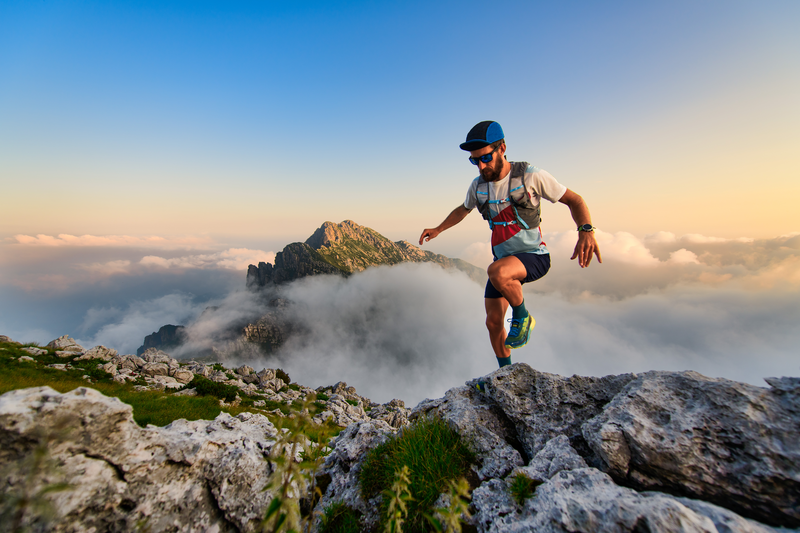
94% of researchers rate our articles as excellent or good
Learn more about the work of our research integrity team to safeguard the quality of each article we publish.
Find out more
REVIEW article
Front. Neurol. , 02 June 2023
Sec. Movement Disorders
Volume 14 - 2023 | https://doi.org/10.3389/fneur.2023.1046910
Parkinson’s disease (PD) is the second most common neurodegenerative disease globally. Levodopa (L-dopa) has been the cornerstone for treating Parkinson’s since the 1960s. However, complications such as “wearing-off” and dyskinesia inevitably appear with disease progression. With the further development of microbiomics in recent years, It has been recognized that gut microbiota plays a crucial role in Parkinson’s disease pathogenesis. However, Little is known about the impact of gut microbiota in PD treatment, especially in levodopa metabolism. This review examines the possible mechanisms of gut microbiota, such as Helicobacter pylori, Enterobacter faecalis, and Clostridium sporogenes, affecting L-dopa absorption. Furthermore, we review the current status of gut microbiota intervention strategies, providing new insights into the treatment of PD.
Parkinson’s disease (PD) is the most rapidly growing disorder worldwide and will affect 12 million people by 2024 (1). The pathology is characterized by dopaminergic neurodegeneration and misfolded synuclein accumulation. Since its introduction in the 1960s, L-dopa (L-dopa) has been the gold standard for treating Parkinson’s disease (2). Most Parkinson’s patients are eventually treated with L-dopa at different stages of the disease. Dopamine, the metabolite of L-dopa decarboxylase, is responsible for improving Parkinson’s disease symptoms. However, L-dopa is mainly decarboxylated in peripheral tissues, especially in the gastrointestinal tract; only 1%–5% of L-dopa eventually reaches the central system (3). On the one hand, peripheral L-dopa metabolism reduces its bioavailability and clinical effectiveness; on the other hand, it causes various complications such as orthostatic hypotension and arrhythmia (4). Therefore, L-dopa is routinely combined with decarboxylase inhibitors to reduce peripheral metabolism and enhance its bioavailability. However, even in the presence of decarboxylase inhibitors, up to half of levodopa is still metabolized in the periphery (5, 6). It is an urgent problem to increase the L-dopa bioavailability.
The gastrointestinal tract is an important site of mutual contact between humans and the environment. More than 100 trillion microorganisms reside in the gut, forming a complex community collectively known as the gut microbiota (7). These microorganisms have a wide range of physiological functions, host immunity regulation, and intestinal epithelial integrity maintenance (8). In particular, the microorganism contribution to drug metabolism is receiving increasing attention. Currently, There is already evidence that intestinal microorganisms such as Enterobacter faecalis, Helicobacter pylori, and Clostridium sporogenes can affect the metabolism and absorption of L-dopa (9–11). Moreover, interventions targeting gut microbiota have been proved to improve the clinical efficacy of L-dopaincluding antibiotics, dietary interventions, and Fecal microbiota transplantation (FMT) (12–14). Therefore, future researches are expected to conduct interventions for gut microbiota as the potential therapeutic target for PD. This review elaborates on the mechanisms of gut microbiota affecting L-dopa metabolism and emphasizes currently available intervention strategies targeting gut microbiota.
This review is aimed to discuss the link between gut microbiota and levodopa absorption and metabolism, focusing on the contribution of modulating gut microbiota to improve the efficacy of levodopa in clinical studies. Based on previous basic studies, we first identified the gut microbiota involved in levodopa metabolism (Table 1), and we then searched the articles between 2000 and 2021 based on the different combination of search terms “eradication bacteria” “levodopa” and “Parkinson’s disease”and obtained a total of 50 articles. Then, we excluded reviews and irrelevant studies after including a total of 21 studies after reading the abstract or title. Finally, a total of 10 studies were included based on the content of the studies after reading the full text. More details of the method are shown in Figure 1. The characteristics of 10 studies are shown in Table 2.
Parkinson’s disease is multisystemic and imposes a severe burden on society. Apart from classic motor symptoms, non-motor symptoms are highly prevalent, including gastrointestinal and autonomic dysfunction (29, 30). Moreover, About 80% of PD patients experience gastrointestinal symptoms before experiencing motor symptoms (31). There is increasing evidence that gut microbiota can contribute to synucleinopathy and inflammation (32, 33). Therefore, Targeting the gut microbiota holds great promise for PD treatment.
