- 1Department of Otolaryngology-Head and Neck Surgery, Xijing Hospital, Air Force Military Medical University, Xi'an, China
- 2Division of Mechanics and Acoustics, National Institute of Metrology, Beijing, China
Purpose: Aging is a process associated with degeneration and dysfunction of peripheral vestibular system or apparatus. This study aimed to investigate the influence of aging on ocular vestibular-evoked myogenic potential (oVEMP) response rates and recording parameters using the B81 bone vibrator and compare them with air conduction stimuli (ACS) oVEMP response characteristics.
Methods: In 60 healthy participants aged 10–71 years (mean age 39.9; 29 male participants), the oVEMP response was elicited using a B81 bone vibrator and an ER-3A insert earphone. The effects of age and stimulus on oVEMP response rates and recording parameters were evaluated.
Results: Response rates and amplitudes declined with aging using either ACS or bone-conducted vibration (BCV) stimulation, particularly in individuals over 60 years of age, whereas thresholds increased and N1 latencies were prolonged. BCV showed fewer risks of absent oVEMP response than ACS (p = 0.002). BCV acquired higher amplitudes (p < 0.001), lower thresholds, and shorter N1 and P1 latencies (all p < 0.001) than ACS.
Conclusions: The absence of an oVEMP response may be attributed to aging rather than a concurrent vestibular disorder. B81-BCV likely produces higher mechanical drives to the vestibular hair cells at safer and non-traumatic levels compared with ACS and therefore may be more likely to evoke a response in the elderly cohort, whose vestibular function and mechanical sensitivity have declined. Thus, B81-BCV stimulation is more effective and safer to elicit oVEMPs, and it should be recommended when ACS fails in the clinic, particularly in the elderly population.
Introduction
Aging causes continuous deterioration of human body, including the vestibular system (1, 2). Although the effects of aging on the functioning of the semicircular canals and saccule have been extensively investigated (3, 4), its impact on the utricle remains the least studied component.
Ocular vestibular-evoked myogenic potential (oVEMP), an excitatory electromyographic (EMG) response measured over the inferior oblique muscle that assesses contralateral utricular macula and superior vestibular nerve function, is increasingly being used in clinical practice (5, 6). The most commonly used clinical parameters of the oVEMP include response latency, amplitude, threshold, interaural amplitude asymmetry (IADR), and asymmetry ratio (AR). The oVEMP is reported to be helpful in the diagnosis of various vestibular disorders such as vestibular neuritis, Meniere's disease, and benign paroxysmal positional vertigo (7–11). Moreover, it is particularly significant in diagnosing third window disorder of the inner ear when combined with cervical vestibular-evoked myogenic potential which probes saccular macular function (12–14).
Ocular vestibular-evoked myogenic potentials can be elicited by a variety of stimuli, including air conduction stimuli (ACS) and bone-conducted vibration (BCV) stimuli, such as transient onset tone bursts or pulses, as well as galvanic stimulation (GVS) (5, 6, 15). However, when employing ACS oVEMP, the response rates and amplitudes decrease with aging and frequency tuning switches to higher frequencies (16–18), likely a feature of changes in morphology and mechanics, such as increased stiffness of the macula and otoconial layer. It was discovered that the absence of oVEMP responses was six times higher for those in their 40s, 50s, and 60s, and 13 times higher for people in their 70s than for those in their 20s (18). As a result, it is difficult to determine whether a missing ACS oVEMP response is related to vestibular disorders or whether ACS is an inefficient stimulus in the elderly population. Furthermore, in patients with middle ear disorder or pressure equalization tube dysfunction, ACS oVEMP may result in declined or absent responses. An air-bone gap of 9 dB nHL significantly reduces ACS VEMP responses (19), and an air-bone gap of 20 dB nHL completely abolishes ACS VEMP responses (20). Moreover, lower response rates for ACS oVEMP may be present in the ears following cochlear implantation due to air-bone gaps (21).
Bone-conducted vibration may be an alternative method to elicit oVEMPs, although the response rates of BCV oVEMPs can also be influenced by aging (15, 22). It has been reported that BCV may bypass the middle ear and be more effective in stimulating utricular neurons than ACS (23). This has been shown in mammalian models in vivo, where disrupting the ossicular chain and/or the conductive fluid layer between the stapes footplate and utricular macula abolished ACS utricular microphonics and vestibular short-latency-evoked potentials, but did not effect BCV-evoked responses (24). Furthermore, the use of a reflex hammer and lateral pulses (mini-shaker) in the interaural plane may be less affected by age assuming the receptor hair cells, and afferent neurons are functional (15). Other studies have demonstrated that bone-oscillating devices (Radioear B71) can elicit oVEMPs with higher response rates and amplitudes (15). Whereas BCV may be safer and provide more effective otolith organ stimulation than ACS, the B71 has been reported to be less dependable than the use of mini-shaker and impulse hammer in adults (25, 26). The Radioear B81 was recently designed to have a higher output and less distortion than traditional Radioear B71 (27). However, studies of normative data on the B81-BCV oVEMP responses are few, particularly in elderly Asians. Therefore, this study aimed to investigate the influence of aging on oVEMP response rates and recording parameters using the B81 bone vibrator and compare them with ACS oVEMP response characteristics. We hypothesized that aging might result in the decline of ACS and BCV induced oVEMP responses in healthy participants. Moreover, the response rates and amplitudes of the oVEMP would be higher for B81-BCV than for ACS, particularly in elderly individuals.
Materials and methods
Participants
A total of 60 healthy participants ranging from 10 to 71 years old (mean age 39.9 years; 29 males) were sorted into six age groups based on the decades of life. Individuals with a history of known conductive hearing loss (CHL), dizziness, balance symptoms, high blood pressure, diabetes, and neurologic illnesses were excluded. All participants underwent pure-tone audiometry and immittance assessment before oVEMP testing to rule out a conductive pathology. Those showing “A” type tympanogram were included in this study. The participant's hearing was considered normal if the pure-tone average (PTA) was ≤20 dB nHL. Participants were excluded if they had a CHL, defined as an air-bone gap >10 dB nHL at two consecutive frequencies. Symmetrical sensorineural hearing loss (SNHL), PTA ≤35 dB nHL, was permissible in participants aged >60 years. Participants with asymmetric oVEMP responses, with an IADR >0.33, were excluded for possible unilateral vestibular system impairment (18). This study was approved by the Medical Ethics Committee of the First Affiliated Hospital of the Air Force Medical University (No. KY20222045-C-1), and the informed consent was obtained from each participant.
