence of magnesium oxide nanoparticle fabricated through laser ablation method. Spectrochim Acta A Mol Biomol Spectrosc. (2022) 278:121372. doi: 10.1016/j.saa.2022.121372
81. John T, Adler J, Elsner C, Petzold J, Krueger M, Martin LL, et al. Mechanistic insights into the size-dependent effects of nanoparticles on inhibiting and accelerating amyloid fibril formation. J Colloid Interface Sci. (2022) 622:804–18. doi: 10.1016/j.jcis.2022.04.134
82. Mohammad-Beigi H, Zanganeh M, Scavenius C, Eskandari H, Farzadfard A, Shojaosadati SA, et al. A protein corona modulates interactions of α-synuclein with nanoparticles and alters the rates of the microscopic steps of amyloid formation. ACS Nano. (2022) 16:1102–18. doi: 10.1021/acsnano.1c08825
83. Fernández-Bertólez N, Costa C, Brandão F, Brandao F, Teixeira JP, Pásaro E, et al. Toxicological aspects of iron oxide nanoparticles. Adv Exp Med Biol. (2022) 1357:303–50. doi: 10.1007/978-3-030-88071-2_13
84. Teleanu DM, Chircov C, Grumezescu AM, Teleanu RI. Neurotoxicity of nanomaterials: an up-to-date overview. Nanomaterials. (2019) 9:E96. doi: 10.3390/nano9010096
85. Shu Y, Ma M, Pan X, Shafiq M, Yu H, Chen H. Cobalt protoporphyrin-induced nano-self-assembly for CT imaging, magnetic-guidance, and antioxidative protection of stem cells in pulmonary fibrosis treatment. Bioact Mater. (2023) 21:129–41. doi: 10.1016/j.bioactmat.2022.08.008
86. Calderón-Garcidueñas L, Villarreal-Calderón R, Valencia-Salazar G, Henríquez-Roldán C, Gutiérrez-Castrellón P, Torres-Jardón R, et al. Systemic inflammation, endothelial dysfunction, and activation in clinically healthy children exposed to air pollutants. Inhal Toxicol. (2008) 20:499–506. doi: 10.1080/08958370701864797
87. Calderón-Garcidueñas L, Rodriguez-Alcaraz A, García R, Ramírez L, Barragan G. Nasal inflammatory responses in children exposed to a polluted urban atmosphere. J Toxicol Environ Health. (1995) 45:427–37. doi: 10.1080/15287399509532006
88. Calderon-Garciduenas L, Osnaya-Brizuela N, Ramirez-Martinez L, Villarreal-Calderon A. DNA strand breaks in human nasal respiratory epithelium are induced upon exposure to urban pollution. Environ Health Perspect. (1996) 104:160–8. doi: 10.1289/ehp.96104160
89. Calderón-Garcidueñas L, Osnaya N, Rodríguez-Alcaraz A, Villarreal-Calderón A. DNA damage in nasal respiratory epithelium from children exposed to urban pollution. Environ Mol Mutagen. (1997) 30:11–20. doi: 10.1002/(SICI)1098-2280(1997)30:1<11::AID-EM3>3.0.CO;2-F
90. Calderón-Garcidueñas L, Rodríguez-Alcaraz A, Villarreal-Calderón A, Lyght O, Janszen D, Morgan KT. Nasal epithelium as a sentinel for airborne environmental pollution. Toxicol Sci. (1998) 46:352–64. doi: 10.1093/toxsci/46.2.352
91. Mozzoni P, Iodice S, Persico N, Ferrari L, Pinelli S, Corradi M, et al. Maternal air pollution exposure during the first trimester of pregnancy and markers of inflammation and endothelial dysfunction. Environ Res. (2022) 212 (Pt. A):113216. doi: 10.1016/j.envres.2022.113216
92. Wu X, Cao X, Lintelmann J, Peters A, Koening W, Zimmermann R, et al. Assessment of the association of exposure to polycyclic aromatic hydrocarbons, oxidative stress, and inflammation: a cross-sectional study in Augsburg, Germany. Int J Hyg Environ Health. (2022) 244:113993. doi: 10.1016/j.ijheh.2022.113993
93. Orysiak J, Mlynarczyk M, Piec R, Jakubiak A. Lifestyle and environmental factors may induce airway and systemic inflammation in firefighters. Environ Sci Pollut Res Int. (2022) 29:73741–68. doi: 10.1007/s11356-022-22479-x
94. Huang C, Kritikos M, Serrano Sosa M, Hagan T, Domkan A, Meliker J, et al. World trade center site exposure duration is associated with hippocampal and cerebral white matter neuroinflammation. Mol Neurobiol. (2023) 60:160–70. doi: 10.1007/s12035-022-03059-z
95. Diab O, DePierro J, Cancelmo L, Schaffer J, Schechter C, Dasaro CR, et al. Mental healthcare needs in world trade center responders: results from a large, population-based health monitoring cohort. Adm Policy Ment Health. (2020) 47:427–34. doi: 10.1007/s10488-019-00998-z