There have been more than 20 studies comparing the gut microbiota composition of PD patients with those of healthy controls. Moreover, more than 100 research have documented remarkable variations in α- and β-diversity as well as the relationship between disease severity and gut microbiota abundance (34, 35). Although there is no consensus on the microbiota composition in PD, It has been reported that Lactobacillus and Akkermansia have been enriched, while Prevotella and Faecalibacterium have been reduced in many studies (36–38). Notably, the microbiota composition of PD patients varies significantly due to their different regions and population. For example, the Lactobacillaceae family is universally enriched in western PD patients and less frequently found in china PD patients (39–41). However, whether gut microbiota alterations are a cause or a consequence of PD remains unclear.
The pathogenesis of Parkinson’s disease appears to be strongly influenced by immunity. The inflammatory response in PD used to be thought to originate in the brain, however, there is a new concept that inflammation originates from the gastrointestinal tract. Since Braak (42) first proposed a gut-driven model, the gut microbiota facilitates the α-synuclein formation and transfer from the periphery to the brain. Increasing animal models confirmed that microbiota dysbiosis might trigger an inflammatory cascade response, leading to α-synuclein aggregation and dopaminergic neuron damage (43–45).
The gut microbiota contributes to immunity and inflammation through the following mechanisms: (i) gut microbiota dysbiosis affects microglia function. Gut microbiota dysbiosis can influence the microglia’s maturation and function, exacerbate neuroinflammation and promote the development of PD (46). (ii) Gut microbiota dysbiosis promotes abnormal activation of toll-like receptors (TLR). Lipopolysaccharides (LPS), endotoxins produced by gut microbiota, is an essential ligand of TLRs to increase microglia activation and release pro-inflammatory cytokines, causing an inflammatory response and aggravating substantia nigra (SN) damage (47, 48). (iii) Gut microbiota dysbiosis lead to the disturbance of metabolites. Short-chain fatty acids (SCFA), gut microbiota metabolites, contribute to energy metabolism and intestinal homeostasis. SCFA, especially butyrate, the primary energy substrate for colonic epithelial cells, is associated with intestinal barrier integrity (49, 50). Moreover, SCFA can promote microglia activation and α-syn aggregation in the central system (33).
L-dopa has been the cornerstone of PD drug treatment since the 1960s. After oral administration and passing through the stomach, L-dopa is absorbed in the duodenum and proximal jejunum. Notably, levodopa transport is mediated by an amino acid transport carrier, especially the SLC7A9-SLC3A1 (apical), SLC7A8-SLC3A2 (blood-brain barrier, basolateral), and SLC16A10 (basolateral) (51, 52).
Levodopa can compete with other neutral amino acids for binding carriers; therefore, avoid combining levodopa with dietary amino acids to reduce competition for transport in the intestine and blood-brain barrier. Most orally administered levodopa is metabolized in the periphery and distributed by skeletal muscles, only 1% of L-dopa can successfully enter the brain (53). Although combined L-dopa and decarboxylase inhibitors can reduce peripheral metabolism and increase effective levels in the brain, they still cannot meet clinical needs. Non-physiological administration will cause pulse-like stimulation of dopamine receptors on postsynaptic membranes, resulting in changes in striatal signal output pathways that ultimately lead to motor complications (54). Thus, improving the pharmacodynamics of L-dopa administration is essential for optimizing PD treatment.
Since the 1970s, There was already evidence that the gut microbiota can metabolize levodopa (55). Until recently, researchers identified the specific gut microbes, genes, and enzymes involved in levodopa metabolism. Tyrosine decarboxylase (TDC), mainly produced by Enterococcus faecalis, converts L-dopa to dopamine in the intestine (16) (15). Moreover, the microbiota-dependent conversion is not affected by aromatic L-amino acid decarboxylase (AADC) inhibitors. Furthermore, TDC expression was positively correlated with daily dose of levodopa and negatively correlated with plasma levodopa levels (16). Rekdal et al. (15) also confirmed that AADC inhibitors did not prevent microbiota-dependent conversion. In addition, they identified a particular compound, alpha-fluoromethyltyrosine (AFMT), which effectively blocks the microbiota-dependent conversion. These two seminal studies bring new insight into traditional AADC inhibitor supplementary therapy. We believe we can further reduce peripheral metabolism, increase central levodopa levels, and improve clinical efficacy by modulating gut microbiota in the future.
It has been proven that Helicobacter pylori (HP) can affect L-dopa’s efficacy, and symptoms improved after HP eradication (20, 21, 56). Lee et al. (22) conducted an observational study of HP and PD. The study found a considerable increase in L-dopa “on-time” after HP eradication in PD patients (58.1 ± 25.6 ~ 234.4 ± 66.5 min). Similar results were reported in another study, the onset time of L-dopa was shortened by 14 min, and the average on-time was increased by 38 min after 12 weeks of HP eradication. Moreover, the unified Parkinson’s disease rating scale (UPDRS) and Parkinson’s disease questionnaire 39 (PDQ-39) were significantly improved (26). Liu and colleagues (27) conducted long-term research (one year) on HP eradication and reported similar results. Patients with HP-positive PD had significantly lower UPDRS scores one year after HP eradication, especially in the fine motor aspect of the hands and feet. We speculate that the mechanisms of HP affect levodopa efficacy in the following ways: one possibility is that HP infection may delay gastric emptying, resulting in delayed L-dopa entry and impaired L-dopa transport in the duodenum (17). In addition, HP can use L-dopa as a supplement for its own growth to maintain its ecological niche (57). Finally, The vitro studies have shown that HP adhesins can directly interact with L-dopa, leading to a reduction in the effective concentration (19, 58). However, in a recent double-blind controlled trial, there was no remarkable improvement in motor and non-motor symptoms after HP eradication (13). This result contradicts the previous study, and further larger samples with strict screening criteria and follow-up are needed to demonstrate the therapeutic importance of HP eradication in PD patients. Although it is questionable whether HP eradication provides long-term benefits for PD, we need to be aware that HP infection can cause a variety of gastric diseases and even gastric cancer. Therefore, it is advocated that PD patients with motor fluctuations or wearing-off should go for HP detection and treatment.
