- 1Department of Neurology, Chang Gung Memorial Hospital at Chiayi, Chang-Gung University College of Medicine, Chiayi City, Taiwan
- 2Department of Diagnostic Radiology, Chang Gung Memorial Hospital at Chiayi, Chang-Gung University College of Medicine, Chiayi City, Taiwan
- 3Department of Neurosurgery, Chang Gung Memorial Hospital at Chiayi, Chang-Gung University College of Medicine, Chiayi City, Taiwan
Background and purpose: The early identification of cardioembolic stroke is critical for the early initiation of anticoagulant treatment. However, it can be challenging to identify the major cardiac source, particularly since the predominant source, paroxysmal atrial fibrillation (AF), may not be present at the time of stroke. In this study, we aimed to evaluate imaging predictors for unrecognized AF in patients with acute ischemic stroke.
Methods: We performed a cross-sectional analysis of data and magnetic resonance imaging (MRI) scans from two prospective cohorts of patients who underwent serial 12-lead electrocardiography and 24-h Holter monitoring to detect unrecognized AF. The imaging patterns in diffusion-weighted imaging and imaging characteristics were assessed and classified. A logistic regression model was used to identify predictive factors for newly detected AF in patients with acute ischemic stroke.
Results: A total of 734 patients were recruited for analysis, with a median age of 72 (interquartile range: 65–79) years and a median National Institutes of Health Stroke Scale score of 4 (interquartile range: 2–6). Of these patients, 64 (8.7%) had newly detected AF during the follow-up period. Stepwise multivariate logistic regression revealed that age ≥75 years [adjusted odds ratio (aOR) 5.66, 95% confidence interval (CI) 2.98–10.75], receiving recombinant tissue plasminogen activator treatment (aOR 4.36, 95% CI 1.65–11.54), congestive heart failure (aOR 6.73, 95% CI 1.85–24.48), early hemorrhage in MRI (aOR 3.62, 95% CI 1.52–8.61), single cortical infarct (aOR 6.49, 95% CI 2.35–17.92), and territorial infarcts (aOR 3.54, 95% CI 1.06–11.75) were associated with newly detected AF. The C-statistic of the prediction model for newly detected AF was 0.764.
Conclusion: Initial MRI at the time of stroke may be useful to predict which patients have cardioembolic stroke caused by unrecognized AF. Further studies are warranted to verify these findings and their application to high-risk patients.
Introduction
Cardioembolic stroke has been reported to account for about one fifth of all cases of ischemic stroke (1), and it is associated with higher stroke severity and recurrence rate (2, 3). Atrial fibrillation (AF) is the leading cause of cardioembolic stroke (4), and oral anticoagulants are the most effective method to prevent cardioembolic stroke recurrence in patients with AF (5, 6). Therefore, the early diagnosis of AF after stroke is critical to allow for the early initiation of anticoagulant treatment. However, it can be challenging to identify AF, particularly as paroxysmal AF may not be present at the time of stroke. In these situations, 24-h Holter monitoring is the standard method to detect AF, however it is still far from satisfactory. Extended electrocardiogram monitoring may improve the detection rate of AF, however it is expensive and inconvenient, limiting its widespread use in clinical practice. Therefore, a method to accurately identify patients with cardioembolism from unrecognized AF is urgently needed.
Previous studies have used several clinical scales to predict new-onset AF, including CHA2DS2-VASc (congestive heart failure, hypertension, age ≥75 years, diabetes mellitus, prior stroke or transient ischemic attack, vascular disease, age 65–74 years, female) (7). Cohorts for Heart and Aging Research in Genomic Epidemiology-AF (CHARGE-AF) (8), and Electronic Health Record–Based AF (EHR-AF) scores (9). These AF scales have also been shown to be highly associated with cardioembolic stroke (10). However, the use of these scales requires a thorough survey and extensive clinical information to enable calculation of the scores, and their sensitivity and specificity are still far from satisfactory, which limits their usage in the clinic.
Magnetic resonance imaging (MRI) is widely used in clinical practice to identify acute ischemic stroke, and it may be useful to identify cardioembolic stroke from unrecognized AF at the time of stroke. Several infarct patterns on MRI support the diagnosis of cardioembolism, including multiple simultaneous infarcts located in one or more major arterial territories of the anterior and/or posterior circulation (11), single cortical infarction or cortical-subcortical infarct without large artery occlusion (12). In addition, the presence of a susceptibility vessel sign (SVS) in a gradient recalled echo (GRE) MR imaging sequence is associated with erythrocyte-rich thrombus and cardioembolism (13).
In this study, we aimed to evaluate the MRI characteristics associated with newly detected AF among two prospective cohorts of patients with acute ischemic stroke who did not have AF at baseline.
Materials and methods
Patients
We analyzed data from two prospective cohorts at Chang Gung Memorial Hospital (14). The first cohort was derived from the Atrial Fibrillation Trial to Evaluate Real-world Procedures for their Utility in helping to Lower Stroke Events (AFTER-PULSE), which compared the detection rate of AF using serial 12-lead electrocardiography vs. 24-h Holter monitoring within 3 months after the index ischemic stroke event, and included elderly patients with no known AF between October 2015 and July 2018 (14). The second cohort was derived from an observational study conducted between January 2014 and September 2017, which evaluated the correlation between left atrial enlargement and new-onset AF among patients with no known AF after their index ischemic stroke event; all patients underwent serial 12-lead electrocardiography and were followed up for 6 months.
Among these two cohorts, we selected patients who had undergone MRI within 5 days after the index stroke event and had a visible acute infarction in diffusion-weighted imaging (DWI). A detailed flow chart of patient selection is shown in Figure 1. In both cohorts, data on sex, age, and a medical history of diabetes mellitus, hypertension, hypercholesterolemia, congestive heart failure, prior cerebrovascular disease and prior coronary artery disease were recorded. Systolic and diastolic blood pressure values, blood cell counts and biochemistry data were collected on admission. Neurological deficits were evaluated using the National Institutes of Health Stroke Scale (NIHSS) when the patient arrived at hospital, and the modified Rankin scale at the 90th day.
