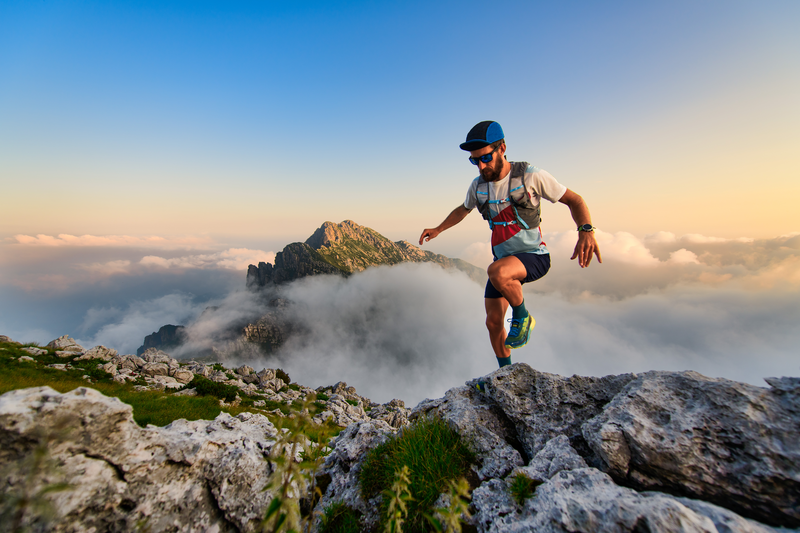
94% of researchers rate our articles as excellent or good
Learn more about the work of our research integrity team to safeguard the quality of each article we publish.
Find out more
REVIEW article
Front. Neurol. , 27 July 2022
Sec. Headache and Neurogenic Pain
Volume 13 - 2022 | https://doi.org/10.3389/fneur.2022.930383
This article is part of the Research Topic Biomarkers in Migraine Beyond Diagnosis View all 10 articles
The multi-functional neuropeptide calcitonin gene-related peptide (CGRP) plays a major role in the pathophysiology of migraine. The detection of elevated CGRP levels during acute migraine headache was the first evidence of the importance of the peptide. Since then, elevated CGRP levels have been detected not only during spontaneous and experimentally induced migraine attacks but also interictally. However, the detection of CGRP in peripheral blood shows conflicting results. In this respect, alternative detection methods are needed and have been already proposed. This article summarizes what we have learned from studies investigating CGRP in jugular and peripheral blood and reviews the latest state of research concerning the detection of CGRP in saliva and tear fluid as well as their contribution to our understanding of migraine pathophysiology.
Migraine is a highly prevalent disorder with a complex pathophysiology involving the peripheral and central nervous system (1–4). Although many aspects of the pathophysiology remain elusive the importance of the trigemino-vascular system (TVS) with its peripheral and central afferents connecting intracranial vasculature and meninges to the brainstem plays a key role in the generation of migraine pain (5–7). Activation of the trigeminal system leads to release of vasoactive neuropeptides, in particular calcitonin gene-related peptide (CGRP), followed by neurogenic inflammation, nociceptive modulation and peripheral and central sensitization (4, 8). The importance of CGRP in migraine pathophysiology is highly supported by different research results:
(1) CGRP levels are elevated in ictal (during the migraine attack) and interictal (48–72 h headache and medication-free) migraine patients (2),
(2) CGRP levels are reduced after abortive and prophylactic treatment (2),
(3) CGRP can induce migraine-like headaches in migraine patients (9, 10),
(4) CGRP antagonists and CGRP antibodies are effective abortive and prophylactic migraine treatments, respectively (11, 12).
This article reviews our current understanding of CGRP in migraine pathophysiology. It focuses on studies investigating CGRP in different migraine states and discusses what we have learned from measuring CGRP as a marker for migraine.
Calcitonin gene-related peptide (CGRP) is a 37 amino acid regulatory neuropeptide and potent microvascular vasodilator that was first described in 1982 (13). It belongs to the calcitonin family comprising calcitonin, adrenomedullin, adrenomedullin 2 (intermedin) and amylin (14). In humans, two forms, α-CGRP and β-CGRP, are described (15). They show structural similarity and share 94% homology as well as identical binding affinity and intensity (13, 16). Here, the term CGRP will be used, unless otherwise essential.
α-CGRP is located in the central and peripheral nervous system and is primarily produced and stored in Aδ- and C-fiber sensory neurons in the trigeminal ganglion (TG) and dorsal root ganglia (DRG) (16, 17). α-CGRP is produced via tissue-specific alternative splicing from the Calcitonin I gene on chromosome 11, that also gives rise to calcitonin (18). First, a pre-mRNA transcript of the Calcitonin I gene is produced, then exon 4 is spliced out and the transcript is translated in a 121 amino acid pro-hormone. Finally, it is cleaved in the mature 37 amino acid polypeptide and stored in dense-core vesicles for transport to axon terminals (19, 20). β-CGRP is found primarily in the enteric nervous system and pituitary gland. It stems from the Calcitonin II gene, also located on chromosome 11 (16, 21).
CGRP can be subdivided into four sections (16, 22, 23): the N-terminus end, consisting of seven amino acids, is a ring-like structure formed by a disulfide bond at amino acid 2 and 7 (16). It is responsible for receptor activation and affinity (23). Amino acids 8–18 form an α-helix, which is responsible for orientation of CGRP and efficient receptor binding (23, 24). Amino acids 19–27 are present as β- or γ-twist (22). Although little is known, this part seems to be involved in receptor binding. The C-terminus (amino acids 28–37) builds the binding epitope and interacts with the N-terminus of the CGRP receptor (16, 25).
The Calcitonin family members bind to G-protein coupled receptors (GPCRs) to exert their actions (26).
The CGRP receptor is a membrane-bound heterodimer comprising the calcitonin receptor-like receptor (CLR) and the receptor activity modifying protein 1 (RAMP1) (16, 27, 28). Further, the two cytosolic proteins, receptor component protein (RCP) and the α-subunit of the GS protein (GαS) belong to the receptor complex. All components are needed to form a functional receptor which is distributed within the peripheral and central nervous system as well as the cardiovascular system (29).
The CLR is a member of the class B “secretin-like” family of G protein-coupled receptors (GPCR). It is structured in seven transmembrane-spanning domains with an extracellular N-terminus and a cytosolic C-terminus. By binding of CGRP to the N-terminus the signaling cascade is initiated (16).
RAMP1 belongs to the RAMP family including RAMP1, RAMP2, and RAMP3. It is specific to the CGRP receptor and consists of one transmembrane-spanning domain with a long extracellular N-terminal domain and a short intracellular C-terminus. It is responsible for high affinity binding of CGRP and receptor trafficking (30, 31).
If the CLR is combined with RAMP2 or RAMP3, respectively, receptors for adrenomedullin or adrenomedullin 2 are formed (32).
RCP is needed for signal transduction, in detail it connects the CLR and the cytosolic G protein-mediated signaling pathway leading to the production of cyclic adenosine monophosphate (cAMP) (2).
After CGRP binding, the receptor is phosphorylated and internalized (2, 26).
The AMY1 receptor formed by the calcitonin receptor (CTR) interacting with RAMP1 is another CGRP receptor (26, 32), however its physiological relevance needs to be determined (28). In vitro, both CGRP and amylin bind to the AMY1 receptor. Due to high potency of CGRP at this receptor and its widespread distribution a physiological role has been hypothesized (27).