As previously mentioned, the vast majority is delivered to the circulatory system by intestinal amino acid transport carriers and only 10% of unabsorbed residual L-dopa remains in the intestine as a substrate for other bacterial species (3). However, researchers found that microbiota can interact with residual levodopa and influence intestinal homeostasis through its metabolites. van Kessel et al. (10) found that Clostridium sporogenes can deaminate L-dopa to 3-(3,4-dihydroxy phenyl) propionic acid that can inhibit ileal motility in vitro. It is crucial to identify the potential effects of bacteria on drug metabolism to improve efficacy and reduce unwanted side effects.
Small intestinal bacterial overgrowth (SIBO) refers to gut dysbiosis marked by a high number or unusual type of bacteria in the small intestine. Although SIBO is not a disease, it can cause a wide range of gastrointestinal symptoms, for example, abdominal distension, burping, flatulence, diarrhea, and constipation. Eleven studies had reported the prevalence of SIBO in PD ranging from 46%–67% (59). SIBO has been reported to have more severe motor functions and longer off-time compared to PD without SIBO (25, 60). Furthermore, Significant improvement in motor dysfunction in patients with Parkinson’s disease after the SIBO eradication in a non-randomized trial (25). The same results were also reported in another prospective study, where motor function improved (p < 0.05)after the correction of SIBO with FMT (61). SIBO-induced peripheral inflammation increases intestinal permeability and bacterial translocation, triggering microglia activation and enhancing alpha-synuclein aggregation, which plays an important role in the pathogenesis of PD (62, 63). In addition, SIBO-induced inflammation can act on enterochromaffin-like cells and cause delayed gastric emptying (64). More importantly, SIBO can cause inflammation of the proximal jejunum (the main absorption site of levodopa) and impair levodopa absorption (65). In conclusion, the eradication of SIBO serves as a potential disease-modifying intervention to slow the progression of PD and optimize Parkinson’s treatment by improving L-dopa efficacy.
Obviously, Traditional oral formulations of levodopa no longer meet current needs, and new formulations hold great promise for the future. Accordion Pill CD-L-dopa, a new oral formulation of gastric retention extended-release with unique multi-layer planar structure, retains in the stomach for up to 12 h and improves daily “off-time” (66). However, whether the drug is affected by delayed gastric emptying and gut microbiota is not known. Additionally, Non-oral formulations have been extensively studied. (i) intestinal route: L-dopa-carbidopa intestinal gel (LCIG), continuously transported in the jejunum via an external pump, can effectively reduce motor fluctuations and improves “on” time (67). (ii) Transcutaneous route: ND0612 is administered subcutaneously via a pump-patch device for steady plasma levels. It has been reported to be well tolerated and ease dyskinesia (68). (iii) Inhalation route: CVT-301 is a powder formulation of levodopa delivered by a breath-driven inhaler. CVT-301 rapidly increases the plasma concentration of L-dopa with less fluctuation in blood concentration (69). However, it is worth noting that whether intranasal microorganisms affect the efficacy of inhaled formulations deserves further study. Overall non-oral formulations allow levodopa to enter the body’s circulation more quickly and reliably than traditional oral formulations (new formulations of L-dopa in Table 3).
Novel strategies targeting gut microbiota in treating PD focus on normalizing abnormal bacteria and reducing neuroinflammation and neuronal damage, slowing the course of the disease. Several studies have shown that dietary interventions, probiotics, and prebiotics are beneficial in treating PD (73–75). Dietary interventions and supplementing omega-3 fatty acids and vitamins can accelerate CNS barrier repair, reduce neuroinflammation, and slow down neurodegenerative processes. Evidence suggests that omega-3 fatty acids indirectly block the activation of TLR4 and other TLRs, which reduces mitochondrial dysfunction and the accumulation of synaptic nuclides (76). In addition, it was found that omega-3 fatty acids could attenuate the damage of dopaminergic neurons by LPS by inhibiting NF-κB activation (77). Furthermore, probiotics have been shown to increase glucose metabolism, reduce peripheral inflammation, and improve motor and non-motor function in pre- and post-clinical studies (78, 79). Besides, FMT can also rebuild gut microbiota and maintain intestinal homeostasis. In our previous study, FMT reduced the expression of α-syn and inhibited the activation of microglia in SN (80). Specific decarboxylase inhibitors AFMT, engineered gut bacteria, live bacteria drugs, and synthetic bacteria, may also have potential therapeutic value for PD. Consequently, these novel potential treatment approaches must undergo carefully designed randomized clinical trials to determine their benefits and safety.
As mentioned above, It is promising to reduce drug metabolism and inflammation in the intestine by inhibiting specific bacteria. Antibiotics are chemicals that can inhibit or eliminate certain microorganisms. Moreover, antibiotics have now been repurposed because of their anti-inflammatory and neuroprotective properties. Rifaximin is a broad-spectrum antibiotic that is considered an effective treatment for SIBO due to its broad spectrum (81). In addition, Rifampicin has been found to cross the BBB in animal models, prevent neurodegeneration of nigrostriatal dopaminergic neurons, reduce microglial inflammation, and improve mitochondrial dysfunction (82, 83). Tetracyclines are broad-spectrum antibiotics and can eliminate microbiota in high concentrations. It could reduce pro-inflammatory molecules and inhibit matrix metalloproteinase activity (84, 85). Moreover, ceftriaxone (CEF) is a b-lactam antibiotic most frequently used in hospital-acquired infections. Recent studies have highlighted the therapeutic effects of CEF on neurodegenerative diseases. For example, CEF can modulate inflammation and gut microbiota and has neuroprotective effects in PD mice (86). However, It is unclear which antibiotic type and dose is appropriate for patients with PD.