96. Seil K, Takemoto E, Farfel MR, Huynh M, Li J. Exploratory case study of suicide among a sample of 9/11 survivors. Int J Environ Res Public Health. (2021) 19:57. doi: 10.3390/ijerph19010057
97. Gibson R, Whealin JM, Dasaro CR, Udasin IG, Crane M, Moline JM, et al. Prevalence and correlates of suicidal ideation in world trade center responders: results from a population-based health monitoring cohort. J Affect Disord. (2022) 306:62–70. doi: 10.1016/j.jad.2022.03.011
98. He S, Klevebro S, Baldanzi G, Pershagen G, Lundberg B, Eneroth K, et al. Ambient air pollution and inflammation-related proteins during early childhood. Environ Res. (2022) 215 (Pt. 2):114364. doi: 10.1016/j.envres.2022.114364
99. Rolo D, Assunção R, Ventura C, Alvito P, Gonçalves L, Martins C, et al. Adverse outcome pathways associated with the ingestion of titanium dioxide nanoparticles-a systematic review. Nanomaterials. (2022) 12:3275. doi: 10.3390/nano12193275
100. Park EJ, Kim SN, Yoon C, Cho JW, Lee GH, Kim DW, et al. Repeated intratracheal instillation of zinc oxide nanoparticles induced pulmonary damage and a systemic inflammatory response in cynomolgus monkeys. Nanotoxicology. (2021) 15:621–35. doi: 10.1080/17435390.2021.1905899
101. Suthar JK, Vaidya A, Ravindran S. Toxic implications of silver nanoparticles on the central nervous system: a systematic literature review. J Appl Toxicol. (2023) 43:4–21. doi: 10.1002/jat.4317
102. Ribeiro LW, Pietri M, Ardila-Osorio H, Baudry A, Boudet-Devaud F, Bizingre C, et al. Titanium dioxide and carbon black nanoparticles disrupt neuronal homeostasis via excessive activation of cellular prion protein signaling. Part Fibre Toxicol. (2022) 19:48. doi: 10.1186/s12989-022-00490-x
103. Mohammadipour A, Abudayyak M. Hippocampal toxicity of metal base nanoparticles. Is there a relationship between nanoparticles and psychiatric disorders? Rev Environ Health. (2021) 37:35–44. doi: 10.1515/reveh-2021-0006
104. Mostovenko E, Saunders S, Muldoon PP, Bishop L, Campen MJ, Erdely A, et al. Carbon nanotube exposure triggers a cerebral peptidomic response: barrier compromise, neuroinflammation, and a hyperexcited state. Toxicol Sci. (2021) 182:107–19. doi: 10.1093/toxsci/kfab042
105. Ehsanifar M, Montazeri Z, Taheri MA, Rafati M, Behjati M, Karimian M. Hippocampal inflammation and oxidative stress following exposure to diesel exhaust nanoparticles in male and female mice. Neurochem Int. (2021) 145:104989. doi: 10.1016/j.neuint.2021.104989
106. Novoa C, Salazar P, Cisternas P, Gherardelli C, Vera-Salazar R, Zolezzi JM, et al. Inflammation context in Alzheimer's disease, a relationship intricate to define. Biol Res. (2022) 55:39. doi: 10.1186/s40659-022-00404-3
107. Nunomura A, Perry G. RNA and oxidative stress in alzheimer's disease: focus on microRNAs. Oxid Med Cell Longev. (2020) 2020:2638130. doi: 10.1155/2020/2638130
108. Minchev D, Kazakova M, Sarafian V. Neuroinflammation and autophagy in parkinson's disease-novel perspectives. Int J Mol Sci. (2022) 23:14997. doi: 10.3390/ijms232314997
109. de Luna N, Carbayo A, Dols-Icardo O, Turon-Sans J, Reyes-Leiva D, Illan-Gala I, et al. Neuroinflammation-related proteins NOD2 and spp1 are abnormally upregulated in amyotrophic lateral sclerosis. Neurol Neuroimmunol Neuroinflamm. (2022) 10:e200072. doi: 10.1212/NXI.0000000000200072
110. Utermann G. Alzheimer's disease. The apolipoprotein E connection. Curr Biol. (1994) 4:362–5. doi: 10.1016/S0960-9822(00)00081-6
111. Arai H, Higuchi S, Sasaki H. Apolipoprotein E genotyping and cerebrospinal fluid tau protein: implications for the clinical diagnosis of Alzheimer's disease. Gerontology. (1997) 43 (Suppl. 1):2–10. doi: 10.1159/000213879
112. Butterfield DA, Mattson MP. Apolipoprotein E and oxidative stress in brain with relevance to Alzheimer's disease. Neurobiol Dis. (2020) 138:104795. doi: 10.1016/j.nbd.2020.104795