oVEMP testing
The oVEMP responses were acquired using the Interacoustics Eclipse EP1/25 System (Interacoustics, Middelfart, DK) in a conventional sound isolation room. Participants were instructed to lie down comfortably on an examination bed and avoid any extraneous movements of the body, limb, or neck. The electrode configuration included the placement of the active electrode approximately 1 cm below the lower eye-lid closest to the inferior oblique when staring up, the reference electrodes cross-posted 2 cm below the active electrode, and the ground electrode on the forehead, as found to be optimal and used previously (4, 28). Before electrode installation, the skin overlying the electrode locations was cleaned with a commercially available abrasive skin preparation gel to reduce electrode impedance. Using commercially available conductive paste, gold-plated cup-shaped electrodes were inserted at these spots and fastened with adhesive tape. This was important to ensure that altered oVEMP amplitudes were not due to the factors related to the electrode. The absolute and interelectrode impedances were maintained below 5 and 2 kΩ, respectively. The parameter settings of oVEMP are shown in Table 1, as we previously depicted (29).
Air conduction stimuli and BCV oVEMP testing were performed using an ER-3A insert earphone and B81 bone vibrator (Radioear, USA), respectively. A B81 bone vibrator was placed on the mastoid using a standard bone conduction headband. Participants were asked to focus their gaze upward by 30° on a target attached to the wall during the recording. The stimulus intensity used by tone bursts of 500 Hz was 100 dB nHL for ACS and 70 dB nHL for B81-BCV. The outcomes of the oVEMPs were recorded from the contralateral ocular muscles during the initial stimulus intensity. After eliciting a typical waveform, the stimulus intensity was reduced in 5-dB decrements until no response was elicited. The threshold was the minimum stimulus intensity required to elicit reproducible oVEMP responses. We measured the output intensity of the ER-3A insert earphone and the B81 using a common calibration system, as previously reported (29, 30). For bone-conducted force calibration, an artificial mastoid (model 4930, Bruel & Kjaer, DK) was used as a transducer. The analysis was carried out using a data acquisition system (model 3160, Bruel & Kjaer, DK) and PULSE software package (version 20, Bruel & Kjaer, DK). For ACS, the measured peak-to-peak equivalent sound pressure level (peSPL) is referenced to 20 μPa of the air-conduction sound level, whereas for BCV, the measured peak-to-peak vibratory force level (peVFL) is referenced to 1 μN of the vibratory force level. The human mastoid is not as simple as an artificial mastoid concerning mechanical point impedance. Therefore, the position of the vibrator will be adjusted repeatedly to obtain the best response, considering input force and response sensitivity.
The typical oVEMP is a biphasic waveform. The first wavepeak is the N1 with a latency of approximately 10 ms, followed by the P1 at ~15 ms. Figure 1 depicts a healthy participant's representative oVEMP tracings for ACS and BCV stimuli. If the oVEMP N1-P1 differed in latency from the typical 10–15 ms, then it was only acceptable if it deviated by several milliseconds, between 8 and 22 ms. Outcome measures for oVEMP testing included the N1 and P1 latencies, N1–P1 amplitudes, thresholds, IADR, and AR. The IADR and AR between a subject's ears were calculated using the following formula (31):
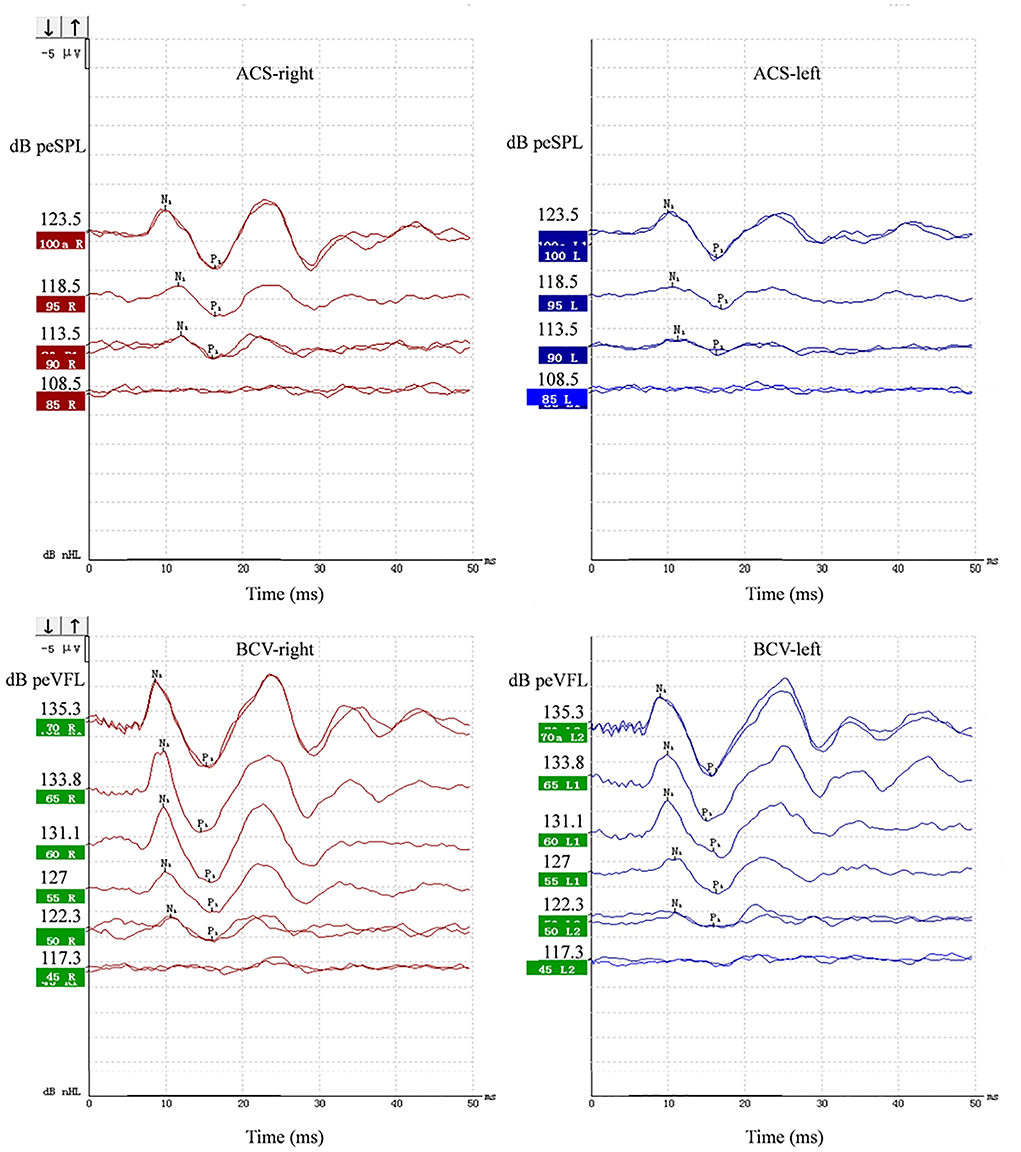
Figure 1. Representative ACS and BCV oVEMPs from a 19-year-old female. Primitive test value of tone burst parameter and corresponding sound pressure level or force level value were all marked on the Y-axis of each wave. The unit was peak-to-peak equivalent sound pressure level (peSPL) when using ACS and peak-to-peak force equivalent vibratory force level (peVFL) when using BCV.