L-dopa is the primary medication for PD and can improve motor symptoms in the different stages of the disease. However, as the disease progresses, there are complications such as decreased efficacy and dyskinesia, and L-dopa will not continue to delay progression. The interaction between gut bacteria and drugs may offer new insights into treating Parkinson’s disease.
Based on this perspective, microbiome research offers a bright future for the field. Looking toward the future, we should explore various study designs that manipulate the gut microbiota, including using selective antibiotics to reduce the abundance of TDC, using probiotics to repopulate gut bacteria, and exploring the potential role of fecal bacteria transplantation. There is limited evidence supporting these beneficial effects in preclinical and clinical settings. Thus, it requires more high-quality, multicenter clinical trials to determine efficacy and safety.
ZZ contributed to the writing and conceptualization of the manuscript, including all figures and data involved. MY and FLY revised the content and format of the manuscript and made equally important contributions. All authors contributed to the article and approved the submitted version.
I want to express my thanks to all those who have helped me. First, special thanks to MY, from whose guidance I have greatly benefited. I am especially indebted to FY, who encouraged me and provided helpful advice. I am also grateful to my friends and family for their encouragement and financial support.
The authors declare that the research was conducted in the absence of any commercial or financial relationships that could be construed as a potential conflict of interest.
All claims expressed in this article are solely those of the authors and do not necessarily represent those of their affiliated organizations, or those of the publisher, the editors and the reviewers. Any product that may be evaluated in this article, or claim that may be made by its manufacturer, is not guaranteed or endorsed by the publisher.
1. Dorsey, E, Sherer, T, Okun, M, and Bloem, B. The emerging evidence of the Parkinson pandemic. J Parkinsons Dis. (2018) 8:S3–8. doi: 10.3233/JPD-181474
2. Vijiaratnam, N, Simuni, T, Bandmann, O, Morris, H, and Foltynie, T. Progress towards therapies for disease modification in Parkinson's disease. Lancet Neurol. (2021) 20:559–72. doi: 10.1016/S1474-4422(21)00061-2
3. Morgan, JP, Bianchine, JR, Spiegel, HE, Rivera-Calimlim, L, and Hersey, RM. Metabolism of levodopa in patients with Parkinson's disease. Radioactive and fluorometric assays. Arch Neurol. (1971) 25:39–44. doi: 10.1001/archneur.1971.00490010049007
4. Nyholm, D, and Lennernäs, H. Irregular gastrointestinal drug absorption in Parkinson's disease. Expert Opin Drug Metab Toxicol. (2008) 4:193–203. doi: 10.1517/17425255.4.2.193
6. Tissot, R, Gaillard, JM, Guggisberg, M, Gauthier, G, and De Ajuriaguerra, J. Therapy of Parkinson's syndrome with L-dopa per OS combined with a decarboxylase inhibitor (Ro 46.02). Neurol Neurocir Psiquiatr. (1969) 10:1–25.
7. Chen, Y, Zhou, J, and Wang, L. Role and mechanism of gut microbiota in human disease. Front Cell Infect Microbiol. (2021) 11:625913. doi: 10.3389/fcimb.2021.625913
8. Adak, A, and Khan, MR. An insight into gut microbiota and its functionalities. Cell Mol Life Sci. (2019) 76:473–93. doi: 10.1007/s00018-018-2943-4
9. Nyholm, D, and Hellström, P. Effects of Helicobacter pylori on levodopa pharmacokinetics. J Parkinsons Dis. (2021) 11:61–9. doi: 10.3233/JPD-202298
10. van Kessel, S, de Jong, HR, Winkel, S, van Leeuwen, S, Nelemans, S, Permentier, H, et al. Gut bacterial deamination of residual levodopa medication for Parkinson's disease. BMC Biol. (2020) 18:137. doi: 10.1186/s12915-020-00876-3
11. Xiang, S, Ji, J, Li, S, Cao, X, Xu, W, Tan, L, et al. Efficacy and safety of probiotics for the treatment of Alzheimer's disease, mild cognitive impairment, and Parkinson's disease: a systematic review and meta-analysis. Front Aging Neurosci. (2022) 14:730036. doi: 10.3389/fnagi.2022.730036
12. Tamtaji, OR, Taghizadeh, M, Daneshvar Kakhaki, R, Kouchaki, E, Bahmani, F, Borzabadi, S, et al. Clinical and metabolic response to probiotic administration in people with Parkinson's disease: a randomized, double-blind, placebo-controlled trial. Clin Nutr. (2019) 38:1031–5. doi: 10.1016/j.clnu.2018.05.018
13. Tan, AH, Lim, S-Y, Mahadeva, S, Loke, MF, Tan, JY, Ang, BH, et al. Helicobacter pylori eradication in Parkinson's disease: a randomized placebo-controlled trial. Mov Disord. (2020) 35:2250–60. doi: 10.1002/mds.28248
14. Xue, L-J, Yang, X-Z, Tong, Q, Shen, P, Ma, S-J, Wu, S-N, et al. Fecal microbiota transplantation therapy for Parkinson's disease: a preliminary study. Medicine. (2020) 99:E22035. doi: 10.1097/MD.0000000000022035