MRI protocol and image analysis
All data were collected using a 3 Tesla Siemens Verio MRI system (Siemens Medical System, Erlangen, Germany) or a 1.5-T Philips Gyroscan Intera scanner (Philips Medical Systems, Best, The Netherlands).
Standard sequences included axial DWI, fluid-attenuated inversion recovery images, axial T1- and T2-weighted images, and three-dimensional time of flight angiography covering the extracranial carotid artery and circle of Willis. Patients also received either axial T2*-GRE imaging or susceptibility-weighted imaging (SWI). The imaging data were evaluated by two stroke neurologists, who were blinded to the clinical information. If there were any discrepancies in the interpretation of the images, the two readers discussed the data further or consulted a third reader to form a consensus.
Definition of imaging predictors
Classification of DWI patterns
We divided the patients into the following groups based on the observed DWI patterns with reference to previous reports (Supplementary Figure 1) (11, 12, 15, 16), and the illustrated patients are shown in Figure 2 and Supplementary Figure 2.
1. Territorial infarct, involving territories of the internal carotid artery (ICA) or middle cerebral artery (MCA), with at least one division. The DWI pattern should be homogenous, including cortical and subcortical areas. Small separate infarctions in the same vascular territory or in different territories were also classified as territorial infarcts.
2. Single cortical infarct, with a length <30 mm and not involving the subcortical area.
3. Single subcortical infarct (diameter ≤20 mm) in the penetrating artery territories.
4. Single subcortical infarct (diameter ≥20 mm) in the penetrating artery territories.
5. Small scattered cortical or subcortical infarcts, defined as small scattered cortical infarctions with a length <30 mm or multiple subcortical lesions but not restricted to the penetrating artery territories.
6. Border zone infarcts, including internal border zone infarction in the MCA territory or infarction at the MCA-anterior cerebral artery or MCA-posterior cerebral artery cortical border zones.
7. Other cortical and subcortical infarcts, defined as lesions ≥30 mm in one vascular territory but not classified in the patterns above.
8. Multiple territories: multiple infarcts in different vascular territories, including in both left and right ICA territories or in both anterior and posterior circulation territories.
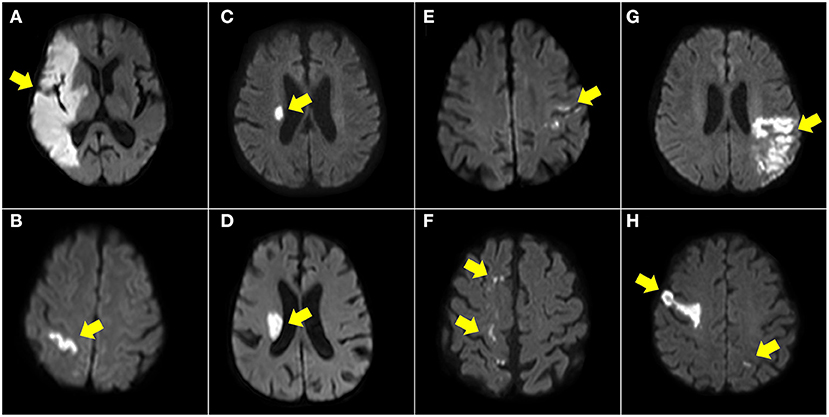
Figure 2. Illustration of DWI patterns in anterior circulation. Territory infarct (A), single cortical infarct (B), single subcortical infarct (<20 mm) (C), single subcortical infarct (>20 mm) (D), small scattered cortical or subcortical infarcts (E), border zone infarcts (F), other cortical and subcortical infarcts (G), and multiple territories (H).
Early hemorrhage
Hemorrhagic transformation after ischemic stroke is related to cardioembolism due to a large core infarction and recanalization. The radiologic appearance of hemorrhagic transformation after ischemic stroke was defined according to the European Cooperative Acute Stroke Study II trial, including hemorrhagic infarction and parenchymal infarction (17). These hemorrhages appeared as hypointense signals within or next to the areas of infarction in SWI or GRE imaging (Figures 3C,D), excluding hemorrhage mimics, such as vessels, mineralization, air-bone interfaces, partial volume artifacts, or microbleeds. Early hemorrhage was defined as any hemorrhagic transformation within 5 days of stroke onset.
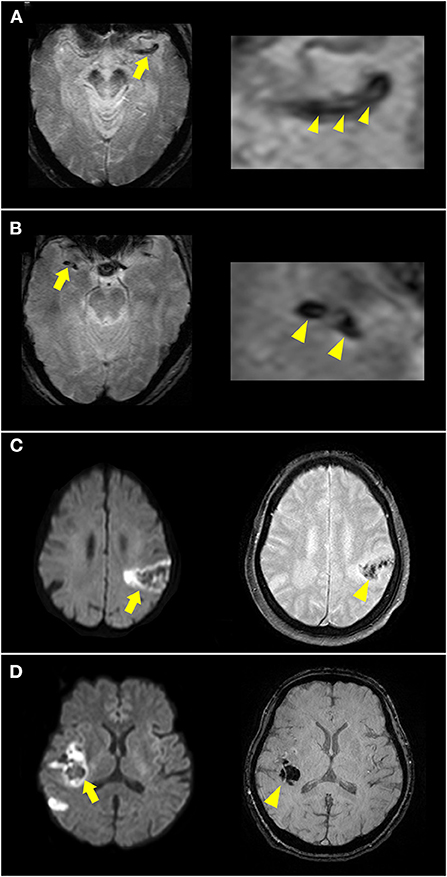
Figure 3. Illustration of susceptibility vessel sign (SVS) and early hemorrhage. (A) A hypointense signal was noted at the left occluded middle cerebral artery (MCA) [(A), arrow] in T2*-weighted imaging, with a low-intensity core surrounded by a signal of higher intensity [(A) arrowheads], suggesting a 2-layered SVS. (B) A homogenous hypointense signal was noted at the right occluded MCA [(B), arrow and arrowheads] in T2*-weighted imaging suggesting SVS. (C) DWI showed acute infarction in the left MCA territory with restricted diffusion and hypointense lesions inside (arrow). T2*-weighted imaging showed hypointense signal changes (arrowhead) suggesting hemorrhagic infarction. (D) DWI showed acute infarction in the right MCA territory (arrow). Susceptibility-weighted imaging showed a hypointense space-occupying lesion (arrowhead) suggesting parenchymal hemorrhage.