Statistical analysis
Pearson's chi-square test and Fisher's exact test were used to compare the response rates of oVEMPs between the stimulus and age groups. Logistic regression analysis was performed to determine the effects of age (continuous), sex, side, and stimulus on absent oVEMP responses. Spearman's correlation analysis was used to show the relationship between age and recording parameters of the oVEMP. A linear mixed-effects model was employed to model the recording parameters of oVEMP, and the model was adjusted for sex, age (continuous), stimulus, and side. All statistical analyses were performed using SPSS v.24.0 (SPSS, Inc., Chicago, IL, USA). Differences were considered statistically significant if the p-value was <0.05.
Results
Effects of aging and stimulus on response rates of oVEMPs
The demographics and response rates of oVEMPs for each stimulus across different age groups are shown in Table 2. The oVEMPs were present in both ears of all participants up to the age of 40 for ACS and 50 for BCV. There was no statistically significant difference in response rates between the ACS and BCV groups in the age groups of 40–49 years (Fisher's exact test, p = 0.106), 50–59 years (Fisher's exact test, p = 0.235), and ≥60 years (χ2 = 3.64, p = 0.057). However, the BCV group showed significantly higher response rates than the ACS group (χ2 = 6.72, p = 0.010), considering all patients (Figure 2A).
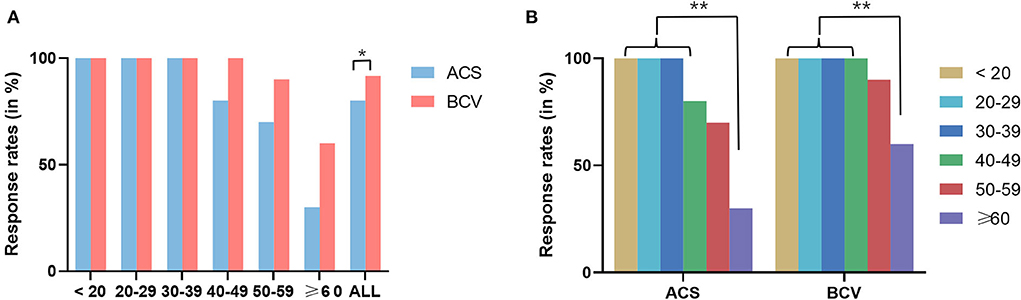
Figure 2. (A) Comparison of response rates between ACS and BCV oVEMPs in different age groups using Pearson's chi-square test and Fisher's exact test. (B) Comparison of response rates between different groups of age in ACS and BCV using Fisher's exact test with Bonferroni adjusted multiple comparisons for pair-wise comparisons. Star-marked comparisons are statistically significant at *p < 0.05 and **p < 0.01.
In both the ACS and BCV groups, Fisher's exact test with Bonferroni-adjusted multiple comparisons for pair-wise comparisons across different age groups found no significant difference in response rates between the age groups up to 60 years, as shown in Figure 2B. However, the age groups up to 50 years showed significantly higher response rates than those older than 60 years for both ACS and BCV (p < 0.001). The age group of 50–60 years showed no statistically significant difference in response rates compared with the age groups beyond 60 years for both ACS and BCV.
The logistic regression model with the enter method was statistically significant in determining the effects of age (continuous), sex, side, and stimulus on an absent oVEMP response (χ2 = 28.63, p < 0.001). As shown in Table 3, the model was adjusted for sex, age (continuous), and stimulus. Men had a greater risk of absent oVEMP responses than women (OR = 2.607). BCV showed fewer risks of absent oVEMP response than ACS (OR = 0.210). Furthermore, the risk of the absence of an oVEMP response increased with age (OR = 1.144).
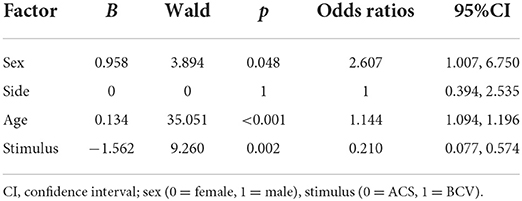
Table 3. Logistic regression analysis to evaluate factors with odds ratios (95% confidence interval) for absent oVEMP responses.
Effects of aging and stimulus on recording parameters of OVEMPs
The recording parameters of the oVEMP for each stimulus across different age groups are shown in Table 4. Figure 3 shows the linear regression curves depicting the relationship between age and the recording parameters. The results revealed a significantly negative correlation between age and amplitude for both ACS (R2 = 0.06, p = 0.02) and BCV (R2 = 0.23, p < 0.001) (Figure 3A). The correlation between age and thresholds was significantly positive for BCV (R2 = 0.20, p < 0.001), whereas not for ACS (Figure 3B). A significant positive correlation was found between age and N1 latencies for BCV (R2 = 0.07, p = 0.006), with no correlation for ACS (Figure 3C). Age showed no significant correlation with P1 latencies (Figure 3D), IADR, or AR for both ACS and BCV.