15. Maini Rekdal, V, Bess, EN, Bisanz, JE, Turnbaugh, PJ, and Balskus, EP. Discovery and inhibition of an interspecies gut bacterial pathway for levodopa metabolism. Science. (2019) 364:eaau6323. doi: 10.1126/science.aau6323
16. van Kessel, SP, Frye, AK, El-Gendy, AO, Castejon, M, Keshavarzian, A, van Dijk, G, et al. Gut bacterial tyrosine decarboxylases restrict levels of levodopa in the treatment of Parkinson's disease. Nat Commun. (2019) 10:310. doi: 10.1038/s41467-019-08294-y
17. Lolekha, P, Sriphanom, T, and Vilaichone, R-K. Helicobacter pylori eradication improves motor fluctuations in advanced Parkinson's disease patients: a prospective cohort study (HP-PD trial). PLoS One. (2021) 16:E0251042. doi: 10.1371/journal.pone.0251042
18. Mridula, KR, Borgohain, R, Chandrasekhar Reddy, V, Bandaru, V, and Suryaprabha, T. Association of Helicobacter pylori with Parkinson's disease. J Clin Neurol. (2017) 13:181–6. doi: 10.3988/jcn.2017.13.2.181
19. Niehues, M, and Hensel, A. In-vitro interaction of L-dopa with bacterial Adhesins of Helicobacter pylori: an explanation for clinicial differences in bioavailability? J Pharm Pharmacol. (2009) 61:1303–7. doi: 10.1211/jpp/61.10.0005
20. Pierantozzi, M, Pietroiusti, A, Galante, A, Sancesario, G, Lunardi, G, Fedele, E, et al. Helicobacter pylori-induced reduction of acute levodopa absorption in Parkinson's disease patients. Ann Neurol. (2001) 50:686–7. doi: 10.1002/ana.1267
21. Pierantozzi, M, Pietroiusti, A, Brusa, L, Galati, S, Stefani, A, Lunardi, G, et al. Helicobacter pylori eradication and L-dopa absorption in patients with Pd and motor fluctuations. Neurology. (2006) 66:1824–9. doi: 10.1212/01.wnl.0000221672.01272.ba
22. Lee, WY, Yoon, WT, Shin, HY, Jeon, SH, and Rhee, P-L. Helicobacter pylori infection and motor fluctuations in patients with Parkinson's disease. Mov Disord. (2008) 23:1696–700. doi: 10.1002/mds.22190
23. Dobbs, SM, Dobbs, RJ, Weller, C, Charlett, A, Bjarnason, IT, Lawson, AJ, et al. Differential effect of Helicobacter pylori eradication on time-trends in Brady/Hypokinesia and rigidity in idiopathic parkinsonism. Helicobacter. (2010) 15:279–94. doi: 10.1111/j.1523-5378.2010.00768.x
24. Dobbs, SM, Charlett, A, Dobbs, RJ, Weller, C, Iguodala, O, Smee, C, et al. Antimicrobial surveillance in idiopathic parkinsonism: indication-specific improvement in Hypokinesia following Helicobacter pylori eradication and non-specific effect of antimicrobials for other indications in worsening rigidity. Helicobacter. (2013) 18:187–96. doi: 10.1111/hel.12035
25. Fasano, A, Bove, F, Gabrielli, M, Petracca, M, Zocco, MA, Ragazzoni, E, et al. The role of small intestinal bacterial overgrowth in Parkinson's disease. Mov Disord. (2013) 28:1241–9. doi: 10.1002/mds.25522
26. Hashim, H, Azmin, S, Razlan, H, Yahya, NW, Tan, HJ, Manaf, MRA, et al. Eradication of Helicobacter pylori infection improves levodopa action, clinical symptoms and quality of life in patients with Parkinson's disease. PLoS One. (2014) 9:E112330. doi: 10.1371/journal.pone.0112330
27. Liu, H, Su, W, Li, S, Du, W, Ma, X, Jin, Y, et al. Eradication of Helicobacter pylori infection might improve clinical status of patients with Parkinson's disease, especially on bradykinesia. Clin Neurol Neurosurg. (2017) 160:101–4. doi: 10.1016/j.clineuro.2017.07.003
28. Mridula, KR, Borgohain, R, Chandrasekhar Reddy, V, Bandaru, VCh, and Suryaprabha, T. Association of Helicobacter pylori with Parkinson’s Disease. J. Clin. Neurol. 13:181–186. doi: 10.3988/jcn.2017.13.2.181
29. Pfeiffer, RF. Non-motor symptoms in Parkinson's disease. Parkinsonism Relat Disord. (2016) 22:S119–22. doi: 10.1016/j.parkreldis.2015.09.004
30. Schapira, AHV, Chaudhuri, KR, and Jenner, P. Non-motor features of Parkinson disease. Nat Rev Neurosci. (2017) 18:435–50. doi: 10.1038/nrn.2017.62
31. Cersosimo, MG, Raina, GB, Pecci, C, Pellene, A, Calandra, CR, Gutiérrez, C, et al. Gastrointestinal manifestations in Parkinson's disease: prevalence and occurrence before motor symptoms. J Neurol. (2013) 260:1332–8. doi: 10.1007/s00415-012-6801-2
32. Sampson, TR, Challis, C, Jain, N, Moiseyenko, A, Ladinsky, MS, Shastri, GG, et al. A gut bacterial amyloid promotes Α-synuclein aggregation and motor impairment in mice. eLife. (2020) 9:e53111. doi: 10.7554/eLife.53111
33. Sampson, TR, Debelius, JW, Thron, T, Janssen, S, Shastri, GG, Ilhan, ZE, et al. Gut microbiota regulate motor deficits and Neuroinflammation in a model of Parkinson's disease. Cells. (2016) 167:1469–1480.e12. doi: 10.1016/j.cell.2016.11.018