SVS in SWI and GRE imaging
SVSs from deoxygenated hemoglobin in red clots and imaging markers are highly related to cardioembolism. SVS was defined as a hypointense signal in the symptomatic occlusive vessel on GRE imaging or SWI that was larger than the contralateral arterial diameter (Figures 3A,B).
Statistical analysis
Descriptive statistics were presented as frequencies, means and standard deviations, or medians and interquartile ranges (IQRs), as appropriate. The Kolmogorov-Smirnov test was used to examine the normality of continuous variables, which were then compared using a Student's t-test or Mann-Whitney U-test, as appropriate. Categorical data were analyzed using a chi-squared test or Fisher's exact test. All tests were two-tailed, and a p-value < 0.05 was considered to indicate a statistically significant difference.
Factors potentially associated with newly-detected AF were evaluated using descriptive statistics. Univariable logistic regression models were used to evaluate candidate variables. Odds ratios (ORs) together with 95% confidence intervals (CIs) were reported, and p-values < 0.05 were considered to indicate a statistically significant difference. We built a multivariable regression model based on all potential predictors using forward stepwise selection with p < 0.05. Then the “nomolog” package was used to establish a predictive model and generate the nomogram to predict newly detected AF. We also examined discrimination using the C-statistic in our regression model, CHA2DS2-VASc, CHARGE-AF, and EHR-AF scores. Analyses were performed using Stata SE software (version 15.1; StataCorp, College Station, TX).
Results
A total of 1,054 patients were selected from the two previous trials (Figure 1). After excluding 263 patients without MRI or without complete MRI sequences and 54 patients with transient ischemic stroke, a total of 734 patients were recruited for analysis. The median age of the patients was 72 (IQR 65–79) years, and the median NIHSS score was 4 (IQR 2–6). Among them, 64 (8.7%) patients had AF during the follow-up period; 46 (71.9%) within 14 days, 8 (12.5%) within 15–90 days, and 10 (15.6%) within 90–180 days following stroke onset. Among the 179 patients with embolic stroke of an undetermined source (ESUS), 30 (16.8%) were detected as having AF.
According to the classification of DWI patterns, there were 18 (2.3%) territorial infarcts, 27 (3.7%) single cortical infarcts, 289 (39.4%) single subcortical infarcts with a diameter <20 mm, 61 (8.3%) single subcortical infarcts with a diameter ≥20 mm, 96 (13.1%) small scattered cortical or subcortical infarcts, 58 (7.9%) border zone infarcts, 143 (19.5%) other cortical and subcortical infarcts, and 42 (5.7%) infarcts in multiple territories. Among the included patients, 301 had >50% stenosis or occlusion of the relevant vessels, 40 (5.5%) had hemorrhagic infarcts, and 4 (0.5%) had parenchymal hemorrhage.
Compared to the patients without newly detected AF (Table 1), the patients with AF were significantly older (80 vs. 71 years; p < 0.001), included more females (48.4 vs. 34.7%; p = 0.028), had a higher rate of ESUS (46.9 vs. 22.2%; p < 0.001), lower incidence of diabetes mellitus (31.3 vs. 47.8%; p = 0.011), higher incidence of receiving recombinant tissue plasminogen activator (rt-PA) treatment (12.5 vs. 4.0%; p = 0.002), and higher incidence of congestive heart failure (7.8 vs. 1.3%; p < 0.001). The imaging patterns of territorial infarcts, single cortical infarct, and early hemorrhage were more likely to be associated with newly detected AF, whereas single subcortical infarcts (diameter <20 mm) and border zone infarcts were less likely to be associated with newly detected AF.
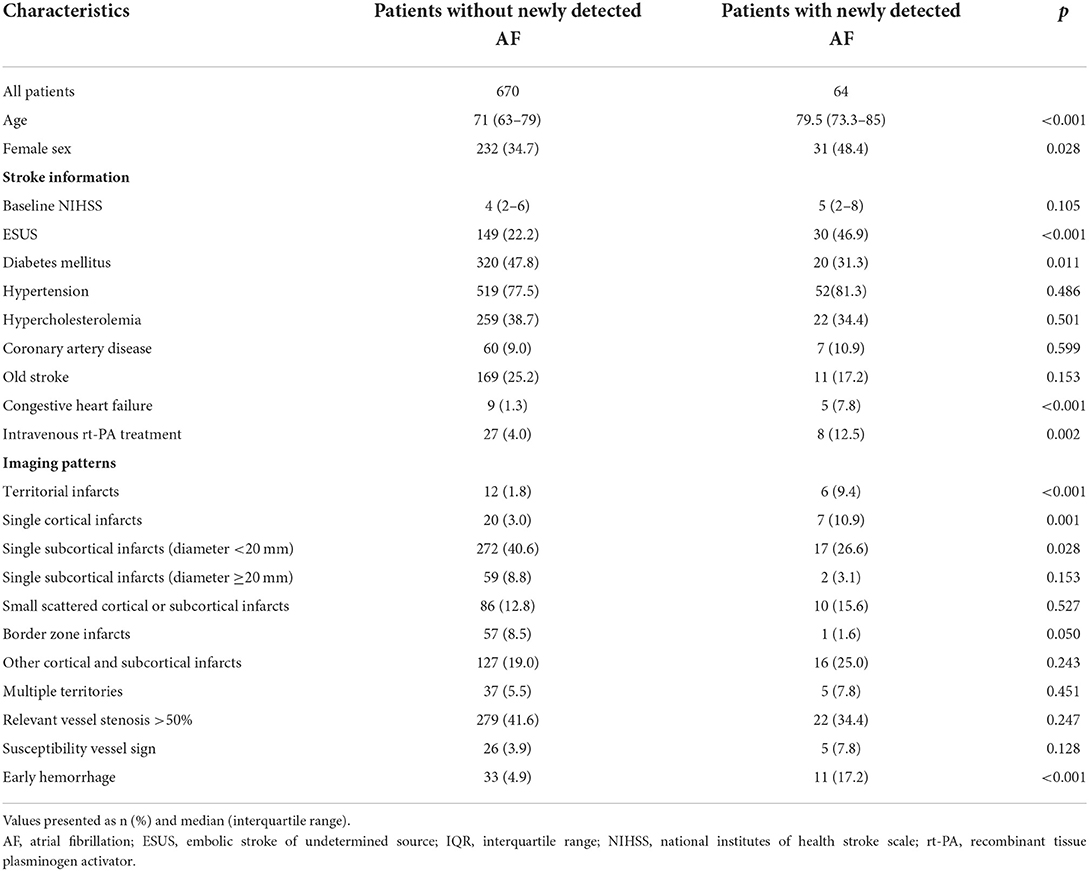
Table 1. Baseline characteristics, imaging findings and outcomes, and correlations with newly detected atrial fibrillation.