The trigeminal nerve, the trigemino-vascular system (TVS) and the trigemino-cervical complex (TCC) play a pivotal role in the generation of migraine pain (5, 33). However, the origin of migraine attacks - whether peripheral or central - remains unclear (3, 34). Recent data suggest a central origin (35, 36), although this is beyond the scope of this review.
Together with the ophthalmic, maxillary and mandibular branches (V1-3), the trigeminal nerve is the largest cranial nerve (V1: 26.000 fibers; V2: 50.000 fibers; V3: 78.000 fibers), responsible for tactile and pain perception of the face and the meninges as well as for motor control of masticatory muscles (37–39).
The ophthalmic division (V1) innervates the upper part of the face, most of the dura mater and cerebral vasculature (see Figure 1) (37, 39, 41). It terminates in the lacrimal, frontal and nasociliary nerve which again give rise to small sensory terminal afferents. Due to the major innervation of intracranial structures by the ophthalmic division, most nociceptive stimuli are conveyed by this branch to the trigeminal ganglion (TG) (39).
Figure 1. CGRP in the trigemino-vascular system. CGRP is released from peripheral afferents of the ophthalmic (V1), mandibular (V2) and maxillary (V3) division of the trigeminal nerve. Different studies showed elevated CGRP levels in saliva and tear fluid in ictal and interictal migraine patients (40). TG; trigeminal ganglion.
The maxillary division (V2) innervates sensitively the mid-part of the face including the upper lip and cheek. Sensitivity of the lower face and motor innervation of the chewing muscles is provided by the mandibular branch (V3) (37, 39).
The trigeminal ganglion (TG) consists of 20.000–150.000 pseudo-unipolar neurons with distal axonal branches forming the abovementioned divisions and a proximal axonal branch reaching the TCC in the brainstem (39, 42). Fifty percent of small- and medium-sized neurons show CGRP immunoreactivity (30, 43) primarily found in sensory neurons and their unmyelinated C-fibers or thinly myelinated Aδ-fibers, not in glial cells. CGRP is commonly colocalized with substance P (SP).
The TG also contains the CGRP receptor, however CGRP and CGRP receptor components are rarely co-expressed (30). CLR and RAMP1 are expressed in 40% of large neurons, satellite glial cells and in the wall of vessels of the TG (30, 44, 45).
TG neurons innervating intracranial vessels store several other neuropeptides like SP, neurokinin A/B, pituitary adenylate cyclase-activating peptide (PACAP), dynorphins, serotonin, amylin and glutamate which are also thought to be involved in migraine pathophysiology (37).
The trigemino-vascular system comprises the trigeminal nerve and its afferents to the intracranial vasculature and the meninges (46, 47). Nociceptive nerve fibers innervate pial, subarachnoid and dural blood vessels. Highest density of trigeminal fibers is found along proximal arteries and decreases in distal vessels, however, it was suggested that small cerebral vessels are also involved in pain (48–50). CGRP is also present in veins, although to a lesser degree. Due to low CGRP levels in blood, it was concluded that the peptide rather acts locally in the vessel wall (16).
Upon activation of the trigeminal system, CGRP and other neuropeptides like SP or PACAP are released from peripheral afferents and subsequently neurogenic inflammation occurs.
Neurogenic inflammation is a neural-driven inflammatory process caused by the release of vasoactive neuropeptides. It is hypothesized to be a key mechanism of migraine pathophysiology (51–54) comprising plasma extravasation and vasodilation leading to nociceptor activation and sensitization (47, 55); Also, activated meningeal nociceptors lead to a release of vasoactive and proinflammatory peptides (55, 56). Nevertheless, the initiation of meningeal inflammation remains unclear. For example, activation by cortical spreading depolarization (CSD) or through the release of inflammatory mediators by mast cells is discussed (3, 4).
Nociceptive signals from the meninges and intracranial vessels are transmitted mainly via the ophthalmic branch (V1) to first-order sensory neurons in the TG. From there, pain signals are conveyed to second-order neurons of the trigemino-cervical complex (TCC) in the brainstem consisting of neurons of the trigeminal nucleus caudalis (TNC) and C1 and C2 dorsal horns of the cervical spinal cord (see Figure 2) (5, 33).
Figure 2. Primary afferents from the meninges and cerebral blood vessels reach the trigeminal ganglion, mostly through the ophthalmic branch (V1). The information is processed via first-order neurons in the TG to second-order neurons in the trigeminal nucleus caudalis forming the trigemino-cervical complex with C1 and C2 dorsal horns of the cervical spinal cord. The TCC projects to different areas in the brainstem (not outlined in this figure) and the thalamus. The activation of the TCC might also activate the trigeminal autonomic reflex. From the thalamus, nociceptive signals are conveyed to (sub-)cortical structures involved in pain perception (16). ACC, anterior cingulate cortex; AMY, amygdala; HYP, hypothalamus; INS, insula; PFC, prefrontal cortex; S1 and S2, somatosensory cortex; SPG, sphenopalatine ganglion; SSN, superior salivary nucleus; TCC, trigemino-cervical complex; TG, trigeminal ganglion; THA, thalamus; TNC, trigeminal nucleus caudalis.
The TCC projects to different areas in the brain stem and the thalamus where nociceptive signals are further processed (4, 5, 33, 57).
From the thalamus, nociceptive signals are projected from third-order neurons to cortical and subcortical structures involved in pain perception (58), but thalamic nuclei are also involved in non-headache symptoms like photo- or phonophobia (33, 59).
In the CNS, pain signals are processed in the so-called pain matrix consisting of the primary and secondary somatosensory cortex, insula, anterior cingulate cortex and prefrontal cortex (4, 58).
In the late 1980s and early 1990s the neurovascular aspect of migraine pathophysiology was brought into focus (60, 61). Further, it became possible to investigate neuropeptides and their role in the innervation of cerebral vasculature and in migraine (62).
First, ictal migraine patients—referring to patients with a migraine headache at the time of study participation- and subsequently other migraine states like interictal or chronic migraine were investigated. These studies established and confirmed the importance of the trigeminal system and the neuropeptide CGRP in the pathophysiology of migraine. To date, only CGRP was reliably detected in migraine patients (6). An overview of studies investigating CGRP in blood, saliva and tear fluid gives Table 1.
In 22 patients with migraine with or without aura blood was collected during acute headache (with a median duration of 3 h) from the external jugular and cubital vein (62). CGRP levels were significantly elevated in migraine patients compared to healthy controls. Interestingly, elevated CGRP levels were only shown in blood drawn from the cranial circulation, but not in peripheral blood. Also, no changes in blood levels of SP, NPY, and VIP were detected.
The importance of the TG was further confirmed in 9 patients undergoing thermocoagulation—a therapeutic procedure to destroy tissue by heat produced by high-frequency electric currents—of the trigeminal ganglion for tic douloureux or atypical facial pain (63).
CGRP levels in the external jugular vein were elevated in patients with facial flushing, but otherwise not. The authors concluded that the activated trigeminal ganglion leads to elevated peptide levels (63).
If CGRP is intended to be used as a biomarker, it needs to serve as an objective disease measurement or indicator of a (patho-)physiological state (64–66). Several studies—especially studies measuring CGRP in the jugular vein- have shown increased CGRP levels during acute migraine and decreased CGRP levels after headache resolution. From these studies, it can be concluded that CGRP is a marker of the migraine attack. However, due to inconsistent findings in the overall studies, standardization of study procedures is needed to draw further conclusions (67–69).