34. Aho, VTE, Pereira, PAB, Voutilainen, S, Paulin, L, Pekkonen, E, Auvinen, P, et al. Gut microbiota in Parkinson's disease: temporal stability and relations to disease progression. eBioMedicine. (2019) 44:691–707. doi: 10.1016/j.ebiom.2019.05.064
35. Romano, S, Savva, G, Bedarf, J, Charles, I, Hildebrand, F, and Narbad, A. Meta-analysis of the Parkinson's disease gut microbiome suggests alterations linked to intestinal inflammation. Npj Parkinson's Dis. (2021) 7:27. doi: 10.1038/s41531-021-00156-z
36. Unger, MM, Spiegel, J, Dillmann, K-U, Grundmann, D, Philippeit, H, Bürmann, J, et al. Short chain fatty acids and gut microbiota differ between patients with Parkinson's disease and age-matched controls. Parkinsonism Relat Disord. (2016) 32:66–72. doi: 10.1016/j.parkreldis.2016.08.019
37. Vascellari, S, Palmas, V, Melis, M, Pisanu, S, Cusano, R, Uva, P, et al. Gut microbiota and metabolome alterations associated with Parkinson's disease. mSystems. (2020) 5:e00561. doi: 10.1128/mSystems.00561-20
38. Wallen, ZD, Appah, M, Dean, MN, Sesler, CL, Factor, SA, Molho, E, et al. Characterizing dysbiosis of gut microbiome in Pd: evidence for overabundance of opportunistic pathogens. Npj Parkinson's Dis. (2020) 6:11. doi: 10.1038/s41531-020-0112-6
39. Li, C, Cui, L, Yang, Y, Miao, J, Zhao, X, Zhang, J, et al. Gut microbiota differs between Parkinson's disease patients and healthy controls in northeast China. Front Mol Neurosci. (2019) 12:171. doi: 10.3389/fnmol.2019.00171
40. Li, F, Wang, P, Chen, Z, Sui, X, Xie, X, and Zhang, J. Alteration of the fecal microbiota in north-eastern Han Chinese population with sporadic Parkinson's disease. Neurosci Lett. (2019) 707:134297. doi: 10.1016/j.neulet.2019.134297
41. Qian, Y, Yang, X, Xu, S, Wu, C, Song, Y, Qin, N, et al. Alteration of the fecal microbiota in Chinese patients with Parkinson's disease. Brain Behav Immun. (2018) 70:194–202. doi: 10.1016/j.bbi.2018.02.016
42. Braak, H, de Vos, RAI, Bohl, J, and Del Tredici, K. Gastric alpha-synuclein immunoreactive inclusions in Meissner's and Auerbach's plexuses in cases staged for Parkinson's disease-related brain pathology. Neurosci Lett. (2006) 396:67–72. doi: 10.1016/j.neulet.2005.11.012
43. Engler, H, Doenlen, R, Riether, C, Engler, A, Niemi, M-B, Besedovsky, HO, et al. Time-dependent alterations of peripheral immune parameters after nigrostriatal dopamine depletion in a rat model of Parkinson's disease. Brain Behav Immun. (2009) 23:518–26. doi: 10.1016/j.bbi.2009.01.018
44. Mayerhofer, R, Fröhlich, EE, Reichmann, F, Farzi, A, Kogelnik, N, Fröhlich, E, et al. Diverse action of lipoteichoic acid and lipopolysaccharide on neuroinflammation, blood-brain barrier disruption, and anxiety in mice. Brain Behav Immun. (2017) 60:174–87. doi: 10.1016/j.bbi.2016.10.011
45. Pellegrini, C, Antonioli, L, Calderone, V, Colucci, R, Fornai, M, and Blandizzi, C. Microbiota-gut-brain axis in health and disease: is NLRP3 inflammasome at the crossroads of microbiota-gut-brain communications? Prog Neurobiol. (2020) 191:101806. doi: 10.1016/j.pneurobio.2020.101806
46. Erny, D, Hrabě de Angelis, AL, Jaitin, D, Wieghofer, P, Staszewski, O, David, E, et al. Host microbiota constantly control maturation and function of microglia in the CNS. Nat Neurosci. (2015) 18:965–77. doi: 10.1038/nn.4030
47. García-Domínguez, I, Veselá, K, García-Revilla, J, Carrillo-Jiménez, A, Roca-Ceballos, MA, Santiago, M, et al. Peripheral inflammation enhances microglia response and Nigral dopaminergic cell death in an MPTP model of Parkinson's disease. Front Cell Neurosci. (2018) 12:398. doi: 10.3389/fncel.2018.00398
48. Hagar, JA, Powell, DA, Aachoui, Y, Ernst, RK, and Miao, EA. Cytoplasmic LPS activates caspase-11: implications in TLR4-independent endotoxic shock. Science. (2013) 341:1250–3. doi: 10.1126/science.1240988
49. Peng, L, Li, Z-R, Green, RS, Holzman, IR, and Lin, J. Butyrate enhances the intestinal barrier by facilitating tight junction assembly via activation of AMP-activated protein kinase in CACO-2 cell monolayers. J Nutr. (2009) 139:1619–25. doi: 10.3945/jn.109.104638
50. Qiao, C-M, Sun, M-F, Jia, X-B, Li, Y, Zhang, B-P, Zhao, L-P, et al. Sodium butyrate exacerbates Parkinson's disease by aggravating neuroinflammation and colonic inflammation in MPTP-induced mice model. Neurochem Res. (2020) 45:2128–42. doi: 10.1007/s11064-020-03074-3
51. Camargo, SMR, Vuille-Dit-Bille, RN, Mariotta, L, Ramadan, T, Huggel, K, Singer, D, et al. The molecular mechanism of intestinal levodopa absorption and its possible implications for the treatment of Parkinson's disease. J Pharmacol Exp Ther. (2014) 351:114–23. doi: 10.1124/jpet.114.216317
52. Uchino, H, Kanai, Y, Kim, DK, Wempe, MF, Chairoungdua, A, Morimoto, E, et al. Transport of amino acid-related compounds mediated by L-type amino acid transporter 1 (LAT1): insights into the mechanisms of substrate recognition. Mol Pharmacol. (2002) 61:729–37. doi: 10.1124/mol.61.4.729