113. Tzioras M, Davies C, Newman A, Jackson R, Spires-Jones T. Invited review: APOE at the interface of inflammation, neurodegeneration and pathological protein spread in Alzheimer's disease. Neuropathol Appl Neurobiol. (2019) 45:327–46. doi: 10.1111/nan.12529
114. Genin E, Hannequin D, Wallon D, Sleegers K, Hiltunen M, Combarros O, et al. APOE and Alzheimer disease: a major gene with semi-dominant inheritance. Mol Psychiatry. (2011) 16:903–7. doi: 10.1038/mp.2011.52
115. Tcw J, Qian L, Pipalia NH, Chao MJ, Liang SA, Shi Y, et al. Cholesterol and matrisome pathways dysregulated in astrocytes and microglia. Cell. (2022) 185:2213–33.e25. doi: 10.1016/j.cell.2022.05.017
116. Nichols JB, Malek-Ahmadi M, Tariot PN, Serrano GE, Sue LI, Beach TG. Vascular lesions, APOE ε4, and Tau pathology in Alzheimer disease. J Neuropathol Exp Neurol. (2021) 80:240–6. doi: 10.1093/jnen/nlaa160
117. Beach TG, Malek-Ahmadi M. Alzheimer's disease neuropathological comorbidities are common in the younger-old. J Alzheimers Dis. (2021) 79:389–400. doi: 10.3233/JAD-201213
118. Mehta RI, Schneider JA. What is “Alzheimer's disease”? The neuropathological heterogeneity of clinically defined Alzheimer's dementia. Curr Opin Neurol. (2021) 34:237–45. doi: 10.1097/WCO.0000000000000912
119. Jellinger KA. Recent update on the heterogeneity of the Alzheimer's disease spectrum. J Neural Transm. (2022) 129:1–24. doi: 10.1007/s00702-021-02449-2
120. Jellinger KA. Clinicopathological analysis of dementia disorders in the elderly–an update. J Alzheimers Dis. (2006) 9 (3 Suppl):61–70. doi: 10.3233/JAD-2006-9S308
121. Jellinger KA. Morphological basis of Parkinson disease-associated cognitive impairment: an update. J Neural Transm. (2022) 129:977–99. doi: 10.1007/s00702-022-02522-4
122. Toledo JB, Liu H, Grothe MJ, Rashid T, Launer L, Shaw LM, et al. Disentangling tau and brain atrophy cluster heterogeneity across the Alzheimer's disease continuum. Alzheimers Dement. (2022) 8:e12305. doi: 10.1002/trc2.12305
123. Carlos AF, Josephs KA. Frontotemporal lobar degeneration with TAR DNA-binding protein 43 (TDP-43): its journey of more than 100 years. J Neurol. (2022) 269:4030–54. doi: 10.1007/s00415-022-11073-3
124. Louis ED, Iglesias-Hernandez D, Hernandez NC, Flowers X, Kuo SH, Vonsattel JPG, et al. Lewy pathology in 231 essential tremor brains from the essential tremor centralized brain repository. J Neuropathol Exp Neurol. (2022) 81:796–806. doi: 10.1093/jnen/nlac068
125. Whitwell J, Martin PR, Graff-Radford J, Machulda M, Sintini I, Buciuc M, et al. Investigating heterogeneity and neuroanatomic correlates of longitudinal clinical decline in atypical Alzheimer disease. Neurology. (2022) 98:e2436–45. doi: 10.1212/WNL.0000000000200336
126. Nelson PT, Brayne C, Flanagan ME, Abner EL, Agrawal S, Attems J, et al. Frequency of LATE neuropathologic change across the spectrum of Alzheimer's disease neuropathology: combined data from 13 community-based or population-based autopsy cohorts. Acta Neuropathol. (2022) 144:27–44. doi: 10.1007/s00401-022-02444-1
127. Frank B, Ally M, Tripodis Y, Puzo C, Labriolo C, Hurley L, et al. Trajectories of cognitive decline in brain donors with autopsy-confirmed alzheimer disease and cerebrovascular disease. Neurology. (2022) 98:e2454–64. doi: 10.1212/WNL.0000000000200304
128. Mohanty R, Ferreira D, Frerich S, Muehlboeck JS, Grothe MJ, Westman E, et al. Neuropathologic features of antemortem atrophy-based subtypes of Alzheimer disease. Neurology. (2022) 99:e323–33. doi: 10.1212/WNL.0000000000200573
129. Calderón-Garcidueñas L, Reynoso-Robles R, Vargas-Martínez J, Gomez-Maqueo-Chew A, Perez-Guille B, Mukherjee PS, et al. Prefrontal white matter pathology in air pollution exposed Mexico City young urbanites and their potential impact on neurovascular unit dysfunction and the development of Alzheimer's disease. Environ Res. (2016) 146:404–17. doi: 10.1016/j.envres.2015.12.031