In multivariate logistic regression analysis (Table 2), age ≥75 years [adjusted odds ratio (aOR) 5.66, 95% CI 2.98–10.75], receiving rt-PA treatment (aOR 4.36, 95% CI 1.65–11.54), congestive heart failure (aOR 6.73, 95% CI 1.85–24.48), early hemorrhage in MRI (aOR 3.62, 95% CI 1.52–8.61), single cortical infarct (aOR 6.49, 95% CI 2.35–17.92), and territorial infarcts (aOR 3.54, 95% CI 1.06–11.75) were associated with newly detected AF. A nomogram of the prediction model for newly detected AF is shown in Figure 4. The C-statistic of the prediction model for newly detected AF was 0.764 for all patients, and 0.811 for the patients with ESUS. Using CHA2DS2-VASc, EHR-AF and CHARGE-AF score, the C-statistics for the prediction of newly detected AF were 0.551, 0.690, and 0.702, respectively, for all patients.
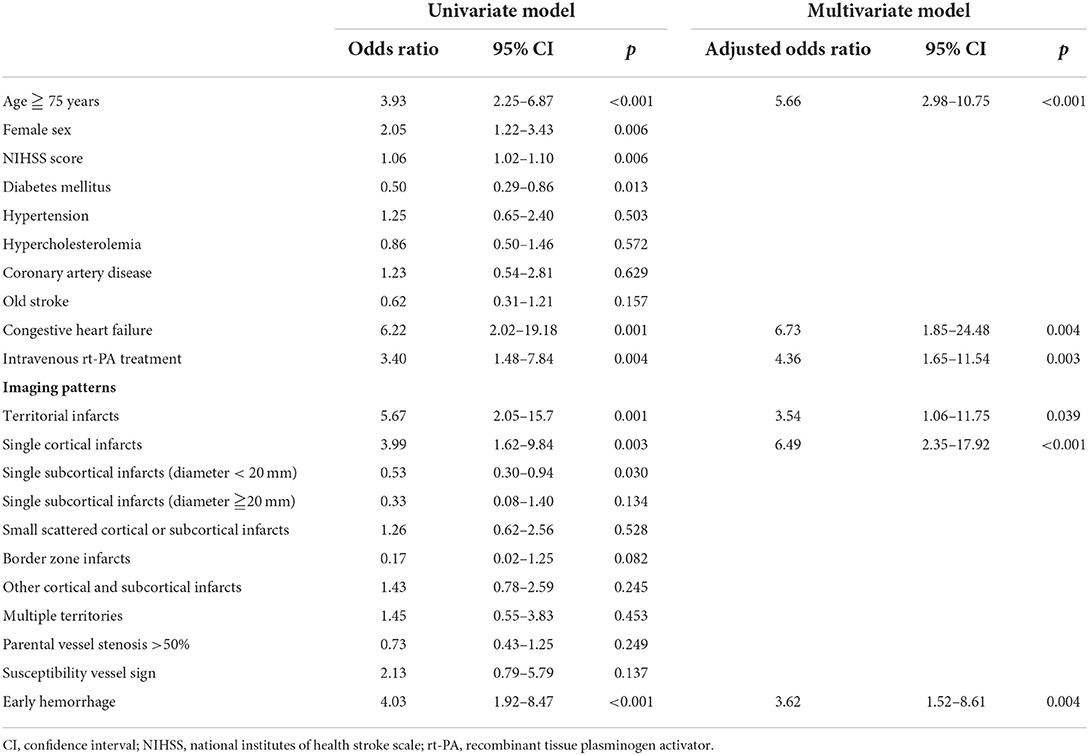
Table 2. Logistic regression for the predictors of newly detected atrial fibrillation in the patients with acute ischemic stroke.