53. Nutt, JG. Pharmacokinetics and pharmacodynamics of levodopa. Mov Disord. (2008) 23:S580–4. doi: 10.1002/mds.22037
54. Leta, V, Jenner, P, Chaudhuri, KR, and Antonini, A. Can therapeutic strategies prevent and manage dyskinesia in Parkinson's disease? an update. Expert Opin Drug Saf. (2019) 18:1203–18. doi: 10.1080/14740338.2019.1681966
55. Sandler, M, Goodwin, BL, and Ruthven, CR. Therapeutic implications in parkinsonism of M-tyramine formation from L-dopa in man. Nature. (1971) 229:414–6. doi: 10.1038/229414a0
56. Pierantozzi, M, Pietroiusti, A, Sancesario, G, Lunardi, G, Fedele, E, Giacomini, P, et al. Reduced L-dopa absorption and increased clinical fluctuations in Helicobacter pylori-infected Parkinson's disease patients. Neurol Sci. (2001) 22:89–91. doi: 10.1007/s100720170061
57. Lyte, M. Microbial endocrinology as a basis for improved L-dopa bioavailability in Parkinson's patients treated for Helicobacter pylori. Med Hypotheses. (2010) 74:895–7. doi: 10.1016/j.mehy.2009.11.001
58. Reynolds, DJ, and Penn, CW. Characteristics of Helicobacter pylori growth in a defined medium and determination of its amino acid requirements. Microbiology. (1994) 140:2649–56. doi: 10.1099/00221287-140-10-2649
59. Li, X, Feng, X, Jiang, Z, and Jiang, Z. Association of small intestinal bacterial overgrowth with Parkinson's disease: a systematic review and Meta-analysis. Gut Pathog. (2021) 13:25. doi: 10.1186/s13099-021-00420-w
60. Tan, AH, Mahadeva, S, Thalha, AM, Gibson, PR, Kiew, CK, Yeat, CM, et al. Small intestinal bacterial overgrowth in Parkinson's disease. Parkinsonism Relat Disord. (2014) 20:535–40. doi: 10.1016/j.parkreldis.2014.02.019
61. Kuai, X-Y, Yao, X-H, Xu, L-J, Zhou, Y-Q, Zhang, L-P, Liu, Y, et al. Evaluation of fecal microbiota transplantation in Parkinson's disease patients with constipation. Microb Cell Factories. (2021) 20:98. doi: 10.1186/s12934-021-01589-0
62. Fu, P, Gao, M, and Yung, KKL. Association of intestinal disorders with Parkinson's disease and Alzheimer's disease: a systematic review and meta-analysis. ACS Chem Neurosci. (2020) 11:395–405. doi: 10.1021/acschemneuro.9b00607
63. García-Domínguez, I, Veselá, K, García-Revilla, J, Carrillo-Jiménez, A, Roca-Ceballos, MA, Santiago, M, et al. Peripheral inflammation enhances microglia response and Nigral dopaminergic cell death in an in vivo MPTP model of Parkinson's disease. Front Cell Neurosci. (2018) 12:398. doi: 10.3389/fncel.2018.00398
64. Lomax, AE, Linden, DR, Mawe, GM, and Sharkey, KA. Effects of gastrointestinal inflammation on Enteroendocrine cells and enteric neural reflex circuits. Auton Neurosci. (2006) 126–127:250–7. doi: 10.1016/j.autneu.2006.02.015
65. Spencer, RP. Intestinal absorption of amino acids. current concepts. Am J Clin Nutr. (1969) 22:292–9. doi: 10.1093/ajcn/22.3.292
66. Lewitt, PA, Giladi, N, and Navon, N. Pharmacokinetics and efficacy of a novel formulation of carbidopa-levodopa (Accordion Pill®) in Parkinson's disease. Parkinsonism Relat Disord. (2019) 65:131–8. doi: 10.1016/j.parkreldis.2019.05.032
67. Freire-Alvarez, E, Kurča, E, Lopez Manzanares, L, Pekkonen, E, Spanaki, C, Vanni, P, et al. Levodopa-carbidopa intestinal gel reduces dyskinesia in Parkinson's disease in a randomized trial. Mov Disord. (2021) 36:2615–23. doi: 10.1002/mds.28703
68. Poewe, W, Stocchi, F, Arkadir, D, Ebersbach, G, Ellenbogen, AL, Giladi, N, et al. Subcutaneous levodopa infusion for Parkinson's disease: 1-year data from the open-label beyond study. Mov Disord. (2021) 36:2687–92. doi: 10.1002/mds.28758
69. Lewitt, PA, Hauser, RA, Pahwa, R, Isaacson, SH, Fernandez, HH, Lew, M, et al. Safety and efficacy of CVT-301 (levodopa inhalation powder) on motor function during off periods in patients with Parkinson's disease: a randomised, double-blind, placebo-controlled phase 3 trial. Lancet Neurol. (2019) 18:145–54. doi: 10.1016/S1474-4422(18)30405-8
70. Müller, T, and Möhr, JD. Efficacy of carbidopa-levodopa extended-release capsules (IPX066) in the treatment of Parkinson disease. Expert Opin Pharmacother. (2018) 19:2063–71. doi: 10.1080/14656566.2018.1538355
71. Nyholm, D, and Jost, WH. Levodopa-entacapone-carbidopa intestinal gel infusion in advanced Parkinson's disease: real-world experience and practical guidance. Ther Adv Neurol Disord. (2022) 15:17562864221108018. doi: 10.1177/17562864221108018
72. Rosebraugh, M, Voight, EA, Moussa, EM, Jameel, F, Lou, X, Zhang, GGZ, et al. Foslevodopa/foscarbidopa: a new subcutaneous treatment for Parkinson's disease. Ann Neurol. (2021) 90:52–61. doi: 10.1002/ana.26073