130. Zucca FA, Capucciati A, Bellei C, Sarna M, Sarna T, Monzani E, et al. Neuromelanins in brain aging and Parkinson's disease: synthesis, structure, neuroinflammatory, and neurodegenerative role. IUBMB Life. (2022) 75:55–65. doi: 10.1002/iub.2654
131. Ceballos D, Zhou M, Herrick R. Metals and particulates exposure from a mobile e-waste shredding truck: a pilot study. Ann Work Expo Health. (2020) 64:890–6. doi: 10.1093/annweh/wxaa058
132. Frazzoli C, Ruggieri F, Battistini B, Orisakwe OE, Igbo JK, Bocca B. E-WASTE threatens health: the scientific solution adopts the one health strategy. Environ Res. (2022) 212 (Pt. A):113227. doi: 10.1016/j.envres.2022.113227
133. Carreras-Sureda A, Kroemer G, Cardenas JC, Hetz C. Balancing energy and protein homeostasis at ER-mitochondria contact sites. Sci Signal. (2022) 15:eabm7524. doi: 10.1126/scisignal.abm7524
134. Degechisa ST, Dabi YT, Gizaw ST. The mitochondrial associated endoplasmic reticulum membranes: a platform for the pathogenesis of inflammation-mediated metabolic diseases. Immun Inflamm Dis. (2022) 10:e647. doi: 10.1002/iid3.647
135. Sahyadri M, Nadiga APR, Mehdi S, Mruthunjaya K, Nayak PG, Parihar VK, et al. Mitochondria-lysosome crosstalk in GBA1-associated Parkinson's disease. 3 Biotech. (2022) 12:230. doi: 10.1007/s13205-022-03261-9
136. Andreu I, Granero-Moya I, Garcia-Manyes S, Roca-Cusachs P. Understanding the role of mechanics in nucleocytoplasmic transport. APL Bioeng. (2022) 6:020901. doi: 10.1063/5.0076034
137. Antón-Fernández A, Vallés-Saiz L, Avila J, Hernández F. Neuronal nuclear tau and neurodegeneration. Neuroscience. (2022). doi: 10.1016/j.neuroscience.2022.07.015. [Epub ahead of print].
138. McKenna MJ, Shao S. The endoplasmic reticulum and the fidelity of nascent protein localization. Cold Spring Harb Perspect Biol. (2022). doi: 10.1101/cshperspect.a041249. [Epub ahead of print].
139. Lee JH, Nixon RA. Autolysosomal acidification failure as a primary driver of Alzheimer disease pathogenesis. Autophagy. (2022) 18:2763–4. doi: 10.1080/15548627.2022.2110729
140. Gegg ME, Schapira AHV. Lysosomal-endolysomal dysfunction and neurodegenerative diseases. Neurobiol Dis. (2022) 173:105843. doi: 10.1016/j.nbd.2022.105843
141. Sorrentino I, Galli M, Medraño-Fernandez I, Sitia R. Transfer of H2O2 from mitochondria to the endoplasmic reticulum via aquaporin-11. Redox Biol. (2022) 55:102410. doi: 10.1016/j.redox.2022.102410
142. Mannino PJ, Lusk CP. Quality control mechanisms that protect nuclear envelope identity and function. J Cell Biol. (2022) 221:e202205123. doi: 10.1083/jcb.202205123
143. Valli M, Uribe C, Mihaescu A, Strafella AP. Neuroimaging of rapid eye movement sleep behavior disorder and its relation to Parkinson's disease. J Neurosci Res. (2022) 100:1815–33. doi: 10.1002/jnr.25099
144. Miyamoto T, Miyamoto M, Numahata K, Onoue H, Akaiwa Y, Sairenchi T. Reduced dopamine transporter binding predicts early transition to lewy body disease in Japanese patients with idiopathic rapid eye movement sleep behavior disorder. J Neurol Sci. (2020) 414:116821. doi: 10.1016/j.jns.2020.116821
145. Zhang F, Niu L, Liu X, Liu Y, Li S, Yu L, et al. Rapid eye movement sleep behavior disorder and neurodegenerative diseases: an update. Aging Dis. (2020) 11:315–26. doi: 10.14336/AD.2019.0324
146. Van Patten R, Mahmood Z, Pickell D, Maye JE, Roesch S, Twamley EW, et al. REM sleep behavior disorder in Parkinson's disease: change in cognitive, psychiatric, and functional outcomes from baseline to 16-47-month follow-up. Arch Clin Neuropsychol. (2022) 37:1–11. doi: 10.1093/arclin/acab037
147. Mizrahi-Kliger AD, Feldmann LK, Kühn AA, Bergman H. Etiologies of insomnia in Parkinson's disease - lessons from human studies and animal models. Exp Neurol. (2022) 350:113976. doi: 10.1016/j.expneurol.2022.113976
148. Gottshall JL, Agyemang AA, O'Neil M, Wei G, Presson A, Hewins B, et al. Sleep quality: a common thread linking depression, post-traumatic stress, and post-concussive symptoms to biomarkers of neurodegeneration following traumatic brain injury. Brain Inj. (2022) 36:633–43. doi: 10.1080/02699052.2022.2037711