Discussion
In this study, we demonstrated that specific infarction patterns, including single cortical infarctions, territorial infarctions, and early hemorrhage in MRI independently predicted newly detected AF in patients with acute ischemic stroke. Our findings also challenge the current imaging definition of ESUS, which includes large single subcortical infarctions, as they were not associated with newly detected AF in the current study. Furthermore, the use of additional imaging parameters improved the predictive accuracy for newly detected AF compared to current scales using clinical characteristics (10). A clinical and imaging prediction model may be useful to determine which high-risk patients should receive extended electrocardiogram monitoring. In keeping with previous studies which reported an association between territorial infarcts and cardioembolic stroke, we also found that territorial infarcts were highly associated with newly detected AF in this study (12, 18–20). For acute stroke caused by large vessel occlusion, cardioembolic stroke tends to have less collateral flow compared with atherosclerotic stroke because of the more abrupt perfusion compromise in cardioembolic occlusion (21, 22). As there are fewer leptomeningeal collaterals in cardioembolic stroke, the infarct pattern is unsurprisingly larger, wedge-shaped and homogenous in both cortical and subcortical areas (18, 23). Single or multiple cortical infarcts in either the cerebral or cerebellar cortex have been reported to be related to cardioembolism or AF, suggesting small or fragmented cardio-emboli (24–26). However, only single cortical infarcts were associated with newly detected AF in our study, and multiple cortical infarcts were not. The reason for this finding could be that multiple cortical infarcts are also related to artery-to-artery embolism from atherosclerotic plaques of steno-occlusive vessels, whereas single cortical infarcts are more likely to be related to the cardioembolism (26).
Multiple simultaneous infarcts in multiple territories have been reported to be more prevalent in patients with an acute ischemic stroke of cardioembolic origin (27) and in patients with occult AF in ESUS (28). However, in the current study, there was no significant difference in infarctions in multiple territories between the patients with or without newly detected AF. The reason for this may be that we excluded patients with a documented risk of cardioembolism and included patients with small vessel occlusion. Therefore, only 5.2% of the patients presented with multiple circulation infarcts in our study. The lack of significant correlation between multiple simultaneous infarcts in multiple territories and AF may also be due to the small sample size. In addition, we did not exclude patients with large atherosclerotic occlusion, which may have also led to multiple circulation infarcts, such as in bilateral anterior cerebral arteries from unilateral ICA or a fetal type posterior cerebral artery. Despite these limitations, our findings are close to clinical practice and provide valuable information that the presence of multiple simultaneous infarctions is not as useful as previously assumed for the prediction of unrecognized AF.
The infarction pattern of single subcortical infarcts with a diameter <20 mm, commonly called lacunar infarcts, was less likely to be associated with newly detected AF in this study, which is comparable with previous reports (12, 27). A single subcortical infarct with a diameter ≥20 mm still showed a non-significant trend toward identifying the absence of AF. The major reason for this may be that larger single subcortical infarcts are usually attributed to branch atheromatous disease caused by atherosclerotic plaques involving parent artery perforators and less to cardioembolic occlusion (29). Nevertheless, large single subcortical infarcts with a diameter ≥20 mm are classified as ESUS according to imaging criteria. Since their pathologies favor an atherosclerotic origin rather than cardioembolism, our findings provide evidence against the current definition of ESUS, which includes single subcortical infarcts ≥20 mm (30). In the RE-SPECT ESUS and NAVIGATE ESUS trials, rivaroxaban and dabigatran were not shown to be beneficial in preventing recurrent ESUS. A possible reason for this finding may be the heterogeneity of the recruited patients (31). In our study, 34% (64/179) of the patients with ESUS had a single subcortical infarction with a diameter ≥20 mm. Therefore, it may be reasonable to remove single subcortical infarcts with a diameter ≥20 mm from the imaging definition of ESUS, and instead use a new definition involving MRI imaging.
Both internal border zone infarction and cortical border zone infarction are usually associated with hemodynamic failure and microembolization from steno-occlusive disease or sometimes systemic hypotension (32, 33). When border zone infarction is combined with stenosis or occlusion of relevant vessels, it is usually presumed and classified to be a large atherosclerotic artery stroke in clinical practice. Hence, it is no surprise that the pattern of border zone infarction was less likely to be associated with newly detected AF in our study. However, time of flight angiography in MRI could not clearly distinguish between atherosclerotic and cardioembolic occlusion, so that occlusion or stenosis >50% of relevant vessels failed to predict newly detected AF. Although SVS with specific morphologies has been shown to be associated with cardioembolic stroke (34, 35), the trend was not statistically significant in our study, probably due to the low number of patients (4.2%) with SVS. Further studies with a larger sample size and a precise definition of SVS are warranted to test its efficacy for the prediction of newly detected AF.
Cardioembolic stroke, especially that caused by AF, is strongly associated with hemorrhagic transformation after acute ischemic stroke, probably due to the inherent characteristics of large infarction core and early recanalization (36–38). T2*-weighted GRE and SWI are sensitive methods of detecting early hemorrhagic transformation after acute ischemic stroke (39, 40). In our study, early hemorrhagic transformation in MRI was an independent predictor for newly detected AF.
In addition to specific imaging patterns, we also found that certain clinical characteristics, including female sex, age ≥75 years, and heart failure, were associated with an increased risk of newly detected AF. These factors are also included in the CHA2DS2-VASc score, which is used to predict the risk of stroke in individuals with AF, and predict the risk of AF in individuals without AF (41, 42). The CHARGE-AF and EHR-AF scores have been used to predict new-onset AF in population-based cohorts and to predict cardioembolic stroke at the time of stroke (9, 10, 43). AF has also been reported to account for ~25% of patients receiving thrombolytic therapy because of worse stroke severity (44, 45). Therefore, the patients receiving intravenous rt-PA treatment were related to newly detected AF. The prediction model in our study was robustly associated with newly detected AF and could moderately discriminate occult cardioembolism from unknown paroxysmal AF. A prediction model including MRI information may improve the accuracy of predicting newly detected AF at the time of stroke.
Compared with the NAVIGATE and RESPECT ESUS trials, the definition of cardiac embolism in the ATTICUS trial included additional risk factors (46). Despite the higher rate of newly detected AF (23%), the trial still failed to demonstrate the efficacy of apixaban in ESUS patients. Moreover, apixaban treatment was not superior to “aspirin with the switch to apixaban in case of AF detection by mandatory cardiac monitoring” in preventing new ischemic lesions during follow-up. This may emphasize the importance of extended electrocardiogram monitoring in patients with ESUS, and our prediction model may be useful to identify high-risk patients.