73. Barichella, M, Pacchetti, C, Bolliri, C, Cassani, E, Iorio, L, Pusani, C, et al. Probiotics and prebiotic fiber for constipation associated with Parkinson disease: an RCT. Neurology. (2016) 87:1274–80. doi: 10.1212/WNL.0000000000003127
74. Mao, XY, Yin, XX, Guan, QW, Xia, QX, Yang, N, Zhou, HH, et al. Dietary nutrition for neurological disease therapy: current status and future directions. Pharmacol Ther. (2021) 226:107861. doi: 10.1016/j.pharmthera.2021.107861
75. Tan, AH, Lim, SY, Chong, KK, Maa, AM, Hor, JW, Lim, JL, et al. Probiotics for constipation in Parkinson disease: a randomized placebo-controlled study. Neurology. (2021) 96:E772–82. doi: 10.1212/WNL.0000000000010998
76. Avallone, R, Vitale, G, and Bertolotti, M. Omega-3 fatty acids and neurodegenerative diseases: new evidence in clinical trials. Int J Mol Sci. (2019) 20:4256. doi: 10.3390/ijms20174256
77. Hernando, S, Requejo, C, Herran, E, Ruiz-Ortega, JA, Morera-Herreras, T, Lafuente, JV, et al. Beneficial effects of n-3 polyunsaturated fatty acids administration in a partial lesion model of Parkinson's disease: the role of glia and NRf2 regulation. Neurobiol Dis. (2019) 121:252–62. doi: 10.1016/j.nbd.2018.10.001
78. He, C, Peng, C, Xu, X, Li, N, Ouyang, Y, Zhu, Y, et al. Probiotics mitigate Helicobacter pylori-induced gastric inflammation and premalignant lesions in INS-GAS mice with the modulation of gastrointestinal microbiota. Helicobacter. (2022) 27:E12898. doi: 10.1111/hel.12898
79. Leta, V, Ray Chaudhuri, K, Milner, O, Chung-Faye, G, Metta, V, Pariante, CM, et al. Neurogenic and anti-inflammatory effects of probiotics in Parkinson's disease: a systematic review of preclinical and clinical evidence. Brain Behav Immun. (2021) 98:59–73. doi: 10.1016/j.bbi.2021.07.026
80. Zhong, Z, Chen, W, Gao, H, Che, N, Xu, M, Yang, L, et al. Fecal microbiota transplantation exerts a protective role in MPTP-induced Parkinson's disease via the TLR4/PI3K/AKT/NF-ΚB pathway stimulated by α-synuclein. Neurochem Res. (2021) 46:3050–8. doi: 10.1007/s11064-021-03411-0
81. Gatta, L, and Scarpignato, C. Systematic review with meta-analysis: rifaximin is effective and safe for the treatment of small intestine bacterial overgrowth. Aliment Pharmacol Ther. (2017) 45:604–16. doi: 10.1111/apt.13928
82. Liang, Y, Zheng, D, Peng, S, Lin, D, Jing, X, Zeng, Z, et al. Rifampicin attenuates rotenone-treated microglia inflammation via improving lysosomal function. Toxicol In Vitro. (2020) 63:104690. doi: 10.1016/j.tiv.2019.104690
83. Yurtsever, İ, Üstündağ, ÜV, Ünal, İ, Ateş, PS, and Emekli-Alturfan, E. Rifampicin decreases neuroinflammation to maintain mitochondrial function and calcium homeostasis in rotenone-treated zebrafish. Drug Chem Toxicol. (2022) 45:1544–51. doi: 10.1080/01480545.2020.1846549
84. Cazalis, J, Tanabe, S-I, Gagnon, G, Sorsa, T, and Grenier, D. Tetracyclines and chemically modified tetracycline-3 (CMT-3) modulate cytokine secretion by lipopolysaccharide-stimulated whole blood. Inflammation. (2009) 32:130–7. doi: 10.1007/s10753-009-9111-9
85. Garrido-Mesa, J, Rodríguez-Nogales, A, Algieri, F, Vezza, T, Hidalgo-Garcia, L, Garrido-Barros, M, et al. Immunomodulatory tetracyclines shape the intestinal inflammatory response inducing mucosal healing and resolution. Br J Pharmacol. (2018) 175:4353–70. doi: 10.1111/bph.14494
Keywords: Parkinson’s disease, levodopa, treatment, absorb, gut bacteria
Citation: Zhong Z, Ye M and Yan F (2023) A review of studies on gut microbiota and levodopa metabolism. Front. Neurol. 14:1046910. doi: 10.3389/fneur.2023.1046910
Received: 17 September 2022; Accepted: 02 May 2023;
Published: 02 June 2023.
Edited by:
Jifeng Guo, Central South University, ChinaReviewed by:
Xiaobo Li, Shanghai Jiao Tong University, ChinaCopyright © 2023 Zhong, Ye and Yan. This is an open-access article distributed under the terms of the Creative Commons Attribution License (CC BY). The use, distribution or reproduction in other forums is permitted, provided the original author(s) and the copyright owner(s) are credited and that the original publication in this journal is cited, in accordance with accepted academic practice. No use, distribution or reproduction is permitted which does not comply with these terms.
*Correspondence: Fuling Yan, bTE1MTUwNjU1NDQzQDE2My5jb20=; Min Ye, eWVtaW4xOTcwQDE2My5jb20=
Disclaimer: All claims expressed in this article are solely those of the authors and do not necessarily represent those of their affiliated organizations, or those of the publisher, the editors and the reviewers. Any product that may be evaluated in this article or claim that may be made by its manufacturer is not guaranteed or endorsed by the publisher.
Research integrity at Frontiers
Learn more about the work of our research integrity team to safeguard the quality of each article we publish.