149. Palagini L, Geoffroy PA, Riemann D. Sleep markers in psychiatry: do insomnia and disturbed sleep play as markers of disrupted neuroplasticity in mood disorders? A proposed model. Curr Med Chem. (2022) 29:5595–605. doi: 10.2174/0929867328666211214164907
150. Niu L, Zhang F, Xu X, Yang Y, Li S, Liu H, et al. Chronic sleep deprivation altered the expression of circadian clock genes and aggravated Alzheimer's disease neuropathology. Brain Pathol. (2022) 32:e13028. doi: 10.1111/bpa.13028
151. Sunkaria A, Bhardwaj S. Sleep disturbance and alzheimer's disease: the glial connection. Neurochem Res. (2022) 47:1799–815. doi: 10.1007/s11064-022-03578-0
152. Miglis MG, Adler CH, Antelmi E, Arnaldi D, Baldelli L, Boeve BF, et al. Biomarkers of conversion to α-synucleinopathy in isolated rapid-eye-movement sleep behaviour disorder. Lancet Neurol. (2021) 20:671–84. doi: 10.1016/S1474-4422(21)00176-9
153. Stang CD, Mullan AF, Hajeb M, Camerucci E, Turcano P, Martin P, et al. Timeline of rapid eye movement sleep behavior disorder in overt alpha-synucleinopathies. Ann Neurol. (2021) 89:293–303. doi: 10.1002/ana.25952
154. Gossard TR, McCarter SJ, Gorres E, Feemster JC, Timm PC, Teigen LN, et al. Quantitative REM sleep without atonia in Parkinson's disease and essential tremor. Mov Disord Clin Pract. (2021) 8:37–43. doi: 10.1002/mdc3.13112
155. McCarter SJ, Gehrking TL, St Louis EK, Suarez MD, Boeve BF, Silber MH, et al. Autonomic dysfunction and phenoconversion in idiopathic REM sleep behavior disorder. Clin Auton Res. (2020) 30:207–13. doi: 10.1007/s10286-020-00674-5
156. Boeve A, Ferman TJ, Aakre J, St Louis E, Machiulda M, Fields J, et al. Excessive daytime sleepiness in major dementia syndromes. Am J Alzheimers Dis Other Demen. (2019) 34:261–4. doi: 10.1177/1533317519828046
157. Postuma RB, Iranzo A, Hu M, Hogl B, Boeve BF, Manni R, et al. Risk and predictors of dementia and parkinsonism in idiopathic REM sleep behaviour disorder: a multicentre study. Brain. (2019) 142:744–59. doi: 10.1093/brain/awz030
158. Dauvilliers Y, Schenck CH, Postuma RB, Iranzo A, Luppi PH, Plazzi G, et al. REM sleep behaviour disorder. Nat Rev Dis Primers. (2018) 4:19. doi: 10.1038/s41572-018-0016-5
159. Savica R, Boeve BF, Mielke MM. When do α-synucleinopathies start? An epidemiological timeline: a review. JAMA Neurol. (2018) 75:503–9. doi: 10.1001/jamaneurol.2017.4243
160. St Louis EK, Boeve AR, Boeve BF. REM sleep behavior disorder in parkinson's disease and other synucleinopathies. Mov Disord. (2017) 32:645–58. doi: 10.1002/mds.27018
161. McCarter SJ, St Louis EK, Boeve BF. Sleep disturbances in frontotemporal dementia. Curr Neurol Neurosci Rep. (2016) 16:85. doi: 10.1007/s11910-016-0680-3
162. Chen M, Li Y, Chen J, Gao L, Sun J, Gu Z, et al. Structural and functional brain alterations in patients with idiopathic rapid eye movement sleep behavior disorder. J Neuroradiol. (2022) 49:66–72. doi: 10.1016/j.neurad.2020.04.007
163. Murray ME, Ferman TJ, Boeve BF, Przybelski SA, Lesnick TG, Leisinger AM, et al. MRI and pathology of REM sleep behavior disorder in dementia with lewy bodies. Neurology. (2013) 81:1681–9. doi: 10.1212/01.wnl.0000435299.57153.f0
164. Urso D, Nigro S, Tafuri B, Gnoni V, Filardi M, De Blasi R, et al. Magnetic resonance parkinsonism index is associated with rem sleep behavior disorder in Parkinson's disease. Brain Sci. (2022) 12:202. doi: 10.3390/brainsci12020202
165. Rémillard-Pelchat D, Rahayel S, Gaubert M, Postuma RB, Montplaisir J, Pelletier A, et al. Comprehensive analysis of brain volume in rem sleep behavior disorder with mild cognitive impairment. J Parkinsons Dis. (2022) 12:229–41. doi: 10.3233/JPD-212691
166. Lee DA, Lee HJ, Park KM. Glymphatic dysfunction in isolated REM sleep behavior disorder. Acta Neurol Scand. (2022) 145:464–70. doi: 10.1111/ane.13573