There are several limitations to this study. First, we presumed that the specific infarction patterns were related to cardioembolism due to unknown paroxysmal AF, however other etiologies of embolic stroke were not explored, such as patent foramen ovale, valvular heart disease, aortic arch atheroma, cancer-associated coagulopathy, etc. Second, some patients only received serial 12-lead electrocardiography and were followed up for 3 months. The incidence of newly detected AF may therefore be underestimated in our study, even though the AF detection rate of 8.7% in our ESUS patients is comparable to the 8.9% reported in a previous trial using an insertable cardiac monitor for 6 months (47). The small sample size and the fact that we did not use prolonged cardiac monitoring may mean that we missed potential predictors for unknown paroxysmal AF. Third, we did not recruit patients with endovascular thrombectomy, so the imaging patterns cannot be applied in these patients. Fourth, our study has the inherent drawbacks of selection and detection bias, since one selected cohort excluded patients <65 years old and end-stage renal disease, and we only selected patients who underwent MRI and had a milder stroke severity. Despite these limitations, our study still provides valuable information which may improve the prediction of newly detected AF after acute ischemic stroke.
Conclusion
In addition to clinically known risk factors for AF, our study revealed that MRI at stroke onset provides critical clues for the prediction of newly detected AF, including single cortical infarcts, territorial infarcts and early hemorrhage. Future studies are warranted to verify this new prediction model and to assess whether the identification of AF can be enhanced to improve outcomes after acute ischemic stroke.
Data availability statement
The original contributions presented in the study are included in the article/Supplementary material, further inquiries can be directed to the corresponding author.
Ethics statement
The protocols of these studies were approved by the Institutional Review Board of Chang Gung Memorial Hospital (103-7597B and 104-9611C). The patients/participants provided their written informed consent to participate in this study.
Author contributions
Y-CH interpreted data, analyzed imaging, and revised the manuscript. C-HC analyzed imaging and drafted the manuscript. ML designed the study and interpreted data. J-DL and J-TY acquired data. Y-HT analyzed the imaging. H-HW conducted the statistical analyses. All authors reviewed the manuscript and gave final approval to the version to be published.
Funding
This study was supported by Chang Gung Memorial Hospital research grants (CMRPG6J0361, CMRPG6J0362, and CORPG6G0223).
Acknowledgments
We thank Yi-Chen Kuo for assisting us with this study.
Conflict of interest
The authors declare that the research was conducted in the absence of any commercial or financial relationships that could be construed as a potential conflict of interest.
Publisher's note
All claims expressed in this article are solely those of the authors and do not necessarily represent those of their affiliated organizations, or those of the publisher, the editors and the reviewers. Any product that may be evaluated in this article, or claim that may be made by its manufacturer, is not guaranteed or endorsed by the publisher.
Supplementary material
The Supplementary Material for this article can be found online at: https://www.frontiersin.org/articles/10.3389/fneur.2022.952462/full#supplementary-material
References
1. Palacio S, Hart RG. Neurologic manifestations of cardiogenic embolism: an update. Neurol Clin. (2002) 20:179–93. doi: 10.1016/S0733-8619(03)00058-6
2. Sage JI, Van Uitert RL. Risk of recurrent stroke in patients with atrial fibrillation and non-valvular heart disease. Stroke. (1983) 14:537–40. doi: 10.1161/01.STR.14.4.537
3. Lin HJ, Wolf PA, Kelly-Hayes M, Beiser AS, Kase CS, Benjamin EJ, et al. Stroke severity in atrial fibrillation. The Framingham study. Stroke. (1996) 27:1760–4. doi: 10.1161/01.STR.27.10.1760
4. Wolf PA, Abbott RD, Kannel WB. Atrial fibrillation as an independent risk factor for stroke: the Framingham study. Stroke. (1991) 22:983–8. doi: 10.1161/01.STR.22.8.983
5. Ntaios G, Papavasileiou V, Makaritsis K, Vemmos K, Michel P, Lip GYH. Real-world setting comparison of nonvitamin-K antagonist oral anticoagulants versus vitamin-K antagonists for stroke prevention in atrial fibrillation: a systematic review and meta-analysis. Stroke. (2017) 48:2494–503. doi: 10.1161/STROKEAHA.117.017549
6. Lip GY, Lane DA. Stroke prevention in atrial fibrillation: a systematic review. J Am Med Assoc. (2015) 313:1950–62. doi: 10.1001/jama.2015.4369
7. Saliba W, Gronich N, Barnett-Griness O, Rennert G. Usefulness of Chads2 and Cha2ds2-Vasc scores in the prediction of new-onset atrial fibrillation: a population-based study. Am J Med. (2016) 129:843–9. doi: 10.1016/j.amjmed.2016.02.029
8. Alonso A, Roetker NS, Soliman EZ, Chen LY, Greenland P, Heckbert SR. Prediction of atrial fibrillation in a racially diverse cohort: the multi-ethnic study of atherosclerosis (mesa). J Am Heart Assoc. (2016) 5:e003077. doi: 10.1161/JAHA.115.003077