Eight migraine patients treated a migraine attack with up to two doses of subcutaneous sumatriptan 3 mg (70). Blood was drawn from the external jugular vein during headache, before abortive treatment, immediately and 2 h after headache resolution. Six patients completely responded to the treatment and CGRP levels were significantly decreased after resolution of headache (70).
A further study investigated not only ictal, but also interictal CGRP levels in juvenile migraineurs compared to healthy controls (71). For interictal measurements migraine patients had to be headache-free for at least 48 h. During migraine attacks blood was drawn within 2–4 h after onset. Ictal CGRP levels were significantly elevated with maximum CGRP levels 2 h after onset. CGRP levels returned to baseline 2 h after spontaneous resolution, as shown in a subset of patients. Interestingly, no difference in CGRP levels was found in interictal migraine patients compared to controls (71).
CGRP levels were also investigated in experimentally-induced migraine attacks (72, 73). The application of nitroglycerin (NTG) is a common model to evoke a migraine-like headache in migraineurs (74, 75). Fifteen female migraineurs and eight healthy controls received nitroglycerin 0.5 mg sublingual. Cubital blood was drawn before NTG application and 60 and 120 min after beginning of a migraine-like headache. If no headache occurred, blood was drawn 5 and 6 h after drug administration (73).
As described before, an immediate headache occurred in a subset of migraineurs and controls and disappeared spontaneously, but no change in CGRP levels was seen during this headache. A migraine-like headache occurred in 2 of 8 controls and 10 of 15 migraine patients with a mean latency of ~6.5 h and a median intensity of 3.5 on the numerical rating scale (NRS). Migraineurs developing a headache showed significantly higher CGRP levels compared to patients without headache. Again, basal CGRP levels didn't show differences between the study groups.
In a following study, this research group investigated the influence of sumatriptan nasal spray 20 mg on CGRP levels in peripheral blood during an experimental migraine attack (72).
Nineteen female migraine patients developed a migraine-like headache attack after the application of sublingual nitroglycerin 0.5 mg. Cubital blood was drawn 120 min after migraine onset, immediately before and 60 min after sumatriptan application.
Based on the sumatriptan response, two groups were divided: patients (n = 6) who improved at least 30% showed significantly decreased CGRP levels, whereas patients (n = 13) who didn't improve accordingly showed no decrease in CGRP levels.
These study results were confirmed and extended by another study monitoring treatment response after rizatriptan in 20 EM patients (76). During six consecutive migraine attacks, the efficacy of rizatriptan was clinically screened. Ten responder (significant pain reduction within 2 h after rizatriptan intake and no headache recurrence within the next 48 h) and 10 non-responder (no significant reduction in pain intensity within 24 h after rizatriptan intake) were chosen.
During a spontaneous migraine attack, patients reached the headache center within 2 h and the external jugular vein was immediately catheterized. CGRP levels were analyzed at the time of catheterization and 1, 2, 4, 6, and 12 h after triptan administration. Ictal CGRP levels were significantly higher in treatment responder compared to non-responder. Further, CGRP levels were significantly reduced in responder, already after 1 h, but even more after 2 h and stayed at this level during the 12 h observation period. CGRP levels in non-responder didn't change significantly over the course of the migraine attack (76).
In spite of these study results, one study didn't find any CGRP level differences in external jugular or antecubital blood ictally and interictally (77). Patients enrolled in this study called the study team at the beginning of a migraine attack, restrained from taking acute medication and blood was drawn within 60 min after initial contact.
Interictal CGRP levels were investigated when patients were headache and abortive medication-free for 72 h. The study group used 2 CGRP assays, however no ictal and interictal differences in CGRP levels could have been detected.
The aforementioned study results highlighted CGRP as a potential marker of trigeminal activation as the neuropeptide is elevated during migraine attacks and reduced after headache resolution (2). From these study results it can be concluded that the neuropeptide represents different (patho-)physiological states of a migraine attack and can be used as biomarker. Further studies investigated interictal CGRP levels and the possible role of CGRP as a biomarker for migraine itself. Moreover, other and less invasive methods were examined and patient groups were chosen due to headache frequency and abortive medication intake.
In interictal episodic migraine (EM) patients elevated (78–80) as well as unchanged CGRP levels were found in peripheral blood compared to healthy controls (71, 73, 77, 81).
The majority of the studies included migraine patients being headache- and abortive medication-free 72 h prior to blood drawing. No correlation between migraine attack frequency and CGRP levels was found (78).
Different studies detected elevated CGRP levels in peripheral blood of chronic migraine (CM) patients compared to healthy controls (82), but also compared to EM patients (83, 84). The studies used different headache- and medication free periods which makes a comparison of the study results difficult (see Table 1).
However, another study investigating serum CGRP levels didn't find differences in CM patients and healthy controls. Migraine patients were headache-free 24 h prior to investigation (86).
CGRP was analyzed as a potential marker for treatment response in CM patients (85, 92, 93). Eighty-three and, respectively, eighty-one CM patients received at least two injections of OnabotulinumtoxinA (155–195 units) following the PREEMPT protocol (94); treatment responder were defined as patients with a ≥50% reduction of headache episodes and a ≥50% subjective benefit (85) or as moderate (reduction of headache episodes and subjective benefit between 33 and 66%), respectively, excellent responder (reduction of headache episodes and subjective benefit > 66%) (92). CGRP levels were determined before and 1 month after OnabotulinumtoxinA administration.
77%, respectively, 75% of CM patients were considered responder. In both studies, pretreatment CGRP levels were significantly higher in responder compared to non-responder. CGRP levels decreased significantly after 1 month in the responder group, whereas this reduction could not have been detected in non-responder (85).
Due to the innervation of the trigeminal nerve and its ophthalmic, maxillary and mandibular branches other sources for detecting CGRP have been investigated in migraine patients (38, 39).
In general, saliva and tear fluid receive increasing attention as diagnostic fluids and to date, few studies have investigated CGRP in saliva and tear fluid (see Table 1) (95).
Former studies detected CGRP in human tears and changes in these peptide levels are hypothesized to represent (patho-)physiological alterations (96). The eye -more precisely the cornea, conjunctiva, meibomian and lacrimal glands- is innervated by sensory, sympathetic and parasympathetic nerves originating in the TG, the superior cervical and pterygopalatine ganglion, respectively (97–99). The cornea is highly innervated by CGRP-positive fibers from the ophthalmic branch (V1) (98), whereas the CGRP-positive innervation of the meibomian and lacrimal glands seems to be scarce (97, 99). Saliva is mainly produced by the parotid, submandibular and sublingual glands as well as numerous minor glands located in the submucosa of the mouth (100). Salivary glands are controlled by the autonomic nervous system, and to a lesser degree innervated by CGRP-positive fibers that evoke salivary secretion (101–103).
Advantages of the measurement of CGRP in these compartments might be higher neuropeptide concentrations due to direct innervation and a non-invasive and easy sample collection which enables repetitive measurements.
Salivary CGRP levels were first investigated in 15 migraineurs compared to 34 healthy subjects in 1990 (87). Saliva was obtained ictally and interictally when patients had restrained from taking abortive medication for 72 h.
Significantly elevated CGRP levels were detected ictally, whereas lower CGRP levels were detected in interictal migraine patients compared to healthy controls (87).