167. Malhotra RK. Neurodegenerative disorders and sleep. Sleep Med Clin. (2018) 13:63–70. doi: 10.1016/j.jsmc.2017.09.006
168. Driver-Dunckley ED, Adler CH. Movement disorders and sleep. Neurol Clin. (2012) 30:1345–58. doi: 10.1016/j.ncl.2012.08.019
169. Standlee J, Malkani R. Sleep dysfunction in movement disorders: a window to the disease biology. Curr Neurol Neurosci Rep. (2022) 22:565–76. doi: 10.1007/s11910-022-01220-8
170. Zamore Z, Veasey SC. Neural consequences of chronic sleep disruption. Trends Neurosci. (2022) 45:678–91. doi: 10.1016/j.tins.2022.05.007
171. Duncan MJ, Guerriero LE, Kohler K, Beechem LE, Gillis BD, Salisbury F, et al. Chronic fragmentation of the daily sleep-wake rhythm increases amyloid-beta levels and neuroinflammation in the 3xtg-ad mouse model of alzheimer's disease. Neuroscience. (2022) 481:111–22. doi: 10.1016/j.neuroscience.2021.11.042
172. Grigg-Damberger MM, Foldvary-Schaefer N. Sleep biomarkers help predict the development of Alzheimer disease. J Clin Neurophysiol. (2022) 39:327–34. doi: 10.1097/WNP.0000000000000818
173. Burke S, Grudzien A, Li T, Abril M, Spadola C, Barnes C, et al. Correlations between sleep disturbance and brain structures associated with neurodegeneration in the national Alzheimer's coordinating center uniform data set. J Clin Neurosci. (2022) 106:204–12. doi: 10.1016/j.jocn.2022.07.012
174. Oh JY, Walsh CM, Ranasinghe K, Mladinov M, Pereira FL, Petersen C, et al. Subcortical neuronal correlates of sleep in neurodegenerative diseases. JAMA Neurol. (2022) 79:498–508. doi: 10.1001/jamaneurol.2022.0429
175. Bhuniya S, Goyal M, Chowdhury N, Mishra P. Intermittent hypoxia and sleep disruption in obstructive sleep apnea increase serum tau and amyloid-beta levels. J Sleep Res. (2022) 31:e13566. doi: 10.1111/jsr.13566
176. Sun H, Gao Y, Li M, Zhang S, Shen T, Yuan X, et al. Altered amyloid-β and tau proteins in neural-derived plasma exosomes in obstructive sleep apnea. Sleep Med. (2022) 94:76–83. doi: 10.1016/j.sleep.2022.03.021
177. Shieu MM, Zaheed A, Shannon C, Chervin RD, Conceicao A, Paulson HL, et al. Positive airway pressure and cognitive disorders in adults with obstructive sleep apnea: a systematic review of the literature. Neurology. (2022) 99:e334–46. doi: 10.1212/WNL.0000000000200383
178. Kirsh L, Cox R, Mongue-Din H, Lawrence R. The prevalence of obstructive sleep apnoea risk factors in individuals with cognitive impairment: south London cross-sectional study. BJPsych Bull. (2022) 45:93–7. doi: 10.1192/bjb.2022.38
179. Liguori C, Mercuri NB, Nuccetelli M, Izzi F, Cordella A, Bernardini S, et al. Obstructive sleep apnea may induce orexinergic system and cerebral β-amyloid metabolism dysregulation: is it a further proof for Alzheimer's disease risk? Sleep Med. (2019) 56:171–6. doi: 10.1016/j.sleep.2019.01.003
180. Chong PLH, Garic D, Shen MD, Lundgaard I, Schwichtenberg AJ. Sleep, cerebrospinal fluid, and the glymphatic system: a systematic review. Sleep Med Rev. (2022) 61:101572. doi: 10.1016/j.smrv.2021.101572
181. Lal C, Ayappa I, Ayas N, Beaudin AE, Hoyos C, Kushida CA, et al. The link between obstructive sleep apnea and neurocognitive impairment: an official American thoracic society workshop report. Ann Am Thorac Soc. (2022) 19:1245–56. doi: 10.1513/AnnalsATS.202205-380ST
182. Coulson RL, Mourrain P, Wang GX. Sleep deficiency as a driver of cellular stress and damage in neurological disorders. Sleep Med Rev. (2022) 63:101616. doi: 10.1016/j.smrv.2022.101616
183. Ramduny J, Bastiani M, Huedepohl R, Sotiropoulos SN, Chechlacz M. The association between inadequate sleep and accelerated brain ageing. Neurobiol Aging. (2022) 114:1–14. doi: 10.1016/j.neurobiolaging.2022.02.005
184. Marcus M, Yasamy MT, Ommeren M, Chisholm D, Saxena S. Depression: A Global Public Health Concern. World Health Organization Paper on Depression (2012). Available online at: https://www.who.int/mental_health/management/depression/who_paper_depression_wfmh_2012.pdf (accessed December 14, 2022).