9. Hulme OL, Khurshid S, Weng L-C, Anderson CD, Wang EY, Ashburner JM, et al. Development and validation of a prediction model for atrial fibrillation using electronic health records. JACC Clin Electrophysiol. (2019) 5:1331–41. doi: 10.1016/j.jacep.2019.07.016
10. Khurshid S, Trinquart L, Weng LC, Hulme OL, Guan W, Ko D, et al. Atrial fibrillation risk and discrimination of cardioembolic from noncardioembolic stroke. Stroke. (2020) 51:1396–403. doi: 10.1161/STROKEAHA.120.028837
11. Rovira A, Grive E, Rovira A, Alvarez-Sabin J. Distribution territories and causative mechanisms of ischemic stroke. Eur Radiol. (2005) 15:416–26. doi: 10.1007/s00330-004-2633-5
12. Kang DW, Chalela JA, Ezzeddine MA, Warach S. Association of ischemic lesion patterns on early diffusion-weighted imaging with toast stroke subtypes. Arch Neurol. (2003) 60:1730–4. doi: 10.1001/archneur.60.12.1730
13. Cho KH, Kim JS, Kwon SU, Cho AH, Kang DW. Significance of susceptibility vessel sign on T2*-weighted gradient echo imaging for identification of stroke subtypes. Stroke. (2005) 36:2379–83. doi: 10.1161/01.STR.0000185932.73486.7a
14. Huang WY, Lee M, Sung SF, Tang SC, Chang KH, Huang YS, et al. Atrial fibrillation trial to evaluate real-world procedures for their utility in helping to lower stroke events: a randomized clinical trial. Int J Stroke. (2021) 16:300–10. doi: 10.1177/1747493020938297
15. Bang OY, Lee PH, Heo KG, Joo US, Yoon SR, Kim SY. Specific Dwi lesion patterns predict prognosis after acute ischaemic stroke within the Mca territory. J Neurol Neurosurg Psychiatry. (2005) 76:1222–8. doi: 10.1136/jnnp.2004.059998
16. Lopez-Cancio E, Matheus MG, Romano JG, Liebeskind DS, Prabhakaran S, Turan TN, et al. Infarct patterns, collaterals and likely causative mechanisms of stroke in symptomatic intracranial atherosclerosis. Cerebrovasc Dis. (2014) 37:417–22. doi: 10.1159/000362922
17. Hacke W, Kaste M, Fieschi C, von Kummer R, Davalos A, Meier D, et al. Randomised double-blind placebo-controlled trial of thrombolytic therapy with intravenous alteplase in acute ischaemic stroke (Ecass Ii). Second European-Australasian Acute Stroke study. Investigators Lancet. (1998) 352:1245–51. doi: 10.1016/S0140-6736(98)08020-9
18. Cho HJ, Yang JH, Jung YH, Kim YD, Choi HY, Nam HS, et al. Cortex-sparing infarctions in patients with occlusion of the middle cerebral artery. J Neurol Neurosurg Psychiatry. (2010) 81:859–63. doi: 10.1136/jnnp.2009.195842
19. Jung JM, Kwon SU, Lee JH, Kang DW. Difference in infarct volume and patterns between cardioembolism and internal carotid artery disease: focus on the degree of cardioembolic risk and carotid stenosis. Cerebrovasc Dis. (2010) 29:490–6. doi: 10.1159/000297965
20. Wessels T, Wessels C, Ellsiepen A, Reuter I, Trittmacher S, Stolz E, et al. Contribution of diffusion-weighted imaging in determination of stroke etiology. Am J Neuroradiol. (2006) 27:35–9.
21. Rebello LC, Bouslama M, Haussen DC, Grossberg JA, Dehkharghani S, Anderson A, et al. Stroke etiology and collaterals: atheroembolic strokes have greater collateral recruitment than cardioembolic strokes. Eur J Neurol. (2017) 24:762–7. doi: 10.1111/ene.13287
22. Lin C-H, Tsai Y-H, Lee J-D, Weng H-H, Yang J-T, Lin L-C, et al. Magnetic resonance perfusion imaging provides a significant tool for the identification of cardioembolic stroke. Curr Neurovasc Res. (2016) 13:271–6. doi: 10.2174/1567202613666160901143040
23. Helgason CM. Cardioembolic stroke: topography and pathogenesis. Cerebrovasc Brain Metab Rev. (1992) 4:28–58.
24. Favilla CG, Ingala E, Jara J, Fessler E, Cucchiara B, Messé SR, et al. Predictors of finding occult atrial fibrillation after cryptogenic stroke. Stroke. (2015) 46:1210–5. doi: 10.1161/STROKEAHA.114.007763
25. Ter Schiphorst A, Tatu L, Thijs V, Demattei C, Thouvenot E, Renard D. Small obliquely oriented cortical cerebellar infarctions are associated with cardioembolic stroke. BMC Neurol. (2019) 19:1328. doi: 10.1186/s12883-019-1328-0
26. Lee DK, Kim JS, Kwon SU, Yoo SH, Kang DW. Lesion patterns and stroke mechanism in atherosclerotic middle cerebral artery disease: early diffusion-weighted imaging study. Stroke. (2005) 36:2583–8. doi: 10.1161/01.STR.0000189999.19948.14
27. Sharobeam A, Churilov L, Parsons M, Donnan GA, Davis SM, Yan B. Patterns of infarction on Mri in patients with acute ischemic stroke and cardio-embolism: a systematic review and meta-analysis. Front Neurol. (2020) 11:606521. doi: 10.3389/fneur.2020.606521
28. Yushan B, Tan BYQ, Ngiam NJ, Chan BPL, Luen TH, Sharma VK, et al. Association between bilateral infarcts pattern and detection of occult atrial fibrillation in embolic stroke of undetermined source (Esus) patients with insertable cardiac monitor (Icm). J Stroke Cerebrovasc Dis. (2019) 28:2448–52. doi: 10.1016/j.jstrokecerebrovasdis.2019.06.025
29. Petrone L, Nannoni S, Del Bene A, Palumbo V, Inzitari D. Branch atheromatous disease: a clinically meaningful, yet unproven concept. Cerebrovasc Dis. (2016) 41:87–95. doi: 10.1159/000442577
30. Kamel H, Navi BB, Parikh NS, Merkler AE, Okin PM, Devereux RB, et al. Machine learning prediction of stroke mechanism in embolic strokes of undetermined source. Stroke. (2020) 51:29305. doi: 10.1161/STROKEAHA.120.029305