Stimulated salivary CGRP levels were shown interictally and ictally in five EM patients compared to five healthy controls (88). Patients had to be headache-free 72 h prior to interictal investigation. After rinsing the mouth, saliva production was stimulated using 2% citric acid applied to the tongue. Initial saliva was discarded in order to avoid mixing unstimulated and stimulated saliva and 5 mL saliva was sampled. Saliva collection has been trained in the clinic and was performed independently by patients at home.
As shown before, the intake of sumatriptan 100 mg reduced ictal CGRP levels compared to unmedicated patients. In contrast to the above mentioned study results, interictal CGRP levels were significantly elevated in migraine patients compared to healthy controls. Importantly, no changes in peptide levels were detected between sampling in the clinic and at home (88).
These study results were extended by monitoring CGRP levels over the course of a spontaneous migraine attack in 22 EM patients by the same study group (89).
Compared to baseline CGRP levels, no change in CGRP levels during premonitory phase could have been detected, but during the occurrence of a mild or moderate headache. After intake of rizatriptan and headache resolution salivary CGRP levels were found to be near baseline levels. As shown before, triptan responder showed a significant increase of ictal CGRP levels, whereas non-responder didn't show significant changes in salivary CGRP levels during the migraine attack.
The authors further differentiated two groups: one group (n = 6) showed already elevated CGRP levels during the premonitory phase, sustained during the headache phase. In contrast, the other group (n = 8) showed highest CGRP levels during a moderate headache.
In a recent study salivary CGRP levels were continuously monitored and investigated interictally and ictally (81). Twenty-two EM patients and twenty-two healthy controls were included. For interictal sampling patients had to be headache-free for 72 h, in every participant peripheral blood was drawn interictally once. Saliva was independently sampled by patients and stored at home. Interictal saliva levels were significantly elevated in EM patients compared to healthy controls, whereas no significant difference was detected in CGRP plasma levels. Forty-nine migraine attacks were monitored by taking saliva samples at headache onset, after 2 and 8 h.
Again, ictal CGRP levels were elevated. Dependent on CGRP levels, the authors stated CGRP-independent and CGRP-dependent migraine attacks with significantly higher CGRP levels. Eighty percent of migraine attacks were CGRP-dependent and 20% were CGRP-independent. Relating to patients, 13 of 22 migraine patients showed only CGRP-dependent, 3 of 22 patients showed exclusively CGRP-independent attacks and 6 patients showed both types of migraine attacks. These study results support the above mentioned results as they indicate that several neuropeptides might be involved to different degrees in a migraine attack.
Salivary CGRP levels in chronic migraine is less investigated. One study showed significantly elevated CGRP levels in 33 CM patients compared to 36 healthy controls in resting whole saliva and periperhal blood (82).
To date, one study investigated salivary CGRP levels in 20 CM patients receiving OnabotulinumtoxinA compared to placebo (90). At inclusion, baseline salivary CGRP levels were determined and patients were divided in two study groups: group A received OnabotulinumtoxinA as described in the PREEMPT protocol (94), group B received saline. After 4 months treatment regimens were switched. Patients were instructed to obtain monthly saliva samples. In both study groups, headache days were significantly reduced after treatment, whereas the reduction of headache days was greater after OnabotulinumtoxinA treatment.
In the OnabotulinumtoxinA group, CGRP levels decreased at month 2 and 3, although this change didn't reach significance which the authors ascribe to little patient number. A respective decrease of CGRP levels was not detected after saline.
In our study group, tear fluid CGRP levels were investigated in 48 EM, 45 CM and 48 healthy controls (91). Interictal (no headache and abortive medication in the last 48 h) and ictal migraineurs visiting our outpatient headache center were continuously included. Tear fluid was sampled using a plastic capillary located at the lateral canthus of both eyes. Blood was drawn from the cubital vein.
In general, we found CGRP levels to be about 140× higher in tear fluid compared to plasma levels. Further, tear fluid CGRP levels were significantly elevated in interictal migraine patients compared to healthy controls. No differences in tear fluid CGRP levels could have been detected in episodic and chronic migraine patients. One explanation for this finding might be the high frequency of migraine days in EM patients.
As shown before, ictal migraine patients who had restrained from taking abortive medication 48 h prior to investigation showed highest CGRP levels, although this only showed to be a trend which is likely due to little patient number (n = 13).
Ictal migraine patients with intake of acute medication 48 h prior to investigation showed significantly reduced CGRP levels in tear fluid compared to interictal and unmedicated ictal patients.
Measuring CGRP in migraine patients has led to a better understanding of pain in migraine pathophysiology as well as it laid the foundation of the development of new abortive and prophylactic treatments (40). Further, the neuropeptide is recognized as a marker for the acute migraine attack and it might be a marker for migraine itself which could help to objectify the diagnosis in the future (67).
However, results of CGRP measurement in peripheral blood remain conflicting as well as comparability and reproducibility is often limited (69, 104).
Most probably, the differences in study results are caused by using distinct methods and inhomogeneous study groups (69).
Recent studies used more controlled inclusion and exclusion criteria, e.g., concerning ictal or interictal migraine or monthly headache frequency. Differences between interictal and ictal migraine patients have been shown in several studies and reduced CGRP levels were detected after intake of abortive medication up to 12 h in blood and 48 h in tear fluid (76, 91).
To date, little is known concerning the influence of monthly migraine frequency. There are studies showing increased CGRP levels in CM compared to EM patients (83, 84), however other studies didn't find significant differences (86, 91) or a correlation with number of the headache days (78).
In this respect, the analysis of CGRP levels in chronic migraine might be especially challenging since headache- and medication-free periods are scarce due to ≥15 headache days/month (105).
However, the investigation of rigorous subgroups concerning headache days, associated symptoms or distinct clinical factors, but also comorbidities, age and gender will contribute to our understanding of CGRP. Further, interferences of the above mentioned factors with the peptide could be investigated which might also explain missing comparability of study results (67, 68).
Almost all studies used different study methods concerning blood drawing, processing and analysis as well as many study protocols haven't been sufficiently described. Thus, direct comparison of the studies is not possible and might be one of the most important explanations of different research results as well as limited reproducibility (69). CGRP is rapidly degraded with a short half-life of 7–9 min (106). This rapid degradation was proposed to cause negative study results in studies with longer processing times (104). Preparation of pre-chilled vials, the application of peptidase inhibitors [although the effect of peptidase inhibitors was also questioned (104)] and storage on ice until immediate processing needs to be carefully considered (69, 104). In this respect, the analysis of CGRP in plasma might be beneficial compared to serum.
Also, various analysis methods like radioimmunoassay (RIA), enzyme immunoassay (EIA) or enzyme-linked immunosorbent assay (ELISA) as well as the implementation of these procedures will affect reproducibility (68). This question was recently addressed and suggestions were proposed for standardization of study protocols (69).
After release, the neuropeptide is thought to be taken up by post-capillary veins and can subsequently be detected in the circulation (69). However, CGRP levels are low in blood and dilution needs to be considered (16). This is especially important if blood is taken peripherally with a wide distance from the location of release.