185. Lee MJ, Nho WY, Jung H, Cho JW, Seo JS, Lee HM, et al. High prevalence of depression and sleep-wake disorders among female emergency medicine residents in South Korea. Ann Med. (2022) 54:846–55. doi: 10.1080/07853890.2022.2053568
186. Chen RF, Cai Y, Zhu ZH, Hou WL, Chen P, Wang J, et al. Sleep disorder as a clinical risk factor of major depression: associated with cognitive impairment. Asian J Psychiatr. (2022) 76:103228. doi: 10.1016/j.ajp.2022.103228
187. Mbous YPV, Nili M, Mohamed R, Dwibedi N. Psychosocial correlates of insomnia among college students. Prev Chronic Dis. (2022) 19:E60. doi: 10.5888/pcd19.220060
188. Xu G, Li X, Xu C, Xie G, Liang J. Effect of insomnia in the major depressive disorder. BMC Neurol. (2022) 22:341. doi: 10.1186/s12883-022-02869-x
189. Villela Nunes PV, Suemoto CK, Rodriguez RD, Paraizo Leite RE, Nascimento C, Pasqualucci CA, et al. Neuropathology of depression in non-demented older adults: a large postmortem study of 741 individuals. Neurobiol Aging. (2022) 117:107–16. doi: 10.1016/j.neurobiolaging.2022.05.007
190. Sarkis RA, Lucey BP. Moving towards core sleep outcomes in neurodegenerative disease-the time is now. Sleep. (2022) 45:zsac112. doi: 10.1093/sleep/zsac112
191. Neylan TC, Walsh CM. Sleep spindles, tau, and neurodegeneration. Sleep. (2022) 45:zsac161. doi: 10.1093/sleep/zsac161
192. Mander BA, Dave A, Lui KK, Sprecher KE, Berisha D, Chappel-Farley MG, et al. Inflammation, tau pathology, and synaptic integrity associated with sleep spindles and memory prior to β-amyloid positivity. Sleep. (2022) 45:zsac135. doi: 10.1093/sleep/zsac135
193. Blackman J, Morrison HD, Lloyd K, Gimson A, Banerjee LV, Green S, et al. The past, present, and future of sleep measurement in mild cognitive impairment and early dementia-towards a core outcome set: a scoping review. Sleep. (2022) 5:zsac077. doi: 10.1093/sleep/zsac077
194. Katsuki F, Gerashchenko D, Brown RE. Alterations of sleep oscillations in Alzheimer's disease: a potential role for GABAergic neurons in the cortex, hippocampus, and thalamus. Brain Res Bull. (2022) 187:181–98. doi: 10.1016/j.brainresbull.2022.07.002
195. Finlayson-Pitts BJ. Atmospheric chemistry. Proc Natl Acad Sci USA. (2010) 107:6566–7. doi: 10.1073/pnas.1003038107
196. McNeill VF. Atmospheric aerosols: clouds, chemistry, and climate. Annu Rev Chem Biomol Eng. (2017) 8:427–44. doi: 10.1146/annurev-chembioeng-060816-101538
197. Singh S A, Suresh S, Singh A, Chandran L, Vellapandian C. Perspectives of ozone induced neuropathology and memory decline in Alzheimer's disease: a systematic review of preclinical evidences. Environ Pollut. (2022) 313:120136. doi: 10.1016/j.envpol.2022.120136
198. Bessagnet B, Allemand N, Putaud JP, Couvidat F, André JM, Simpson D, et al. Emissions of carbonaceous particulate matter and ultrafine particles from vehicles-a scientific review in a cross-cutting context of air pollution and climate change. Appl Sci. (2022) 12:1–52. doi: 10.3390/app12073623
199. Holmén BA, Ayala A. Ultrafine PM emissions from natural gas, oxidation-catalyst diesel, and particle-trap diesel heavy-duty transit buses. Environ Sci Technol. (2002) 36:5041–50. doi: 10.1021/es015884g
200. Sahu M, Tripathi R, Jha NK, Jha SK, Ambasta RK, Kumar P. Cross talk mechanism of disturbed sleep patterns in neurological and psychological disorders. Neurosci Biobehav Rev. (2022) 140:104767. doi: 10.1016/j.neubiorev.2022.104767
201. Abdalla RN, Ansari SA, Hurley MC, Attarian H, Fargen KM, Hirsh JA, et al. Correlation of call burden and sleep deprivation with physician burnout, driving crashes, and medical errors among US neurointerventionalists. AJNR Am J Neuroradiol. (2022) 43:1286–91. doi: 10.3174/ajnr.A7606
202. Lin SX, Cheslack-Postava K, McReynolds L, Amsel L, Bresnahan M, Hoven CW. Adverse childhood experiences and insufficient sleep among U.S. children and adolescents. Acad Pediatr. (2022) 22:965–71. doi: 10.1016/j.acap.2022.02.007
203. Chapman DP, Liu Y, Presley-Cantrell LR, Edwards VJ, Wheaton AG, Perry GS, et al. Adverse childhood experiences and frequent insufficient sleep in 5 U.S. States, 2009: a retrospective cohort study. BMC Public Health. (2013) 13:3. doi: 10.1186/1471-2458-13-3
204. Giddens NT, Juneau P, Manza P, Wiers CE, Volkow ND. Disparities in sleep duration among American children: effects of race and ethnicity, income, age, and sex. Proc Natl Acad Sci USA. (2022) 119:e2120009119. doi: 10.1073/pnas.2120009119
205. Semenza DC, Meldrum RC, Testa A, Jackson DB. Sleep duration, depressive symptoms, and digital self-harm among adolescents. Child Adolesc Ment Health. (2022) 27:103–10. doi: 10.1111/camh.12457