31. Maier IL, Schregel K, Karch A, Weber-Krueger M, Mikolajczyk RT, Stahrenberg R, et al. Association between embolic stroke patterns, esus etiology, and new diagnosis of atrial fibrillation: a secondary data analysis of the find-Af trial. Stroke Res Treat. (2017) 2017:1–6. doi: 10.1155/2017/1391843
32. Forster A, Szabo K, Hennerici MG. Pathophysiological concepts of stroke in hemodynamic risk zones–do hypoperfusion and embolism interact? Nat Clin Pract Neurol. (2008) 4:216–25. doi: 10.1038/ncpneuro0752
33. Man BL, Fu YP, Chan YY, Lam W, Hui AC, Leung WH, et al. Lesion patterns and stroke mechanisms in concurrent atherosclerosis of intracranial and extracranial vessels. Stroke. (2009) 40:3211–5. doi: 10.1161/STROKEAHA.109.557041
34. Zhang R, Zhou Y, Liu C, Zhang M, Yan S, Liebeskind DS, et al. Overestimation of susceptibility vessel sign: a predictive marker of stroke cause. Stroke. (2017) 48:1993–6. doi: 10.1161/STROKEAHA.117.016727
35. Yamamoto N, Satomi J, Tada Y, Harada M, Izumi Y, Nagahiro S, et al. Two-layered susceptibility vessel sign on 3-tesla T2*-weighted imaging is a predictive biomarker of stroke subtype. Stroke. (2015) 46:269–71. doi: 10.1161/STROKEAHA.114.007227
36. Paciaroni M, Agnelli G, Corea F, Ageno W, Alberti A, Lanari A, et al. Early hemorrhagic transformation of brain infarction: rate, predictive factors, and influence on clinical outcome. Stroke. (2008) 39:2249–56. doi: 10.1161/STROKEAHA.107.510321
37. Álvarez-Sabín J, Maisterra O, Santamarina E, Kase CS. Factors influencing haemorrhagic transformation in ischaemic stroke. Lancet Neurol. (2013) 12:689–705. doi: 10.1016/S1474-4422(13)70055-3
38. Molina CA, Montaner J, Abilleira S, Ibarra B, Romero F, Arenillas JF, et al. Timing of spontaneous recanalization and risk of hemorrhagic transformation in acute cardioembolic stroke. Stroke. (2001) 32:1079–84. doi: 10.1161/01.STR.32.5.1079
39. Huang P, Chen CH, Lin WC, Lin RT, Khor GT, Liu CK. Clinical applications of susceptibility weighted imaging in patients with major stroke. J Neurol. (2012) 259:1426–32. doi: 10.1007/s00415-011-6369-2
40. Liu L, Wu B, Zhao J, Cao Y, Dedhia N, Caplan LR, et al. Computed tomography perfusion alberta stroke program early computed tomography score is associated with hemorrhagic transformation after acute cardioembolic stroke. Front Neurol. (2017) 8:591. doi: 10.3389/fneur.2017.00591
41. Zuo M-L, Liu S, Chan K-H, Lau K-K, Chong B-H, Lam K-F, et al. The Chads2 and Cha2ds2–vasc scores predict new occurrence of atrial fibrillation and ischemic stroke. J Intervention Cardiac Electrophysiol. (2013) 37:47–54. doi: 10.1007/s10840-012-9776-0
42. Lip GYH, Nieuwlaat R, Pisters R, Lane DA, Crijns HJGM. Refining clinical risk stratification for predicting stroke and thromboembolism in atrial fibrillation using a novel risk factor-based approach. Chest. (2010) 137:263–72. doi: 10.1378/chest.09-1584
43. Christophersen IE, Yin X, Larson MG, Lubitz SA, Magnani JW, McManus DD, et al. A comparison of the charge–Af and the Cha2ds2-vasc risk scores for prediction of atrial fibrillation in the Framingham Heart Study. Am Heart J. (2016) 178:45–54. doi: 10.1016/j.ahj.2016.05.004
44. Hu Y, Ji C. Efficacy and safety of thrombolysis for acute ischemic stroke with atrial fibrillation: a meta-analysis. BMC Neurol. (2021) 21:66. doi: 10.1186/s12883-021-02095-x
45. Saposnik G Gladstone D Raptis R Zhou L Hart RG Investigators Investigators of the Registry of the Canadian Stroke N. Atrial fibrillation in ischemic stroke: predicting response to thrombolysis and clinical outcomes. Stroke. (2013) 44:99–104. doi: 10.1161/STROKEAHA.112.676551
46. Geisler T, Poli S, Meisner C, Schreieck J, Zuern CS, Nagele T, et al. Apixaban for treatment of embolic stroke of undetermined source (atticus randomized trial): rationale and study design. Int J Stroke. (2017) 12:985-−90. doi: 10.1177/1747493016681019
Keywords: ischemic stroke, cryptogenic stroke, cardioembolic stroke, MRI, atrial fibrillation
Citation: Chen C-H, Lee M, Weng H-H, Lee J-D, Yang J-T, Tsai Y-H and Huang Y-C (2022) Identification of magnetic resonance imaging features for the prediction of unrecognized atrial fibrillation in acute ischemic stroke. Front. Neurol. 13:952462. doi: 10.3389/fneur.2022.952462
Received: 25 May 2022; Accepted: 25 July 2022;
Published: 13 September 2022.
Edited by:
Chengcheng Zhu, University of Washington, United StatesReviewed by:
Zhen Zhang, Longgang Central Hospital, Shenzhen, ChinaHans Worthmann, Hannover Medical School, Germany
Copyright © 2022 Chen, Lee, Weng, Lee, Yang, Tsai and Huang. This is an open-access article distributed under the terms of the Creative Commons Attribution License (CC BY). The use, distribution or reproduction in other forums is permitted, provided the original author(s) and the copyright owner(s) are credited and that the original publication in this journal is cited, in accordance with accepted academic practice. No use, distribution or reproduction is permitted which does not comply with these terms.
*Correspondence: Yen-Chu Huang, eWVuY2h1Lmh1YW5nJiN4MDAwNDA7bXNhLmhpbmV0Lm5ldA==