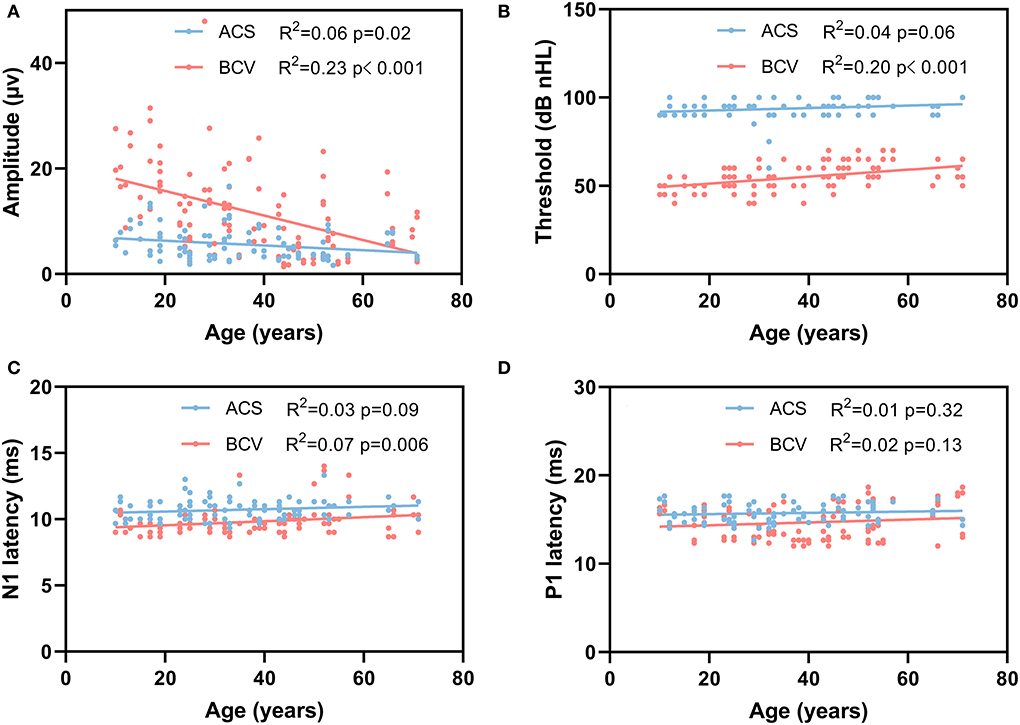
Figure 3. Simple linear regression curves showed the relationship between age and oVEMP recording parameters. (A) A significantly negative correlation between age and amplitude for ACS (p = 0.02) and BCV (p < 0.001). (B) A significantly positive correlation between age and thresholds for BCV (p < 0.001). (C) A significant positive correlation was found between age and N1 latency for BCV (p = 0.006). (D) There was no correlation between the age and P1 latency for ACS and BCV.
Table 5 shows the results of multiple linear regression analysis using a stepwise method. The model was adjusted to evaluate the effects of sex, age (continuous), stimulus, and side on the oVEMP recording parameters. The results indicated that age was positively correlated with thresholds (p < 0.001) and negatively correlated with amplitudes (p < 0.001) and N1 latencies (p = 0.001). BCV oVEMP showed higher amplitudes, lower thresholds, and shorter N1 and P1 latencies (all p < 0.001). Furthermore, sex, age (continuous), and stimulus methods were not significantly correlated with IADR and AR. Interesting, BCV oVEMP amplitudes were always larger compared with ACS stimulation, even after they have declined by >50% in the age cohort of 20–60 (refer to Table 4).
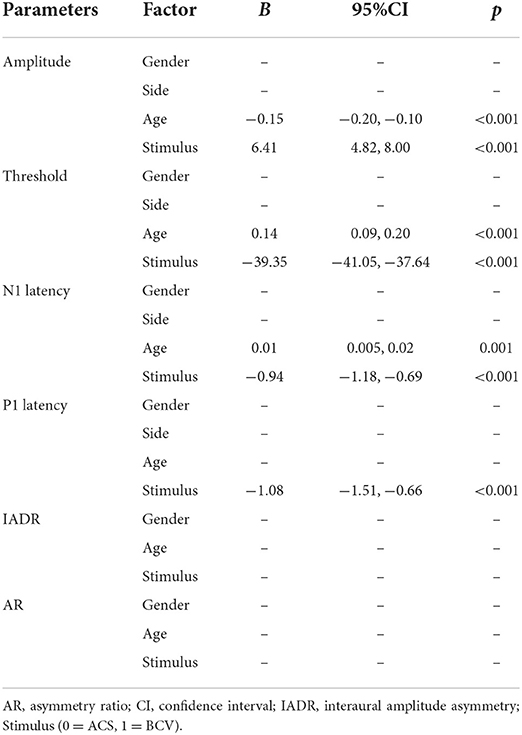
Table 5. Evaluating the effects of gender, age (continuous), stimulus, and side on oVEMP response parameters using multiple linear regression analysis.
Discussion
We explored the effects of aging on the oVEMP response rate and parameters using the B81 bone vibrator and compared them to the ACS oVEMP response characteristics in this study. Similar to ACS oVEMPs, we discovered that the response rates and amplitudes of the B81-BCV oVEMPs declined with aging, particularly in individuals over 60 years of age, whereas the thresholds and N1 latencies increased. Furthermore, B81-BCV showed higher oVEMP response rates, greater amplitudes, lower thresholds, and shorter N1 latencies than ACS did. As a result, B81-BCV would be less susceptible to aging than ACS, which is consistent with our hypothesis. Owing to better response rates and lower thresholds, B81-BCV might be more effective and safer than ACS in eliciting oVEMPs.
Effects of aging on oVEMPs response
Table 6 summarizes many studies conducted to investigate the impact of aging on oVEMPs. Owing to a lack of accessible information, we did not assess non-English publications in this area. Despite the variances in stimulators, most investigations have revealed a decrease in oVEMP response rates and amplitudes with age. These results are consistent with the discovery of age-related degenerative alterations from the end organs of the vestibular system to its central nuclei (37). Previous studies have shown that the mass of the utricular macula decreases and the stiffness of the utricular membrane increases as a result of degeneration associated with aging (38, 39), which therefore requires higher ACS or BCV drive to activate the vestibular hair cells. These degenerative alterations would have resulted in a decrease in amplitudes for the already small-amplitude oVEMP curves in certain people, resulting in merging of the response in the EMG noise. As a result, these responses would have been unidentified, resulting in lower oVEMP response rates in the elderly (4). This study appears to be consistent with earlier findings (4, 15, 18, 22, 31–36). However, those studies showed different onset age of reductions in oVEMP response rates and amplitudes. These differences may be attributed to the stimulator, sample size, and race of the participants.