Alternative approaches like CGRP measurement in saliva or tear fluid has been proposed and their potential role in determination of the neuropeptide has been shown (81, 87, 88, 91). Advantages of these methods are higher CGRP concentrations due to direct innervation which might allow to detect even subtle differences in CGRP levels. CGRP-dependent and CGRP-independent migraine attacks as well as different CGRP levels over the course of a migraine attack have been detected in saliva. In the future, this might lead to a better understanding of the contributing neuropeptides or different expression patterns of these in different patient subgroups.
In this respect, the identification of molecule profiles might be an interesting approach for the future (67).
Further, the proposed sampling techniques are easy applicable and even self-administered sampling is possible. As it has already been shown this gives the opportunity to conduct longitudinal studies in real-life conditions and larger patient number might be easier to recruit since these sampling methods are well accepted by participants.
Taken together, the detection of CGRP in migraine patients has enormously enhanced our understanding of migraine pathophysiology and provided new treatments. To enhance this knowledge, higher standardization of study protocols is needed in order to provide better comparability and reproducibility.
The author confirms being the sole contributor of this work and has approved it for publication.
The author declares that the research was conducted in the absence of any commercial or financial relationships that could be construed as a potential conflict of interest.
All claims expressed in this article are solely those of the authors and do not necessarily represent those of their affiliated organizations, or those of the publisher, the editors and the reviewers. Any product that may be evaluated in this article, or claim that may be made by its manufacturer, is not guaranteed or endorsed by the publisher.
1. Charles A. The pathophysiology of migraine: implications for clinical management. Lancet Neurol. (2018) 17:174–82. doi: 10.1016/S1474-4422(17)30435-0
2. Edvinsson L. The CGRP pathway in migraine as a viable target for therapies. Headache J Head Face Pain. (2018) 58:33–47. doi: 10.1111/head.13305
3. Goadsby PJ, Holland PR, Martins-Oliveira M, Hoffmann J, Schankin C, Akerman S. Pathophysiology of migraine: a disorder of sensory processing. Physiol Rev. (2017) 97:553–622. doi: 10.1152/physrev.00034.2015
4. Pietrobon D, Moskowitz MA. Pathophysiology of migraine. Annu Rev Physiol. (2013) 75:365–91. doi: 10.1146/annurev-physiol-030212-183717
5. Chen S-T, Wu J-W. A new era for migraine: the role of calcitonin gene-related peptide in the trigeminovascular system. Prog Brain Res. (2020) 255:123–42. doi: 10.1016/bs.pbr.2020.05.012
6. Edvinsson L. The Trigeminovascular Pathway: Role of CGRP and CGRP Receptors in Migraine. Headache J Head Face Pain. (2017) 57:47–55. doi: 10.1111/head.13081
7. Messlinger K, Fischer MJ, Lennerz JK. Neuropeptide effects in the trigeminal system: pathophysiology and clinical relevance in migraine. Keio J Med. (2011) 60:82–9. doi: 10.2302/kjm.60.82
8. Russo AF. Calcitonin gene-related peptide (CGRP): a new target for migraine. Annu Rev Pharmacol Toxicol. (2015) 55:533–52. doi: 10.1146/annurev-pharmtox-010814-124701
9. Hansen JM, Hauge AW, Olesen J, Ashina M. Calcitonin gene-related peptide triggers migraine-like attacks in patients with migraine with aura. Cephalalgia. (2010) 30:1179–86. doi: 10.1177/0333102410368444
10. Lassen L, Haderslev P, Jacobsen V, Iversen HK, Sperling B, Olesen J, et al. may play a causative role in migraine. Cephalalgia. (2002) 22:54–61. doi: 10.1046/j.1468-2982.2002.00310.x
11. Deng H, Li G-g, Nie H, Feng Y-y, Guo G-y, Guo W-l, et al. Efficacy and safety of calcitonin-gene-related peptide binding monoclonal antibodies for the preventive treatment of episodic migraine–an updated systematic review and meta-analysis. BMC Neurology. (2020) 20:57. doi: 10.1186/s12883-020-01633-3
12. Ha DK, Kim MJ, Han N, Kwak J-H, Baek I-h. Comparative efficacy of oral calcitonin-gene-related peptide antagonists for the treatment of acute migraine: updated meta-analysis. Clin. Drug Investig. (2021) 41:119–32. doi: 10.1007/s40261-020-00997-1
13. Amara SG, Jonas V, Rosenfeld MG, Ong ES, Evans RM. Alternative RNA processing in calcitonin gene expression generates mRNAs encoding different polypeptide products. Nature. (1982) 298:240–4. doi: 10.1038/298240a0
14. Amara SG, Arriza JL, Leff SE, Swanson LW, Evans RM, Rosenfeld MG. Expression in brain of a messenger RNA encoding a novel neuropeptide homologous to calcitonin gene-related peptide. Science. (1985) 229:1094–7. doi: 10.1126/science.2994212
15. Brain SD, Macintyre I, Williams TJ. A second form of human calcitonin gene-related peptide which is a potent vasodilator. Eur J Pharmacol. (1986) 124:349–52. doi: 10.1016/0014-2999(86)90238-4
16. Russell FA, King R, Smillie S-J, Kodji X, Brain S. Calcitonin gene-related peptide: physiology and pathophysiology. Physiol Rev. (2014) 94:1099–142. doi: 10.1152/physrev.00034.2013
17. Iyengar S, Ossipov MH, Johnson KW. The role of calcitonin gene–related peptide in peripheral and central pain mechanisms including migraine. Pain. (2017) 158:543. doi: 10.1097/j.pain.0000000000000831
18. Lou H, Gagel R. Alternative RNA processing–its role in regulating expression of calcitonin/calcitonin gene-related peptide. J Endocrinol. (1998) 156:401–5. doi: 10.1677/joe.0.1560401
19. Gibson S, Polak J, Bloom S, Sabate I, Mulderry P, Ghatei M, et al. Calcitonin gene-related peptide immunoreactivity in the spinal cord of man and of eight other species. J Neurosci. (1984) 4:3101–11. doi: 10.1523/JNEUROSCI.04-12-03101.1984