206. Deshpande P, Salcedo B, Haq C. Common sleep disorders in children. Am Fam Physician. (2022) 105:168–76.
207. Stahl ST, Insana SP, Hall MH, Buysse DJ. Pediatric sleep disturbances and symptoms of depression in older adults. Int J Aging Hum Dev. (2022) 95:429–39. doi: 10.1177/00914150221077950
208. Logan RW, Hasler BP, Forbes EE, et al. Impact of sleep and circadian rhythms on addiction vulnerability in adolescents. Biol Psychiatry. (2018) 83:987–96. doi: 10.1016/j.biopsych.2017.11.035
209. Petermann-Rocha F, Valera-Gran D, Fernández-Pires P, Martens DS, Júlvez J, Rodriguez-Dehli C, et al. Children who sleep more may have longer telomeres: evidence from a longitudinal population study in Spain. Pediatr Res. (2022). doi: 10.1038/s41390-022-02255-w. [Epub ahead of print].
210. Gładka A, Zatoński T, Rymaszewska J. Association between the long-term exposure to air pollution and depression. Adv Clin Exp Med. (2022) 31:1139–52. doi: 10.17219/acem/149988
211. Gładka A, Rymaszewska J, Zatoński T. Impact of air pollution on depression and suicide. Int J Occup Med Environ Health. (2018) 31:711–21. doi: 10.13075/ijomeh.1896.01277
212. Michalak I, Dziergowska K, Alagawany M, Farag MR, El-Shall NA, Tuli HS, et al. The effect of metal-containing nanoparticles on the health, performance and production of livestock animals and poultry. Vet Q. (2022) 42:68–94. doi: 10.1080/01652176.2022.2073399
213. Keerthana DM, Karmegam N, Manikandan S, Subbaiya R, Song H, Kwon EE, et al. Removal of nanoplastics in water treatment processes: a review. Sci Total Environ. (2022) 845:157168. doi: 10.1016/j.scitotenv.2022.157168
214. Dong L, Chen G, Liu G, Huang X, Xu XM, Li L, et al. A review on recent advances in the applications of composite Fe3O4 magnetic nanoparticles in the food industry. Crit Rev Food Sci Nutr. (2022). 1–29. doi: 10.1080/10408398.2022.2113363. [Epub ahead of print].
215. Kumar P, Pirjola L, Ketzel M, Harrison RM. Nanoparticle emissions from 11 non-vehicle exhaust sources – a review. Atmosph Environ. (2013) 67:252–77. doi: 10.1016/j.atmosenv.2012.11.011
216. Biswas P, Wu CY. Nanoparticles and the environment. J Air Waste Manag Assoc. (2005) 55:708–46. doi: 10.1080/10473289.2005.10464656
Keywords: air pollution, Alzheimer's, nanoparticles, nanoneuropathology, PM2.5, sleep disorders RBD, OSA, depression
Citation: Calderón-Garcidueñas L, Torres-Jardón R, Greenough GP, Kulesza R, González-Maciel A, Reynoso-Robles R, García-Alonso G, Chávez-Franco DA, García-Rojas E, Brito-Aguilar R, Silva-Pereyra HG, Ayala A, Stommel EW and Mukherjee PS (2023) Sleep matters: Neurodegeneration spectrum heterogeneity, combustion and friction ultrafine particles, industrial nanoparticle pollution, and sleep disorders—Denial is not an option. Front. Neurol. 14:1117695. doi: 10.3389/fneur.2023.1117695
Received: 06 December 2022; Accepted: 01 February 2023;
Published: 27 February 2023.
Edited by:
Anna Maria Lavezzi, University of Milan, ItalyReviewed by:
Harm Heusinkveld, National Institute for Public Health and the Environment, NetherlandsLang Tran, Institute of Occupational Medicine, United Kingdom
Irena Maria Nalepa, Maj Institute of Pharmacology (PAN), Poland
Copyright © 2023 Calderón-Garcidueñas, Torres-Jardón, Greenough, Kulesza, González-Maciel, Reynoso-Robles, García-Alonso, Chávez-Franco, García-Rojas, Brito-Aguilar, Silva-Pereyra, Ayala, Stommel and Mukherjee. This is an open-access article distributed under the terms of the Creative Commons Attribution License (CC BY). The use, distribution or reproduction in other forums is permitted, provided the original author(s) and the copyright owner(s) are credited and that the original publication in this journal is cited, in accordance with accepted academic practice. No use, distribution or reproduction is permitted which does not comply with these terms.
*Correspondence: Lilian Calderón-Garcidueñas, lilian.calderon-garciduenas@umontana.edu