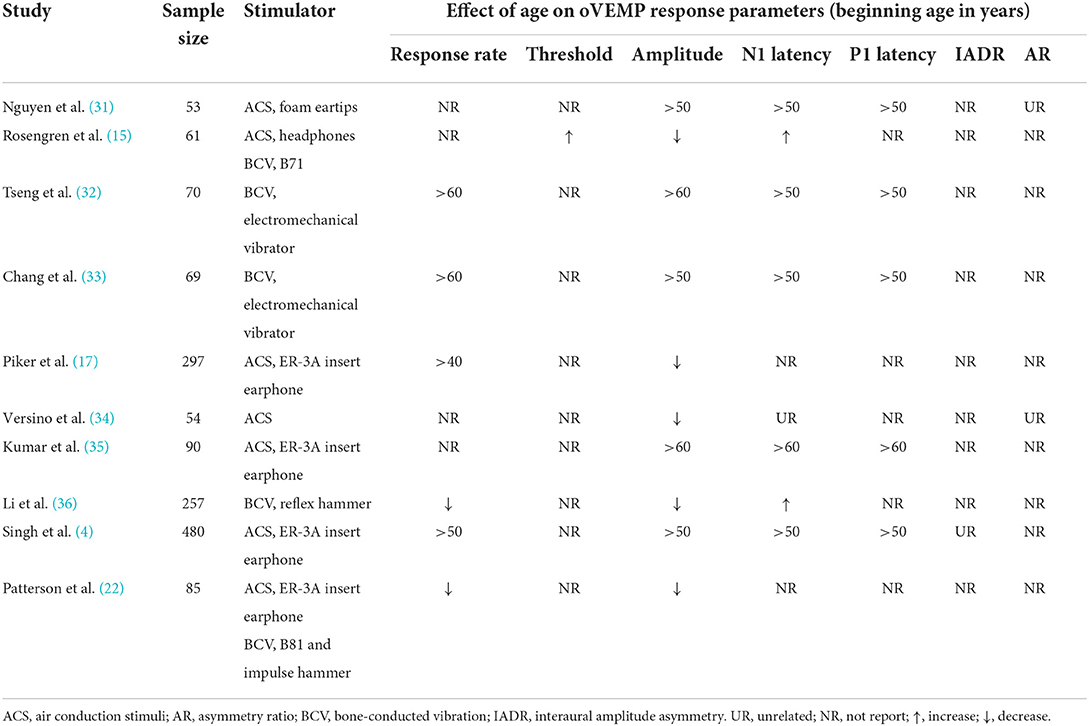
Table 6. Literature review of the effects of age on oVEMP response rates and response parameters using ACS and BCV.
The largest sample study of aging on oVEMP response reported ACS oVEMP response rates of 41.25% in 80 participants aged >60 years, which is somewhat consistent with our study (4). Few studies have reported the effect of aging on the B81-BCV oVEMP response rates and response parameters (Table 6). Surprisingly, Patterson et al. reported oVEMP response rates of 92% for ACS and 83% for the B81 in the age of 60–69, which were greater than our response rates of 30 and 60%, respectively (22). This may have been due to the lower stimulation intensity of B81 with 70 dB nHL (135.3 dB peVFL) in our study, whereas Patterson et al. increased the stimulation intensity to 75dB nHL (138 dB peVFL) when oVEMPs could not be elicited with 70 dB nHL (136 dB peVFL) stimulation (22). In addition, BC sound transmission and its response potentials are affected by stimulation position as well as conditions and manipulations of the head (40). Another surprising finding was the effect of sex on response rates, wherein males demonstrated a greater risk of oVEMP response absence (OR = 2.607). To the best of our knowledge, no reports have indicated that sex influences oVEMP response rates. This sex difference may be due to the limitation of the sample size. The sample size was likely not representative of the general population for each age category, which needs to be expanded in further research.
Meanwhile, we discovered that older people required a more intense stimulus to elicit an oVEMP response, with longer N1 latencies. This is in line with the findings from earlier oVEMP research (15), which may also be explained by vestibular system degeneration (37). Accepting responses with peak latency a few milliseconds beyond the normal range, however, should be done with caution. Late peaks can also be caused by failure to manage the gaze angle (41) or insufficient stimulus intensity. Another finding in our study was that age did not affect IADR and AR, similar to the results of previous studies (4, 31). This may due to that aging is a symmetrical process that equally affects both sides of any two-sided system (42).
ACS and B81-BCV
Our study discovered that the response rates of the B81-BCV oVEMPs were significantly higher than those of the ACS oVEMPs, especially in the elderly population >60 years. Here, the results reveal a 30% greater oVEMP response rate evoked by BCV compared to ACS. This may due to the potential differences in stimulation modes of peripheral receptor activation by ACS and BCV. Previous research has indicated that the saccule is more specific to ACS, whereas BCV stimulates both the saccule and utricle equally (43, 44). Pastras et al. (24) reported that during ACS stimulation, the fluid pressure wave coupling the stapes motion to the utricle primarily produces a transverse motion of the utricular macula, whereas BCV most likely induces a more complex motion of the utricular macula, where lateral motion of the macula may be the dominant drive activating the hair cells. Interestingly, for the same level of macular velocity, the utricular microphonic response amplitude was ~4 times larger during BCV compared to ACS, demonstrating the differences in sensitivity and micromechanical activation modes of utricular stereocilia between BCV and ACS in the mammalian labyrinth (24). These differences likely result in BCV producing higher mechanical input drives to the hair cells than ACS. These differences also likely exist in the human labyrinth, where BCV may be more effective in evoking oVEMPs in the clinic compared to ACS, for a similar level of macular stimulation. Moreover, B81-BCV is a bilateral stimulation of the utricle, with probably concurrent enhancement of the vertical component eye movement, interpreting the higher oVEMP response rates and amplitudes to BCV seen in this study (45).
Furthermore, the BCV oVEMP amplitudes are reduced by >50% from ages 20 to 60, whereas the ACS oVEMP amplitudes remain relatively low (Figure 3A), highlighting that BCV oVEMP amplitude is indeed affected by aging. Moreover, BCV oVEMP thresholds and N1 latency also slightly increase with aging more than ACS. The underlying mechanisms producing these differences are speculated that the mass of the utricular macula reduces and the stiffness of the utricular membrane increases with aging (38, 39). Additionally, the potential differences in stimulation modes of peripheral receptor activation by ACS and BCV likely also play an important role. Hence, the higher response rate for BCV in the elderly cohort is likely due to higher relative amplitudes of the BCV oVEMPs (even in the elderly, which is apparent in Figure 3A) and is likely also influenced by the limitation of using intense acoustic sounds to evoke ACS oVEMPs, which would damage the macular receptors.