20. Kumar A, Williamson M, Hess A, DiPette DJ, Potts JD. Alpha-calcitonin gene related peptide: new therapeutic strategies for the treatment and prevention of cardiovascular disease and migraine. Front Physiol. (2022) 131, 122. doi: 10.3389/fphys.2022.826122
21. Brain SD, Grant AD. Vascular actions of calcitonin gene-related peptide and adrenomedullin. Physiol Rev. (2004) 84:903–34. doi: 10.1152/physrev.00037.2003
22. Conner AC, Hay DL, Howitt S, Kilk K, Langel Ü, Wheatley M, et al. Interaction of calcitonin-gene-related peptide with its receptors. Biochem Soc Trans. (2002) 30:451–5. doi: 10.1042/bst0300451
23. Watkins HA, Rathbone DL, Barwell J, Hay DL, Poyner DR. Structure–activity relationships for α-calcitonin gene-related peptide. Br J Pharmacol. (2013) 170:1308–22. doi: 10.1111/bph.12072
24. Howitt SG, Kilk K, Wang Y, Smith DM, Langel U, Poyner DR. The role of the 8-18 helix of CGRP8-37 in mediating high affinity binding to CGRP receptors; coulombic and steric interactions. Br J Pharmacol. (2003) 138:325–32. doi: 10.1038/sj.bjp.0705040
25. Carpenter KA, Schmidt R, von Mentzer B, Haglund U, Roberts E, Walpole C. Turn structures in CGRP C-terminal analogues promote stable arrangements of key residue side chains. Biochemistry. (2001) 40:8317–25. doi: 10.1021/bi0102860
26. Gingell JJ, Hendrikse ER, Hay DL. New insights into the regulation of CGRP-family receptors. Trends Pharmacol Sci. (2019) 40:71–83. doi: 10.1016/j.tips.2018.11.005
27. Hay DL, Garelja ML, Poyner DR, Walker CS. Update on the pharmacology of calcitonin/CGRP family of peptides: IUPHAR Review 25. Br J Pharmacol. (2018) 175:3–17. doi: 10.1111/bph.14075
28. Hay DL, Walker CS. CGRP and its receptors. Headache J Head Face Pain. (2017) 57:625–36. doi: 10.1111/head.13064
29. Walker C, Hay D. CGRP in the trigeminovascular system: a role for CGRP, adrenomedullin and amylin receptors? Br J Pharmacol. (2013) 170:1293–307. doi: 10.1111/bph.12129
30. Eftekhari S, Salvatore CA, Calamari A, Kane SA, Tajti J, Edvinsson L. Differential distribution of calcitonin gene-related peptide and its receptor components in the human trigeminal ganglion. Neuroscience. (2010) 169:683–96. doi: 10.1016/j.neuroscience.2010.05.016
31. McLatchie LM, Fraser NJ, Main MJ, Wise A, Brown J, Thompson N, et al. RAMPs regulate the transport and ligand specificity of the calcitonin-receptor-like receptor. Nature. (1998) 393:333–9. doi: 10.1038/30666
32. Garelja ML, Walker CS, Hay DL. CGRP receptor antagonists for migraine. Are they also AMY1 receptor antagonists? Br J Pharmacol. (2022) 179:454–9. doi: 10.1111/bph.15585
33. Akerman S, Holland PR, Goadsby PJ. Diencephalic and brainstem mechanisms in migraine. Nat Rev Neurosci. (2011) 12:570. doi: 10.1038/nrn3057
34. Ashina M, Katsarava Z, Do TP, Buse DC, Pozo-Rosich P, Özge A, et al. Migraine: epidemiology and systems of care. Lancet. (2021) 397:1485–95. doi: 10.1016/S0140-6736(20)32160-7
35. Schulte LH, May A. Of generators, networks and migraine attacks. Curr Opin Neurol. (2017) 30:241–5. doi: 10.1097/WCO.0000000000000441
36. Schulte LH, Menz MM, Haaker J, May A. The migraineur's brain networks: continuous resting state fMRI over 30 days. Cephalalgia. (2020) 40:1614–21. doi: 10.1177/0333102420951465
37. Edvinsson J, Viganò A, Alekseeva A, Alieva E, Arruda R, De Luca C, et al. The fifth cranial nerve in headaches. J Headache Pain. (2020) 21:1–17. doi: 10.1186/s10194-020-01134-1
38. Terrier LM, Hadjikhani N, Velut S, Magnain C, Amelot A, Bernard F, et al. The trigeminal system: the meningovascular complex—a review. J Anat. (2021) 239:1–11. doi: 10.1111/joa.13413
39. Terrier L-M, Hadjikhani N, Destrieux C. The trigeminal pathways. J Neurol. (2022) 269:3443–60. doi: 10.1007/s00415-022-11002-4
40. Edvinsson L, Haanes KA, Warfvinge K, Krause DN, CGRP. as the target of new migraine therapies—successful translation from bench to clinic. Nat Rev Neurol. (2018) 14:338. doi: 10.1038/s41582-018-0003-1
41. Lee SH, Shin KJ, Koh KS, Song WC. Visualization of the tentorial innervation of human dura mater. J Anat. (2017) 231:683–9. doi: 10.1111/joa.12659
42. Joo W, Yoshioka F, Funaki T, Mizokami K, Rhoton AL, Jr. Microsurgical anatomy of the trigeminal nerve. Clin Anat. (2014) 27:61–88. doi: 10.1002/ca.22330
43. Tajti J, Uddman R, Möller S, Sundler F, Edvinsson L. Messenger molecules and receptor mRNA in the human trigeminal ganglion. J Auton Nerv Syst. (1999) 76:176–83. doi: 10.1016/S0165-1838(99)00024-7
44. Edvinsson L, Ekman R, Jansen I, McCulloch J, Uddman R. Calcitonin gene-related peptide and cerebral blood vessels: distribution and vasomotor effects. J Cereb Blood Flow Metab. (1987) 7:720–8. doi: 10.1038/jcbfm.1987.126
45. Uddman R, Edvinsson L, Ekman R, Kingman T, McCulloch J. Innervation of the feline cerebral vasculature by nerve fibers containing calcitonin gene-related peptide: trigeminal origin and co-existence with substance P. Neurosci Lett. (1985) 62:131–6. doi: 10.1016/0304-3940(85)90296-4
46. Levy D. Migraine pain and nociceptor activation—where do we stand? Headache J Head Face Pain. (2010) 50:909–16. doi: 10.1111/j.1526-4610.2010.01670.x
47. Olesen J, Burstein R, Ashina M, Tfelt-Hansen P. Origin of pain in migraine: evidence for peripheral sensitisation. Lancet Neurol. (2009) 8:679–90. doi: 10.1016/S1474-4422(09)70090-0
48. Fontaine D, Almairac F, Santucci S, Fernandez C, Dallel R, Pallud J, et al. Dural and pial pain-sensitive structures in humans: new inputs from awake craniotomies. Brain. (2018) 141:1040–8. doi: 10.1093/brain/awy005
49. Liu-Chen L-Y, Han D, Moskowitz M. Pia arachnoid contains substance P originating from trigeminal neurons. Neuroscience. (1983) 9:803–8. doi: 10.1016/0306-4522(83)90268-3
50. Macfarlane R, Moskowitz M. The Innervation of Pial Blood Vessels and Their Role in Cerebrovascular Regulation. New York, NY: Brain Ischemia, Springer (1995). p. 247–59.