Overall, there are some advantages of using BCV over ACS to elicit oVEMPs. First, in this study, the thresholds of BCV were significantly lower than ACS. It is of great significance for BCV to reduce the risk of damage from noise-related hearing loss due to lower thresholds. The high-intensity stimuli required for ACS VEMPs increase the risk of damage from sound pressure exposure in narrower ear canals, with ~3 dB nHL higher SPL in smaller ears (46, 47). Therefore, BCV may be employed instead of ACS to minimize the harm caused by loud stimuli. Second, for patients with CHL, such as middle ear or eustachian tube dysfunction, ACS may be an insufficient stimulus to stimulate the utricle in some individuals owing to attenuation during conduction, resulting in absent or diminished oVEMP response rates and amplitudes. BCV can directly stimulate the utricle and bypass the middle ear, avoiding attenuation of stimulation. Thus, compared to ACS, BCV offers a wider variety of applications. Finally, Merchant et al. reported that mechanical changes result in stiffening of the auditory system in patients with cochlear implantation (21). The response rates of BCV oVEMP were higher than that of ACS in these cochlear implant users, indicating that missing ACS responses were likely due to mechanical alterations rather than concurrent vestibular disorders.
The limitation of B81-BCV
B81-BCV may be a more effective and safer stimulus than ACS in eliciting oVEMPs, especially in pediatric (probably with exudative otitis media) and elderly populations. Nevertheless, there are some limitations to B81-BCV. Declined or absent responses can be caused by small movements and variations in placement for the B81 (40, 48). Due to the limited force output of B71, forehead placement for B71 is not recommended for VEMPs (25). Although B81 has a higher output and less distortion than the B71, the B81 output is likely not strong enough for forehead placement (22, 48). Thus, we suggest mastoid placement for the B81. Variations in the mastoid anatomy and head movements may result in slight movement of the B81 bone vibrator. If the participant and examiner noticed movement during testing, the testing was repeated after adjusting the bone vibrator. However, it is not easy to accomplish the testing without any slight movements of B81 in people with large protruding mastoid processes. Reinforcing with tape may be helpful in reducing the movements required to accomplish testing. Moreover, the examiner should attempt to place it symmetrically on both sides to avoid position-related differences.
Bone-conducted vibration can result in complex harmonic distortions in the skull due to the distorted output from the vibrator/mini-shaker and/or the complex resonances in the cranium. It has been reported that the maximum output range with minimal to no harmonic distortion is between 120 and 128 dB peVFL (49), this will vary with subjects and stimulation parameters/type. Whatever, the stimulus intensity of the B81-BCV ranged from 117.3 dB peVFL to 135.3 dB peVFL in our study, which was outside the linear range. Besides, it has been reported that considering BCV-oVEMP is most sensitive to low-frequency stimulation, the energy at harmonic distortion products of 500 Hz may be unlikely to contribute to response generation (49).
Conclusion
Aging affects oVEMP response rates and amplitudes regardless of stimulus type. Since B81 bone vibrator is more likely to evoke a response with lower intensity acoustic signal, it is more effective and safer than ACS to elicit oVEMPs, especially in the elderly population.
Data availability statement
The raw data supporting the conclusions of this article will be made available by the authors, without undue reservation.
Ethics statement
The studies involving human participants were reviewed and approved by Medical Ethics Committee of the First Affiliated Hospital of the Air Force Medical University (No. KY20222045-C-1). Written informed consent to participate in this study was provided by the participants' legal guardian/next of kin.
Author contributions
YL and DZ designed the study. YL and ZX wrote the manuscript, performed the statistical analysis, and made graphs. BZ did the calibration of acoustic instrument. ZW, MQ, MW, XF, and CR did the data collection. All authors performed data interpretation and approved the manuscript.
Funding
This research was supported by the grants from the National Key research and development program of China (2020YFC2005200) part 3 (2020YFC2005203), the National Natural Science Foundation of China under numbers 82171161 and 82101214, and Xijing Boost-Advanced Discipline Construction Project (XJZT19MDT02).
Acknowledgments
The authors thank the Wiley Editing Services for editing the language and the participants in this study for stimulating cooperation.
Conflict of interest
The authors declare that the research was conducted in the absence of any commercial or financial relationships that could be construed as a potential conflict of interest.
Publisher's note
All claims expressed in this article are solely those of the authors and do not necessarily represent those of their affiliated organizations, or those of the publisher, the editors and the reviewers. Any product that may be evaluated in this article, or claim that may be made by its manufacturer, is not guaranteed or endorsed by the publisher.