51. Bernstein C, Burstein R. Sensitization of the trigeminovascular pathway: perspective and implications to migraine pathophysiology. J Clin Neurol. (2012) 8:89–99. doi: 10.3988/jcn.2012.8.2.89
52. Charles A, Nwaobi SE, Goadsby P. Inflammation in migraine… or not…: a critical evaluation of the evidence. Headache J Head Face Pain. (2021) 61:1575–8. doi: 10.1111/head.14224
53. Messlinger K. Migraine: where and how does the pain originate? Exp Brain Res. (2009) 196:179–93. doi: 10.1007/s00221-009-1756-y
54. Raddant AC, Russo AF. Calcitonin gene-related peptide in migraine: intersection of peripheral inflammation and central modulation. Expert Rev Mol Med. (2011) 13:2067. doi: 10.1017/S1462399411002067
55. Levy D, Burstein R, Kainz V, Jakubowski M, Strassman AM. Mast cell degranulation activates a pain pathway underlying migraine headache. Pain. (2007) 130:166–76. doi: 10.1016/j.pain.2007.03.012
56. Levy D. Endogenous mechanisms underlying the activation and sensitization of meningeal nociceptors: the role of immuno-vascular interactions and cortical spreading depression. Curr Pain Headache Rep. (2012) 16:270–7. doi: 10.1007/s11916-012-0255-1
57. Edvinsson L. Tracing neural connections to pain pathways with relevance to primary headaches. Cephalalgia. (2011) 31:737–47. doi: 10.1177/0333102411398152
58. Noseda R, Jakubowski M, Kainz V, Borsook D, Burstein R. Cortical projections of functionally identified thalamic trigeminovascular neurons: implications for migraine headache and its associated symptoms. J Neurosci. (2011) 31:14204–17. doi: 10.1523/JNEUROSCI.3285-11.2011
59. Noseda R, Bernstein CA, Nir R-R, Lee AJ, Fulton AB, Bertisch SM, et al. Migraine photophobia originating in cone-driven retinal pathways. Brain. (2016) 139:1971–86. doi: 10.1093/brain/aww119
60. Ashina M, Terwindt GM, Al-Karagholi A-M, De Boer I, Lee MJ, Hay DL, et al. Migraine: disease characterisation, biomarkers, precision medicine. Lancet. (2021) 397:1496–504. doi: 10.1016/S0140-6736(20)32162-0
61. Goadsby PJ, Holland PR. An update: pathophysiology of migraine. Neurol Clin. (2019) 37:651–71. doi: 10.1016/j.ncl.2019.07.008
62. Goadsby P, Edvinsson L, Ekman R. Vasoactive peptide release in the extracerebral circulation of humans during migraine headache. Ann Neurol. (1990) 28:183–7. doi: 10.1002/ana.410280213
63. Goadsby P, Edvinsson L, Ekman R. Release of vasoactive peptides in the extracerebral circulation of humans and the cat during activation of the trigeminovascular system. Ann Neurol. (1988) 23:193–6. doi: 10.1002/ana.410230214
64. Ferroni P, Barbanti P, Spila A, Fratangeli F, Aurilia C, Fofi L, et al. Circulating biomarkers in migraine: new opportunities for precision medicine. Curr Med Chem. (2019) 26:6191–206. doi: 10.2174/0929867325666180622122938
65. Lipton RB. Inflaming the need for migraine biomarkers. AAN Enterprises. (2014) 83:2198–9. doi: 10.1212/WNL.0000000000001085
66. Pozo-Rosich P, Coppola G, Pascual J, Schwedt TJ. How does the brain change in chronic migraine? Dev Dis Biomark Cephalal. (2021) 41:613–30. doi: 10.1177/0333102420974359
67. Frederiksen SD. Promote biomarker discovery by identifying homogenous primary headache subgroups. Headache J Head Face Pain. (2019) 59:797–801. doi: 10.1111/head.13499
68. Frederiksen SD, Bekker-Nielsen Dunbar M, Snoer AH, Deen M, Edvinsson L. Serotonin and neuropeptides in blood from episodic and chronic migraine and cluster headache patients in case-control and case-crossover settings: a systematic review and meta-analysis. Headache J Head Face Pain. (2020) 60:1132–64. doi: 10.1111/head.13802
69. Messlinger K, Vogler B, Kuhn A, Sertel-Nakajima J, Frank F, Broessner G, et al. measurements in human plasma–a methodological study. Cephalalgia. (2021) 41:1359–73. doi: 10.1177/03331024211024161
70. Goadsby PJ, Edvinsson L. The trigeminovascular system and migraine: studies characterizing cerebrovascular and neuropeptide changes seen in humans and cats. Ann Neurol. (1993) 33:48–56. doi: 10.1002/ana.410330109
71. Gallai V, Sarchielli P, Floridi A, Franceschini M, Codini M, Glioti G, et al. Vasoactive peptide levels in the plasma of young migraine patients with and without aura assessed both interictally and ictally. Cephalalgia. (1995) 15:384–90. doi: 10.1046/j.1468-29821995.1505384.x
72. Juhász G, Zsombok T, Jakab B, Nemeth J, Szolcsányi J, Bagdy G. Sumatriptan causes parallel decrease in plasma calcitonin gene-related peptide (CGRP) concentration and migraine headache during nitroglycerin induced migraine attack. Cephalalgia. (2005) 25:179–83. doi: 10.1111/j.1468-2982.2005.00836.x
73. Juhasz G, Zsombok T, Modos EA, Olajos S, Jakab B, Nemeth J, et al. NO-induced migraine attack: strong increase in plasma calcitonin gene-related peptide (CGRP) concentration and negative correlation with platelet serotonin release. Pain. (2003) 106:461–70. doi: 10.1016/j.pain.2003.09.008
74. Ashina M, Hansen JM, Á Dunga BO, Olesen J. Human models of migraine - short-term pain for long-term gain. Nat Rev Neurol. (2017) 13:713. doi: 10.1038/nrneurol.2017.137
75. Iversen HK, Olesen J, Tfelt-Hansen P. Intravenous nitroglycerin as an experimental model of vascular headache. Basic characteristics. Pain. (1989) 38:17–24. doi: 10.1016/0304-3959(89)90067-5
76. Sarchielli P, Pini LA, Zanchin G, Alberti A, Maggioni F, Rossi C, et al. Clinical-biochemical correlates of migraine attacks in rizatriptan responders and non-responders. Cephalalgia. (2006) 26:257–65. doi: 10.1111/j.1468-2982.2005.01016.x
77. Tvedskov JF, Lipka K, Ashina M, Iversen HK, Schifter S, Olesen J. No increase of calcitonin gene–related peptide in jugular blood during migraine. Ann Neurol. (2005) 58:561–8. doi: 10.1002/ana.20605
78. Ashina M, Bendtsen L, Jensen R, Schifter S, Olesen J. Evidence for increased plasma levels of calcitonin gene-related peptide in migraine outside of attacks. Pain. (2000) 86:133–8. doi: 10.1016/S0304-3959(00)00232-3
79. Fusayasu E, Kowa H, Takeshima T, Nakaso K, Nakashima K. Increased plasma substance P and CGRP levels, and high ACE activity in migraineurs during headache-free periods. Pain. (2007) 128:209–14. doi: 10.1016/j.pain.2006.09.017
80. Rodríguez-Osorio X, Sobrino T, Brea D, Martínez F, Castillo J, Leira R. Endothelial progenitor cells: a new key for endothelial dysfunction in migraine. Neurology. (2012) 79:474–9. doi: 10.1212/WNL.0b013e31826170ce
81. Alpuente A, Gallardo VJ, Asskour L, Caronna E, Torres-Ferrus M, Pozo-Rosich P. Salivary CGRP can monitor the different migraine phases: CGRP (in) dependent attacks. Cephalalgia. (2021) 42:03331024211040467. doi: 10.1177/03331024211040467
82. Jang MU, Park JW, Kho HS, Chung SC, Chung JW. Plasma and saliva levels of nerve growth factor and neuropeptides in chronic migraine patients. Oral Dis. (2011) 17:187–93. doi: 10.1111/j.1601-0825.2010.01717.x