References
1. Agrawal Y, Merfeld DM, Horak FB, Redfern MS, Manor B, Westlake KP, et al. Aging, vestibular function, and balance: proceedings of a national institute on aging/national institute on deafness and other communication disorders workshop. J Gerontol A Biol Sci Med Sci. (2020) 75:2471–80. doi: 10.1093/gerona/glaa097
2. Brosel S, Laub C, Averdam A, Bender A, Elstner M. Molecular aging of the mammalian vestibular system. Ageing Res Rev. (2016) 26:72–80. doi: 10.1016/j.arr.2015.12.007
3. Agrawal Y, Zuniga MG, Davalos-Bichara M, Schubert MC, Walston JD, Hughes J, et al. Decline in semicircular canal and otolith function with age. Otol Neurotol. (2012) 33:832–9. doi: 10.1097/MAO.0b013e3182545061
4. Singh NK, Firdose H. Characterizing the impact of advancing age on 500 hz tone-burst evoked ocular-vestibular evoked myogenic potentials. Eur Arch Otorhinolaryngol. (2021) 278:4259–68. doi: 10.1007/s00405-020-06542-2
5. Curthoys IS, Iwasaki S, Chihara Y, Ushio M, McGarvie LA, Burgess AM. The ocular vestibular-evoked myogenic potential to air-conducted sound; probable superior vestibular nerve origin. Clin Neurophysiol. (2011) 122:611–6. doi: 10.1016/j.clinph.2010.07.018
6. Curthoys IS. A critical review of the neurophysiological evidence underlying clinical vestibular testing using sound, vibration and galvanic stimuli. Clin Neurophysiol. (2010) 121:132–44. doi: 10.1016/j.clinph.2009.09.027
7. Singh NK, Barman A. Inter-frequency amplitude ratio of ovemp for differentiating Meniere's disease from Bppv: clinical validation using a double-blind approach. Int J Audiol. (2019) 58:21–8. doi: 10.1080/14992027.2018.1529440
8. Jerin C, Berman A, Krause E, Ertl-Wagner B, Gürkov R. Ocular vestibular evoked myogenic potential frequency tuning in certain Menière's disease. Hear Res. (2014) 310:54–9. doi: 10.1016/j.heares.2014.02.001
9. Singh NK, Barman A. Frequency-amplitude ratio of ocular vestibular-evoked myogenic potentials for detecting Meniere's disease: a preliminary investigation. Ear Hear. (2016) 37:365–73. doi: 10.1097/AUD.0000000000000263
10. Xu H, Liang FY, Chen L, Song XC, Tong MC, Thong JF, et al. Evaluation of the utricular and saccular function using oVEMPS and cVEMPs in BPPV patients. J Otolaryngol Head Neck Surg. (2016) 45:12. doi: 10.1186/s40463-016-0125-7
11. Murofushi T, Nakahara H, Yoshimura E. Assessment of the otolith-ocular reflex using ocular vestibular evoked myogenic potentials in patients with episodic lateral tilt sensation. Neurosci Lett. (2012) 515:103–6. doi: 10.1016/j.neulet.2012.02.084
12. Taylor RL, Magnussen JS, Kwok B, Young AS, Ihtijarevic B, Argaet EC, et al. Bone-conducted ovemp latency delays assist in the differential diagnosis of large air-conducted ovemp amplitudes. Front Neurol. (2020) 11:580184. doi: 10.3389/fneur.2020.580184
13. Govender S, Fernando T, Dennis DL, Welgampola MS, Colebatch JG. Properties of 500hz air- and bone-conducted vestibular evoked myogenic potentials (VEMPs) in superior canal dehiscence. Clin Neurophysiol. (2016) 127:2522–31. doi: 10.1016/j.clinph.2016.02.019
14. Tran ED, Swanson A, Sharon JD, Vaisbuch Y, Blevins NH, Fitzgerald MB, et al. Ocular vestibular-evoked myogenic potential amplitudes elicited at 4 Khz optimize detection of superior semicircular canal dehiscence. Front Neurol. (2020) 11:879. doi: 10.3389/fneur.2020.00879
15. Rosengren SM, Govender S, Colebatch JG. Ocular and cervical vestibular evoked myogenic potentials produced by air- and bone-conducted stimuli: comparative properties and effects of age. Clin Neurophysiol. (2011) 122:2282–9. doi: 10.1016/j.clinph.2011.04.001
16. Welgampola MS, Colebatch JG. Vestibulocollic reflexes: normal values and the effect of age. Clin Neurophysiol. (2001) 112:1971–9. doi: 10.1016/S1388-2457(01)00645-9
17. Piker EG, Jacobson GP, Burkard RF, McCaslin DL, Hood LJ. Effects of age on the tuning of the cVEMP and oVEMP. Ear Hear. (2013) 34:e65–73. doi: 10.1097/AUD.0b013e31828fc9f2
18. Piker EG, Baloh RW, Witsell DL, Garrison DB, Lee WT. Assessment of the clinical utility of cervical and ocular vestibular evoked myogenic potential testing in elderly patients. Otol Neurotol. (2015) 36:1238–44. doi: 10.1097/MAO.0000000000000793
19. Bath AP, Harris N, McEwan J, Yardley MP. Effect of conductive hearing loss on the vestibulo-collic reflex. Clin Otolaryngol Allied Sci. (1999) 24:181–3. doi: 10.1046/j.1365-2273.1999.00234.x
20. Halmagyi GM, Colebatch JG, Curthoys IS. New tests of vestibular function. Baillieres Clin Neurol. (1994) 3:485–500.
21. Merchant GR, Schulz KM, Patterson JN, Fitzpatrick D, Janky KL. Effect of cochlear implantation on vestibular evoked myogenic potentials and wideband acoustic immittance. Ear Hear. (2020) 41:1111–24. doi: 10.1097/AUD.0000000000000831
22. Patterson JN, Rodriguez AI, Gordon KR, Honaker JA, Janky KL. Age effects of bone conduction vibration vestibular-evoked myogenic potentials (VEMPs) using B81 and impulse hammer stimuli. Ear Hear. (2021) 42:1328–37. doi: 10.1097/AUD.0000000000001024
23. Wang SJ, Tseng CC, Young YH. Selective effects of head posture on ocular vestibular-evoked myogenic potential (oVEMP) by bone-conducted vibration. Clin Neurophysiol. (2014) 125:621–6. doi: 10.1016/j.clinph.2013.09.002
24. Pastras CJ, Curthoys IS, Brown DJ. Dynamic response to sound and vibration of the guinea pig utricular macula, measured in vivo using laser doppler vibrometry. Hear Res. (2018) 370:232–7. doi: 10.1016/j.heares.2018.08.005
25. Iwasaki S, McGarvie LA, Halmagyi GM, Burgess AM, Kim J, Colebatch JG, et al. Head taps evoke a crossed vestibulo-ocular reflex. Neurology. (2007) 68:1227–9. doi: 10.1212/01.wnl.0000259064.80564.21
26. Greenwalt NL, Patterson JN, Rodriguez AI, Fitzpatrick D, Gordon KR, Janky KL. Bone conduction vibration vestibular evoked myogenic potential (VEMP) testing: reliability in children, adolescents, and young adults. Ear Hear. (2020) 42:355–63. doi: 10.1097/AUD.0000000000000925
27. Jansson KJ, Håkansson B, Johannsen L, Tengstrand T. Electro-Acoustic performance of the new bone vibrator radioear b81: a comparison with the conventional radioear B71. Int J Audiol. (2015) 54:334–40. doi: 10.3109/14992027.2014.980521