83. Cernuda-Morollón E, Larrosa D, Ramón C, Vega J, Martínez-Camblor P, Pascual J. Interictal increase of CGRP levels in peripheral blood as a biomarker for chronic migraine. Neurology. (2013) 81:1191–6. doi: 10.1212/WNL.0b013e3182a6cb72
84. Pérez-Pereda S, Toriello-Suárez M, Ocejo-Vinyals G, Guiral-Foz S, Castillo-Obeso J, Montes-Gómez S, et al. Serum CGRP, VIP, and PACAP usefulness in migraine: a case–control study in chronic migraine patients in real clinical practice. Mol Biol Rep. (2020) 47:7125–38. doi: 10.1007/s11033-020-05781-0
85. Cernuda-Morollón E, Ramón C, Martínez-Camblor P, Serrano-Pertierra E, Larrosa D, Pascual J. OnabotulinumtoxinA decreases interictal CGRP plasma levels in patients with chronic migraine. Pain. (2015) 156:820–4. doi: 10.1097/j.pain.0000000000000119
86. Lee MJ, Lee S-Y, Cho S, Kang E-S, Chung C-S. Feasibility of serum CGRP measurement as a biomarker of chronic migraine: a critical reappraisal. J Headache Pain. (2018) 19:53. doi: 10.1186/s10194-018-0883-x
87. Nicolodi M, Bianco ED. Sensory neuropeptides (substance P, calcitonin gene-related peptide) and vasoactive intestinal polypeptide in human saliva: their pattern in migraine and cluster headache. Cephalalgia. (1990) 10:39–50. doi: 10.1046/j.1468-2982.1990.1001039.x
88. Bellamy JL, Cady RK, Durham PL. Salivary levels of CGRP and VIP in rhinosinusitis and migraine patients. Headache J Head Face Pain. (2006) 46:24–33. doi: 10.1111/j.1526-4610.2006.00294.x
89. Cady RK, Vause CV, Ho TW, Bigal ME, Durham PL. Elevated saliva calcitonin gene-related peptide levels during acute migraine predict therapeutic response to rizatriptan. Headache J Head Face Pain. (2009) 49:1258–66. doi: 10.1111/j.1526-4610.2009.01523.x
90. Cady R, Turner I, Dexter K, Beach M, Cady R, Durham P. An exploratory study of salivary calcitonin gene-related peptide levels relative to acute interventions and preventative treatment with onabotulinumtoxin A in chronic migraine. Headache J Head Face Pain. (2014) 54:269–77. doi: 10.1111/head.12250
91. Kamm K, Straube A, Ruscheweyh R. Calcitonin gene-related peptide levels in tear fluid are elevated in migraine patients compared to healthy controls. Cephalalgia. (2019) 39:1535–43. doi: 10.1177/0333102419856640
92. Cernuda-Morollón E, Martínez-Camblor P, Ramón C, Larrosa D, Serrano-Pertierra E, Pascual J, et al. and VIP levels as predictors of efficacy of Onabotulinumtoxin type A in chronic migraine. Headache J Head Face Pain. (2014) 54:987–95. doi: 10.1111/head.12372
93. Domínguez C, Vieites-Prado A, Pérez-Mato M, Sobrino T, Rodríguez-Osorio X, López A, et al. CGRP and PTX3 as predictors of efficacy of Onabotulinumtoxin type a in chronic migraine: an observational study. Headache J Head Face Pain. (2018) 58:78–87. doi: 10.1111/head.13211
94. Blumenfeld A, Silberstein SD, Dodick DW, Aurora SK, Turkel CC, Binder WJ. Method of injection of onabotulinumtoxinA for chronic migraine: a safe, well-tolerated, and effective treatment paradigm based on the PREEMPT clinical program. Headache J Head Face Pain. (2010) 50:1406–18. doi: 10.1111/j.1526-4610.2010.01766.x
95. Jasim H, Carlsson A, Hedenberg-Magnusson B, Ghafouri B, Ernberg M. Saliva as a medium to detect and measure biomarkers related to pain. Sci Rep. (2018) 8:1–9. doi: 10.1038/s41598-018-21131-4
96. Golebiowski B, Chao C, Stapleton F, Jalbert I. Corneal nerve morphology, sensitivity, and tear neuropeptides in contact lens wear. Optom Vision Sci. (2017) 94:534–42. doi: 10.1097/OPX.0000000000001063
97. Bründl M, Garreis F, Schicht M, Dietrich J, Paulsen F. Characterization of the innervation of the meibomian glands in humans, rats and mice. Ann Anat Anatom Anzeiger. (2021) 233:151609. doi: 10.1016/j.aanat.2020.151609
98. Müller LJ, Marfurt CF, Kruse F, Tervo TM. Corneal nerves: structure, contents and function. Exp Eye Res. (2003) 76:521–42. doi: 10.1016/S0014-4835(03)00050-2
99. Seifert P, Stuppi S, Spitznas M, Weihe E. Differential distribution of neuronal markers and neuropeptides in the human lacrimal gland. Graefe's Arch Clin Exp Ophthalmol. (1996) 234:232–40. doi: 10.1007/BF00430415
100. Proctor GB, Carpenter GH. Regulation of salivary gland function by autonomic nerves. Auton Neurosci. (2007) 133:3–18. doi: 10.1016/j.autneu.2006.10.006
101. Hauser-Kronberger C, Albegger K, Saria A, Hacker G. Neuropeptides in human salivary (submandibular and parotid) glands. Acta Otolaryngol. (1992) 112:343–8. doi: 10.1080/00016489.1992.11665430
102. Sato Y, Itoh H, Suzuki Y, Tatsuta R, Takeyama M. Effect of pilocarpine on substance P and calcitonin gene-related peptide releases correlate with salivary secretion in human saliva and plasma. J Clin Pharm Ther. (2013) 38:19–23. doi: 10.1111/jcpt.12011
103. Van Oosterhout W, Schoonman G, Garrelds I, Danser A, Chan K, Terwindt G, et al. A human capsaicin model to quantitatively assess salivary CGRP secretion. Cephalalgia. (2015) 35:675–82. doi: 10.1177/0333102414553824
104. Edvinsson L, Ekman R, Goadsby PJ. Measurement of vasoactive neuropeptides in biological materials: problems and pitfalls from 30 years of experience and novel future approaches. Cephalalgia. (2010) 30:761–6. doi: 10.1177/0333102409351807
105. Olesen J. Headache classification committee of the international headache society (IHS) the international classification of headache disorders, asbtracts. Cephalalgia. (2018) 38:1–211. doi: 10.1177/0333102417738202
Keywords: migraine, headache, calcitonin gene-related peptide, neuropeptide, trigeminal system
Citation: Kamm K (2022) CGRP and Migraine: What Have We Learned From Measuring CGRP in Migraine Patients So Far? Front. Neurol. 13:930383. doi: 10.3389/fneur.2022.930383
Received: 27 April 2022; Accepted: 21 June 2022;
Published: 27 July 2022.
Edited by:
Xiao-Yan Chen, Chinese PLA General Hospital, ChinaReviewed by:
Lars Edvinsson, Lund University, SwedenCopyright © 2022 Kamm. This is an open-access article distributed under the terms of the Creative Commons Attribution License (CC BY). The use, distribution or reproduction in other forums is permitted, provided the original author(s) and the copyright owner(s) are credited and that the original publication in this journal is cited, in accordance with accepted academic practice. No use, distribution or reproduction is permitted which does not comply with these terms.
*Correspondence: Katharina Kamm, a2F0aGFyaW5hLmthbW1AbWVkLnVuaS1tdWVuY2hlbi5kZQ==
Disclaimer: All claims expressed in this article are solely those of the authors and do not necessarily represent those of their affiliated organizations, or those of the publisher, the editors and the reviewers. Any product that may be evaluated in this article or claim that may be made by its manufacturer is not guaranteed or endorsed by the publisher.
Research integrity at Frontiers
Learn more about the work of our research integrity team to safeguard the quality of each article we publish.