28. Chiarovano E, Zamith F, Vidal PP, de Waele C. Ocular and cervical vemps: a study of 74 patients suffering from peripheral vestibular disorders. Clin Neurophysiol. (2011) 122:1650–9. doi: 10.1016/j.clinph.2011.01.006
29. Lin Y, Zhong B, Fan XQ, Wang MJ, Liu JW, Guo Q, et al. Frequency properties of bone-conducted vibration and sound-induced ocular evoked myogenic potential. Zhonghua Er Bi Yan Hou Tou Jing Wai Ke Za Zhi. (2020) 55:338–43. doi: 10.3760/cma.j.cn115330-20191021-00639
30. Chihara Y, Iwasaki S, Fujimoto C, Ushio M, Yamasoba T, Murofushi T. Frequency tuning properties of ocular vestibular evoked myogenic potentials. Neuroreport. (2009) 20:1491–5. doi: 10.1097/WNR.0b013e3283329b4a
31. Nguyen KD, Welgampola MS, Carey JP. Test-retest reliability and age-related characteristics of the ocular and cervical vestibular evoked myogenic potential tests. Otol Neurotol. (2010) 31:793–802. doi: 10.1097/MAO.0b013e3181e3d60e
32. Tseng CL, Chou CH, Young YH. Aging effect on the ocular vestibular-evoked myogenic potentials. Otol Neurotol. (2010) 31:959–63. doi: 10.1097/MAO.0b013e3181e8fb1a
33. Chang CM, Young YH, Cheng PW. Age-related changes in ocular vestibular-evoked myogenic potentials via galvanic vestibular stimulation and bone-conducted vibration modes. Acta Otolaryngol. (2012) 132:1295–300. doi: 10.3109/00016489.2012.708437
34. Versino M, Colnaghi S, Ranzani M, Alloni R, Bolis C, Sacco S, et al. Ocular vestibular evoked myogenic potentials in response to air-conducted 500 Hz short tones: effect of stimulation procedure (monaural or binaural), age and gender. J Vestib Res. (2015) 25:143–9. doi: 10.3233/VES-150554
35. Kumar K, Bhat JS, Sequeira NM, Bhojwani KM. Ageing Effect on air-conducted ocular vestibular evoked myogenic potential. Audiol Res. (2015) 5:121. doi: 10.4081/audiores.2015.121
36. Li C, Layman AJ, Carey JP, Agrawal Y. Epidemiology of vestibular evoked myogenic potentials: data from the baltimore longitudinal study of aging. Clin Neurophysiol. (2015) 126:2207–15. doi: 10.1016/j.clinph.2015.01.008
37. Rosenhall U. Degenerative Patterns in the Aging Human Vestibular Neuro-Epithelia. Acta Otolaryngol. (1973) 76:208–20. doi: 10.3109/00016487309121501
38. Singh NK, Firdose H. Characterizing the age and stimulus frequency interaction for ocular vestibular-evoked myogenic potentials. Ear Hear. (2018) 39:251–9. doi: 10.1097/AUD.0000000000000482
39. Walther LE, Westhofen M. Presbyvertigo-Aging of otoconia and vestibular sensory cells. J Vestib Res. (2007) 17:89–92. doi: 10.3233/VES-2007-172-303
40. Rosengren SM, Colebatch JG, Young AS, Govender S, Welgampola MS. Vestibular evoked myogenic potentials in practice: methods, pitfalls and clinical applications. Clin Neurophysiol Pract. (2019) 4:47–68. doi: 10.1016/j.cnp.2019.01.005
41. Govender S, Rosengren SM, Colebatch JG. The effect of gaze direction on the ocular vestibular evoked myogenic potential produced by air-conducted sound. Clin Neurophysiol. (2009) 120:1386–91. doi: 10.1016/j.clinph.2009.04.017
42. Singh NK, Firdose H, Barman A. Effect of advancing age on inter-frequency amplitude ratio of ocular vestibular evoked myogenic potentials. Int J Audiol. (2021) 60:995–9. doi: 10.1080/14992027.2021.1893840
43. Curthoys IS, Vulovic V, Burgess AM, Sokolic L, Goonetilleke SC. The response of guinea pig primary utricular and saccular irregular neurons to bone-conducted vibration (Bcv) and air-conducted sound (Acs). Hear Res. (2016) 331:131–43. doi: 10.1016/j.heares.2015.10.019
44. Curthoys IS, Kim J, McPhedran SK, Camp AJ. Bone conducted vibration selectively activates irregular primary otolithic vestibular neurons in the Guinea pig. Exp Brain Res. (2006) 175:256–67. doi: 10.1007/s00221-006-0544-1
45. Curthoys IS, Vulovic V, Burgess AM, Cornell ED, Mezey LE, Macdougall HG, et al. The basis for using bone-conducted vibration or air-conducted sound to test otolithic function. Ann N Y Acad Sci. (2011) 1233:231–41. doi: 10.1111/j.1749-6632.2011.06147.x
46. Thomas MLA, Fitzpatrick D, McCreery R, Janky KL. Big stimulus, little ears: safety in administering vestibular-evoked myogenic potentials in children. J Am Acad Audiol. (2017) 28:395–403. doi: 10.3766/jaaa.15097
47. Rodriguez AI, Thomas MLA, Fitzpatrick D, Janky KL. Effects of high sound exposure during air-conducted vestibular evoked myogenic potential testing in children and young adults. Ear Hear. (2018) 39:269–77. doi: 10.1097/AUD.0000000000000484
48. Fröhlich L, Wilke M, Plontke SK, Rahne T. Influence of bone conduction transducer type and placement on ocular and cervical vestibular evoked myogenic potentials. Sci Rep. (2021) 11:8500. doi: 10.1038/s41598-021-87682-1
Keywords: oVEMP, aging, B81 bone vibrator, vestibular disorder, normal data
Citation: Xu Z, Wang Z, Zhong B, Wang M, Fan X, Ren C, Qi M, Lin Y and Zha D (2022) Effects of aging on ocular vestibular-evoked myogenic potential using ER-3A insert earphone and B81 bone vibrator. Front. Neurol. 13:956996. doi: 10.3389/fneur.2022.956996
Received: 30 May 2022; Accepted: 01 August 2022;
Published: 25 August 2022.
Edited by:
Jose Antonio Lopez-Escamez, Universidad de Granada, SpainReviewed by:
Christopher Pastras, The University of Sydney, AustraliaAngel Ramos-macias, University of Las Palmas de Gran Canaria, Spain
Copyright © 2022 Xu, Wang, Zhong, Wang, Fan, Ren, Qi, Lin and Zha. This is an open-access article distributed under the terms of the Creative Commons Attribution License (CC BY). The use, distribution or reproduction in other forums is permitted, provided the original author(s) and the copyright owner(s) are credited and that the original publication in this journal is cited, in accordance with accepted academic practice. No use, distribution or reproduction is permitted which does not comply with these terms.
*Correspondence: Ying Lin, bHl0ZW1wbGUmI3gwMDA0MDtmbW11LmVkdS5jbg==; Dingjun Zha, emhhZGp1biYjeDAwMDQwO2ZtbXUuZWR1LmNu
†These authors have contributed equally to this work